DOI:
10.1039/C9RA05895H
(Paper)
RSC Adv., 2020,
10, 5371-5384
LC-MS analysis of Myrica rubra extract and its hypotensive effects via the inhibition of GLUT 1 and activation of the NO/Akt/eNOS signaling pathway†
Received
30th July 2019
, Accepted 5th January 2020
First published on 4th February 2020
Abstract
In the area of medicine food homology, Myrica rubra ((Lour.) Siebold & Zucc.) has been used in medicine as an astringent and anti-diarrheal. However, there are few in-depth studies evaluating the antihypertensive chemical components and antihypertensive mechanisms of Myrica rubra. Thus, the aim in this study was to assess the protective effects of an ethanol extract of bayberry (BE) on spontaneous hypertension in rats. In this study, liquid chromatography-mass spectroscopy (LC-MS) coupled with biochemical assays and western blot have been employed to study the protective effects of BE against hypertension. A total of 28 compounds were identified in BE. According to this study, treatment with BE (2 g kg−1) resulted in the potent and persistent reduction of high blood pressure, even after drug withdrawal. The results indicate that the mechanisms of action might involve protection against damage to the vascular structure. Bayberry extract could enhance the endothelium-independent vascular function, inhibiting the abnormal proliferation of smooth muscle by inhibition of glucose transporter-1 (GLUT 1) and regulation of nitric oxide (NO)/serine/threonine kinases (Akt)/endothelial nitric oxide synthase (eNOS). The results of molecular docking and in vitro research indicated six compounds in BE that might be responsible for the antihypertensive effect attributed to GLUT 1, eNOS and Akt, and further in vivo studies are needed to verify this.
1. Introduction
Hypertension is a common condition that causes cardiovascular disease and related complications, such as stroke, heart attack and heart failure. It is often accompanied by metabolic disorders, especially dyslipidemia.1 Every year, approximately 17 million people worldwide die from cardiovascular disease, accounting for nearly one-third of all deaths. Of these, 9.4 million deaths are caused by complications from hypertension. In 2008, global statistics showed that 40% of adults aged 25 and over were diagnosed with high blood pressure.2,3 According to recent health reports, hypertension is considered to be a worldwide health concern that will affect 1.56 billion people by 2025.4
The pathogenesis of hypertension is complex, and most forms of hypertension lead to increased vasomotor tension, resulting in an increase in total peripheral resistance.5 Nitric oxide (NO) synthesized from L-arginine by nitric oxide synthase (NOS) plays a key role in regulating blood flow, blood pressure and oxygen delivery.6 Endothelial NOS (eNOS) is a key rate-limiting enzyme in NO formation. The expression and biological activity of eNOS directly affects the formation of NO.7 NOS contains neuronal NOS (nNOS), inducible NOS (iNOS) and eNOS.8 Angiotensin II (Ang II) is a peptide hormone of the renin–angiotensin–aldosterone system and causes pleiotropic effects on the vasculature. As an effective vasoconstrictor, it acts by the Ang II receptor 1, which is mainly expressed on resistance vessels, and thus Ang II increases peripheral resistance to enhance blood pressure. Thus, increase in Ang II signaling is considered to be a strong stimulus for arterial aging, and Ang II affects the structural, molecular, functional, and biomechanical properties of the arterial wall.9–11
Blood pressure (BP) is controlled by the renin–angiotensin system, in which the renin-derived, renin-catalyzed rate of angiotensinogen conversion to angiotensin I (AT-I) is limited by an inactive decapeptide. Angiotensin-converting enzyme (ACE) plays a crucial role in the regulation of blood pressure, and catalyzes the cleavage of the carboxy-terminal His–Leu dipeptide of AT-1 into angiotensin II, which is an effective octapeptide vasopressor.12 Recently, studies have shown that inflammation plays a crucial role in the development of essential hypertension.13,14 Tumor necrosis factor-α (TNF-α) stimulates the production of cytokines, enhances the expression of adhesion molecules and increases the activation of neutrophils. In 2008, Navarro-González et al.15 found that serum TNF-α levels were higher in hypertensive patients than in healthy controls.
Among the available antihypertensive drugs, diuretics, ACE inhibitors, calcium-channel blockers and also alpha- and beta-blocker drugs are the first choice of treatment for mild to moderate hypertension. Like most synthetic drugs they have long-lasting adverse effects, including cough, high blood sugar, weakness/fatigue, rash, kidney damage and so on.16 Considering increasing awareness of high blood pressure, healthier lifestyles are being introduced to the public, such as eating a balanced diet, reducing salt and alcohol intake, and reducing tobacco use. It is easy to achieve the desired state of health by controlling the type of food people consume. Under these circumstances, the functional food market, which was once a niche market, is booming. The demand for healthier food has prompted food scientists to try to develop a food system that can treat hypertension, beyond the basic nutrients of carbohydrates, protein and fat.
Historically, traditional herbal medicines have been the treatment strategy for Chinese medical systems to treat a variety of diseases. However, Myrica rubra is rarely used to treat high blood pressure, and few studies have reported its mechanism of action for blood pressure. The Chinese bayberry,17 which is used in medicine and food, is a plant of the genus Myrica distributed in East Asia. It has been planted in southern China for more than 2000 years. The leaves, bark and fruit of the tree have astringent effects, antihypertensive effects, detoxification effects and anti-diarrheal effects in Chinese medicine.18,19 In addition, bayberry extracts show other biological activities such as liver protection,20 antioxidant activity,21 and antiviral effects.22 The main groups of secondary metabolites are flavonoids, tannins,23 triterpenoids24 and diarylheptanoid acid.25
The aim of this study was to assess the protective effect of BE on spontaneous hypertension in rats (SHR) and its potential mechanism of action based on a novel strategy that incorporates LC-MS, western blotting and molecular docking. As far as we know, this is the first in-depth study to evaluate the antihypertensive chemical components and antihypertensive mechanisms of BE.
2. Materials and methods
2.1 Chemicals and reagents
Ethanol (EtOH) (95%) was purchased from Tianjin University Chemical experimental factory (Tianjin, China). Chromatographic grade acetonitrile and methanol were obtained from ThermoFisher (USA). Sodium 3-trimethylsilyl [2,2,3,3-d4] propionate (TSP) was acquired from Cambridge Isotope Laboratories Inc. (Andover, MA, USA). Deuterated water (D2O) was purchased from Norell (Landisville, NJ, USA). Nω-Nitro-L-arginine methyl ester (L-NAME, >99%), analytical grade Na2HPO4·2H2O and NaH2PO4·12H2O were obtained, respectively, from Guangfu Fine Chemical Research Institute (Tianjin, China) and Zhiyuan Chemical Reagent Co., Ltd (Tianjin, China). Phosphate buffer was prepared with Na2HPO4·2H2O and NaH2PO4·12H2O (0.1 M, pH 7.4), containing 10% D2O and 0.1 mM L−1 of TSP. All other reagents and chemicals were of analytical grade.
Anti-eNOS, anti-Akt, anti-eNOS, and anti-eAkt were the products from Boster (Wuhan, China), whereas fluorescein isothiocyanate (FITC)-conjugated goat anti-rabbit IgG was purchased from Beyotime Biotechnology (Jiangsu, China). Antibodies of eNOS-Ser1177 and Akt-Ser473 were purchased from Cell Signaling Technology (Boston, USA). The anti-rabbit IgG conjugated to horseradish peroxide was purchased from Santa Cruz Biotechnology (Texas, USA). Enzyme-linked immunosorbent assay (ELISA) kits were manufactured by Nanjing Jiancheng Biotechnology (Nanjing, China).
The bayberry was bought from a local drugstore and identified as Myrica rubra (Sieb. & Zucc.), which was deposited in Wuhan University. The survey was initiated by Prof. Hong Ding. Human umbilical vein endothelial cells (HUVECs) were purchased from the China Centre for Type Culture Collection.
