DOI:
10.1039/D0MA00403K
(Paper)
Mater. Adv., 2020,
1, 2339-2345
ZnO nanostructures: a heterogeneous catalyst for the synthesis of benzoxanthene and pyranopyrazole scaffolds via a multi-component reaction strategy†
Received
10th June 2020
, Accepted 21st August 2020
First published on 21st August 2020
Abstract
A hydrothermal technique is employed for the preparation of nanostructured ZnO. The reaction was carried out at 180 °C for 2 h in the presence of ethylenediamine (EDA) as a capping agent and was characterized by X-ray diffraction (XRD), scanning electron microscopy (FESEM), and transmission electron microscopy (TEM). XRD indicates the formation of highly crystalline ZnO having a wurtzite structure. FESEM analysis validates the formation of a submicron size spherical shaped marigold flower like morphology. The nanostructured ZnO was screened for its catalytic activity as a heterogeneous catalyst for the multicomponent synthesis of benzoxanthenes and pyranopyrazole derivatives in good to excellent yield. The major advantages associated with this methodology include ease of separation and reusability of the catalyst, easy work-up and short reaction time.
Introduction
Multi-component reactions (MCRs) play an important role in total synthesis and combinatorial chemistry because of their ability to synthesize heterocyclic scaffolds with numerous degrees of structural diversity. Such reactions have received considerable attention because of their simplicity and economical aspects that allow chemists to explore the synthesis of structurally diverse organic compounds.1 The nanostructured metal oxides such as ZnO, CuO, MgO, TiO2 Fe2O3etc. have attracted much attention due to their unique properties which offer greater surface-to-volume ratio and higher catalytic activity.2
Synthesis of heterocyclic compounds has received substantial attention due to their wide range of pharmacological and biological applications. The benzoxanthenes are a very important class of biologically active heterocyclic compounds in medicinal fields. They exhibit mainly anti-inflammatory, antiviral and antibacterial activities.3–5 They can also be used as dyes,6 pH-sensitive fluorescent materials for the visualization of bimolecular assemblies7 and laser technologies.8 Numerous methods have been reported for the synthesis of benzoxanthene derivatives, which include trontiumtriflate,9 silicasulphuricacid,10 P2O5 and indium(III) chloride,11,12 methane sulfonic acid,13 NaHSO4–SiO2,14p-dodecylbenzenesulfonic acid (DBSA),15,16 tetrabutylammoniumhydrogensulphate (TBAHS).17 imidazolium based ionic liquid [bmim]PF6,18 and trimethylsilyl chloride (TMSCL).19 Pyranopyrazole derivatives are a significant class of biologically active heterocycles. They exhibit various biological activities such as anticancer, antimicrobial, analgesic, anti-inflammatory, mollucicidal and fungicidal activities. These derivatives are also used as cosmetics and pigments.20–24 Various methods have been reported for the synthesis of these derivatives such as Prolinetriflate,25 PTSA in ionic liquid,26 magnetic nano particle supported dual ionic liquid 3-sulfobutyl-1-(3-propyltriethoxysilane)imidazolium hydrogen sulfate/SiO2–Fe3O4.27 Nanocrystalline ZnO,28 and basic ionic liquids.29 Although the traditional synthetic methodologies offer efficiency, most of them suffer from certain limitations such as low yields of the products, use of harsh reaction conditions, toxic solvents and catalysts compared to nanostructured materials. The semiconducting material ZnO in its nanostructured form has been extensively used in a wide range of technological applications such as electrical engineering, photodegradation of microorganisms, catalysis, and optical and optoelectronic devices.30–36 In the present work nanostructured ZnO is utilized as a heterogeneous catalyst in multi-component organic transformations and can be used for the formation of binary or ternary composite nanomaterials for a variety of applications.37,38 In this regard, we have developed a simple, efficient, non-toxic heterogeneous catalytic system for the multi-component synthesis of heterocyclic scaffolds like benzoxanthene and pyranopyrazole derivatives via one pot four component reactions.
Experimental
Materials and reagents
For the synthesis of ZnO nanostructures, zinc nitrate (Zn(NO3)2·6H2O), sodium hydroxide (NaOH), and ethylenediamine (EDA) of analytical grade were used as received. All other chemicals, reagents and solvents were of analytical grade and used as received without further purification.
