DOI:
10.1039/D0FO01109F
(Paper)
Food Funct., 2020,
11, 6446-6457
Contributions of viscosity and friction properties to oral and haptic texture perception of iced coffees
Received
29th April 2020
, Accepted 28th June 2020
First published on 29th June 2020
Abstract
Creaminess is affected by bulk properties (i.e. viscosity) and surfaces properties (i.e. friction). This study aimed (i) to assess contributions of viscosity and friction properties to creaminess, thickness and slipperiness perception; and (ii) to compare oral and haptic thickness and slipperiness perception of iced coffees. Three iced coffees differing in viscosity and friction properties were prepared: low viscosity – high friction (LV-HF); low viscosity – low friction (LV-LF) and high viscosity – low friction (HV-LF) iced coffee. Viscosity of iced coffees was adjusted by addition of maltodextrin, and viscosity of HV-LF was 2.5 times higher than that of LV-HF and LV-LF (10 vs. 4 mPa s at 100 s−1). Friction coefficients of LV-LF were reduced by addition of polyethylene glycol (PEG, Mw 6000), and were up to 25% lower than those of LV-HF. Forty-seven untrained panellists (18–27 years) performed two-alternative forced choice (2-AFC) and rank-rating tests to compare creaminess by oral assessment, and thickness and slipperiness by oral and haptic assessment. Results from 2-AFC and rank-rating congruently showed that HV-LF was creamier, thicker and more slippery than LV-HF and LV-LF, both orally and haptically. LV-LF was orally perceived as less creamy and less thick, but haptically as more slippery than LV-HF. Creaminess was more strongly correlated to thickness than to slipperiness. Oral and haptic evaluation of thickness was congruent, whereas differences between oral and haptic slipperiness evaluation were product-dependent. We conclude that increasing viscosity enhances creaminess, whereas increasing lubrication is not necessarily sufficient to increase creaminess in iced coffees.
1. Introduction
Creaminess is a desired mouthfeel property in foods1,2 and can generally be enhanced by increasing fat content.3–5 However, nowadays the food industry aims to reduce the fat content of foods while maintaining desired mouthfeel properties. More specifically, retaining creaminess in low-fat foods poses a challenge. Several ingredients have been suggested to mimic fat in terms of mouthfeel perception, such as modified starches,6 inulin,7,8 microbubbles9 and microparticulated whey protein.10,11 Nonetheless, no substitute has yet been found that can provide creaminess similar to that of fat.12 A better understanding of the mechanisms underlying creaminess perception and the physical-chemical food properties contributing to creaminess may help to develop fat-reduced foods with desirable sensory properties.
Increasing the complexity of tongue movements during oral processing enhances creaminess perception of semi-solids.13 The fact that a combination of tongue movements is required for optimal creaminess perception suggests that creaminess of foods is affected by more than one single food property. During oral processing, a shift from the rheological domain to the tribological domain occurs.14,15 Bulk rheological properties such as viscosity dominate the beginning of oral processing, and are related to thickness perception.4,16–18 Tribological properties are important during subsequent stages of oral processing, when the film between tongue and palate becomes thinner. Tribology is the study of the wear, friction and lubrication of interacting surfaces in relative motion.15 As foods are subjected to friction due to squeezing and pressing between tongue and palate, tribology provides a tool to elucidate sensory perception of mouthfeel attributes by linking friction to sensory properties. Tribological properties have, for instance, been linked to slipperiness perception of guar gum solutions19 and particle dispersions.20 In summary, both rheological and tribological food properties contribute to creaminess.12,21,22
Increasing the viscosity of a liquid food can enhance its creaminess, as more viscous foods are generally perceived to be creamier.23 One of the earliest works on creaminess perception was performed by Wood,24 who concluded that soups require a viscosity of at least 50 mPa s in order to be perceived as creamy. Another study on model soups confirmed that creaminess increased when the viscosity of soups increased.25 In two studies on o/w emulsions, Akhtar and colleagues4,18 observed that increased viscosity by addition of hydrocolloids resulted in enhanced creaminess. Similarly, van Aken et al.26 increased o/w emulsion viscosity by addition of gum arabic, which resulted in higher creaminess ratings according to a trained panel. Furthermore, Janhøj et al.27 found that creaminess of acidified milk drinks increased with increasing viscosity.
In addition to viscosity, friction properties are increasingly recognised to contribute to creaminess of liquid foods.12,22,28,29 The majority of studies that investigated the effect of friction on texture perception modified friction properties by altering either fat or protein content. For instance, reduced friction due to increased fat or protein content resulted in higher creaminess in milks,8,30 custards31 and yoghurts.32 Moreover, a negative correlation between friction and perceived smoothness was found in o/w emulsions with varying oil content.33 Laguna et al.34 demonstrated that discrimination between fat-free and full-fat dairy products was mainly based on differences in tribological properties. By varying the fat or protein content, these studies did not only alter friction properties, but rheological properties of the foods as well. Increasing the fat or protein content reduced friction, but at the same time increased viscosity, two parameters that both have a positive effect on creaminess. Hence, strictly speaking, from these studies one cannot assess the individual, relative contributions of viscosity and friction properties to perception of texture attributes such as creaminess.
Texture can be evaluated by different approaches, such as visually, haptically or orally. Shama and colleagues35,36 compared oral and non-oral methods for viscosity evaluation and concluded that stimuli used for such evaluations depend on the evaluation method employed. Correspondingly, liquids were perceived as thicker when evaluated by stirring with a spatula, compared to stirring with the index finger.37 Christensen & Casper38 found that solutions thickened by sodium alginate were perceived to be thicker when assessed orally compared to visual or haptic evaluation. In a study on o/w emulsions, oral and haptic smoothness scores were generally similar, but emulsions were better discriminated by oral smoothness.33 Differences between oral and haptic texture perception might be caused by differences in the mechanoreceptors involved in sensing texture. Mechanoreceptors in the human skin and oral cavity comprise slowly adapting (SA) and rapidly adapting (RA) receptors.39,40 The majority of mechanoreceptors on the tongue and finger tips consists of RA receptors,40,41 whereas SA receptors are more predominant in other parts of the human body.42–44 Although SA and RA receptors have been found in similar proportions and function similarly in the oral cavity and the finger tips, mechanoreceptors on the tongue have been found to be more sensitive to force.40,45
While it is well known that rheological and tribological properties influence texture perception of foods, less is known about the relative contributions of rheological and tribological properties to texture perception of attributes such as creaminess. Therefore, the objective of this study was to better understand the effects of viscosity and friction properties on oral and haptic texture perception by varying viscosity of iced coffees with minimal changes in friction properties and vice versa. Viscosities of iced coffees were varied by adding maltodextrin, whereas friction properties were adjusted by adding polyethylene glycol (PEG), a food grade polymer (E1521) that can be used as a lubricant in foods without considerably changing viscosity.46 Especially high molecular weight PEG (Mw >1
000
000 g mol−1) can reduce friction in aqueous solutions considerably without strongly affecting viscosity. Legislation limits the use of PEG in foods to low molecular weight PEG (Mw < 10
000 g mol−1). The goal of this study was (i) to assess contributions of viscosity and friction properties to creaminess, thickness and slipperiness perception of iced coffees; and (ii) to compare oral and haptic thickness and slipperiness perception of these beverages.