2.2 Extract preparation
The bayberry was extracted three times with 95% EtOH (85 °C, 2 h each) reflux, and the combined extract was concentrated under reduced pressure for further chemical analysis and animal experiments.
2.3 LC-MS analysis of BE
The chemical composition of the BE was identified by LC-MS. BE was analyzed on the UHPLC Q Exactive Orbitrap MS equipment (ThermoFisher, USA). A ThermoFisher U3000 system was installed with a Waters BEH C18 column (2.1 mm × 100 mm, 1.7 μm) with an electrospray ionization ion source. The Z-spray ionization source was maintained at 300 °C and the spray voltage was kept at 3.5 kV in positive ionization mode. Additional operating parameters were capillary temperature: 320 °C, lens voltage: 55 kPa, mass resolution: 70
000, and mass scan range: 100–1500 m/z in full-scan mode.
Separation of biological samples was carried out at a flow rate of 0.2 mL min−1 and a column temperature of 30 °C, with an injection volume of 5 μL and a detection wavelength of 210 nm. The mobile phase was composed of (A) 0.1% formic acid aqueous solution and (B) acetonitrile at a flow rate of 0.2 mL min−1, and the gradient elution was optimized as follows: 0–5 min, 5–15% B; 5–10 min, 15–20% B; 10–15 min, 25–35% B; 15–20 min, 35–40% B; 40–60% B; 24–24.5 min, 60–5% B; 24.5–27 min, 5% B.
2.4 Animal experiments
Eight-week-old male SHR and SD rats (180–220 g) were purchased from Beijing Vital River Laboratories Co., Ltd. (SCXK (Jing) 2016-0006, Beijing, China). After 1 week of domestication, eight male SD rats were treated as the control group, and the normotensive group was given a basic diet. Forty-eight male SHR rats were randomly divided into the following six groups (n = 8): SHR, rats were given distilled water; SHR-nifedipine (Ni), rats were administered with 10 mg kg−1 nifedipine; SHR-low dose (LD), rats were administered BE at a dosage of 1 g kg−1; SHR-medium dose (MD), rats were administered with 2 g kg−1 BE; SHR-high dose (HD), rats were administered with 4 g kg−1 BE. All groups were given a free diet. The procedures employed for the treatment with BE are shown in Fig. 1. The rats were kept in well-ventilated rooms, at temperatures of 25 ± 2 °C, in cycles of light and dark of 12 hours, and with relative humidity between 60 and 80%. All experiments were conducted according to the guidelines of the University of Houston and protocols were approved by Institutional Animal Care and Use Committee (IACUC), Wuhan University Center for Animal Experiment, Wuhan, China (AUP no. S201411012I).
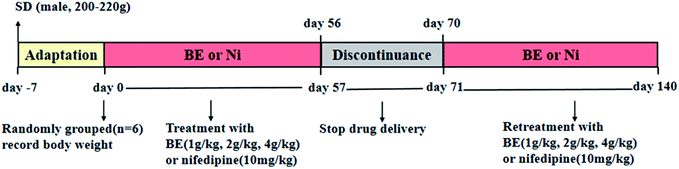 |
| Fig. 1 SHR rat treatment with nifedipine (Ni) and bayberry extract (BE). | |
2.5 Bodyweight
The bodyweight of every rat was recorded at 14:30 every two days throughout the whole experiment, so that we could supervise the body condition of each rat.
2.6 Blood pressure variability
A blood pressure analysis system (Tai Meng Scientific and Technologic Co., Ltd, Chengdu, China) was used to measure systolic blood pressure (SBP), diastolic blood pressure (DBP) and mean blood pressure (MBP) of awake rats every week, following establishment of the model. The final value was calculated from eight successive measurements.
2.7 Histology and morphological analyses of thoracic aorta and kidney
The thoracic aorta and kidney were fixed with 10% (v/v) neutral formalin for 48 hours. To enhance the fixation effect, the thoracic aorta and kidney were placed in rubber-covered ampoules and filled with 10% (v/v) neutral formalin to two-thirds of the maximum volume. The thoracic aorta were embedded in paraffin, sectioned at 4 μm, and stained with hematoxylin and eosin (H&E) and Verhoeff's Van Gieson stain (EVG) to observe the change. After administration, the effect on the kidney was observed by Masson's trichrome staining. Images were obtained and studied under light microscopy. Each part was magnified 100 times, and a random slide was taken from each set of eight animals.
2.8 Electron microscope scanning of thoracic aorta
A Philips XL30E scanning electron microscope (SEM) was used to detect the endothelial surface of various arteries, the images were scanned, and the degree of injury was observed.
2.9 Determination of biochemical index levels
After anesthesia, the femoral artery of rats was taken for blood supply, and serum was prepared by centrifugation at 13
000 rpm for 10 min. Serum was stored at −80 °C for further analysis. The levels of NO, Ang II, endothelin 1 (ET-1) and TNF-α were determined with the corresponding ELISA kits according to the manufacturer's instructions.
2.10 Immunohistological staining
To estimate the expression of GLUT 1 and eNOS proteins in the blood vessels, immunofluorescence and immunohistochemical staining were used, with reference to the literature.26 Images were randomly captured by using an inverted fluorescent microscope (Olympus, Japan) with a charge coupled device camera system at fixed exposure times for comparison. The statistical analyses of incorporation of GLUT 1 and eNOS in thoracic aortas were calculated using ImagePro Plus software (Bethesda, MD, USA).
2.11 Western blot analysis
The thoracic aorta specimens were homogenized in lysis buffer containing 0.1 mM phenylmethanesulfonyl as protease inhibitor,27 loaded with 120 μg protein on 10% SDS polyacrylamide gel and then transferred into polyvinylidene difluoride membranes. Then, the proteins were transferred to polyvinylidene fluoride membranes and incubated with 5% bovine serum albumin to block non-specific binding. The membranes were incubated at 4 °C overnight and probed with eNOS-Ser1177, Akt-Ser473 (dilution 1
:
1000), eNOS and Akt (dilution 1
:
200) as the target. After washing three times, the secondary antibody anti-rabbit IgG-conjugated horseradish peroxide (diluted 1
:
5000) was used to incubate the blots, the immunoreactivity was observed by chemiluminescence, and the relative expression was quantified.
2.12 Calculations and statistical analysis
Data are expressed as mean ± SEM, and n represents the number of animals. GraphPad Prism 5.0 software was used for statistical analysis. One-way analysis of variance (ANOVA) was used to compare the mean values of different groups, and SPSS 16.0 software was used for multiple comparisons. Two-tailed p < 0.05 was considered statistically significant (α = 0.05).
2.13 Cell proliferation assay
The compounds (1, 3, 4, 7, 8, 9, 11, 12, 19, 21, 23, and 27) were dissolved in dimethyl sulfoxide (DMSO) and, respectively, diluted to the required concentration (0.02, 0.2, 2, 20 mg mL−1) using a culture solution. During the logarithmic phase of adherence of HUVECs, pancreatin was added. After dissociation, the culture solution was added (10% DMEM of fetal bovine serum), and this cell suspension was 105 units per mL. Suspension (100 μL) was added into each well of a 96-well culture plate and cultured for 24 h. The HUVECs were divided into 13 groups and each group was treated with L-NAME (100 μmol L−1), L-NAME and compounds (1, 3, 4, 7, 8, 9, 11, 12, 19, 21, 23, 27) for 24 h after the culture solution was removed, and with the control group only using culture solution. The supernatant was discarded and 10 μL of fresh MTT solution (5 mg mL−1) was added into each well. After culturing for 4 h, the solution in each well was removed and mixed with 100 μL of DMSO. Optical density was measured at 570 nm using the enzyme-labeled instrument. Each concentration was repeated three times.
Cell survival rate (CSR) was measured by the MTT method, CSR% = (experiment group OD − blank group OD)/(control group OD − blank group OD) × 100%.