Synthesis of ZnO nanostructures
For the synthesis of ZnO nanostructures, 10 mmol of zinc nitrate (Zn(NO3)2·6H2O) and 20 mmol of NaOH were dissolved separately in 15 mL of distilled water respectively. The NaOH solution was dropwise added to zinc nitrate solution with constant stirring. To this mixture separately prepared EDA solution (0.5 mL in 20 mL distilled water) was added dropwise and the stirring was continued for 15 min. The whole mixture was then transferred to a stainless steel autoclave containing a 100 mL Teflon container. The autoclave was sealed properly and heated at 180 °C for 2 h in an electric oven. After completion of the reaction the autoclave was naturally cooled down to room temperature. The obtained reaction mass was washed with deionised water in order remove the excess NaOH and unreacted precursor. Then a final wash was given with ethanol. The sample was dried at 80 °C in a vacuum oven for 1 h, and the dried sample was ground in a mortar and pestle and used as such for further analysis and to evaluate its catalytic activity study.
Characterization
The prepared ZnO nanostructures were characterized with different spectroscopic and microscopic techniques. The phases were identified using a powder XRD technique (Bruker AXS model D-8, 10 to 70° range, scan rate = 10° min−1) equipped with a monochromator and Ni-filtered Cu Kα (1.5406 Å) radiation. The as synthesised ZnO nanostructures were studied morphologically using FESEM (HITACHI S-4800). Microstructure analyses were carried out using a field emission transmission electron micrograph (FETEM) performed using a JEOL JEM-2200 FS microscope operating at 200 kV. 1HNMR spectra of organic compounds were recorded on a Varian 400 MHz NMR spectrophotometer with CDCl3 or DMSO-d6 as a solvent. Coupling constants (J) are given in Hertz. Melting points were determined on a Buchi M-560. Mass spectral data were obtained from LC-MS (Shimadzu 2010). The reaction was monitored by thin layer chromatography (TLC) on Merck's silica gel plates (60 F254). Photoluminescence spectra (PL) were recorded by the photoluminescence spectrometer ShimadzuRF-5301.
Synthesis of benzoxanthene
In a 25 mL round bottom flask a stoichiometric mixture of β-naphthol (1.0 mmol), aromatic aldehydes (1.0 mmol) and 1,3 dicarbonyl compound (dimedone) (1.0 mmol) were mixed together with a catalytic amount (5 mol%) of ZnO in the presence of ethanol
:
water (5 mL) and refluxed for 1–2 h.
The progress of the reaction was monitored by TLC (30% hexane/ethyl acetate). After completion of the reaction, the catalyst was filtered and the resulting product was extracted with ethyl acetate, dried over anhydrous sodium sulfate and the solvent was evaporated under reduced pressure. The crude product was purified by silica column chromatography using hexane/ethyl acetate (90
:
10 v/v) as an eluent. Proton chemical shifts (δ) are relative to TMS (δ = 0) as an internal standard and expressed in ppm. Coupling constants (J) are given in Hertz. The characterization data, physical constants and NMR of these compounds were found to be identical with those reported in the literature (Table S1, ESI†).
Synthesis of pyranopyrazole
In a 25 mL round bottom flask, ethyl acetoacetate (1.0 mmol), hydrazine hydrate (1.0 mmol), aldehyde (1 mmol), and malononitrile (1 mmol) were mixed together and a catalytic amount (5 mol%) of ZnO was added in the presence of aq. ethanol (5 mL). The reaction was stirred at room temperature for 15–30 min,
The progress of the reaction was monitored by TLC (30% hexane/ethyl acetate). After completion of the reaction, the catalyst was filtered and the resulting product was extracted with ethyl acetate, dried over anhydrous sodium sulfate and the solvent was evaporated under reduced pressure. The crude product was purified by silica column chromatography using hexane/ethyl acetate (Table S2, ESI†).