2. Materials & methods
2.1. Product preparation
Three iced coffees differing in viscosity and friction properties were prepared: low viscosity – high friction (LV-HF); low viscosity – low friction (LV-LF); and high viscosity – low friction (HV-LF) iced coffee (Table 2). Products were prepared from commercially available ready-to-drink milk-based iced coffee (20% coffee; 0.9% fat (w/v); Koffiecino, Holland Foodz, the Netherlands). Viscosity of HV-LF was adjusted by adding 30 wt% maltodextrin (Fantomalt, Nutricia, Danone, France), and friction properties of LV-LF were adjusted by adding 7 wt% polyethylene glycol (PEG (E-1521); Mw 6000, Merck, Germany). No PEG was added to HV-LF, as the increase in viscosity due to maltodextrin addition was accompanied by a decrease in friction properties. Since addition of PEG to LV-LF resulted in a small increase in viscosity, 16.8 wt% maltodextrin was added to LV-HF to obtain matching shear viscosities. Iced coffees were mixed with the ingredients for 30 min at room temperature to ensure dissolution of maltodextrin and PEG. Products were freshly prepared each day and stored in the refrigerator at 4 °C until 1 h before use.
2.2. Characterisation of viscosity and friction properties
Flow curves of the iced coffees were determined using an MCR 302 rheometer equipped with a double gap concentric cylinder geometry (DG26.7/Ti, Anton Paar, Austria). Flow curves were measured while increasing the shear rate from 0.1 to 1000 s−1 in 50 logarithmic steps in 10 min. Measurements were performed in triplicate at 35 °C.
Friction properties of the three iced coffees were characterised using a ball-on-three-pins set-up in an MCR 302 rheometer (Anton Paar, Austria). A glass ball was used to represent the human palate, whereas cylindrical shaped polydimethylsiloxane (PDMS) pins were used to mimic the hardness and surface of the human tongue. One mL of sample was transferred to the sample holder. Each test consisted of three consecutive runs of 10 min in which sliding speeds were increased from 0.0001–2200 rpm (equivalent to 4 × 10−5–103 mm s−1) using a logarithmic ramp, while a normal force of 1 N was applied. Friction coefficients were obtained as the ratio of the frictional force divided by the normal load. Runs were separated by 5 min breaks in which a normal force of 1 N was applied. Data from the second run was used for analysis. Tests were conducted in triplicate at 35 °C to simulate the oral environment. Prior to measuring iced coffees, PDMS surfaces were run-in by (i) one run with 1 mL demineralised water and (ii) one run with 1 mL plain iced coffee.
For measures of friction properties of the iced coffees in the presence of saliva, unstimulated saliva was collected over 15 minutes from one healthy volunteer after a fasting period of 60 min based on the protocol of Silletti et al.47 After rinsing the mouth with water, saliva was collected with closed lips and expectorated in a plastic tube. Saliva was centrifuged at 956g for 10 min to remove cellular debris and was subsequently kept on ice. Saliva was mixed with iced coffee (ratio 1
:
1) just before addition to the sample holder. The same experimental conditions were used as for the tribological measurements of the iced coffees without saliva. Data from the second run was used for analysis. Tests were conducted in triplicate at 35 °C to simulate the oral environment. Prior to measuring the saliva-iced coffee mixtures, PDMS surfaces were run-in by (i) one run with 1 mL demineralised water and (ii) three runs with 1 mL saliva.
2.3. Participants
Dutch-speaking participants were recruited from the surroundings of Wageningen using flyers and social media. Individuals were eligible for participation in the study when they were between 18–35 years and had a BMI between 18.5–25 kg m−2. Individuals were excluded from participation when they had problems with general or oral health, when they did not have normal smell and taste function or when they had mastication or swallowing disorders. Individuals with food allergies or intolerances for milk products and pregnant or breastfeeding women were excluded from participation. A total of 50 subjects agreed on participation in the study, of which n = 47 (8 male, 39 female) completed all test sessions. Mean age was 21.5 (±2.2) years and mean BMI was 21.7 (±1.7) kg m−2. Most participants were frequent coffee drinkers, as 47% consumed coffee daily and 31% consumed coffee multiple times a week. Participants signed an informed consent and completed a general questionnaire in the first test session. Participants received financial reimbursement after completion of the three test sessions.
2.4. Sensory evaluation
Participants (n = 47) completed three test sessions on separate days. In each test session, two sensory methods were used: the 2-alternative forced choice method (2-AFC) followed by rank-rating, both of which are described in more detail below. A test session lasted approximately 30 min. During each test session only one of the following sensory texture attributes was evaluated: creaminess, thickness or slipperiness. The order in which participants assessed each of the three attributes was randomised. In test sessions in which participants evaluated creaminess, the 2-AFC method and rank-rating were performed orally by tasting the iced coffees. In test sessions in which thickness or slipperiness of the iced coffees were evaluated, the two sensory methods were first performed orally by tasting, followed by performing the same two sensory methods by haptic evaluation in hand. Haptic assessment was performed by rubbing the iced coffee between the fingers. Creaminess was not evaluated haptically, as we assumed that a meaningful evaluation of creaminess by hand is not possible.
To become acquainted with the attributes to be evaluated, participants received two reference products that represented products low and high in the respective attribute. Subsequently, an example question was presented to familiarise participants with the sensory method and the products used in the study. For the example question, two of the actual products (LV-HF, LV-LF or HV-LF) were presented to the participant. Definitions of the attributes and instructions were provided to the participants (Table 1).
Table 1 Definitions and instructions of attributes (translated into English from Dutch) and reference products
|
|
Definition/instruction |
Reference products |
Low intensity |
High intensity |
Oral |
Creaminess |
“The degree to which you experience a silky, rich, full feeling in your mouth” |
Skim milk |
Full-fat milk |
Thickness |
“How easily does the product flow in your mouth?” |
Plain iced coffee |
Iced coffee + 50% maltodextrin |
Slipperiness |
“How easily does your tongue move over your palate when you consume the product?” |
Water |
Olive oil |
|
Haptic |
Thickness |
“Evaluate the thickness of the product by rubbing a small amount between thumb and index finger” |
Plain iced coffee |
Iced coffee + 50% maltodextrin |
Slipperiness |
“Evaluate the slipperiness of the product by rubbing a small amount between thumb and index finger” |
Water |
Aqueous PEG solution (Mw 4 × 106, 1.0 g L−1) |
Participants were seated in individual sensory booths with standard white light and were asked to refrain from drinking coffee two hours prior to the test session. Participants wore nose clips during the entire session, as this facilitated focusing on texture attributes only and limited the perception of off-flavours caused by addition of PEG to LV-LF. Iced coffees (15–20 mL) were presented in 30 mL transparent plastic cups labelled with random 3-digit numbers. Participants were asked to expectorate the product after evaluation, and to rinse their mouth after tasting each set of iced coffees. Crackers and water were provided for palate cleansing between the product pairs. For sessions in which attributes were assessed haptically, participants used tap water and tissues to clean their hands. Data was collected in Dutch using EyeQuestion® (Logic8, the Netherlands).
2.4.1. 2-Alternative forced choice method (2-AFC).
