DOI:
10.1039/D0NA00376J
(Paper)
Nanoscale Adv., 2020,
2, 3542-3549
Bottom-up synthesis of titanophosphate nanosheets by the aqueous solution process†
Received
8th May 2020
, Accepted 7th July 2020
First published on 7th July 2020
Abstract
The synthesis of titanophosphate nanosheets in aqueous sols was examined by the bottom-up process. The nanosheets were formed by mixing titanium iso-propoxide, phosphoric acid, and tetraalkylammonium hydroxide (NR4OH) aqueous solutions, followed by diluting with water and heating at 80 °C, forming translucent aqueous sols of titanophosphate nanosheets with the same crystal structure as layered titanium phosphate Ti2O3(H2PO4)2·2H2O. Whether the nanosheets were crystallized depended on the reactions during the mixing of reagents before the water dilution. By controlling the acid–base reactions between the Ti species, phosphoric acid, and the hydroxides of bulky cations in the aqueous sols, the one-pot process yielded highly water-dispersible, flake-like titanophosphate nanosheets. Under some synthetic conditions, nanosheets formed even in weakly basic aqueous sols. These nanosheets can be coated on a substrate with low alkali-resistance, or used for the removal of metal ions from neutral aqueous solutions.
Introduction
Metalate nanosheets are two-dimensional materials with highly anisotropic shapes; accordingly, they have attracted great interest. Electronic devices built from metalate nanosheets exhibit intriguing properties.1–4 Aqueous sols of metalate nanosheets with large lateral size also possess interesting features, such as liquid crystallinity, optical trapping properties, and laser-induced mesostructual manipulation.5–7 Conventionally, metalate nanosheets are synthesized by two-step ion exchanges, which intercalate bulky cations such as N(C4H9)4+ into the interlayers of layered metalates.8–12 The intercalation of bulky cations leads to the exfoliation of layered metalates and the consequent formation of metalate nanosheets. Alternatively, we have formed metalate nanosheets by a bottom-up synthesis in aqueous solution.12–18 The chemical reactions in the sols form layered metalates with bulky cations in their interlayers. These layered metalates are exfoliated, yielding metalate nanosheets as highly water-dispersible colloidal particles, which can be applied as coating sols for thin film fabrication by solution processes.13,19 In our previous work, many types of metalate nanosheets were synthesized in strongly basic aqueous sols, which can corrode the substrates. To avoid this problem, bottom-up synthesis of metalate nanosheets in acidic or neutral aqueous sols is desirable. Because they flocculate, metalate nanosheets are also useful for the removal of metal ions from aqueous solutions. A further advantage of this application is the high dispersibility of nanosheets synthesized by the bottom-up process. However, metalate nanosheets synthesized by the bottom-up process are partially amorphous in neutral and acidic aqueous solutions.20 Bottom-up synthesis in acidic or neutral aqueous solutions would expand the range of removal metal ions by the metalate nanosheets.
Titanate nanosheets (Ti1.825O40.7−) dispersed in transparent aqueous sols have been synthesized by mixing titanium iso-propoxide (Ti[OCH(CH3)2]; TIP) and tetramethylammonium hydroxide (N(CH3)4OH; TMAOH) aqueous solution in water by the bottom-up process.12 The acid–base reactions between TMAOH and the TIP hydrolysis product Ti(OH)4 yield layered titanates with interlayered cations TMA+ in aqueous sols. The layered titanates are swollen and exfoliated, to form the titanate nanosheets. In such acid–base reactions, Ti(OH)4 behaves as a very weak acid, so the aqueous solutions must be strongly basic. Instead, we envisaged the bottom-up synthesis of nanosheets using Ti(OH)4 as a base. In this case, layered titanium phosphates would be formed by the acid–base reaction between phosphoric acid H3PO4 and Ti(OH)4. Sequential acid–base reactions between the resulting layered titanium phosphates and TMAOH would lead to the exfoliation of the layered titanium phosphates, forming titanophosphate nanosheets. Mixing of TIP, H3PO4, and TMAOH can potentially yield titanophosphate nanosheets in neutral aqueous sols.
Nanostructured titanium phosphates such as layered titanium phosphates can be used in various applications.21–25 Among the known crystal structures of layered titanium phosphates are α-titanium phosphate (Ti(HPO4)2 H2O),26 γ-titanium phosphate (Ti(H2PO4)PO4 2H2O),26 π-titanium phosphate (Ti2O(PO4)2 H2O),27,28 and ρ-titanium phosphate (Ti2O(PO4)2 H2O).27,28 These layered titanium phosphates uptake alkaline and alkaline earth metal ions in aqueous solutions through ion-exchange reactions.27 Yada et al. reported the presence of various micro- and nano-structures in layered titanium-phosphate thin films, which affect their wettabilities in water-drop test.29 Moreover, nanocomposite thin films of silver nanoparticles and layered silver titanophosphates exhibit excellent antibacterial activities.30 Different inorganic-organic nanocomposites of layered titanium phosphates and organic species have been extensively studied as metal species removers from aqueous solutions.31–35 These layered titanium phosphates are hydrothermally synthesized by the bottom-up process in aqueous sols, but the bottom-up synthesis of titanophosphate nanosheets has not been reported. When forming titanophosphate nanosheets in aqueous sols, the acid–base reactions between Ti4+ species, phosphate ions, and bulky cations must be properly controlled.