Results and discussion
In order to investigate the crystalline phases, the synthesized ZnO was analysed with the XRD technique and the XRD pattern is depicted in Fig. 1. The intense XRD peaks confirm the formation of highly crystalline ZnO. The observed XRD peaks at 2θ = 31.7, 34.4, 36.2, 47.6, 56.7, and 62.8° are attributed to (hkl) planes (100), (002), (101), (102), (110) and (103) respectively. The peak positions validate the formation of crystalline ZnO having a hexagonal wurtzite phase. XRD matches with the reported ZnO pattern (JCPDS No. 36-1451) indicating high phase purity and crystallinity. The average crystallite size calculated using Scherer's equation is found to be 30 nm. The observed lattice constants of the prepared ZnO nanostructure having hexagonal wurtzite phase are a = b = 3.24 Å and c = 5.20 Å. After confirming the crystalline ZnO, further morphological study of a ZnO nanostructure by FESEM and TEM analysis was performed.
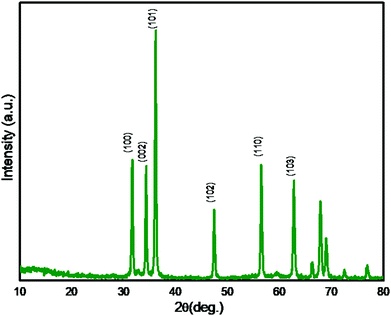 |
| Fig. 1 XRD pattern of ZnO nanostructures. | |
FESEM analysis
The morphological study of the prepared ZnO nanostructures was carried out using the FESEM technique and the images are presented in Fig. 2. FESEM analysis indicates the formation of submicron sized spherical shape marigold flower like hierarchical morphology having a size in the range of 1 to 1.5 μm.
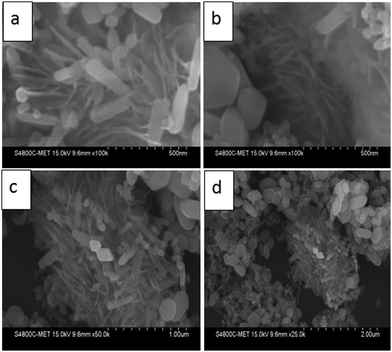 |
| Fig. 2 FESEM of ZnO nanostructures with different resolutions (reaction conditions 180 °C for 2 h). | |
This hierarchical ZnO flower is made up of small nanosized petals having very thin nanosheets of thickness 10–12 nm and along with the flower like morphology, hexagonal shaped plate and nanorods with 50 to 100 nm thickness were also observed. But these nanorods and plates are very few as compared to flowers. The use of EDA leads to the formation of a flower like morphology as it forms a complex with zinc ions (Zn–EDA) and restricts the existence of free zinc ions in solution resulting in controlled formation of zinc nuclei. At higher temperature during the reaction the controlled decomposition of this complex leads to the formation of particular shaped morphology; a detailed mechanism study is in progress.
TEM analysis
TEM micrographs of the as synthesized hierarchical ZnO nanostructures along with the selected area diffraction pattern (SAED) are shown in Fig. 3.
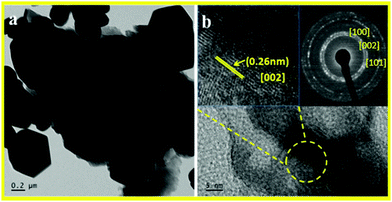 |
| Fig. 3 TEM micrographs of ZnO nanostructures and SAED image (inset of (b), 180 °C for 2 h). | |
TEM micrographs of ZnO nanostructures prepared at 180 °C for 2 h showed formation of hexagonal, spherical and petal like mixed phase morphology. (TEM image a and b). SAED shown in the inset of Fig. 3b indicates the formation of highly crystalline hexagonal wurtzite phase ZnO nanostructures. The high resolution TEM (HRTEM) image indicates an inter planar spacing of 0.262 nm corresponding to the [002] plane. From the TEM images it can be seen that the flowers are formed via self-assembling of very thin nanopetals which are created by self-alignment of tiny ZnO nanostructures. The concentric fused rings can be observed in the SAED pattern and match with the hexagonal phase of ZnO, thereby supporting XRD analysis (inset of Fig. 3b).