In each test session, the three iced coffees were first evaluated by using the 2-alternative forced choice method (2-AFC). Each of the iced coffees was compared with both other products using three 2-AFC tests. All comparisons were performed in duplicate within one session. The order of pairs and of products within pairs was randomised among participants. For each pair of iced coffees, participants were asked to determine which of the two products was more intense in the attribute of interest (i.e. ‘which of the two products is creamier?’). Participants were allowed to re-taste products if desired and were forced to guess if no difference could be detected.
2.4.2 Rank-rating.
In each test session, evaluation using the 2-AFC method was followed by a rank-rating test. The rank-rating test was performed to obtain an estimate of the perceived differences between the three iced coffees. In the rank-rating test the three iced coffees were presented simultaneously. Participants placed the products on an unstructured 100 mm line scale that represented the attribute of interest, anchored from “not at all” on the left to “extremely” on the right. The order of products within the set was randomised among participants, and participants were asked to evaluate the products from left to right. Participants were allowed to re-taste the products if desired.
2.5. Data analysis
For the 2-AFC method, percentages of products chosen to be more intense than their counterpart were determined, and the Bradley–Terry model was applied to the multiple paired comparisons data.48 Results from rank-rating were reported as mean values with standard error. A repeated measures ANOVA with participants as random factor was performed on rank-rating scores of each attribute to determine significant differences between the three iced coffees. Two-way repeated measures ANOVAs were performed to test for significant differences between haptic and oral rank-rating scores of thickness and slipperiness. Pearson correlation coefficients were determined between the sensory attributes using pooled rank-rating scores of the three iced coffees. Data was analysed using RStudio (version 3.5.2) and a significance level of α = 0.05 was used.
3. Results
3.1. Viscosity and friction properties
Mean shear viscosities (±SD) of the three iced coffees at 35 °C are shown in Fig. 1. Flow curves of LV-HF and LV-LF overlap, whereas the viscosity of HV-LF is approximately 2.5 times higher within this range of shear rates (Table 2). Mean friction coefficients (±SD) of the three iced coffees as a function of sliding speed are displayed in Fig. 2 in absence (a) and presence (b) of saliva. In both conditions (with and without saliva), highest friction coefficients were observed for LV-HF for nearly the entire sliding speed range. Addition of PEG (LV-LF) led to a reduction in friction coefficient relative to LF-HF in presence and absence of saliva, without affecting viscosity (Fig. 1). Addition of 30 wt% maltodextrin to iced coffee resulted in an increase in viscosity (Fig. 1) and a decrease in friction coefficient (Fig. 2, Table 2). In the presence of saliva, boundary friction was generally lower, and the mixed regime shifted towards higher sliding speeds (Fig. 2b). Moreover, differences in friction between the three products became smaller in the presence of saliva.
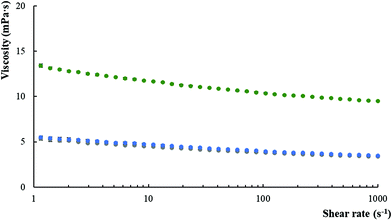 |
| Fig. 1 Flow curves of the three iced coffees (35 °C). LV-HF = grey; LV-LF = blue; HV-LF = green. LV-HF denotes low viscosity – high friction, LV-LF low viscosity – low friction and HV-LF high viscosity – low friction iced coffee. Error bars represent standard deviation of triplicate measures. | |
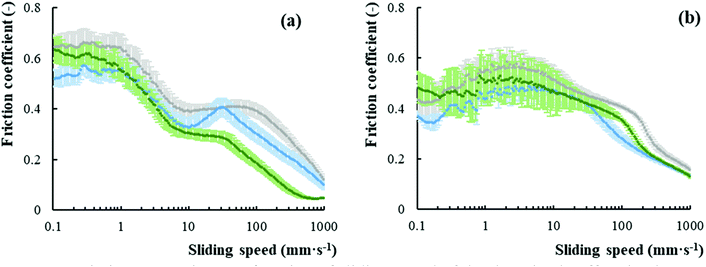 |
| Fig. 2 Friction properties as a function of sliding speed of the three iced coffees in absence (a) and presence (b) of saliva. LV-HF = grey; LV-LF = blue; HV-LF = green. LV-HF denotes low viscosity – high friction, LV-LF low viscosity – low friction and HV-LF high viscosity – low friction iced coffee. Error bars represent the standard deviation of triplicate (a) or duplicate (b) measures. | |
Table 2 Overview of composition (wt% maltodextrin (MD) and wt% PEG), viscosity (mean η (±SD) at 1, 10 and 100 s−1 at 35 °C) and friction properties (mean friction coefficient μ (±SD) in absence of saliva at 35 °C) of the three iced coffees. Friction coefficients at 1 mm s−1 (boundary regime), 10 mm s−1 and 100 mm s−1 (mixed regime), and exponent b (slope of curve in the mixed regime between 100–1000 mm s−1) are displayed. LV-HF denotes low viscosity – high friction, LV-LF low viscosity – low friction and HV-LF high viscosity – low friction iced coffee
|
wt% MD |
wt% PEG |
Shear viscosity η (mPa s) |
Friction coefficient μ |
1 s−1 |
10 s−1 |
100 s−1 |
1 mm s−1 |
10 mm s−1 |
100 mm s−1 |
Exponent b |
LV-HF |
16.8 |
— |
5.4 ± 0.1 |
4.6 ± 0.1 |
3.9 ± 0.0 |
0.63 ± 0.05 |
0.39 ± 0.02 |
0.39 ± 0.03 |
−0.494 |
LV-LF |
— |
7.0 |
5.6 ± 0.2 |
4.7 ± 0.1 |
4.0 ± 0.0 |
0.56 ± 0.05 |
0.32 ± 0.04 |
0.30 ± 0.03 |
−0.457 |
HV-LF |
30.0 |
— |
13.3 ± 0.1 |
11.8 ± 0.1 |
10.5 ± 0.1 |
0.55 ± 0.04 |
0.31 ± 0.02 |
0.18 ± 0.02 |
−0.699 |
3.2. Paired comparisons
The frequency of selection of iced coffees being perceived as more intense than their counterpart was obtained from 2-AFC tests (Fig. 3). HV-LF was consistently chosen as more intense for all attributes when compared with LV-HF and LV-LF iced coffees. This implies that HV-LF iced coffee was, both orally and haptically, perceived to be thicker, more slippery and creamier than the LV-HF and the LV-LF iced coffees. LV-HF was orally perceived to be thicker and creamier than LV-LF. Concerning haptic evaluation of slipperiness, LV-LF was found to be more slippery than LV-HF. There were no significant differences between LV-HF and LV-LF iced coffees regarding haptic thickness and oral slipperiness.