This study has several objectives. We first synthesize titanophosphate nanosheets by the bottom-up process in aqueous sols, and then examine their formation mechanism. To this end, we study how the reactions between the Ti4+ species, phosphate ions, and bulky cations affect the titanophosphate nanosheet formation. Based on the obtained results, we also investigate the synthesis of titanophosphate nanosheets by the bottom-up process in neutral or very weakly basic aqueous sols. Finally, we investigate whether neutral or very weakly basic aqueous sols of titanophosphate nanosheets can form thin films on substrates with low alkali resistance, and/or remove metal ions from neutral aqueous solutions.
Experimental
Materials
TIP (1st grade), 85% phosphoric acid (guaranteed reagent), 2-propanol (1st grade), and copper acetate monohydrate (special grade) were purchased from FUJIFILM Wako Pure Chemical Co. Prior to use, TIP was distilled and 2-propanol was both dehydrated and distilled. The other reagents were used as received. The reagents of the tetraalkylammonium hydroxides are listed in Table 1.
Table 1 NR4OH reagents used
|
Abbreviation |
Concentration |
|
N(CH3)4OH |
TMAOH |
25% (2.8 M) |
FUJIFILM Wako pure chemical (special grade) |
15% (1.6 M) |
FUJIFILM Wako pure chemical (analytical grade) |
6% (0.7 M) |
(Dilution of 15% TMAOH) |
N(C2H5)4OH |
TEAOH |
20% (1.4 M) |
FUJIFILM Wako pure chemical (1st grade) |
10% (0.7 M) |
FUJIFILM Wako pure chemical (special grade) |
N(C3H7)4OH |
TPAOH |
23% (1.1 M) |
Tokyo chemical industry co. (The concentration was determined by titration) |
10% (0.5 M) |
FUJIFILM Wako pure chemical (special grade) |
N(C4H9)4OH |
TBAOH |
40% (1.5 M) |
Tokyo chemical industry co. |
20% (0.8 M) |
(Dilution of 40% TMAOH) |
Synthesis
Layered titanium phosphates were synthesized as follows: TIP (8.0 mmol) and 85% H3PO4 solution containing 8.0 mmol H3PO4 were mixed at room temperature for 2 h. The mixture was diluted by distilled water to 40 mL, and then stirred for 2 h. The Ti concentration in the reaction mixtures was 0.2 mol L−1, and the molar ratio of TIP/H3PO4 was varied from 1/1 to 1/20. The aqueous mixtures were then transferred to a closed vessel, and heated at 60–150 °C for 1 day. The resultant precipitates containing layered titanium phosphate were collected by centrifugation, and then washed three times with 2-propanol.
Titanophosphate nanosheets were synthesized by the bottom-up process as follows: TIP (0.336 mmol), 85% H3PO4 solution (0.336 mmol of H3PO4), and tetraalkylammonium hydroxide (NR4OH) aqueous solution were mixed at room temperature for 2 h. The used NR4OH solutions are listed in Table 1. The mixture was diluted to 16.8 mL with distilled water. The Ti concentration in the resulting reaction sols was 0.2 mol L−1, and the molar ratio of TIP/H3PO4 was 1/1. The reaction sols were transferred into a closed vessel, and heated at 80 °C for 1 day, forming titanophosphate nanosheet sols.
The influence of the mixing sequence of TIP, H3PO4, and TMAOH was investigated as follows: first, two out of the three reagents [TIP, 85% H3PO4 solution, and 25% TMAOH aqueous reagent (2.8 M)] were mixed at room temperature for 1 day. Next, the other reagent was added, and the mixture was stirred for 2 h. The molar ratio of the starting materials was TIP
:
H3PO4
:
TMAOH = 1
:
1
:
2. The mixture was diluted to 0.2 mol-Ti L−1 with distilled water, and stirred at room temperature for 2 h. Finally, the resultant reaction sols were transferred to a closed vessel, and heated at 80 °C for 1 day.
The corrosion of glass substrates by titanophosphate nanosheet sols was investigated on Corning Eagle glass and borosilicate slide glass. The titanophosphate nanosheet sols (200 μL) were placed on the glass substrates (13 mm × 35 mm). The application was limited to 200 μL to avoid spillage of the sols from the substrate. The sols were evaporated on the substrates under ambient conditions.
The removal of aqueous Cu2+ by titanophosphate nanosheets was investigated as follows: copper acetate monohydrate (Cu(OCOCH3)2 H2O; 10 mmol) was dissolved in 20 mL of water. The titanophosphate nanosheet sols prepared at a TPAOH/TIP molar ratio of 2.2 were evaporated at approximately 4 kPa. After adding the resulting titanophosphate nanosheets (70 mg) to the copper acetate aqueous solution, the mixture was stirred for 3 days at room temperature. The precipitates and supernatant were then separated by centrifugation, and the precipitates were washed in mixed 2-propanol and water for 3 min by centrifugation at 6000 rpm.
Characterization
X-ray diffraction (XRD) measurements were performed on a Rigaku Ultima IV diffractometer with monochromatic CuKα irradiation. The XRD patterns were recorded at 2° min−1 in the 2θ range 2° to 70°.