Growth mechanism for ZnO nanostructures
The growth mechanism for the formation of ZnO nanostructures is proposed and may follow the following pathways (Scheme 3a–d). During a hydrothermal reaction, the precursors slowly mixed and decomposed into tiny nuclei of ZnO (Scheme 3a and b). Primarily, tiny nuclei of ZnO are formed in the supersaturated solution and further growth of nanoparticles takes place with time (Scheme 3c). Under the optimized reaction conditions (180 °C/hydrothermal, 2 h & EDA), the ZnO nuclei were grown to form hierarchical (hexagonal, spherical and petal like mixed phase morphology) ZnO nanostructures.
The room temperature PL spectrum of the ZnO nanostructures was obtained with an excitation wavelength of 350 nm and is shown in Fig. S7 (ESI†). It shows two distinct peaks at 392 and 490 nm, the sharp and strong ultra violet emission peak at 392 nm corresponds to band edge emission and the broad emission peak centered at 490 nm is observed due to oxygen vacancies in the ZnO lattice.39
Synthesis of benzoxanthene and pyranopyrazole derivatives
We initially optimized the reaction conditions for the multicomponent reaction of β-naphthol, benzaldehyde and dimedone to afford the corresponding benzoxanthene derivative. The various reaction parameters comprising amount of catalyst, influence of solvent and temperature were evaluated for the reactions.
Screening of catalyst
To establish the general reaction conditions for the synthesis of benzoxanthenes, a model reaction of β-naphthol, p-nitrobenzaldehyde and dimedone was studied with and without ZnO nanostructured material as a catalyst and the results are summarized in Table 1. The reaction was sluggish and did not proceed in the absence of catalyst even after prolonged reaction time. The use of ZnO nanostructured material afforded good yield of the desired benzoxanthenes.
Table 1 Optimization of amount of catalyst
Entry |
ZnO (x mmol) |
Yielda (%) |
Isolated yield after chromatographic separation. Reaction conditions: aldehyde (1 mmol), 2-naphthol (1 mmol), 1, 3 dicarbonyl compound (1 mmol), catalyst (5 mol%) were stirred in 5 mL ethanol : water at reflux temperature for 1–2 h.
|
1 |
0.5 |
65 |
2 |
1.0 |
78 |
3 |
2.5 |
85 |
4 |
5.0 |
92 |
5 |
7.5 |
94 |
6 |
10.0 |
94 |
In order to find the appropriate concentration of ZnO for synthesis, the amount of catalyst varied from 0.5 mol% to 10 mol%, and the results are depicted in Table 1. With increase in catalyst amount the yield of the reaction increases from 65% to 92% respectively. With a higher catalyst amount (>5 mol%) the yield of the product was around 94% this indicates that there was not much increase in the yield. The increase in the catalyst amount did not show any significant improvement in the yield of the resultant product. Based on the above observations 5 mol% catalyst was used for the synthesis of benzoxanthene derivatives.
Screening of solvent
The effect of various solvents on the yield of benzoxanthene was also explored using 5 mol% ZnO nanostructured material and the results are summarized in Table 2.
Table 2 Effect of solvent
Entry |
Solvent |
Time |
Yielda (%) |
Isolated yield after chromatographic separation. Reaction conditions: aldehyde (1 mmol), 2-naphthol (1 mmol), 1,3 dicarbonyl compound (1 mmol), catalyst (5 mol%) were stirred in 5 mL solvent ethanol : water at reflux temperature.
|
1 |
H2O |
6 h |
>20 |
2 |
EtOH : H2O |
60 min |
92 |
3 |
MeOH |
90 min |
85 |
4 |
CH3CN |
60 min |
89 |
5 |
DCM |
75 min |
80 |
6 |
THF |
60 min |
82 |
7 |
CHCl3 |
60 min |
86 |
9 |
DMF |
90 min |
65 |
10 |
Toluene |
120 min |
55 |
When the reaction was carried out in water it afforded the desired product in lower yields (20%). After extended reaction time this may due to the inhomogeneity of the reactants. It was observed that the yield of benzoxanthenes in aqueous ethanol (50%) was almost more than 92% whereas, in methanol it was 85%. Other polar aprotic solvents (CH3CN, DCM, THF, CHCl3, DMF) afforded moderate yield (Table 2, entry 4 to 9), while a non-polar solvent (Table 2, entry 10) resulted in the desired product in lower yield, which required longer reaction time. Among the studied solvents aqueous ethanol (50%) gave an excellent yield of benzoxanthene within 1–2 h of reaction time. For further investigation we used 5 mol% of catalyst and aqueous ethanol (50%) as a solvent system.