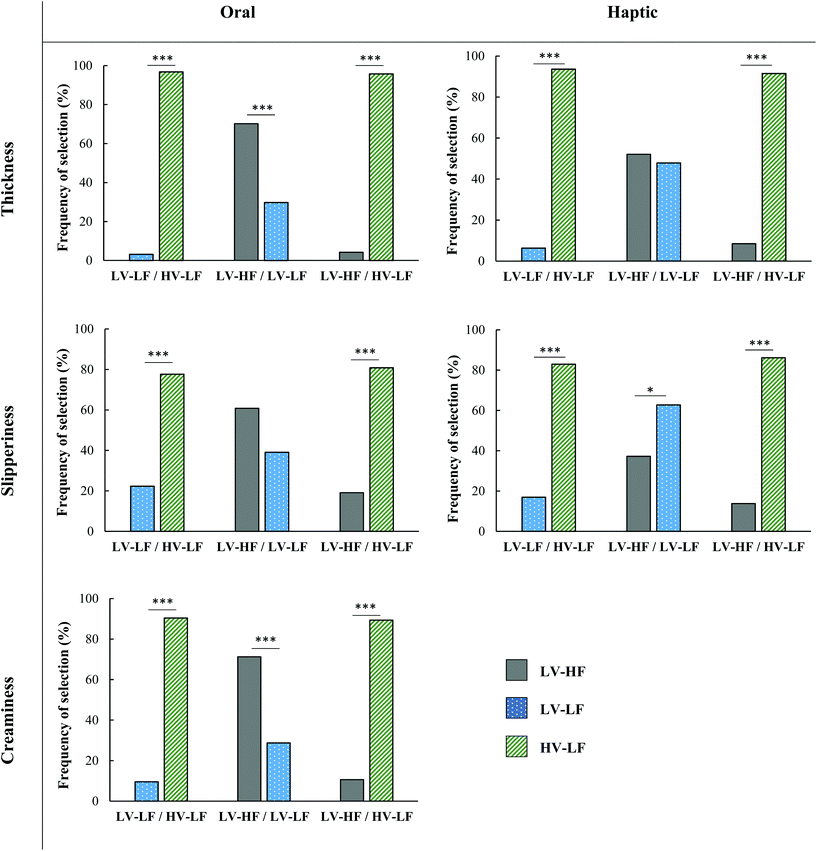 |
| Fig. 3 2-Alternative forced choice comparisons (2-AFC): frequency of selection of iced coffees chosen to be more intense in oral and haptic thickness, oral and haptic slipperiness, and oral creaminess (n = 47, n = 46 for oral slipperiness comparisons of LV-HF and LV-LF; all in duplicate). LV-HF denotes low viscosity – high friction, LV-LF low viscosity – low friction and HV-LF high viscosity – low friction iced coffee. Asterisks indicate statistically significant differences: (*) p < 0.05; (***) p < 0.001. | |
When comparing oral evaluation of thickness and slipperiness with those from haptic evaluation, results are consistent for paired comparisons that included HV-LF iced coffee. In oral and haptic assessment of both thickness and slipperiness, HV-LF was perceived as thicker and more slippery than LV-HF and LV-LF. However, oral and haptic thickness were not evaluated similarly for paired comparisons between LV-HF and LV-LF. No significant difference in thickness was found between the two iced coffees during haptic assessment, while LV-HF was perceived to be thicker when assessed orally. Regarding slipperiness perception, no significant difference was found between LV-HF and LV-LF during oral assessment, whereas LV-LF was found to be more slippery when assessed haptically.
3.3. Perceived intensities (rank-rating)
Mean perceived intensities of the attributes obtained from rank-rating are shown in Fig. 4. Results from rank-rating are generally in agreement with the results obtained from paired comparisons. HV-LF was perceived to be higher in intensity compared to LV-HF and LV-LF for all texture attributes. No significant differences were found between LV-HF and LV-LF in terms of haptic thickness and oral slipperiness. LV-LF was orally perceived as less creamy and less thick, but haptically more slippery than LV-HF.
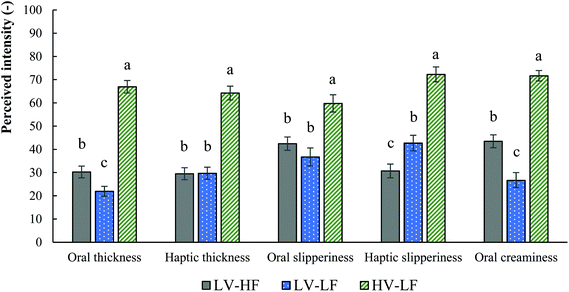 |
| Fig. 4 Mean perceived intensities (±SEM) of oral and haptic thickness, oral and haptic slipperiness and oral creaminess of LV-HF, LV-LF and HV-LF iced coffees (n = 47, except for oral creaminess (n = 46)). LV-HF denotes low viscosity – high friction, LV-LF low viscosity – low friction and HV-LF high viscosity – low friction iced coffee. Within each attribute, statistically significant differences are present between the iced coffees (p < 0.001). Products containing the same letter are not significantly different from each other. | |
Thickness perception was not affected by evaluation method (oral vs. haptic; p = 0.46), but a main effect of product was found (LV-HF, LV-LF, HV-LF; p < 0.001). For slipperiness no main effect of evaluation method was found (p = 0.39), but a main effect of product (p < 0.001) and an interaction between product and evaluation method were found (p < 0.001). This indicates that thickness was evaluated similarly during oral and haptic assessment, whereas oral and haptic slipperiness ratings were less congruent.
3.4. Correlations between creaminess, slipperiness and thickness
Pearson correlation coefficients between rank-rating scores of the different texture attributes were calculated. Creaminess was positively correlated with oral thickness (r = 0.64, p < 0.001) and haptic thickness (r = 0.49, p < 0.001). Moderate positive correlations were found between creaminess and oral slipperiness (r = 0.35, p < 0.001) and haptic slipperiness (r = 0.45, p < 0.001). Rank-rating scores obtained from oral assessment of thickness correlated positively with those of haptic assessment (r = 0.59, p < 0.001). Slightly weaker positive correlations were found between oral and haptic slipperiness (r = 0.44, p < 0.001).
4. Discussion
This study aimed (i) to assess contributions of viscosity and friction properties to creaminess, thickness and slipperiness perception of iced coffees; and (ii) to compare oral and haptic thickness and slipperiness perception of these beverages. Results from paired comparisons and rank-rating were in good agreement and show that high-viscosity iced coffee (HV-LF) was more intense in all attributes (creaminess, oral and haptic thickness, oral and haptic slipperiness) compared to low-viscosity iced coffees. When comparing the two low-viscosity products, low-friction iced coffee (LV-LF) was orally perceived as less creamy and less thick, and haptically more slippery than high-friction iced coffee (LV-HF). Creaminess was stronger correlated to thickness than to slipperiness.
4.1. Effect of viscosity on thickness, slipperiness and creaminess perception
Results from paired comparisons and rank-rating uniformly demonstrate that increasing the viscosity of iced coffees enhanced oral and haptic perception of thickness and slipperiness, and oral perception of creaminess. These results reflect those of other studies, that found large effects of viscosity on texture perception of o/w emulsions.4,18,26 On the other hand, viscosity may not be the most important driver of texture perception in semi-solid foods, such as sour cream,49 cream cheese50 and other dairy products.23
Participants were able to distinguish oral thickness of iced coffees with viscosities differing by a factor of 2.5. This was expected, as Camacho et al.51 reported a Just Noticeable Difference (JND) for oral thickness of 3.1 mPa s in thin liquids (η = 10 mPa s) and the viscosity difference in the current study was twice this reported JND value (Table 2). Moreover, participants were able to discriminate low- and high-viscosity iced coffees based on haptic thickness. This is in line with results from Zhong et al.,52 who reported that a 1.83 and 2.05 fold increase in viscosity could be detected by haptic assessment of thickened water and thickened milk, respectively.