3. Results
3.1 Chemical analysis of BE
Compounds in the BE were identified by LC-MS analysis of mass-to-charge ratios and fragmentation (MS2) using data described in the literature and the GNPS database (Global Natural Products Social Molecular Networking). Negative-ion and positive-ion modes were used to identify the corresponding signals. A total of 28 compounds were identified, including 18 flavonoids28–35 (compounds 1–18), 8 organic acids (compounds 19–26) and 3 amino acid (compounds 27–29) (Fig. 2 and Table 1). The structures of all the identified compounds are shown in ESI Fig. S1.†
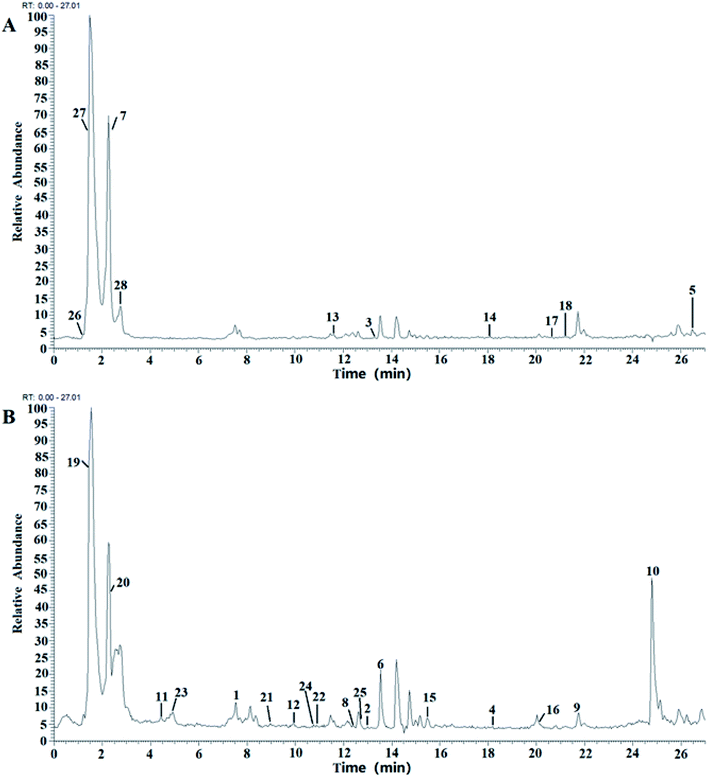 |
| Fig. 2 LC-MS chromatograms of BE, in (A) positive-ion mode and (B) negative-ion mode. | |
Table 1 Chemical constituents identified in the ethanol ether extract of Myrica rubra
No. |
t
R
|
Molecular formula |
Selected ion |
Experimental m/z |
Theoretical m/z |
Error (ppm) |
MS/MS fragmentation (m/z) |
Identification |
1 |
7.77 |
C20H18O11 |
[M − H]− |
433.0765 |
433.0765 |
0.000 |
301.2014, 273.0534, 244.8873 |
Quercetin-3-O-α-arabinopyranoside |
2 |
13.09 |
C21H20O12 |
[M − H]− |
463.0871 |
463.0878 |
−0.151 |
301.2014, 273.0534, 255.8373, 179.0340 |
Quercetin-3-O-galactoside |
3 |
13.14 |
C27H30O16 |
[M + H]+ |
611.1606 |
611.1608 |
−0.327 |
303.3091, 163.0031 |
Rutin |
4 |
18.25 |
C15H10O7 |
[M − H]− |
301.0342 |
301.0349 |
2.063 |
285.0393, 257.0444, 229.0495, 165.0182 |
Quercetin |
5 |
26.5 |
C30H40O20 |
[M + H]+ |
757.2185 |
757.2152 |
4.360 |
611.3409, 465.2311, 303.3091 |
Quercetin-3-O-2′,6′-dirhamnosylglucoside |
6 |
13.49 |
C21H20O12 |
[M − H]− |
463.0871 |
463.0878 |
−1.510 |
301.2014, 273.0534, 255.8373, 179.0340 |
Quercetin-7-O-β-D-glucoside |
7 |
2.23 |
C21H20O11 |
[M + H]+ |
449.1078 |
449.1061 |
3.790 |
287.0117 |
Kaempferol-3-O-β-D-glucopyranoside |
8 |
12.21 |
C21H18O13 |
[M − H]− |
477.0663 |
477.0652 |
2.310 |
301.2014, 179.0340 |
Kaempferol-3-β-glucuronide |
9 |
21.87 |
C27H30O15 |
[M − H]− |
593.1500 |
593.1500 |
0.000 |
477.2924, 285.0925 |
Kaempferol-3-O-rutinoside |
10 |
24.59 |
C27H30O15 |
[M − H]− |
593.1500 |
593.1499 |
−0.170 |
285.0925 |
Kaempferol-3-O-D-glucopyranosyl-(1–2)-L-rhamnoside |
11 |
4.24 |
C15H12O8 |
[M − H]− |
319.0462 |
319.0456 |
4.354 |
150.9455, 180.9722 |
Dihydromyricetin |
12 |
9.96 |
C15H10O8 |
[M − H]− |
317.0301 |
317.0301 |
0.000 |
271.0694, 151.0343 |
Myricetin |
13 |
11.49 |
C21H22O12 |
[M + H]+ |
467.1184 |
467.1170 |
3.000 |
303.3091, 229.0321 |
Morin-3-O-galactoside |
14 |
18.15 |
C15H10O7 |
[M + H]+ |
303.0499 |
303.5000 |
−0.392 |
229.0321 |
Morin |
15 |
15.43 |
C16H12O7 |
[M − H]− |
315.0499 |
315.0504 |
−0.159 |
300.9910, 272.0534, 163.0390 |
Isorhamnetin |
16 |
20.4 |
C15H12O5 |
[M − H]− |
271.0600 |
271.0605 |
−0.174 |
187.8600, 165.0546 |
Naringenin |
17 |
20.7 |
C17H14O7 |
[M + H]+ |
331.0812 |
331.0815 |
−0.910 |
253.0974 |
Cirsiliol |
18 |
21.13 |
C15H10O6 |
[M + H]+ |
287.0550 |
287.0551 |
−0.350 |
139.0181 |
Luteolin |
19 |
1.57 |
C4H6O5 |
[M − H]− |
133.0134 |
133.0131 |
2.200 |
115.0025, 89.0232, 71.0127 |
DL-Malic acid |
20 |
2.38 |
C6H8O7 |
[M − H]− |
191.0192 |
191.0186 |
0.895 |
87.0076, 85.0283, 111.0076, 173.0081 |
Citric acid |
21 |
8.9 |
C7H6O3 |
[M − H]− |
137.0493 |
137.0233 |
2.600 |
93.0334, 65.0384 |
Salicylic acid |
22 |
10.99 |
C7H6O4 |
[M − H]− |
153.0186 |
153.0182 |
0.424 |
67.0178, 109.0283, 91.0178, 135.0077 |
2,4-Dihydroxybenzoic acid |
23 |
4.07 |
C7H6O2 |
[M − H]− |
169.0131 |
169.0135 |
−2.370 |
153.0182, 125.0961 |
Gallic acid |
24 |
10.78 |
C9H8O4 |
[M − H]− |
179.0338 |
179.0340 |
0.362 |
135.0441, 107.0489, 97.0281 |
Caffeic acid |
25 |
13.16 |
C10H10O4 |
[M − H]− |
193.0502 |
193.0495 |
1.216 |
149.0597, 134.0362, 90.9322, 61.9872 |
Ferulic acid |
26 |
1.33 |
C6H14N4O2 |
[M + H]+ |
175.1193 |
175.1189 |
2.043 |
158.0927, 130.0977, 116.071 |
DL-Arginine |
27 |
1.52 |
C5H7NO3 |
[M + H]+ |
130.0501 |
130.0502 |
2.540 |
84.0450, 70.0658 |
L-Pyroglutamic acid |
28 |
2.68 |
C9H11NO2 |
[M + H]+ |
166.0862 |
166.0862 |
0.200 |
120.0812, 103.0546 |
L-Phenylalanine |
3.1.1 Quercetin derivatives.
Quercetin generated the [M + H]+ ion at m/z 303.0500 in the positive-ion mode, and the [M − H]− ion at m/z 301.0349 in the negative-ion mode. In the MS2 spectrum, it yielded m/z 285.0393 and 257.0444 by neutral loss of H2O and H2O + CO, and further yielded m/z 229.0495 and 165.0182 owing to retro Diels–Alder (RDA) rearrangement in the positive-ion mode.36 Compounds 1–5 were identified as quercetin glycosides, which showed fragments common to those of quercetin and also gave specific fragmentation patterns corresponding to the loss of different substituents for each compound. For example, quercetin-3-O-2′,6′-dirhamnosylglucoside (5) showed the pseudomolecular ions of m/z 757.2152 [M + H]+. The MS2 of m/z 757.2152 produced m/z 611.3409 [M + H-rha]+, 465.2311 [M + H-2rha]+, 303.3091 [M + H-2rha-glu]+, 285.0760 and 257.0999 (Fig. S2†).