Effect of temperature
The effect of temperature was studied by carrying out the control reaction at room temperature and under reflux temperature in the presence of nanostructured ZnO and aqueous ethanol as a solvent. The best result was obtained at reflux temperature (78–80 °C). At room temperature (25–27 °C) the reaction did not proceed even after prolonged reaction time.
To explore the further applicability of this reaction using the optimized reaction conditions, we extended the methodology for the synthesis of diverse derivatives of benzoxanthene using various aromatic aldehydes with either electron-releasing or electron-withdrawing substituents in the ortho, meta and para positions (Scheme 1), and the results are summarized in Table 3.
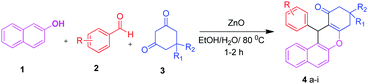 |
| Scheme 1 ZnO catalysed synthesis of benzoxanthene. | |
Table 3 Synthesis of benzoxanthenes
Reaction conditions: Isolated yield after chromatographic separation. Reaction conditions: aldehyde (1 mmol), 2-naphthol (1 mmol), 1,3 dicarbonyl compound (1 mmol), catalyst (5 mol%) were stirred in 5 mL ethanol–water at reflux temperature for 1–2 h. |
|
The benzaldehyde and halogenated benzaldehydes gave almost more than 87% yield (Table 3, entries 4a, 4b, 4e, 4i). 4-Nitrobenzaldehyde afforded 92% yield of the corresponding benzoxanthene in a shorter time (Table 3, entry 4c). It was observed that the reaction proceeds at a faster rate with all the aldehydes possessing electron-withdrawing groups on the aromatic ring than aldehydes possessing electron-donating substituents (Table 3, entries 4d, 4f, 4g, 4h & 4j) giving good to excellent yield of the corresponding products.
After effective application of ZnO nanostructures as a heterogeneous catalyst for the synthesis of benzoxanthene derivatives we further extended the methodology for multi-component synthesis of pyranopyrazole derivatives. (Scheme 2).
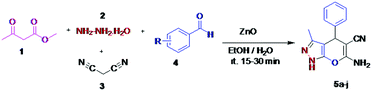 |
| Scheme 2 ZnO catalysed synthesis of pyranopyrazole. | |
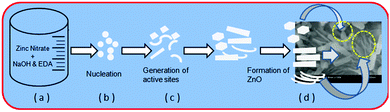 |
| Scheme 3 Plausible growth mechanism of ZnO nanostructures ((d) is the FESEM image of the ZnO nanostructures). | |
The multi-component reaction of aromatic aldehydes with ethylacetoacetate, hydrazine hydrate and malononitrile in the presence of ZnO nano structures as a heterogeneous catalyst is described under optimized reaction with respect to the amount of catalyst and solvent as mentioned in Scheme 1.
To optimize the reaction conditions, the model reaction was studied with 4-chlorobenzaldehyde, ethyl acetoacetate, malononitrile and hydrazine hydrate. Various solvents were studied for the optimization of the reaction conditions and the best results were obtained with ethanol–water at room temperature. A wide range of aromatic aldehydes were successfully converted to the analogous pyranopyrazole derivatives under the optimized reaction conditions. It was found that the ZnO nano structured material effectively catalyzes the reaction within 15–30 min at room temperature affording good to excellent yield of the corresponding derivatives. These results are depicted in Table 4.