Transmission electron microscopy (TEM) images were captured by a JEOL JEM-2100 system at an accelerating voltage of 200 kV. The samples were prepared by evaporating a drop of sample sol onto a Cu grid supported with a Formvar thin film. The Cu grid was subjected to hydrophilic treatment before use.
The Raman spectra were acquired on a JASCO NRS-1000 model using a 532 nm incident beam in the Raman shift range 200–1500 cm−1. The exposure period was 5–20 s. The number of accumulations was set to 3.
Atomic force microscope (AFM) images were captured on a Seiko instrument SPI3800 model by using a silicon tip cantilever (16 N m−1) in tapping mode. The samples were prepared by dropping diluted nanosheet sols on a mica substrate, followed by drying at 100 °C for 10 min.
Ultraviolet-visible (UV-vis) spectra were measured on a Hitachi U-4100 model using a dual beam in transmittance mode. The samples in solution were transferred into the quartz glass cuvette (10 × 10 × 45 mm3), and their spectra were recorded from 1000 nm to 200 nm at 300 nm min−1.
The pH values of the aqueous sols were measured by a Horiba D-54 model. The electrode for measurements of strongly basic solutions (Horiba 9632-10D model) was used.
Results and discussion
Bottom-up synthesis of layered titanium phosphate in aqueous solution
Titanophosphate nanosheets are prepared by exfoliating layered titanium phosphates. Some of the known types of layered titanium phosphates were described in the Introduction section. We first examined the crystal structure of layered titanium phosphate synthesized by the bottom-up process. TIP and H3PO4 were mixed at a molar ratio of 1
:
1, yielding an amorphous mixture. After heating at 60–150 °C, layered titanate with a chemical composition of Ti2O3(H2PO4)2·2H2O31 was obtained (Fig. 1). The Ti2O3(H2PO4)2·2H2O crystallized at low pH (∼2). When H3PO4 was added in large amounts (i.e., at H3PO4/TIP ≥ 5) and the mixture was heated at 100 °C, α-layered titanium phosphate was formed (Fig. S1 in the ESI†). However, under the synthetic conditions of the present study, other layered titanium phosphates were not formed.
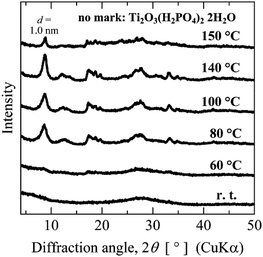 |
| Fig. 1 XRD patterns of layered titanium phosphates synthesized by heating the aqueous mixture of TIP and H3PO4 at different temperatures. | |
Fig. 2b shows the morphology (TEM image) of the layered titanium phosphate Ti2O3(H2PO4)2·2H2O synthesized at 80 °C. The titanium phosphate nanosheets were precipitated as agglomerated particles approximately 1 μm in size (Fig. 2a). Thus, the titanium phosphate nanosheets were probably agglomerated in the aqueous sols, but not exfoliated.
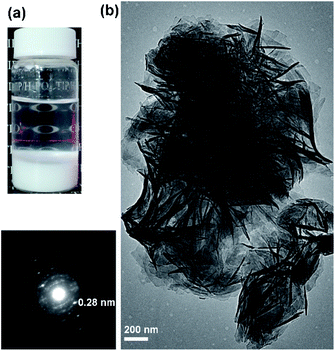 |
| Fig. 2 (a) Appearance, (b) TEM images, and electron diffraction pattern of layered titanium phosphates synthesized by heating the aqueous mixture of TIP and H3PO4 at 80 °C. | |
Bottom-up synthesis of titanophosphate nanosheets in aqueous sols
The bottom-up synthesis of titanophosphate nanosheets was examined by reacting TIP, H3PO4, and NR4OH in aqueous sols. As mentioned above, a 1
:
1 molar ratio of TIP and H3PO4 reacted to form a layered titanium phosphate Ti2O3(H2PO4)2·2H2O. The added NR4OH is expected to react with Ti2O3(H2PO4)2·2H2O by an acid–base reaction, intercalating the bulky cations NR4+ into the interlayers of the layered titanium phosphate, and eventually forming titanophosphate nanosheets by the swelling and exfoliation of layered titanium phosphate. In this experiment, TIP, 85% H3PO4 solution, and 25% TMAOH solution were mixed at a molar ratio of 1/1/2 and then diluted by water. The pH of the sol was approximately 8. After heating the mixtures at 80 °C, translucent aqueous sols were yielded (Fig. 3a), which were evaporated on a glass substrate. In the XRD patterns of the obtained thin films, the d-spacings between the peaks were ordered as 1
:
1/2
:
1/3, indicating a highly oriented layered compound (Fig. 3b). That is, titanophosphate nanosheets were formed by the bottom-up process in aqueous sols. After synthesis, the nanosheet sols remained intact even after several months of storage. Moreover, the basal spacing of the layered compounds (∼1.7 nm, obtained in stacked titanophosphate nanosheets) was independent of the type of alkylammonium ions except in the synthesis using TBAOH (see Fig. S2 in the ESI†). The spacing was larger in the layered compounds than in Ti2O3(H2PO4)2·2H2O (1.0 nm). The basal spacing was probably largely influenced by the water molecules in the interlayer. Meanwhile, the Raman spectra of the titanophosphate nanosheets and layered titanium phosphate were similar, but with shifted peaks (Fig. 3c). The removal of H+ from the Ti2O3(H2PO4)2 layers and the exfoliation process likely distorted the titanophosphate layers. The Raman spectra of the titanophosphate nanosheets prepared from TEAOH and TPAOH were also measured (Fig. S3 in the ESI†). The Raman spectra were independent of the NR4OH type used in the synthesis, indicating that the titanophosphate nanosheets prepared from TEAOH and TPAOH had the same structure as those prepared from TMAOH.