Liquid foods are generally perceived as creamier when viscosity increases,4,18,23–27 which explains why high-viscosity iced coffee was perceived as creamier than the low-viscosity products. As slipperiness has been reported to be related to friction in guar gum solutions19 and particle dispersions,20 a positive effect of viscosity on slipperiness was not expected in this study. In line with our results, others have demonstrated that more viscous liquids (i.e. gum-thickened water,53 apple juice, orange juice and soymilk54) were perceived to be more slippery in mouth. An explanation may be provided by Kokini and colleagues,21,55 who postulated that slipperiness is induced by the total force applied on the tongue. According to their model, slipperiness is proportional to both frictional and viscous forces, which might provide an explanation for our observation that higher viscosity resulted in increased oral and haptic slipperiness. This rationale is supported by the fact that more viscous fluids display reduced friction properties,56,57 which are in turn related to slipperiness perception.19,20,31
4.2. Effect of friction properties on thickness, slipperiness and creaminess perception
Results from paired comparisons and rank-rating of low-viscosity iced coffees show that reduced friction by addition of PEG (LV-LF vs. LV-HF) leads to decreased oral thickness and creaminess, but increased haptic slipperiness. Nevertheless, sensory differences due to addition of PEG were generally smaller than those elicited by increasing the viscosity of iced coffee. The fact that sensory differences between low-viscosity products were relatively small suggests that the difference in friction properties only led to subtle effects in texture perception. It should be noted that the range of friction properties covered in this study (maximum difference in friction coefficients between iced coffees was a factor 1.3) was smaller than that of viscosity (maximum difference in viscosity between iced coffees was a factor 2.5), which can partially explain the limited effect of friction properties on sensory characteristics of the iced coffees (see section 4.5 Limitations).
Reduction of friction by addition of maltodextrin resulted in increased perceived oral and haptic thickness, whereas addition of PEG did not affect perceived haptic thickness of low-viscosity iced coffees (Fig. 3 and 4). This was in line with our expectations, as thickness perception is associated with viscosity4,16–18 and the two low-viscosity iced coffees were iso-viscous. In contrast, perceived oral thickness decreased when friction was reduced by adding PEG. In a study on vanilla custard desserts,31 no correlation was found between friction properties and perceived thickness evaluated by a trained panel. A dumping effect may have occurred as participants in the current study were instructed to focus on one attribute, while other attributes were present that distinguished the products.
As expected, low-friction iced coffees were haptically perceived as more slippery than high-friction iced coffee. Reduction of friction by addition of maltodextrin also enhanced oral slipperiness of the high-viscosity product, whereas addition of PEG did not affect oral slipperiness of low-viscosity iced coffee. As negative relationships between friction and oral slipperiness were previously established in guar gum solutions,19 particle dispersions20 and vanilla desserts,31 a similar effect was expected in this study. The fact that participants only perceived a difference in slipperiness during haptic evaluation of low-viscosity products, suggests a possible effect of the oral environment on slipperiness perception of iced coffees. Iced coffees are warmed by the oral surfaces and diluted by saliva, which may consequently affect oral texture perception of the beverages. Saliva affects lubrication58 and the presence of saliva in the oral environment might therefore affect texture perception of the iced coffees.59 Moreover, food-saliva interactions might have occurred that affect texture perception.60–62 These factors may clarify why differences in friction properties between the three iced coffees become smaller upon addition of saliva (Fig. 2b), as has previously been reported by Joyner et al.63 as well. It should be noted that the iced coffees were mixed with saliva at a 1
:
1 ratio, i.e. the products were diluted, which may partially explain the smaller differences in friction properties upon addition of saliva. A mixing ratio of 1
:
1 was chosen to test whether saliva had any effect on friction properties of iced coffees. This mixing ratio overestimates the amount of saliva mixed with iced coffee, since under realistic drinking conditions the amount of saliva that mixes with iced coffee during oral processing is likely to be considerably smaller due to the short residence time of iced coffee in the oral cavity.
Reduced friction was hypothesised to enhance creaminess of iced coffees, as this has been described in several reviews.12,28,29 While this was observed upon addition of maltodextrin in high-viscosity iced coffee, this was not the case when PEG was added to low-viscosity iced coffee. When comparing the low-viscosity products, low-friction iced coffee was perceived as less creamy than high-friction iced coffee (Fig. 3 and 4). In a comparable study on milks, no effect on creaminess was observed by a trained panel after reduction of friction by addition of inulin.8 It is known that perceived thickness is important for creaminess of foods.22,23 The fact that participants perceived low-viscosity iced coffee with low friction as less thick than the high-friction product (Fig. 3 and 4) might therefore have caused a reduction in perceived creaminess.
4.3. Comparison of effect of viscosity and friction properties on creaminess perception
Identifying the roles of viscosity and friction properties could provide fundamental answers to understanding texture perception, and more specifically creaminess, of iced coffees. It was hypothesised that higher viscosity and lower friction would enhance creaminess of iced coffees.21,22 Results from paired comparisons and rank-rating confirm that higher viscosity resulted in enhanced creaminess perception. Reduction of friction by addition of PEG, on the other hand, did not have the expected positive impact on creaminess. This finding is contrary to those from previous studies on milk,30 custard desserts,31 yoghurts32 and cream cheese50 in which perception of creaminess was associated with lower friction coefficients. The reduction in friction properties achieved in this study was not necessarily sufficient to increase creaminess of iced coffees, whereas simultaneously increasing viscosity and decreasing friction enhanced creaminess.
Since viscosity is related to thickness perception4,16–18 and friction properties are related to slipperiness perception,19,20 it was hypothesised that perceived thickness and slipperiness are related to creaminess perception.1 In the current study, correlation coefficients between creaminess and oral thickness scores were higher (r = 0.64) compared to creaminess and oral slipperiness scores (r = 0.35). This finding is in agreement with results from others who suggested that viscosity was the major contributor to creaminess in o/w emulsions4,18 and acidified milk drinks,27 and that adjustment of friction properties did not have a large effect on creaminess of milks.8
Our results can be compared with early work of Kokini and colleagues,55 who modelled creaminess perception in liquid foods. They determined that the creaminess of liquid foods could be predicted by perceived thickness, smoothness and slipperiness, using the following formula (R2 = 0.88):
In line with our findings, the authors concluded that thickness contributes more to creaminess perception than slipperiness. Nevertheless, they also established a large contribution of perceived smoothness to the prediction of perceived creaminess. Kokini and colleagues postulated that smoothness is related to friction forces on the tongue. Our results suggest that small differences in friction properties are not sufficient to modify creaminess of iced coffees. Friction properties only become dominant during later stages of oral processing,14,15 which may explain why a smaller contribution of friction properties to creaminess was found in the current study. Viscosity might be more important for creaminess perception of iced coffees, as oral texture perception is initially governed by viscous properties of foods. During this early stage of oral processing, viscosity may ensure that the iced coffee is retained in the mouth long enough for creaminess to be perceived.24 However, the oral processing time of iced coffee is relatively short and might therefore be too short for friction properties to be perceived.