Table 4 Synthesis of pyranopyrazole derivatives
Reaction conditions: Isolated yield after chromatographic separation. Reaction conditions: ethyl acetoacetate (1.0 mmol), hydrazine hydrate (1.0 mmol), aldehyde (1 mmol), malononitrile (1 mmol) were stirred in ethanol–water for 10–15 min at room temperature, catalyst (5 mol%). |
|
Unsubstituted aromatic aldehyde (benzaldehyde) afforded 90% yield (Table 4, entry 5a); however amongst the halogenated aldehydes, 4-chlorobenzaldehyde (Table 4, entry 5b) furnished 98% yield of the resultant pyranopyrazole derivative within 10 min of reaction time, whereas other halogenated aldehydes afforded more than 90% yield (Table 4, entries 5e and 5i). The reaction of 4-nitrobenzaldehyde was completed within 10 min giving 94% yield of the corresponding product (Table 4, entry 5c). Aldehydes having electron releasing groups also reacted within 15–30 min with good to excellent yield (85–90%) of pyranopyrazole derivatives. (Table 4 entries 5d, 5g and 5j). It was observed that the majority of reactions proceed at a faster rate with ZnO nanostructured material at room temperature. Das et al. have reported nano crystalline ZnO (10 mol%) catalyzed one pot multicomponent reactions for the synthesis of pyranopyrazole derivatives in water
:
ethanol at room temperature affording 83 to 98% yield in 3 h.40
Recycling study
The reusability of the catalyst was also studied under the optimized reaction conditions. After completion of the reaction the catalyst was recovered, filtered and washed repeatedly with ethyl acetate and dried.
It was reused under the optimized reaction conditions and it was found that the yields of benzoxanthene and pyranopyrazole were almost comparable. The catalyst recovered after the first cycle was used for the subsequent four cycles and the yield of the corresponding product obtained in each cycle is summarized in Table 5. It was observed that the % yield of these derivatives was negligibly lowered even after five cycles. These observations suggest that the catalyst surface remains active during successive cycles. Although the slight decrease in the catalytic activity of ZnO might be due to the deactivation of the active sites of the catalyst. Also, control experimental study of a commercial ZnO sample (bulk size) with as synthesized ZnO nanostructures was carried out. In comparison with the as-synthesized ZnO nanostructures the commercial ZnO sample (bulk size) shows 40 to 50% less reaction yields.
Table 5 Reusability of the catalyst
Entry |
Number of cycles |
Yielda (%) |
Isolated yield.
Used catalyst from fourth recycle, heated at 150 °C for 60 min in a N2 atmosphere. Reaction conditions: 1 : 1 : 1 mmol reactant with catalyst (5 mol%) were stirred in 5 mL ethanol.
|
1 |
Fresh |
95 |
2 |
First |
92 |
3 |
Second |
89 |
4 |
Third |
85 |
5 |
Fourth |
80 |
6b |
Fifth |
94 |
Plausible reaction mechanism for the synthesis of benzoxanthene
A plausible reaction mechanism for the synthesis of benzoxanthene derivatives using hierarchical ZnO nanostructures is illustrated in Scheme 4. Hierarchical ZnO nanostructure co-ordinate with carbonyl oxygen and enhance the electrophilicity of the carbonyl carbon of aldehydes (1) followed by subsequent attack of dimedone to give an alkene intermediate (2). The nucleophilic attack of an oxygen atom of β-naphthol (3) to the electron deficient intermediate (2) affords intermediate (4). The subsequent elimination of hydrogen followed by a water molecule (5–6) results in the desired benzoxanthene derivatives (7).
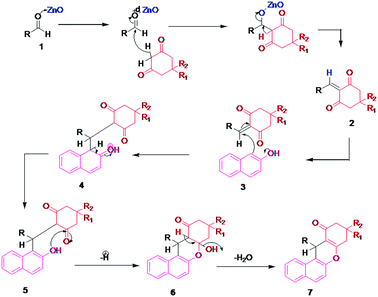 |
| Scheme 4 Proposed reaction mechanism for the synthesis of benzoxanthene derivatives. | |
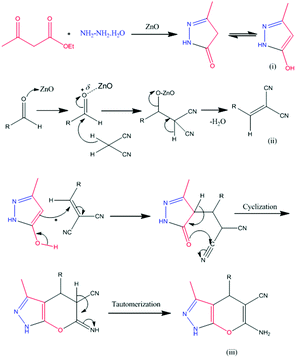 |
| Scheme 5 Proposed reaction mechanism for the synthesis of pyranopyrazole derivatives. | |
Plausible reaction mechanism for the synthesis of pyranopyrazole
Ethyl acetoacetate is activated by ZnO nanostructures followed by attack of hydrazine, which results in the formation of pyrazolone, intermediate (i). Also, the carbonyl group of aldehyde is activated by nanostructured ZnO and then it undergoes Knoevenagel condensation with malononitrile to afford intermediate (ii). The interaction between intermediate (i) and intermediate (ii) enables Michael addition type reaction. The intramolecular cyclization followed by tautomerization gives the corresponding pyranopyrazole derivatives (iii) (Scheme 5).