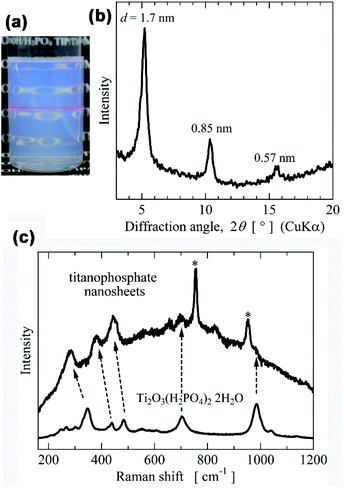 |
| Fig. 3 (a) Appearance, (b) XRD pattern and (c) Raman spectra of titanophosphate nanosheets synthesized by heating of the aqueous mixture of TIP, H3PO4, and TMAOH with a molar ratio of TIP : H3PO4 : TMAOH = 1 : 1 : 2 at 80 °C. The marks * are assigned to TMA+ ion. | |
The morphology of the titanophosphate nanosheets was investigated by TEM. The titanophosphate nanosheets were flake-like shapes (Fig. 4a) with much smaller lateral sizes (5 to 50 nm) than layered titanium phosphate Ti2O3(H2PO4)2·2H2O. Moreover, in high-resolution TEM images, lattice fringes with an interval of ∼0.28 nm were observed (Fig. 4b). This interval was very close to the d-spacing of diffraction spot in the electron diffraction of Ti2O3(H2PO4)2·2H2O (see Fig. 2). These results confirmed the similar crystal structure of titanophosphate nanosheets and Ti2O3(H2PO4)2·2H2O. Furthermore, the AFM images of the titanophosphate nanosheets revealed many flake-like nanosheets of monolayer thickness (∼1.5 nm, Fig. 4c). We surmised that many of the titanophosphate nanosheets existed as monolayers in the aqueous sols.
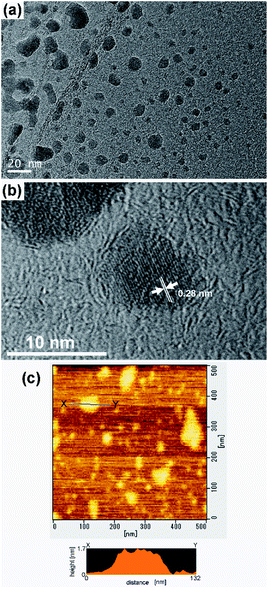 |
| Fig. 4 (a and b) TEM and (c) AFM images of titanophosphate nanosheets synthesized by heating of the aqueous mixture of TIP, H3PO4, and TMAOH with a molar ratio of TIP : H3PO4 : TMAOH = 1 : 1 : 2 at 80 °C. | |
Influence of mixing sequence of the reagents on the formation of titanophosphate nanosheets
Titanophosphate nanosheets were synthesized by acid–base reactions between H3PO4 and Ti(OH)4, followed by acid–base reactions between the obtained Ti2O3(H2PO4)2·2H2O and NR4OH. Thus, the addition sequence of TIP, H3PO4 and NR4OH should influence the formation of titanophosphate nanosheets. To explore this influence, two of three reagents [TIP, H3PO4 (85%), and TMAOH (25%)] were mixed for 1 day. Next, the other reagent was added, and the mixture was stirred for 2 h, then diluted with water. The pH of the sols prepared by all mixing sequence was approximately 8. Finally, the mixture was heated at 80 °C for 1 day. Fig. 5 shows the experimental results of mixing TIP
:
H3PO4
:
TMAOH at a molar ratio of 1
:
1
:
2 and heating at 80 °C.
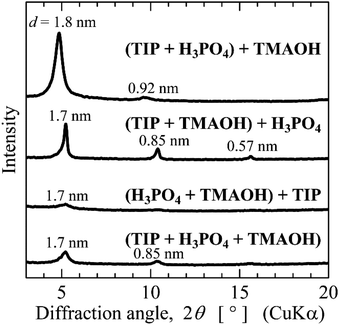 |
| Fig. 5 XRD patterns of the thin films fabricated by evaporating the titanophosphate nanosheet sols prepared by different mixing sequences of TIP, 85% H3PO4 solution, and 25% TMAOH solution at a molar ratio of TIP : H3PO4 : TMAOH = 1 : 1 : 2. | |
Although adding the reagents in any sequence yielded titanophosphate nanosheets, the amount of the formed nanosheets depended on the addition sequence, and increased in the following order: (H3PO4 + TMAOH) + TIP < (TIP + H3PO4 + TMAOH) < (TIP + TMAOH) + H3PO4 < (TIP + H3PO4) + TMAOH. The nanosheet yield was maximized by initially mixing TIP and H3PO4 and later adding TMAOH. This indicates that the proposed reactions − formation of layered titanium phosphate by the acid–base reaction of TIP and H3PO4, followed by the acid–base reaction of layered titanium phosphate and TMAOH − achieved the bottom-up synthesis of titanophosphate nanosheets.