4.4. Comparison of oral and haptic assessment of texture attributes
The second aim of the study was to compare oral evaluations of thickness and slipperiness with haptic evaluations. Although iced coffee is usually not touched by the hands during consumption, we included haptic evaluation to obtain a complete image on how texture perception might be influenced by evaluation methods. Participants were able to orally and haptically discriminate low viscous iced coffees with viscosities differing by a factor of 2.5 based on both thickness and slipperiness. They were moreover able to discriminate haptic slipperiness of low viscous iced coffees with friction coefficients differing by 25%, whereas these iced coffees could not be discriminated based on oral slipperiness. Despite the fact that mechanoreceptors on the tongue have been reported to be more sensitive to force than those in the finger tips,40,45 we found comparable results for different evaluation methods of thickness (i.e. oral vs. haptic). In contrast, others found that liquids were perceived as more viscous when evaluated orally compared to non-oral methods38 or that oral viscosity discrimination was slightly better than haptic discrimination of syrup solutions.64 While in the current study rank-rating scores for oral thickness were congruent with those of haptic thickness, an interaction effect between evaluation method and product was found for slipperiness scores. Accordingly, the correlation between oral and haptic rank-rating scores for thickness (r = 0.59) is higher than the correlation between oral and haptic slipperiness scores (r = 0.44). All in all, our results imply that oral and haptic thickness are evaluated similarly, whereas haptic slipperiness is assessed differently from oral slipperiness.
4.5. Limitations
This study aimed to assess the individual contributions of viscosity and friction properties to texture perception of iced coffees. The effect of viscosity was assessed by comparing iced coffees with different viscosities, but similar friction properties. However, as viscosity and friction are physically related concepts, preparing liquids that vary in viscosity but not in friction poses a challenge. In practice it was difficult to obtain similar friction coefficients over the entire sliding speed range, as low friction was obtained in two ways: (a) by adding PEG to retain low viscosity; and (b) by adding maltodextrin, hence this decrease in friction was accompanied by an increase in viscosity. As friction in the mixed regime depends on surface properties as well as bulk viscosity of the liquid,15,56,57 it was not possible to formulate a low-viscosity product with similar friction properties in the mixed regime as the high-viscosity iced coffee. Low-viscosity iced coffee (LV-LF) had reduced friction coefficients in the boundary regime (<1 mm s−1), whereas high-viscosity iced coffee (HV-LF) exhibited reduced friction coefficients in the mixed regime (10–1000 mm s−1). The fact that viscosity affects friction also explains why friction coefficients of the two low-viscosity products are comparable in the mixed regime, as viscosity is an important determinant for friction in this regime. Differences between the products regarding friction therefore depend on the sliding speed that is discussed, which makes it more difficult to compare the low-friction iced coffees.
Our results suggest that viscosity might contribute more to creaminess of iced coffees than friction properties. Although this is in accordance with previous findings on o/w emulsions,4,18 milks8 and acidified milk drinks,27 the difference in friction properties between high- and low-friction iced coffees in this study might have been too small to be reflected in perception of texture attributes. Viscosity of the iced coffees was varied by a factor of 2.5, whereas friction was varied by a factor of maximum 1.3 due to addition of PEG. If we assume a linear or semi-log relationship between the physical-chemical food properties and perceived creaminess, it is reasonable that a larger contribution of viscosity is found, as viscosity of the iced coffees was varied to a higher degree. Larger effects on perceived creaminess and slipperiness might arise when friction properties are modified to a larger degree. PEG with higher Mw is capable of reducing friction properties to a larger extent without considerably affecting viscosity. However, due to legal restrictions regarding the use of high Mw PEG in foods,65,66 such studies would be limited to haptic evaluations only.
Care should be taken when comparing results from the current study to other studies linking tribological properties to sensory attributes. The comprehensive review by Sarkar & Krop67 highlights that such tribology-sensory relationships are only valid for the specific food and experimental set-up used, as friction properties depend on the interplay of the food with the surfaces of the tribo-pair. Due to this and the fact that only iced coffees were used, results from the current study cannot be generalised to liquid foods. Further research using a standardised tribological set-up and methodology is needed to establish clear relationships between friction and perception of specific texture attributes.
Due to the addition of maltodextrin or PEG to the iced coffees, the final fat content of the three products was not identical (0.63–0.84% (w/v)). Chojnicka-Paszun et al.30 found that friction properties and perceived creaminess of milks are independent of fat content at fat contents below 1%. Furthermore, Akhtar et al.18 showed that fat content had no effect on perception of thickness and creaminess of low-viscosity emulsions (8 mPa s). Therefore, we argue that the small difference in fat content did not considerably influence the friction properties or perceived creaminess of the iced coffees.
As only small differences between the iced coffees were expected, a 2-AFC test was performed. Such discrimination tests do not require trained panellists and are generally better at detecting small differences between products compared to intensity ratings.68 However, as we were also interested in the magnitude of the differences between the products, the discrimination test was followed by a rank-rating procedure. The fact that results from both sensory methods led to the same conclusions indicates that participants were consistent in evaluating the iced coffees. While dumping effects may be eliminated by using a trained panel, results from an untrained panel are more representative of perception by consumers.
5. Conclusions
To the best of our knowledge, this is the first study that aimed to assess the relative contributions of viscosity and friction properties to texture perception by varying viscosity of iced coffees with minimal changes in friction properties and vice versa. This study has identified that increasing the viscosity enhances creaminess, thickness and slipperiness of iced coffees. On the other hand, reduced friction in the boundary regime resulted in lower oral thickness and creaminess scores, but increased perception of haptic slipperiness of iced coffees. Creaminess was stronger correlated to thickness than to slipperiness. The oral processing time of iced coffees was possibly too short for textural differences related to friction properties to be perceived, as texture perception is initially governed by viscous properties of food, thereby limiting the time for friction properties to be perceived. Therefore, in development of low-fat milk-based beverages with creamy texture, product developers might want to focus more on viscosity than friction properties. Future research should focus on developing a range of foods (i.e. liquid to semi-solid) that differ considerably in friction properties without strongly affecting viscosity to elucidate the individual contributions of friction and viscosity to creaminess perception in foods.
Conflicts of interest
There are no conflicts to declare.
Acknowledgements
This research was performed within the framework of the “Molecular aspects of biopolymers defining food texture perception and oral digestion” project funded by NWO (The Netherlands Organization for Scientific Research), grant number 731.017.201, Unilever and Anton Paar. The project partners (University of Amsterdam, Unilever, Anton Paar) have contributed to the project through regular discussion.
References
- N. J. Richardson-Harman, R. Stevens, S. Walker, J. Gamble, M. Miller, M. Wong and A. McPherson, Mapping consumer perceptions of creaminess and liking for liquid dairy products, Food Qual. Prefer., 2000, 11, 239–246 CrossRef.
- G. Ares, A. Giménez, C. Barreiro and A. Gámbaro, Use of an open-ended question to identify drivers of liking of milk desserts. Comparison with preference mapping techniques, Food Qual. Prefer., 2010, 21, 286–294 CrossRef.
- D. Kilcast and S. Clegg, Sensory perception of creaminess and its relationship with food structure, Food Qual. Prefer., 2002, 13, 609–623 CrossRef.