3.1.2 Kaempferol derivatives.
Kaempferol is the dihydroxylation derivative of quercetin at the B ring of C3. It showed the pseudomolecular ion at m/z 287.0549, which was 16 Da less than that of quercetin. It produced a product ion at m/z 269.0453 [M + H–H2O]+ in the MS2 spectrum, and the ions at m/z 241.0496, 213.0549 and 165.0181 were related to the RDA fragmentation pathway.36 A total of four kaempferol glycosides (compounds 7–10) were identified. Compounds 9 and 10, which were eluted at a retention time (tR) of 21.87 and 24.59 min, respectively, showed the dominant protonated ion at m/z 593.1499 [M − H]−. The MS2 spectrum of compound 9 presented fragment ions at m/z 447.2747 [M − H-rha]−, 285.0925 [M − H-rha-glu]−, 211.0119 and 163.0390, while compound 10 showed fragment ions at m/z 431.1392 [M − H-glu]− and 285.0925 [M − H-glu-rha]−. The different fragmentation patterns above suggested that compounds 9 and 10 were kaempferol-3-O-rutinoside and kaempferol-3-O-D-glucopyranosyl-(1–2)-L-rhamnoside, respectively.
3.1.3 Myricetin derivatives.
Myricetin showed pseudomolecular ions at m/z 317.0301 [M − H]−. The MS2 spectrum showed product ions at m/z 271.0694 [M − H–H2O–CO]− and 151.0343 [M − H–C8H6O4]−. Based on the observed common fragmentation pattern above, two myricetin derivatives (11 and 12) were also detected.
In addition, six flavonols with another aglycone, including isorhamnetin, two anthocyanidins (morin and morin-3-O-galactoside), cirsiliol, luteolin and naringenin were also identified, and the fragmentation pathways of these compounds were similar.
3.1.4 Organic acids and amino acid.
The eight common organic acids or phenolics (compounds 19–25) and three amino acids (26–28) were also identified in BE. Diagnostic mass fragments obtained in the negative mode at 133.0134, 191.0192, 137.0493, 153.0186, 169.0131, 179.0338 and 193.0502 characterized the aglycones as DL-malic acid, citric acid, salicylic acid (and 93.0334, 65.0384), 2,4-dihydroxybenzoic acid, gallic acid, caffeic acid, and ferulic acid, respectively. Furthermore, DL-arginine, L-pyroglutamic acid and L-phenylalanine were also identified in BE.
It is clear that flavonoids are the main components of BE.
3.2 Effect of BE on animal weight and blood pressure
As shown in Fig. 3 (top left), the bodyweight of each rat was recorded. In the control group, the body weight of the rats increased by 25% in 140 days. Administration of BE prevented SHR-induced loss of body weight, maintaining a near-normal weight, but Ni administration failed to do this.
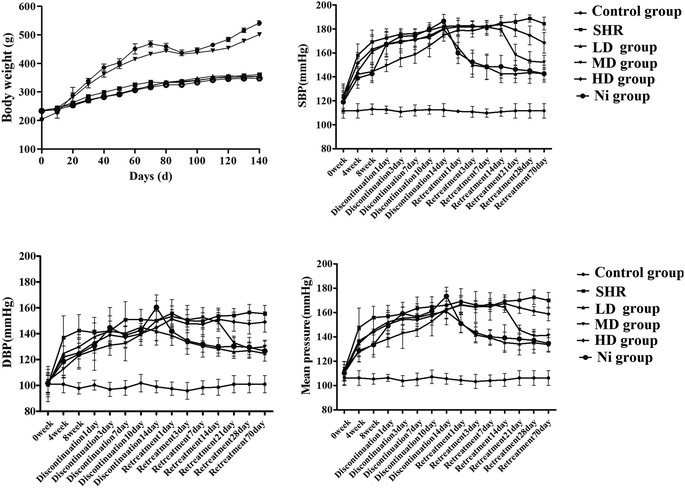 |
| Fig. 3 Body weight and blood pressure from SHR rats treated with nifedipine (Ni) and bayberry extract (BE). (Top left) Body weight gain; (top right) systolic blood pressure, SBP; (bottom left) diastolic blood pressure, DBP; (bottom right) mean blood pressure, MBP. | |
Administration of nifedipine (10 mg kg−1) resulted in a gradual decrease in blood pressure (BP) in the first week (DBP 12.8%, SBP 15.8%, MBP 13.3% versus the model group at the end of eight weeks, p < 0.01). At the same time, after treatment with BE with a series of test concentrations, as shown in Fig. 3, those with increased blood pressure showed a significant reduction in blood pressure compared with the SHR group, until week 8 (p < 0.01). Then, treatment was discontinued for two weeks to determine the continuous curative effect. Surprisingly, a significant increase in blood pressure was observed in the Ni group compared with the control group, while the LD group (1 g kg−1) and the MD group (2 g kg−1) showed persistent modification of the pressure flex functions, accompanied by decreases in BP (Fig. 3). The blood pressure decreased rapidly after one day of retreatment with Ni, and its regulation was similar to that of the MD group (p < 0.05). However, blood pressure in the LD and HD groups was reduced until day 21 of retreatment, and blood pressure was higher than in the MD and Ni groups.
When the rats were given Ni at the same time, the negative effects were weakened in the early stage of blood pressure elevation (Fig. 3). Nevertheless, the increase in blood pressure during withdrawal illustrates that Ni has no significant effect. Compared with Ni, the effect of BE progresses slowly during treatment, but it can effectively reduce the SBP of SHR after stopping the drug (Fig. 3). A recent study37 has shown rapid loss of vascular protection after discontinuation of Ni, with the result that systolic blood pressure returns to an abnormal value of SHR within 24 h. Our study indicates that BE was superior to endothelium-dependent vasodilation in Ni.
3.3 Pathological examination
The effects of BE on the cross-sectional area of the thoracic aorta in SHR rats was observed by H&E and EVG staining. The results of H&E staining showed that the endothelia of thoracic aorta were intact and smooth compared with SHR. The results for EVG staining indicated that the elastin wavy structure of the model group was disordered and the intima-media thickness was thickened compared with the control. After treatment with BE or Ni, the changes in SHR rats could be significantly attenuated, especially for the MD group (Fig. 4A).