The initial mixing of TIP and TMAOH followed by H3PO4 addition also obtained a high nanosheet yield. The TIP and TMAOH formed titanate nanosheet sols. When H3PO4 was added, it favourably reacted with the titanate nanosheets to form titanophosphate nanosheets.
The initial mixing of H3PO4 and TMAOH followed by TIP addition provided the smallest nanosheet yield. The neutral solution formed by mixing H3PO4 and TMAOH probably reduced the reactivity of the Ti species formed by TIP hydrolysis. Thus, the reaction of the Ti species with H3PO4 or NR4OH played an important role in the bottom-up synthesis of the titanophosphate nanosheets.
One-pot synthesis of titanophosphate nanosheets by using different NR4OH reagents
NR4OH aqueous solution reagents with different concentrations are commercially available. To test whether the concentrations of NR4OH reagents influence the nanosheet formation, the NR4OH concentrations and types were varied in the one-pot synthesis of titanophosphate nanosheet sols. The NR4OH solutions (TMAOH, TEAOH, TPAOH, and TBAOH) were added at low (0.5–0.8 M) and high (1.1–1.6 M) concentrations, and the obtained products were compared. First, the TIP, 85% H3PO4 aqueous solution and NR4OH solutions were mixed for 2 h, and the mixtures were diluted with water. For all samples, the Ti and P concentrations were adjusted to 0.2 M by the H2O addition. The pH values of the sols depended on the NR4OH/Ti4+ ratios as shown in Table 2, but were independent of the types and concentrations of the NR4OH solutions. To crystallize the titanophosphate nanosheets, the aqueous mixtures were heated at 80 °C for 1 day. Fig. 6 compares the XRD patterns of the thin films fabricated from the TIP
:
H3PO4
:
TPAOH = 1
:
1
:
2.2 sols prepared at low and high concentrations of TPAOH. When added at low concentrations, the TPAOH solutions obtained very high yields of titanophosphate nanosheets, but the high-concentration TPAOH solutions obtained very few nanosheets. Obviously, the concentration of the TPAOH reagent influenced the titanophosphate nanosheet formation. Thus, the crystallization of titanophosphate nanosheets was determined by the reactions during the mixing before dilution by water.
Table 2 Influence of the concentration of NR4OH solutions on the crystallization of titanophosphate nanosheetsa
x= |
1TIP : 1H3PO4 : xNR4OH |
2.0 (pH 8) |
2.2 (pH 9) |
2.3 (pH 10) |
2.5 (pH 11) |
The marks OO, O, Δ, and × represent that the amounts of formed titanophosphate nanosheets were very large, large, medium, and small, respectively.
|
High concentration
|
TMAOH (1.6 M) |
× |
× |
× |
O |
TEAOH (1.4 M) |
Δ |
× |
× |
× |
TPAOH (1.1 M) |
× |
× |
× |
× |
TBAOH (1.5 M) |
× |
× |
× |
× |
![[thin space (1/6-em)]](https://www.rsc.org/images/entities/char_2009.gif) |
Low concentration
|
TMAOH (0.7 M) |
× |
× |
Δ |
Δ |
TEAOH (0.7 M) |
× |
Δ |
Δ |
O |
TPAOH (0.5 M) |
Δ |
OO |
OO |
O |
TBAOH (0.8 M) |
× |
× |
× |
× |
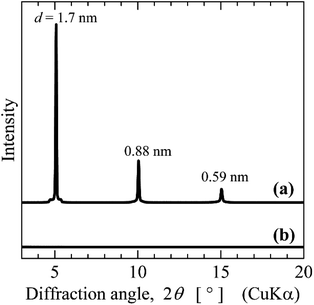 |
| Fig. 6 XRD patterns of the thin films fabricated by evaporating the titanophosphate nanosheet sols prepared by using TPAOH solutions with (a) low and (b) high concentrations (0.5 and 1.1 M) at a molar ratio of TIP : H3PO4 : TPAOH = 1 : 1 : 2.2. | |
Table 2 lists the titanophosphate nanosheet syntheses in various NR4OH solutions at different molar ratios of NR4OH/Ti4+. The amounts of synthesized titanophosphate nanosheets were evaluated from the peak intensities in the XRD patterns of the thin films formed from the nanosheet sols. The XRD measurements of some samples were performed on powders prepared by evaporating the nanosheet sols (Fig. S4 in the ESI†). The high correlation of XRD peak intensities between powder and thin film samples confirmed that the amount of titanophosphate nanosheets can be determined from the XRD patterns of the thin films fabricated from nanosheet sols.