- M. Akhtar, B. S. Murray and E. Dickinson, Perception of creaminess of model oil-in-water dairy emulsions: Influence of the shear-thinning nature of a viscosity-controlling hydrocolloid, Food Hydrocolloids, 2006, 20, 839–847 CrossRef CAS.
- G. Sala, F. van de Velde, M. A. Cohen Stuart and G. A. van Aken, Oil droplet release from emulsion-filled gels in relation to sensory perception, Food Hydrocolloids, 2007, 21, 977–985 CrossRef CAS.
- Y. Chen, Y. She, R. Zhang, J. Wang, X. Zhang and X. Gou, Use of starch-based fat replacers in foods as a strategy to reduce dietary intake of fat and risk of metabolic diseases, Food Sci. Nutr., 2020, 8, 16–22 CrossRef CAS PubMed.
- D. Guggisberg, J. Cuthbert-Steven, P. Piccinali, U. Bütikofer and P. Eberhard, Rheological, microstructural and sensory characterization of low-fat and whole milk set yoghurt as influenced by inulin addition, Int. Dairy J., 2009, 19, 107–115 CrossRef CAS.
- D. Meyer, J. Vermulst, R. H. Tromp and E. H. A. De Hoog, The effect of inulin on tribology and sensory profiles of skimmed milk, J. Texture Stud., 2011, 42, 387–393 CrossRef.
- T. A. Rovers, G. Sala, E. van der Linden and M. B. Meinders, Potential of microbubbles as fat replacer: Effect on rheological, tribological and sensorial properties of model food systems, J. Texture Stud., 2016, 47, 220–230 CrossRef.
- K. Liu, Y. Tian, M. Stieger, E. van der Linden and F. van de Velde, Evidence for ball-bearing mechanism of microparticulated whey protein as fat replacer in liquid and semi-solid multi-component model foods, Food Hydrocolloids, 2016, 52, 403–414 CrossRef CAS.
- K. Liu, M. Stieger, E. van der Linden and F. van de Velde, Effect of microparticulated whey protein on sensory properties of liquid and semi-solid model foods, Food Hydrocolloids, 2016, 60, 186–198 CrossRef CAS.
- R. Upadhyay, T. Aktar and J. Chen, Perception of creaminess in foods, J. Texture Stud., 2020, 51(3), 375–388 CrossRef PubMed.
- R. A. de Wijk, L. Engelen and J. F. Prinz, The role of intra-oral manipulation in the perception of sensory attributes, Appetite, 2003, 40, 1–7 CrossRef PubMed.
- J. Chen and J. R. Stokes, Rheology and tribology: Two distinctive regimes of food texture sensation, Trends Food Sci. Technol., 2012, 25, 4–12 CrossRef CAS.
- J. R. Stokes, M. W. Boehm and S. K. Baier, Oral processing, texture and mouthfeel: From rheology to tribology and beyond, Curr. Opin. Colloid Interface Sci., 2013, 18, 349–359 CrossRef CAS.
- A. N. Cutler, E. R. Morris and L. J. Taylor, Oral perception of viscosity in fluid foods and model systems, J. Texture Stud., 1983, 14, 377–395 CrossRef.
- R. K. Richardson, E. R. Morris, S. B. Ross-Murphy, L. J. Taylor and I. C. M. Dea, Characterization of the perceived texture of thickened systems by dynamic viscosity measurements, Food Hydrocolloids, 1989, 3, 175–191 CrossRef.
- M. Akhtar, J. Stenzel, B. S. Murray and E. Dickinson, Factors affecting the perception of creaminess of oil-in-water emulsions, Food Hydrocolloids, 2005, 19, 521–526 CrossRef CAS.
- M. E. Malone, I. A. M. Appelqvist and I. T. Norton, Oral behaviour of food hydrocolloids and emulsions. Part 1. Lubrication and deposition considerations, Food Hydrocolloids, 2003, 17, 763–773 CrossRef CAS.
- A. Chojnicka-Paszun, S. Doussinault and H. H. J. de Jongh, Sensorial analysis of polysaccharide–gelled protein particle dispersions in relation to lubrication and viscosity properties, Food Res. Int., 2014, 56, 199–210 CrossRef CAS.
- J. L. Kokini, The physical basis of liquid food texture and texture-taste interactions, J. Food Eng., 1987, 6, 51–81 CrossRef.
- J. L. Kokini and E. L. Cussler, Predicting the texture of liquid and melting semi-solid foods, J. Food Sci., 1983, 48, 1221–1225 CrossRef.
- M. B. Frøst and T. Janhøj, Understanding creaminess, Int. Dairy J., 2007, 17, 1298–1311 CrossRef.
- F. W. Wood, An approach to understanding creaminess, Starch/Staerke, 1974, 26, 127–130 CrossRef CAS.
- N. Daget and M. Joerg, Creamy perception II: In model soups, J. Texture Stud., 1991, 22, 169–189 CrossRef.
- G. A. van Aken, M. H. Vingerhoeds and R. A. de Wijk, Textural perception of liquid emulsions: Role of oil content, oil viscosity and emulsion viscosity, Food Hydrocolloids, 2011, 25, 789–796 CrossRef CAS.
- T. Janhøj, M. B. Frøst and R. Ipsen, Sensory and rheological characterization of acidified milk drinks, Food Hydrocolloids, 2008, 22, 798–806 CrossRef.
- E. Dickinson, On the road to understanding and control of creaminess perception in food colloids, Food Hydrocolloids, 2018, 77, 372–385 CrossRef CAS.
- R. A. de Wijk, M. E. J. Terpstra, A. M. Janssen and J. F. Prinz, Perceived creaminess of semi-solid foods, Trends Food Sci. Technol., 2006, 17, 412–422 CrossRef CAS.
- A. Chojnicka-Paszun, H. H. J. de Jongh and C. G. de Kruif, Sensory perception and lubrication properties of milk: Influence of fat content, Int. Dairy J., 2012, 26, 15–22 CrossRef.
- R. A. de Wijk and J. F. Prinz, The role of friction in perceived oral texture, Food Qual. Prefer., 2005, 16, 121–129 CrossRef.
- A. Sonne, M. Busch-Stockfisch, J. Weiss and J. Hinrichs, Improved mapping of in-mouth creaminess of semi-solid dairy products by combining rheology, particle size, and tribology data, LWT – Food Sci. Technol., 2014, 59, 342–347 CrossRef CAS.
- R. Upadhyay and J. Chen, Smoothness as a tactile percept: Correlating ‘oral’ tribology with sensory measurements, Food Hydrocolloids, 2019, 87, 38–47 CrossRef CAS.
- L. Laguna, G. Farrell, M. Bryant, A. Morina and A. Sarkar, Relating rheology and tribology of commercial dairy colloids to sensory perception, Food Funct., 2017, 8, 563–573 RSC.
- F. Shama, C. Parkinson and P. Sherman, Identification of stimuli controlling the sensory evaluation of viscosity I. Non-oral methods, J. Texture Stud., 1973, 4, 102–110 CrossRef.
- F. Shama and P. Sherman, Identification of stimuli controlling the sensory evaluation of viscosity II. Oral methods, J. Texture Stud., 1973, 4, 111–118 CrossRef.