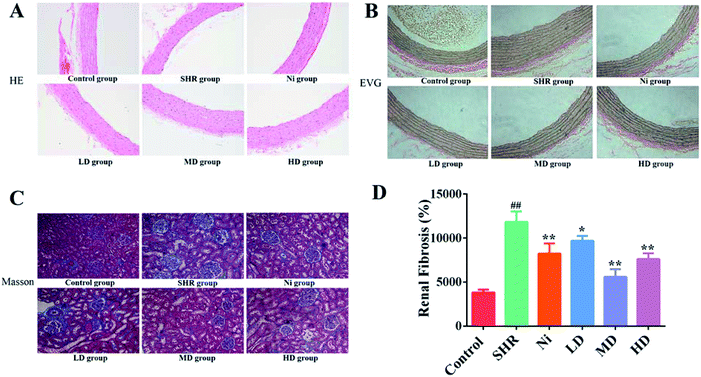 |
| Fig. 4 Histopathological photomicrographs of thoracic aorta: (A) H&E staining and (B) EVG staining. (C) Masson's trichrome staining and (D) the calculation of renal fibrosis area. Ni, nifedipine group (10 mg kg−1); LD, low dose group (1 g kg−1); MD, middle dose group (2 g kg−1); HD, high dose group (4 g kg−1). Values are expressed as mean ± SEM (n = 6 rats). ##p < 0.01 compared to normal group; *p < 0.05; **p < 0.01 compared to no treatment. | |
The elastic tissue of the rat aortic wall can be visually stained using EVG staining.38 Decreased relative elastin content in the aorta may lead to elevated blood pressure in spontaneously hypertensive rats.39 It was reported that40 vacuolar endothelium and incomplete circulating endothelial cells in the aorta of hypertensive rats destroyed the tissue structure. These results indicate that BE could restore the aortic wall of rats to maintain continued decrease in blood pressure (Fig. 4B).
There is much evidence that hypertension is a critical risk factor for chronic kidney disease and end-stage renal disease, which can lead to progression of renal fibrosis and renal failure.41,42 Renal fibrosis is a key indicator for evaluating the effect of blood pressure. In order to find out whether there was a change in renal fibrosis after treatment administration, we performed a Masson's trichrome staining of kidney (Fig. 4C). The renal interstitial collagen deposition in the SHR group was significantly greater (p < 0.01) than in the control group. The degree of renal interstitial collagen deposition was reduced in the drug-administered groups compared with the model group (p < 0.05).
3.4 Electron microscope scanning of thoracic aorta
Blood vessel endothelium could be clearly recognized by SEM. As shown in Fig. 5, well arranged and distributed blood vessels were observed in the control group. However, blood vessel endothelium disorders and damage could be found in the SHR group. This effect was attenuated after treatment with BE in the LD and MD dose groups. In particular, the regulatory effect in the MD group was almost the same as that of the control group.
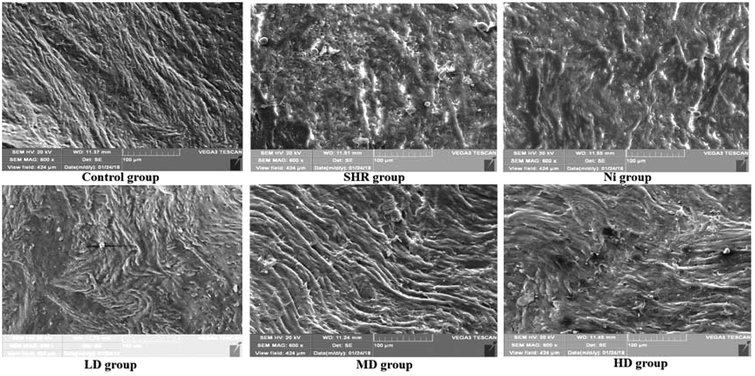 |
| Fig. 5 SEM images of rat thoracic aorta: LD, low dose group (1 g kg−1); MD, middle dose group (2 g kg−1); HD, high dose group (4 g kg−1). | |
3.5 Determination of biochemical markers
Several cytokines have been found to be closely related to hypertension, such as Ang II, ET-1 and TNF-α. Therefore, changes in these mediators in the model and treatment groups were tested by ELISA. The concentration of Ang II and ET-1 in the SHR group increased significantly (p < 0.01) (Fig. 6). The levels of Ang II and ET-1 were attenuated (p < 0.05) after treatment with BE at all concentrations tested. In addition, the level of TNF-α was decreased by treatment with BE (Fig. 6, bottom right). For these cytokines, the best regulatory effect was for the MD dose group and was similar to normal controls, and better than for Ni treatment.
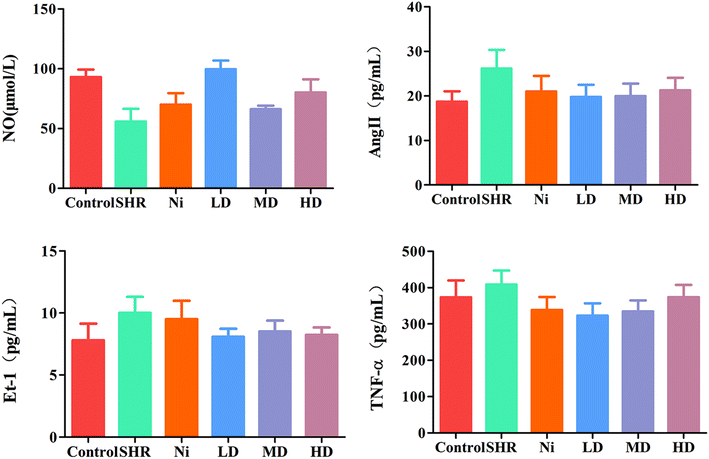 |
| Fig. 6 Quantification of cytokines of NO, Ang II, Et-1 and TNF-α in serum as determined by ELISA. Results are representative of four individual experiments and expressed as mean ± SEM, with the significance accepted at ##p < 0.01 versus control (no drug intervention). *p < 0.05 and **p < 0.01 versus only BE intervention group. | |
Compared with the control group, the NO level in the SHR group was significantly decreased (p < 0.01), and it was significantly increased after treatment with LD or MD doses of BE. The MD group revealed a significant (p < 0.01) improvement, of about 78%, in the concentration of NO, and the curative effect was better than that of the Ni group (Fig. 6, top left).
3.6 Effects of BE on GLUT 1 and phosphorylation of Akt/eNOS pathway tissue
Most researchers consider that GLUT 1 is the main protein that promotes glucose transport across the plasma membranes of mammalian cells.43 Overexpression of GLUT 1 leads to thickening of the vascular endothelium. Biological evidence suggested that eNOS is associated with hypertension, because it is a crucial regulator of cardiovascular homeostasis. eNOS regulates the basic vasodilation function of blood pressure by vascular endothelial catalysis of NO synthesis. In addition, by regulating blood vessel diameter, eNOS is a key factor affecting cardiovascular health, and has an anti-proliferative effect and provides an anti-apoptotic environment for cardiovascular disease.44 Immunofluorescence analyses showed that the thoracic aortic level of the protein GLUT 1 was significantly elevated (p < 0.01) in SHR (Fig. 7A and D) compared with the control. Nevertheless, the expression of GLUT 1 in thoracic aorta of the BE group was significantly reduced. The GLUT 1 levels of the MD group showed a remarkable decrease of about 50%. On the other hand, eNOS did not increase in the SHR group, but sharply decreased (Fig. 7C and F) and the concentration of eNOS was elevated after administration with BE at all of the tested doses. It is worth noting that the levels of eNOS in the MD group were similar to those in the Ni group.