In general, the diluted NR4OH solutions yielded more titanophosphate nanosheets than highly concentrated solutions, but there were some exceptions, such as TMAOH/Ti4+ = 2.5. As mentioned above, the initial mixing of NR4OH with H3PO4 produced few titanophosphate nanosheets, whereas reacting Ti species with NR4OH or H3PO4 facilitated the nanosheet formation. These results imply that highly concentrated NR4OH solutions facilitated the reactions of NR4OH with H3PO4, whereas lowly concentrated NR4OH solutions facilitated the reactions of Ti species with NR4OH or H3PO4. Moreover, increasing the length of the alkyl chain in NR4+ enhanced the effect of NR4OH concentration on the titanophosphate nanosheet formation, and extended the region of nanosheet formation toward neutral conditions (except in the TBAOH cases). The alkyl chain length and hydration of NR4+ probably largely influenced the titanophosphate nanosheet formation. Therefore, we infer that hydration of an appropriate NR4+ type will facilitate the reactions of NR4OH with Ti species.
Fig. 7a shows the XRD patterns of the layered titanophosphate thin films fabricated by evaporating the titanophosphate nanosheet sols prepared from highly concentrated TMAOH and TBAOH and lowly concentrated TEAOH and TPAOH (NR4OH/Ti4+ = 2.5). The XRD pattern of the sample prepared from lowly concentrated TPAOH (TPAOH/Ti4+ = 2.0–2.5) is shown in Fig. 7b. Fig. 7 shows the results of the samples yielding the largest amounts of titanophosphate nanosheets for each NR4OH. At a NR4OH/Ti4+ ratio of 2.5, the yields of titanophosphate nanosheets were similar in TMAOH, TEAOH, and TPAOH, but TBAOH yielded few nanosheets. Likely, the TBA+ ion was too large and bulky to intercalate in the layered titanophosphate. In contrast, the TPAOH preparation obtained a large amount of titanophosphate nanosheets even in very weakly basic sols (pH ∼ 9 for TPAOH/Ti4+ = 2.2). TEM images of the formed nanosheets are shown in Fig. S5a in the ESI.† Flake-like nanosheets were observed. TEM images of the titanophosphate nanosheets synthesized from other sols are given in Fig. S5 in the ESI.† The lateral size of the synthesized titanophosphate nanosheets ranged from approximately 5 nm to 20 nm, and the structures were flake-like. As mentioned above, the 25% TMAOH reagent (2.8 M) at a TMAOH/Ti4+ ratio of 2.0 also obtained a relatively high nanosheet yield at pH 8, when the adding sequence was (TIP + H3PO4) + TMAOH. Thus, under the appropriate synthetic conditions, titanophosphate nanosheets were formed even in very weakly basic aqueous sols.
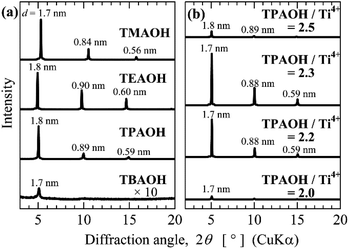 |
| Fig. 7 XRD patterns of the thin films fabricated by evaporating (a) the titanophosphate nanosheet sols prepared by using different NR4OH solutions at a molar ratio of TIP : H3PO4 : NR4OH = 1 : 1 : 2.5, and (b) the nanosheet sols prepared by using 0.5 M TPAOH solutions at different TPAOH/TIP molar ratios of 2.0 to 2.5. | |
Influence of the basicity of titanophosphate nanosheet sols on the corrosion of substrates
Borosilicate glass may corrode in basic aqueous solutions, because silicate or borate reacts with OH− ion. The corrosion of glass substrates might contaminate thin film with alkaline ions released from the glass, thereby degrading its quality. As the titanophosphate nanosheet sols were synthesized in very weakly basic aqueous sols by the bottom-up process, glass substrates should not be corroded by the sols. Therefore, the aqueous sols in this study are suitable for thin-film fabrication by the sol–gel method or the chemical bath deposition method. To verify that the corrosion of glass substrates was inhibited by our method, we tested two glass substrates: Corning Eagle glass and a borosilicate slide glass with high and low resistance to alkalis, respectively. The titanophosphate nanosheet sols prepared at TPAOH/Ti4+ ratios of 2.2 (pH 9) and 2.5 (pH 11) were placed on the glass substrates, and evaporated under ambient conditions. The XRDs of the resulting thin films are shown in Fig. 8. The thin film formed from nanosheet sols with pH ∼11 exhibited very sharp diffraction peaks of layered titanophosphate (resulting from the stacked titanophosphate nanosheets) on Eagle glass, but weak, broad, and split peaks on the borosilicate glass substrate. The peak degradation was likely attributable to corrosion of the substrate and consequent contamination by alkaline species. Conversely, the films formed from the nanosheet sols with pH ∼ 9 exhibited very sharp XRD peaks on both the Eagle glass and borosilicate glass substrates. Thus, titanophosphate nanosheet sols prepared by the bottom-up process in aqueous solution yielded layered titanophosphate thin films even on low-alkali resistant glass.