- W. M. Bergmann Tiest, A. C. Vrijling and A. M. Kappers, Haptic discrimination and matching of viscosity, IEEE Trans. Haptics, 2012, 6, 24–34 Search PubMed.
- C. Christensen and L. Casper, Oral and nonoral perception of solution viscosity, J. Food Sci., 1987, 52, 445–447 CrossRef.
-
L. Engelen and R. A. de Wijk, in Food Oral Processing: Fundamentals of Eating and Sensory Perception, Wiley-Blackwell, 2012, ch. 8, pp. 157–176, DOI:10.1002/9781444360943.ch8.
- M. Trulsson and G. K. Essick, Low-threshold mechanoreceptive afferents in the human lingual nerve, J. Neurophysiol., 1997, 77, 737–748 CrossRef CAS PubMed.
- R. S. Johansson and A. Vallbo, Tactile sensibility in the human hand: relative and absolute densities of four types of mechanoreceptive units in glabrous skin, J. Physiol., 1979, 286, 283–300 CrossRef CAS PubMed.
- B. B. Edin and J. H. Abbs, Finger movement responses of cutaneous mechanoreceptors in the dorsal skin of the human hand, J. Neurophysiol., 1991, 65, 657–670 CrossRef CAS PubMed.
- R. S. Johansson, M. Trulsson, K. Olsson and K.-G. Westberg, Mechanoreceptor activity from the human face and oral mucosa, Exp. Brain Res., 1988, 72, 204–208 CrossRef CAS PubMed.
- M. Nordin and K. E. Hagbarth, Mechanoreceptive units in the human infra-orbital nerve, Acta Physiol. Scand., 1989, 135, 149–161 CrossRef CAS PubMed.
- E. M. Rath and G. K. Essick, Perioral somesthetic sensibility: do the skin of the lower face and the midface exhibit comparable sensitivity?, J. Oral Maxillofac. Surg., 1990, 48, 1181–1190 CrossRef CAS PubMed.
-
P. L. Matlock, W. L. Brown and N. A. Clinton, in Synthetic lubricants and high-performance functional fluids, ed. L. R. Rudnick and R. L. Shubkin, Marcel Dekker, New York, 2nd edn, 1999, ch. 6., polyalkylene glycols, 159–194 Search PubMed.
- E. Silletti, M. H. Vingerhoeds, W. Norde and G. A. Van Aken, The role of electrostatics in saliva-induced emulsion flocculation, Food Hydrocolloids, 2007, 21, 596–606 CrossRef CAS.
- H. Turner and D. Firth, Bradley-Terry models in R: the BradleyTerry2 package, J. Stat. Softw., 2012, 48, 1–21 Search PubMed.
- S. M. Jervis, P. Gerard, S. Drake, K. Lopetcharat and M. A. Drake, The perception of creaminess in sour cream, J. Sens. Stud., 2014, 29, 248–257 CrossRef.
- T. Janhøj, M. B. Frøst, J. Prinz and R. Ipsen, Sensory and instrumental characterization of low-fat and non-fat cream cheese, Int. J. Food Prop., 2009, 12, 211–227 CrossRef.
- S. Camacho, M. Dop, C. Graaf and M. Stieger, Just noticeable differences and Weber fraction of oral thickness perception of model beverages, J. Food Sci., 2015, 80, 1583–1588 CrossRef PubMed.
- L. Zhong, E. K. Hadde, Z. Zhou, Y. Xia and J. Chen, Sensory discrimination of the viscosity of thickened liquids for dysphagia management, J. Sens. Stud., 2018, 33, e12464 CrossRef.
- J. J.-X. Ong, C. M. Steele and L. M. Duizer, Challenges to assumptions regarding oral shear rate during oral processing and swallowing based on sensory testing with thickened liquids, Food Hydrocolloids, 2018, 84, 173–180 CrossRef CAS PubMed.
- H. Kim, H. I. Hwang, K. W. Song and J. Lee, Sensory and rheological characteristics of thickened liquids differing concentrations of a xanthan gum-based thickener, J. Texture Stud., 2017, 48, 571–585 CrossRef PubMed.
- J. L. Kokini, J. B. Kadane and E. L. Cussler, Liquid texture perceived in the mouth, J. Texture Stud., 1977, 8, 195–218 CrossRef.
- J. de Vicente, J. R. Stokes and H. A. Spikes, Lubrication properties of non-adsorbing polymer solutions in soft elastohydrodynamic (EHD) contacts, Tribol. Int., 2005, 38, 515–526 CrossRef CAS.
- J. de Vicente, J. R. Stokes and H. A. Spikes, Soft lubrication of model hydrocolloids, Food Hydrocolloids, 2006, 20, 483–491 CrossRef CAS.
- L. A. Tabak, In defense of the oral cavity: structure, biosynthesis, and function of salivary mucins, Annu. Rev. Physiol., 1995, 57, 547–564 CrossRef CAS PubMed.
- L. Engelen, R. A. de Wijk, J. F. Prinz, A. M. Janssen, A. van der Bilt, H. Weenen and F. Bosman, A comparison of the effects of added saliva, α-amylase and water on texture perception in semisolids, Physiol. Behav., 2003, 78, 805–811 CrossRef CAS PubMed.
- N. Selway and J. R. Stokes, Insights into the dynamics of oral lubrication and mouthfeel using soft tribology: Differentiating semi-fluid foods with similar rheology, Food Res. Int., 2013, 54, 423–431 CrossRef.
- D. M. Dresselhuis, E. H. A. de Hoog, M. A. Cohen Stuart, M. H. Vingerhoeds and G. A. van Aken, The occurrence of in-mouth coalescence of emulsion droplets in relation to perception of fat, Food Hydrocolloids, 2008, 22, 1170–1183 CrossRef CAS.
- D. Rossetti, G. Yakubov, J. Stokes, A.-M. Williamson and G. Fuller, Interaction of human whole saliva and astringent dietary compounds investigated by interfacial shear rheology, Food Hydrocolloids, 2008, 22, 1068–1078 CrossRef CAS.
- H. S. Joyner, C. W. Pernell and C. R. Daubert, Impact of formulation and saliva on acid milk gel friction behavior, J. Food Sci., 2014, 79, E867–E880 CrossRef CAS PubMed.
- T. Aktar, J. Chen, R. Ettelaie and M. Holmes, Evaluation of the sensory correlation between touch sensitivity and the capacity to discriminate viscosity, J. Sens. Stud., 2015, 30, 98–107 CrossRef.
-
Joint FAO/WHO Expert Committee on Food Additives (JECFA)/Food and Agriculture Organization of the United Nations (FAO), Evaluation of Certain Food Additives, Twenty-third Report of the Joint FAO/WHO Expert Committee on Food Additives, World Health Organization, Geneva, 1980, 17–18 Search PubMed.
- Code of Federal Regulations, Journal, 1984, §172.820.
- A. Sarkar and E. M. Krop, Marrying oral tribology to sensory perception: a systematic review, Curr. Opin. Food Sci., 2019, 27, 64–73 CrossRef PubMed.
-
H. T. Lawless and H. Heymann, Sensory Evaluation of Food: Principles and Practices, Springer Science & Business Media, 2010 Search PubMed.
|
This journal is © The Royal Society of Chemistry 2020 |
Click here to see how this site uses Cookies. View our privacy policy here.