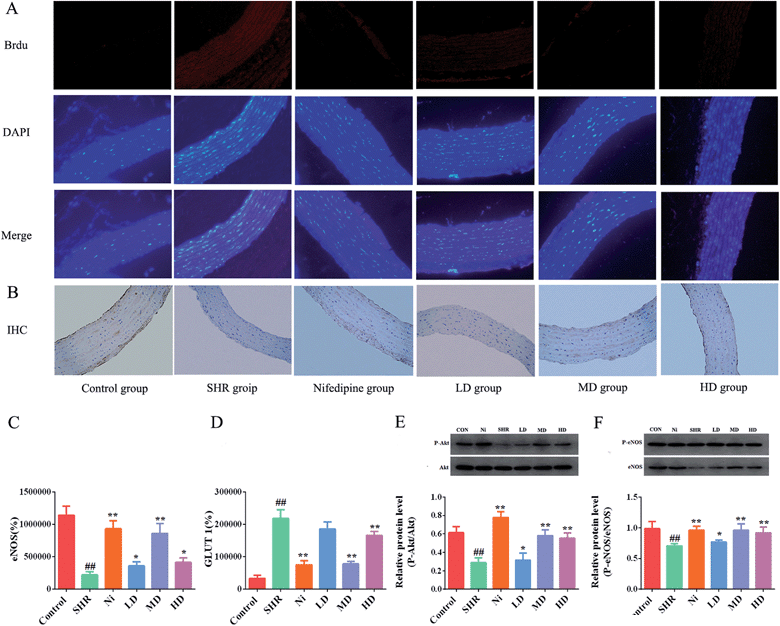 |
| Fig. 7 Analysis determining the expression of phosphorylation of GLUT 1, eNOS, and Akt in vascular endothelium. (A) Immunofluorescence. (B) Immunohistochemical analysis. (C) Optical density of GLUT 1 immunofluorescence. (D) Area of eNOS immunohistochemistry. The levels of phosphorylation eNOS and total eNOS proteins were quantified by densitometry. (E) The levels of phosphorylation Akt and total Akt proteins were quantified by densitometry (F). Each value represents mean ± SEM and the significance accepted at ## indicates p < 0.01, compared with the respective normal control group. * and ** indicate p < 0.05 and p < 0.01, respectively, compared with the SHR group. | |
In vivo, Akt/protein kinase B plays a crucial role in regulating phosphorylation of eNOS-Ser1177.45 Previous studies have shown that the signaling pathway can be activated in vitro,46 so we investigated whether the effects of BE on vasodilatation and vascular dysfunction were mediated through activation of eNOS-Ser1177 and Akt-Ser473 in the aorta. The Akt-Ser473 phosphorylation and total Akt expression in the aorta of the SHR group were reduced significantly compared with the normal controls (p < 0.01). As shown in Fig. 7E, after prophylactic administration with BE, the phosphorylation levels of Akt were increased (p < 0.05). In addition, the medium dose group showed the best regulation effect with respect to normal controls, and its regulation effect was similar to that of Ni. The eNOS levels were increased while Akt-Ser473 phosphorylation was upregulated in the meantime. Compared with the control group, the level of eNOS in the SHR group was significantly lower (p < 0.01) and increased after treatment with BE at all tested doses. The MD group showed an obvious (p < 0.05) increase of about 35.8% in eNOS level, and the adjustment effect was similar to that of the Ni group (Fig. 7F).
3.7 Molecular docking analysis
Molecular docking analysis was also performed in order to explore the active ingredients in BE that had efficacy in hypertension. With GLUT 1, eNOS and Akt as target proteins we found their structures in the RCSB Protein Data Bank (http://www.rcsb.org/pdb/) and, along with the structures of the 28 compounds in BE, these were imported into system dock (http://systemsdock.unit.oist.jp/). On the basis of molecular docking, active ingredients of BE with tight binding to the protein target, using a docking score of >4.52,47 were filtered out, and the scores are tabulated in Table 2. Twelve compounds identified in BE could be found to bind to GLUT 1, eNOS and Akt protein targets, indicating that these components were effective in treating hypertension by regulating GLUT 1, eNOS and Akt cytokines.
Table 2 The docking scores of 28 active ingredients and GLUT1, eNOS, Akt, in BE
Target gene |
PDB ID |
Compound |
Docking score |
Compound |
Docking score |
GLUT 1 |
4JA4
|
Quercetin-3-O-α-arabinopyranoside |
6.321 |
Isorhamnetin |
3.549 |
eNOS |
3EAH
|
5.431 |
4.219 |
AKT |
3QKK
|
5.763 |
3.489 |
GLUT 1 |
4JA4
|
Quercetin-3-O-galactoside |
5.212 |
Naringenin |
5.342 |
eNOS |
3EAH
|
4.211 |
5.216 |
AKT |
3QKK
|
3.872 |
3.496 |
GLUT 1 |
4JA4
|
Rutin |
7.651 |
Cirsilion |
3.459 |
eNOS |
3EAH
|
5.057 |
4.457 |
AKT |
3QKK
|
5.941 |
4.398 |
GLUT 1 |
4JA4
|
Quercetin |
5.972 |
Luteolin |
3.459 |
eNOS |
3EAH
|
6.258 |
4.457 |
AKT |
3QKK
|
4.987 |
4.398 |
GLUT 1 |
4JA4
|
Quercetin-3-O-2′,6′-dirhamnosylglucoside |
4.012 |
DL-Malic acid |
4.485 |
eNOS |
3EAH
|
3.521 |
5.296 |
AKT |
3QKK
|
4.621 |
5.431 |
GLUT 1 |
4JA4
|
Hyperin |
3.123 |
Citric acid |
3.156 |
eNOS |
3EAH
|
4.022 |
4.471 |
AKT |
3QKK
|
4.316 |
4.137 |
GLUT 1 |
4JA4
|
Kaempferol-3-O-β-D-glucopyranoside |
4.655 |
Salicylic acid |
7.954 |
eNOS |
3EAH
|
6.213 |
5.463 |
AKT |
3QKK
|
5.232 |
6.954 |
GLUT 1 |
4JA4
|
Kaempferol-3-β-glucuronide |
7.298 |
2,4-Dihydroxybenzoic acid |
5.098 |
eNOS |
3EAH
|
5.433 |
5.071 |
AKT |
3QKK
|
5.008 |
5.413 |
GLUT 1 |
4JA4
|
Kaempferol-3-O-rutinoside |
4.721 |
Gallic acid |
4.651 |
eNOS |
3EAH
|
5.071 |
4.072 |
AKT |
3QKK
|
4.921 |
4.987 |
GLUT 1 |
4JA4
|
Kaempferol-3-O-D-glucopyranosyl-(1–2)-L-rhamnoside |
4.442 |
Caffeic acid |
3.265 |
eNOS |
3EAH
|
3.143 |
4.594 |
AKT |
3QKK
|
4.321 |
6.841 |
GLUT 1 |
4JA4
|
Dihydromyricetin |
8.456 |
Ferulic acid |
3.891 |
eNOS |
3EAH
|
6.266 |
4.095 |
AKT |
3QKK
|
6.461 |
4.397 |
GLUT 1 |
4JA4
|
Myricetin |
7.951 |
DL-Arginine |
5.713 |
eNOS |
3EAH
|
6.335 |
5.342 |
AKT |
3QKK
|
4.687 |
6.341 |
GLUT 1 |
4JA4
|
Morin-3-O-galactoside |
4.123 |
L-Pyroglutamic acid |
5.153 |
eNOS |
3EAH
|
4.765 |
3.994 |
AKT |
3QKK
|
3.065 |
3.074 |
GLUT 1 |
4JA4
|
Morin |
4.321 |
L-Phenylalanine |
4.485 |
eNOS |
3EAH
|
4.544 |
5.296 |
AKT |
3QKK
|
5.021 |
5.431 |
3.8 Compounds 3, 4, 11, 12, 23, and 27 increased cellular viability and stimulated NO production in HUVECs
In order to further explore the active substances of BE that have protective effects on blood vessels, we used the L-NAME-induced HUVECs hypertension model for MTT assay experiments. The results (Fig. S3†) show that compounds 3, 4, 11, 12, 23, and 27 had obvious protective effects on L-NAME-induced HUVECs. However, there were three compounds (7, 19, and 21) that hardly protected HUVECs from damage, and compounds 1, 7, and 9 had a certain protective effect on the damage caused by L-NAME.
The concentration of NO is crucial in the progression of vascular dysfunction as well. Therefore, we measured the NO content in the supernatant fluid of the HUVEC culture medium. The levels of NO for compounds 3, 4, 11, 12, 23, and 27 were significantly increased compared with the M (L-NAME) group. The NO release capacity was also increased by treatment with compounds 1, 7, or 9, but showed no significant difference. In addition, compounds 7, 9, and 21 could not add to the capacity to release NO (Fig. 8).