Conclusions
In a nutshell, we have successfully used a hydrothermal technique for the synthesis of a highly crystalline hexagonal ZnO nanostructure having spherical and petal like mixed morphology. Furthermore, the effective use of the synthesized hierarchical ZnO nanostructures as a heterogeneous catalyst was evaluated for the synthesis of benzoxanthene and pyranopyrazole derivatives via one-pot multi-component reactions in good to high yields under ambient reaction conditions. In addition, the catalyst could be easily recovered by simple filtration and reused for several cycles without significant loss in its catalytic activity.
Conflicts of interest
There are no conflicts to declare.
Acknowledgements
The authors sincerely acknowledge Centre for materials for electronics technology (C-MET) Pune and Haribhai V. Desai College, Pune for characterization. We also thank Ness Wadia Nanomaterial Research Centre, NowrosjeeWadia College, Pune for providing lab facilities.
References
- L. Weber, K. Illegen and M. Almstetter, Synlett, 1999, 366–374 CrossRef CAS.
- N. Koukbai, E. Kolvari, A. Khazaei, M. A. Zolfigol, B. Shirmardi-Shaghasemi and H. R. Khavasi, Chem. Commun., 2011, 47, 9230–9232 RSC.
- J. P. Poupelin, G. Saint-Ruf, O. Foussard-Blanpin, G. Marcisse, G. Uchida-Ernouf and R. Lacroix, Eur. J. Med. Chem., 1978, 13, 67–71 CAS.
-
R. W. Lambert, J. A. Martin, J. H. Merrett, K. E. B. Parkes and G. J. Thomas, PCT Int. Appl. WO9706178, 1997 Search PubMed.
-
T. Hideo and J. Teruomi (Sankyo Co), Japan Pat., 56005480, 1981 Search PubMed.
-
S. M. Menchen, S. C. Benson, J. Y. L. Lam, W. Zhen, D. Sun, B. B. Rosenblum, S. H. Khan and M. Taing, US Pat. 6583168, June 24, 2003 Search PubMed.
- C. G. Knight and T. Stephens, Biochem. J., 1989, 258, 683–687 CrossRef CAS.
- M. Ahmad, T. A. King, D. K. Ko, B. H. Cha and J. J. Lee, J. Phys. D: Appl. Phys., 2002, 35, 1473–1476 CrossRef CAS.
- J. Li, W. Tang, L. Lu and W. Su, Tetrahedron Lett., 2008, 49, 7117–7120 CAS.
- G. M. Nazeruddin, M. S. Pandharpatte and K. B. Mulani, Indian J. Chem., 2011, 50B, 1532–1537 CAS.
- K. G. Verma, K. Raghuvanshi, R. K. Verma, P. Dwivedi and M. S. Singh, Tetrahedron, 2011, 67, 3698–3704 Search PubMed.
- G. C. Nandi, S. Samai, R. Kumar and M. S. Singh, Tetrahedron, 2009, 65, 7129–7134 CAS.
- Y. B. Shen and G. W. Wang, ARKIVOC, 2008, xvi, 1–8 Search PubMed.
- B. Das, P. Thirupathi, K. Ravinder Reddy, B. Ravikanyh and L. Nagarapu, Catal. Commun., 2006, 737–742 Search PubMed.
- T. S. Jin, J. S. Zhang, A. Q. Wang and T. S. Li, Ultrason. Sonochem., 2006, 13, 220–224 CrossRef CAS.
- T. S. Jin, J. S. Zang, J. C. Xiao, A. Q. Wang and T. S. Li, Synlett, 2004, 866–870 CrossRef CAS.
- H. N. Karade, M. Sathe and M. P. Kaushik, ARKIVOC, 2007, xiii, 252–258 Search PubMed.
- M. Kidwai, K. Singhal and S. Kukreja, Can. J. Chem., 2008, 86, 799–802 CrossRef CAS.