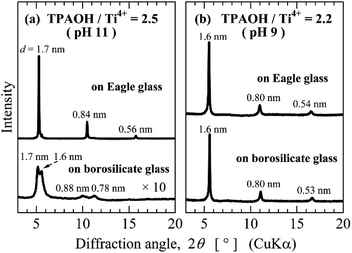 |
| Fig. 8 XRD patterns of the thin films fabricated by evaporating the titanophosphate nanosheet sols, which were prepared at a molar ratio of TIP : H3PO4 : TPAOH = 1 : 1 : (a) 2.5 and (b) 2.2, on Eagle glass and borosilicate glass substrates. | |
Removal of Cu2+ ion from neutral aqueous solutions by the titanophosphate nanosheets
Owing to their flocculation and ion-exchange behaviours, metalate nanosheets and layered metalates are effective removers of metal ions from aqueous solutions. To examine the removal of metal ions from neutral aqueous solution, we employed the titanophosphate nanosheets obtained in very weakly basic aqueous sols. The titanophosphate nanosheet sols prepared at TPAOH/Ti4+ = 2.2 (pH 9) were evaporated to form a layered titanophosphate powder. When this powder was added to aqueous solutions of copper acetate (Cu2+ = 0.01 mol L−1), precipitates were formed and the colour of the Cu(OCOCH3)2 aqueous solution changed from bluish to colourless (Fig. 9a). After adding the nanosheet powder, the absorption around 800 nm vanished from the UV-vis spectrum (Fig. 9a). The XRD measurements showed that the precipitates were amorphous (Fig. 9b). When a mixture of amorphous phase and titanophosphate nanosheets was added to Cu(OCOCH3)2 aqueous solution, the formed precipitates included anatase-type TiO2 (Fig. S6 in the ESI†). As the titanophosphate nanosheets prepared at TPAOH/Ti4+ = 2.2 (pH 9) did not form anatase precipitates, the amorphization of titanophosphate nanosheets did not occur, and Cu2+ was probably removed by the flocculation of titanophosphate nanosheets.
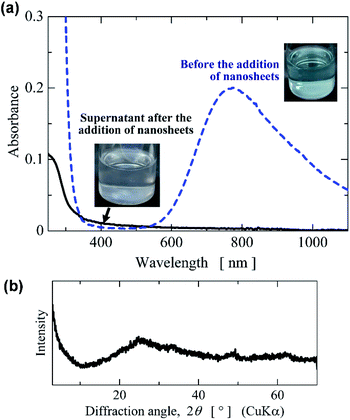 |
| Fig. 9 (a) Appearance and UV-vis spectra of copper acetate aqueous solutions before and after the addition of titanophosphate nanosheets. (b) XRD pattern of the precipitates obtained by adding titanophosphate nanosheets to copper acetate aqueous solution. | |
Conclusions
Previously, we synthesized metalate nanosheets by a bottom-up aqueous solution process. However, in many cases, the nanosheet synthesis required strongly basic aqueous sols. In thin film fabrication by solution processes, strongly basic solutions are undesirable because they corrode the substrates. In this study, highly water-dispersible titanophosphate nanosheets were synthesized by the bottom-up process in very weakly basic aqueous sols. With controlled acid–base reactions among the NR4OH, Ti4+ species and H3PO4 in the one-pot synthesis, an aqueous mixtures of TIP, H3PO4, and NR4OH yielded titanophosphate nanosheets at high yield. Specifically, the alkyl chain length and hydration state of NR4+ influenced the reaction between NR4OH and the Ti species. Moreover, the resulting titanophosphate nanosheets had the same crystal structure as layered titanium phosphate Ti2O3(H2PO4)2·2H2O. The nanosheets were flake-like with a lateral size of 5–50 nm. Furthermore, as the titanophosphate nanosheets were synthesized in very weakly basic sols, the resulting aqueous sols can be coated as nanosheets on glass substrates with low alkali resistance. In flocculated form, the nanosheets can also sequester Cu2+ ions from neutral aqueous solutions.
The results of this study will guide the synthesis of other metallophosphate nanosheets, and can expand the applications of nanosheets synthesized by bottom-up processes.
Conflicts of interest
There are no conflicts to declare.
Acknowledgements
This study was supported by KAKENHI (Grant-in-Aid for Scientific Research 17K06014) from Japan Society for the Promotion of Science (JSPS).
Notes and references
- M. Osada and T. Sasaki, J. Mater. Chem., 2009, 19, 2503–2511 RSC.
- J. L. Gunjakar, I. Y. Kim, J. M. Lee, Y. K. Jo and S.-J. Hwang, J. Phys. Chem. C, 2014, 118, 3847–3863 CrossRef CAS.
- M. Osada, Y. Ebina, H. Funakubo, S. Yokoyama, T. Kiguchi, K. Takada and T. Sasaki, Adv. Mater., 2006, 18, 1023–1027 CrossRef CAS.
- S. Sekizaki, M. Osada and K. Nagashio, Nanoscale, 2017, 9, 6471–6477 RSC.
- T. Nakato and N. Miyamoto, Materials, 2009, 2, 1734–1761 CrossRef CAS.
- N. Miyamoto and T. Nakato, Isr. J. Chem., 2012, 52, 881–894 CrossRef CAS.
- T. Nakato, Y. Higashi, W. Ishitobi, T. Nagashita, M. Tominaga, Y. Suzuki, T. Iwai and J. Kawamata, Langmuir, 2019, 35, 5568–5573 CrossRef CAS PubMed.
- T. Sasaki, M. Watanabe, H. Hashizume, H. Yamada and H. Nakagawa, J. Am. Chem. Soc., 1996, 118, 8329–8335 CrossRef CAS.
- T. Sasaki, M. Watanabe, H. Hashizume, H. Yamada and H. Nakagawa, Chem. Commun., 1996, 229–230 RSC.