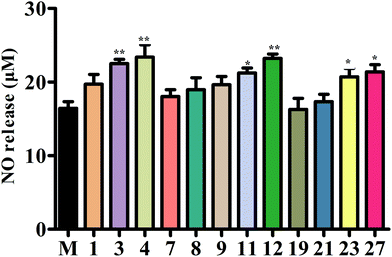 |
| Fig. 8 The concentration of NO. Secreted NO was measured by the Griess assay in cell culture medium. | |
In vitro study further revealed that compounds 3, 4, 11, 12, 23, and 27 may play the most important role in antihypertensive effects. However, further in vivo studies are needed to validate these active compounds in BE.
4. Discussion
Angiotensin II is a peptide hormone of the renin–angiotensin–aldosterone system, which has pleiotropic effects on the vascular system and is a key mediator of hypertensive nephropathy. It plays a crucial role in the development of renal fibrosis and inflammation.48 ET-1 is the most effective endogenous vasoconstrictor, and is strongly linked with the pathogenesis of hypertension.18 In addition to increasing BP through vasoconstriction, ET-1 also contributes to the regulation of renal sodium and water, and causes vascular remodeling and endothelial dysfunction in the vascular system, which is common in hypertension. Therefore, blocking ET-1 may lower blood pressure and provide broader cardiovascular protection.49 Several studies50,51 have demonstrated that TNF-α levels in the serum of hypertensive patients were higher than those in healthy patients. Results suggest that BE could reduce the production of Ang II, ET-1 and TNF-α, which could lower inflammation and hypertension.
NO is a critical factor in maintaining various normal vascular functions in the cardiovascular system. It is regulated by means of vasodilatation and anti-atherosclerosis.52 Decrease in NO bioavailability is considered to be a hallmark of endothelial dysfunction and plays a critical role in regulating blood pressure elevation.53 It has been reported that direct inhibition of intrarenal NO can cause elevated blood pressure.54 In this study, NO level was significantly increased after BE treatment, and the effect was close to that of the control group. These data indicate that the antihypertensive effect of BE was also associated with nutrition of endothelium function by eNOS/NO regulation.
GLUT 1 is a crucial enzyme regulating glucose metabolism.55 In the past few decades, consumption of fructose has increased rapidly, and at the same time the incidence of hypertension has risen.56 Glucose uptake is controlled by the surface level of glucose transporters and the activity of those transporters. Maher et al. revealed that GLUT 1 was overexpressed in brain microvascular endothelial cells of hypertensive patients.57 In this study, GLUT 1 could be decreased by BE, which shows that BE had a better effect in maintaining normal vascular function.
Endothelial dysfunction is a characteristic of underlying vascular disease and can be simply defined as a decrease in the endothelium-dependent vasodilatation, suggesting that eNOS levels are downregulated.58 A previous study59 has shown that Akt and eNOS phosphorylation play a key role in controlling vascular tone and controlling systolic blood pressure reduction. Our current results demonstrate that BE had a great protective effect on aortic endothelial function and promoted eNOS protein in the aorta. Our research provides strong evidence that BE is superior to Ni in endothelial-dependent vasodilation.
The Akt–eNOS pathway plays a crucial role in the endothelial function of the entire aorta, especially in the vascular endothelium. According to recent studies,60,61 activation of Akt increases the effectiveness of NO by phosphorylation of eNOS at Ser1177. The expression of p-Akt (Ser473) and p-eNOS (Ser1177) in vessel endothelia of SHR rats was significantly reduced after administration of BE. These data provide the vital statistical proof for maintaining hypertension, vascular structure damage, endothelial-associated vascular function, and inhabiting abnormal proliferation of smooth muscle cells. Meanwhile, the levels of NO in blood vessels of SHR rats were decreased, and increased after BE curing. Medical treatment enhanced the production of NO by increasing the expression of p-eNOS and p-Akt.62 Thus, BE improved the endothelium-dependent vascular function mediated by Akt–eNOS phosphorylation, suggesting significant advantages in the continuous modification of baroreflex functions for BP reduction.
5. Conclusions
Our results confirmed the hypothesis that oral administration of ethanol extracts of bayberry could lower blood pressure in spontaneously hypertensive rats. Among 28 compounds identified in BE, 12 compounds (compounds 1, 3, 4, 7–9, 11, 12, 19, 21, 23, and 27) probably interacted with GLUT 1, eNOS and Akt for their antihypertensive effect. In a further in vitro study, compounds 3, 4, 11, 12, 23, and 27 showed a significant protective effect on the L-NAME-induced HUVEC hypertension model. However, further in vivo studies are needed to validate the active compounds in BE. These results suggest that BE has an advantage in preventing hypertension compared with traditional therapies. The results presented here indicate that BE directly promotes NO release and eNOS activation in endothelial cells through the phosphorylation of Akt, and that BE plays a crucial role in controlling vascular remodeling in the pathogenesis of hypertension. Hypertension is one of the main symptoms of lifestyle-related diseases. Our results also indicate that Myrica rubra, as a functional food, has significant potential to prevent hypertension and its complications in daily life.
Ethics statement
All experiments in this study were carried out in accordance with the NIH Guide for the Care and Use of Laboratory Animals, and maximum efforts were made to minimize animal suffering and the number of animals necessary for the capture of reliable data.
Conflicts of interest
We confirm that this manuscript has not been published by another journal. All authors have approved the manuscript and agree with its submission to your journal. The authors declare that there is no conflict of interest regarding the publication of this paper.
Acknowledgements
We thank Scientific Instrument Center of Wuhan University for the LC-MS analysis.
References
- T. Rajeshwari, B. Raja and J. Manivannan,
et al., Valproic acid prevents the deregulation of lipid metabolism and renal renin-angiotensin system in L-NAME induced nitric oxide deficient hypertensive rats, Environ. Toxicol. Pharmacol., 2014, 37, 936–945 CrossRef CAS PubMed.
- S. S. Lim, T. Vos and A. D. Flaxman,
et al., A comparative risk assessment of burden of disease and injury attributable to 67 risk factors and risk factor clusters in 21 regions, 1990-2010: a systematic analysis for the Global Burden of Disease Study 2010, Lancet, 2012, 380, 2224–2260 CrossRef.
- F. Dong, D. Wang and L. Pan,
et al., Disparities in Hypertension Prevalence, Awareness, Treatment and Control between Bouyei and Han: Results from a Bi-Ethnic Health Survey in Developing Regions from South China, Int. J. Environ. Res. Public Health, 2016, 13, 233 CrossRef PubMed.
- P. M. Kearney, M. Whelton and K. Reynolds,
et al., Global burden of hypertension: analysis of worldwide data, Lancet, 2005, 365, 217–223 CrossRef.
- A. Wirth, Z. Benyo and M. Lukasova,
et al., G12-G13-LARG-mediated signaling in vascular smooth muscle is required for salt-induced hypertension, Nat. Med., 2008, 14, 64–68 CrossRef CAS PubMed.
- A. C. Straub, A. W. Lohman and M. Billaud,
et al., Endothelial cell expression of haemoglobin alpha regulates nitric oxide signalling, Nature, 2012, 491, 473–477 CrossRef CAS PubMed.
- J. F. Gielis, L. Quirynen and J. J. Briede,
et al., Pathogenetic role of endothelial nitric oxide synthase uncoupling during lung ischaemia-reperfusion injury, Eur. J. Cardiothorac. Surg., 2017, 52, 256–263 CrossRef PubMed.
- U. Forstermann and W. C. Sessa, Nitric oxide synthases: regulation and function, Eur. Heart J., 2012, 33, 829–837 CrossRef PubMed , 837a.
- Y. Zhou, W. P. Dirksen and G. J. Babu,
et al., Differential vasoconstrictions induced by angiotensin II: role of AT1 and AT2 receptors in isolated C57BL/6J mouse blood vessels, Am. J. Physiol. Heart Circ. Physiol., 2003, 285, H2797–H2803 CrossRef CAS PubMed.