- S. Kantevari, R. Bantu and L. Nagarapu, ARKIVOC, 2006, xvi, 136–148 Search PubMed.
-
G. P. Ellis, The Chemistry of Heterocyclic Compounds. Chromenes, Chromanes and Chromones, ed. A. Weissberger and E. C. Taylor, John Wiley, New York, 1977, ch. 11, p. 11 Search PubMed.
- E. A. Hafez, M. H. Elnagdi, A. G. A. Elagemey and F. M. A. A. El-Taweel, Heterocycles, 1987, 26, 903–907 CrossRef CAS.
- M. A. Sofan, F. M. A. A. El-Taweel and M. H. Liebigs Elnagdi, Ann. Chem., 1989, 935–936 CAS.
- A. Galil, F. B. Riad, S. Sherif and M. Elnagdi, Chem. Lett., 1982, 1123–1126 CrossRef.
- M. Kidwai, S. Saxena, M. Khan and S. Thukral, Bioorg. Med. Chem. Lett., 2005, 15, 4295–4298 CrossRef CAS.
- J. Li, L. Lu and W. Su, Tetrahedron Lett., 2010, 51, 2434–2437 CrossRef CAS.
- J. M. Khurana and D. Magoo, Tetrahedron Lett., 2009, 50, 4777–4780 CrossRef CAS.
- Q. Zhang, H. Su, J. Luo and Y. Wei, Green Chem., 2012, 14, 201–208 RSC.
- P. Ghosh and A. Das, J. Org. Chem., 2013, 78, 6170–6181 CrossRef CAS.
- K. Kong, H. L. Wang, D. Fang and Z. L. Liu, Catal. Commun., 2008, 9, 650–673 CrossRef.
- N. Sakai, G. K. Prasad, Y. Ebina, K. Takada and T. Sakai, Chem. Mater., 2006, 18, 3596–3598 CrossRef CAS.
- D. Shao, M. Yu, H. Sun, T. Hu, J. Lian and S. Swayer, Nanoscale, 2013, 5, 3664–3667 RSC.
- P. S. Mane, W. J. Lee, H. M. Pathan and S. H. Han, J. Phys. Chem. B, 2005, 109, 24254–24259 CrossRef.
- N. Madhusudhana, K. Yogendra and K. M. Mahadevan, Res. J. Chem. Sci., 2012, 2(5), 72–77 Search PubMed.
- O. Seven, B. Dindar, S. Aydemir, D. Metin, M. A. Ozinel and S. Icli, J. Photochem. Photobiol., A, 2004, 165, 103–107 CrossRef CAS.
- D. G. Kumbhar, V. U. Pandit, S. D. Deshmukh, J. D. Ambekar, S. S. Arbuj and S. B. Rane, J. Nanoeng. Nanomanuf., 2015, 5, 227–231 CrossRef CAS.
- V. U. Pandit, S. S. Arbuj, R. Hawaldar, P. V. Kshirsagar, U. P. Mulik, S. W. Gosavi, C. J. Park and B. B. Kale, J. Mater. Chem. A, 2015, 3, 4338–4344 RSC.
- V. U. Pandit, S. S. Arbuj, U. P. Mulik and B. B. Kale, Environ. Sci. Technol., 2014, 48, 4178–4183 CrossRef CAS.
- V. U. Pandit, S. S. Arbuj, Y. B. Pandit, S. D. Naik, S. B. Rane, U. P. Mulik, S. W. Gosawi and B. B. Kale, RSC Adv., 2015, 5, 10326–10331 RSC.
- S. S. Arbuj, N. Rumale, A. Pokle, J. D. Ambekar, S. B. Rane, U. P. Mulikand and D. P. Amalnerkar, Sci. Adv. Mater., 2014, 6, 269–275 CrossRef CAS.
- P. Bhattacharyya, K. Pradhan, S. Paul and A. R. Das, Tetrahedron Lett., 2012, 53(35), 4687–4691 CrossRef CAS.
Footnote |
† Electronic supplementary information (ESI) available. See DOI: 10.1039/d0ma00403k |
|
This journal is © The Royal Society of Chemistry 2020 |
Click here to see how this site uses Cookies. View our privacy policy here.