- M. Osada and T. Sasaki, Adv. Mater., 2012, 24, 210–228 CrossRef CAS PubMed.
- R. Ma and T. Sasaki, Adv. Mater., 2010, 22, 5082–5104 CrossRef CAS PubMed.
- T. Ohya, A. Nakayama, T. Ban, Y. Ohya and Y. Takahashi, Chem. Mater., 2002, 14, 3082–3089 CrossRef CAS.
- T. Ban, S. Yoshikawa and Y. Ohya, J. Colloid Interface Sci., 2011, 364, 85–91 CrossRef CAS.
- T. Ban, S. Yoshikawa and Y. Ohya, CrystEngComm, 2012, 14, 7709–7714 RSC.
- T. Ban, T. Ito and Y. Ohya, Inorg. Chem., 2013, 52, 10520–10524 CrossRef CAS PubMed.
- T. Ban, S. Iriyama and Y. Ohya, Adv. Powder Technol., 2018, 29, 537–542 CrossRef CAS.
- T. Ban, T. Wakita, R. Yokoyama, T. Miyake and Y. Ohya, CrystEngComm, 2018, 20, 3559–3568 RSC.
- T. Ban, T. Kaiden and Y. Ohya, Cryst. Growth Des., 2019, 19, 6903–6910 CrossRef CAS.
- T. Ban, T. Ito and Y. Ohya, J. Sol-Gel Sci. Technol., 2013, 68, 88–94 CrossRef CAS.
- T. Ban, T. Nakagawa and Y. Ohya, Cryst. Growth Des., 2015, 15, 1801–1807 CrossRef CAS.
- H. Li, Y. Sun, Z.-Y. Yuan, Y.-P. Zhu and T.-Y. Ma, Angew. Chem., Int. Ed., 2018, 57, 3222–3227 CrossRef CAS PubMed.
- Y. Zhu, G. Hasegawa, K. Kanamori and K. Nakanishi, Inorg. Chem. Front., 2018, 5, 1397–1404 RSC.
- A. Bhaumik and S. Inagaki, J. Am. Chem. Soc., 2001, 123, 691–696 CrossRef CAS PubMed.
- Z. Yin, Y. Sakamoto, J. Yu, S. Sun, O. Terasaki and R. Xu, J. Am. Chem. Soc., 2004, 126, 8882–8883 CrossRef CAS PubMed.
- J. Peng, L.-N. Feng, Z.-J. Ren, L.-P. Jiang and J.-J. Zhu, Small, 2011, 7, 2921–2928 CrossRef CAS PubMed.
- A. N. Christensen, E. K. Anderson, I. G. Anderson, G. Albrti, M. Nielsen and M. S. Lehmann, Acta Chem. Scand., 1990, 44, 865–872 CrossRef CAS.
- A. I. Bortun, S. A. Khainakov, L. N. Bortun, D. M. Poojary, J. Rodriguez, J. R. Garcia and A. Clearfield, Chem. Mater., 1997, 9, 1805–1811 CrossRef CAS.
- D. M. Poojary, A. I. Bortun, L. N. Bortun and A. Clearfield, J. Solid State Chem., 1997, 132, 213–223 CrossRef CAS.
- M. Yada, Y. Inoue, A. Sakamoto, T. Torikai and T. Watari, ACS Appl. Mater. Interfaces, 2014, 6, 7695–7704 CrossRef CAS PubMed.
- M. Yada, Y. Inoue, T. Morita, S. Imamura, T. Torikai and T. Watari, Thin Solid Films, 2017, 628, 184–189 CrossRef CAS.
- L. Kőrösi, S. Papp and I. Dékány, Chem. Mater., 2010, 22, 4356–4363 CrossRef.
- W. Zhang, S. Hietala, L. Khriachtchev, T. Hatanpää, B. Doshi and R. Koivula, ACS Appl. Mater. Interfaces, 2018, 10, 22083–22093 CrossRef CAS PubMed.
- C. Chen, Y.-L. Yang, K.-L. Huang, Z.-H. Sun, W. Wang, Z. Yi, Y.-L. Liu and W.-Q. Pang, Polyhedron, 2004, 23, 3033–3042 CrossRef CAS.
- O. A. Khainakova, A. Espina, C. Trobajo, S. A. Khainakov, J. R. Garcia and A. I. Bortan, Stud. Surf. Sci. Catal., 2002, 144, 701–708 CrossRef CAS.
- A. Hayashi, H. Nakayama and M. Tsuhako, Bull. Chem. Soc. Jpn., 2002, 75, 1991–1996 CrossRef CAS.
Footnote |
† Electronic supplementary information (ESI) available: XRD patterns of the layered titanium phosphates synthesized at different TIP/H3PO4 molar ratios, XRD patterns and Raman spectra of the thin films formed from titanophosphate nanosheet sols with different NR4OH, XRD patterns of powders prepared from different titanophosphate nanosheet sols, TEM image of different titanophosphate nanosheets, and XRD pattern of the precipitates obtained by adding a mixture of amorphous phase and titanophosphate nanosheets to aqueous solution of copper acetate. See DOI: 10.1039/d0na00376j |
|
This journal is © The Royal Society of Chemistry 2020 |
Click here to see how this site uses Cookies. View our privacy policy here.