- P. Fransen, C. E. Van Hove and A. J. Leloup,
et al., Effect of angiotensin II-induced arterial hypertension on the voltage-dependent contractions of mouse arteries, Pflüg. Arch., 2016, 468, 257–267 CrossRef CAS PubMed.
- A. Russell and S. Watts, Vascular reactivity of isolated thoracic aorta of the C57BL/6J mouse, J. Pharmacol. Exp. Ther., 2000, 294, 598–604 CAS.
- R. E. Aluko, Antihypertensive peptides from food proteins, Annu. Rev. Food Sci. Technol., 2015, 6, 235–262 CrossRef CAS PubMed.
- Y. Solak, B. Afsar and N. D. Vaziri,
et al., Hypertension as an autoimmune and inflammatory disease, Hypertens. Res., 2016, 39, 567–573 CrossRef CAS PubMed.
- S. M. Krishnan, J. K. Dowling and Y. H. Ling,
et al., Inflammasome activity is essential for one kidney/deoxycorticosterone acetate/salt-induced hypertension in mice, Br. J. Pharmacol., 2016, 173, 752–765 CrossRef CAS PubMed.
- J. F. Navarro-González, C. Mora and M. Muros,
et al., Association of tumor necrosis factor-alpha with early target organ damage in newly diagnosed patients with essential hypertension, J. Hypertens., 2008, 26, 2168–2175 CrossRef PubMed.
- H. L. Elliott, Gender differences with antihypertensive drug treatment, J. Hypertens., 2002, 20, 1491–1492 CrossRef CAS PubMed.
- H. Matsuda, T. Morikawa and J. Tao,
et al., Bioactive constituents of Chinese natural medicines. VII. Inhibitors of degranulation in RBL-2H3 cells and absolute stereostructures of three new diarylheptanoid glycosides from the bark of Myrica rubra, Chem. Pharm. Bull., 2002, 50, 208–215 CrossRef CAS PubMed.
- M. Yoshikawa, H. Shimada and N. Nishida,
et al., Antidiabetic principles of natural medicines. II. Aldose reductase and alpha-glucosidase inhibitors from Brazilian natural medicine, the leaves of Myrcia multiflora DC. (Myrtaceae): structures of myrciacitrins I and II and myrciaphenones A and B, Chem. Pharm. Bull., 1998, 46, 113–119 CrossRef CAS PubMed.
- H. Matsuda, T. Morikawa and J. Tao,
et al., Bioactive constituents of Chinese natural medicines. VII. Inhibitors of degranulation in RBL-2H3 cells and absolute stereostructures of three new diarylheptanoid glycosides from the bark of Myrica rubra, Chem. Pharm. Bull., 2002, 50, 208–215 CrossRef CAS PubMed.
- L. Xu, J. Gao and Y. Wang,
et al., Myrica rubra Extracts Protect the Liver from CCl(4)-Induced Damage, J. Evidence-Based Complementary Altern. Med., 2011, 2011, 518302 Search PubMed.
- N. Kumar, P. Bhandari and B. Singh,
et al., Antioxidant activity and ultra-performance LC-electrospray ionization-quadrupole time-of-flight mass spectrometry for phenolics-based fingerprinting of Rose species: Rosa damascena, Rosa bourboniana and Rosa brunonii, Food Chem. Toxicol., 2009, 47, 361–367 CrossRef CAS PubMed.
- J. Oszmianski, A. Wojdylo and J. Gorzelany,
et al., Identification and characterization of low molecular weight polyphenols in berry leaf extracts by HPLC-DAD and LC-ESI/MS, J. Agric. Food Chem., 2011, 59, 12830–12835 CrossRef CAS PubMed.
- H. X. Cui, J. H. Chen and J. W. Li,
et al., Protection of Anthocyanin from Myrica rubra against Cerebral Ischemia-Reperfusion Injury via Modulation of the TLR4/NF-kappaB and NLRP3 Pathways, Molecules, 2018, 23, 1788–1799 CrossRef PubMed.
- T. Inoue, Y. Arai and M. Nagai, Diarylheptanoids in the bark of Myrica rubra Sieb. et Zucc, Yakugaku Zasshi, 1984, 104, 37–41 CrossRef CAS PubMed.
- T. Inoue, Constituents of Acer nikoense and Myrica rubra. On diarylheptanoids, Yakugaku Zasshi, 1993, 113, 181–197 CrossRef CAS PubMed.
- A. J. Tipton, J. B. Musall and G. R. Crislip,
et al., Greater transforming growth factor-beta in adult female SHR is dependent on blood pressure, but does not account for sex differences in renal T-regulatory cells, Am. J. Physiol. Renal. Physiol., 2017, 313, F847–F853 CrossRef PubMed.
- B. Piotrkowski, V. Calabro and M. Galleano,
et al., (-)-Epicatechin prevents alterations in the metabolism of superoxide anion and nitric oxide in the hearts of L-NAME-treated rats, Food Funct., 2015, 6, 155–161 RSC.
- J. Wisniewski, M. G. Fleszar and J. Piechowicz,
et al., A novel mass spectrometry-based method for simultaneous determination of asymmetric and symmetric dimethylarginine, l-arginine and l-citrulline optimized for LC-MS-TOF and LC-MS/MS, Biomed. Chromatogr., 2017, 31, e3994 CrossRef PubMed.
- Y. Shi, S. Tse and B. Rago,
et al., Quantification of fumarate and investigation of endogenous and exogenous fumarate stability in rat plasma by LC-MS/MS, Bioanalysis, 2016, 8, 661–675 CrossRef CAS PubMed.
- M. Peitzsch, D. Pelzel and P. Lattke,
et al., Preservation of urine free catecholamines and their free O-methylated metabolites with citric acid as an alternative to hydrochloric acid for LC-MS/MS-based analyses, Clin. Chem. Lab. Med., 2016, 54, 37–43 CAS.
- P. Purwaha, L. P. Silva and D. H. Hawke,
et al., An artifact in LC-MS/MS measurement of glutamine and glutamic acid: in-source cyclization to pyroglutamic acid, Anal. Chem., 2014, 86, 5633–5637 CrossRef CAS PubMed.
- L. Liu, X. Yin and X. Wang,
et al., Determination of dihydromyricetin in rat plasma by LC-MS/MS and its application to a pharmacokinetic study, Pharm. Biol., 2017, 55, 657–662 CrossRef CAS PubMed.
- J. Sun, F. Liang and Y. Bin,
et al., Screening non-colored phenolics in red wines using liquid chromatography/ultraviolet and mass spectrometry/mass spectrometry libraries, Molecules, 2007, 12, 679–693 CrossRef CAS PubMed.
- J. Lee, B. L. S. Chan and A. E. Mitchell, Identification/quantification of free and bound phenolic acids in peel and pulp of apples (Malus domestica) using high resolution mass spectrometry (HRMS), Food Chem., 2017, 215, 301–310 CrossRef CAS PubMed.
- Z. Li, X. Guo and Z. Cao,
et al., New MS network analysis pattern for the rapid identification of constituents from traditional Chinese medicine prescription Lishukang capsules in vitro and in vivo based onUHPLC/Q-TOF-MS, Talanta, 2018, 189, 606–621 CrossRef CAS PubMed.
- S. T. Yu, J. Li and L. Guo,
et al., Integrated liquid chromatography-mass spectrometry and nuclear magnetic resonance spectra for the comprehensive characterization of various components in the Shuxuening injection, J. Chromatogr. A, 2019, 1599, 125–135 CrossRef CAS PubMed.
- V. Vaja, P. Ochodnicky and P. Krenek,
et al., Rapid large artery remodeling following the administration and withdrawal of calcium channel blockers in spontaneously hypertensive rats, Eur. J. Pharmacol., 2009, 619, 85–91 CrossRef CAS PubMed.
- K. Niwa, J. K. Perloff and S. M. Bhuta,
et al., Structural abnormalities of great arterial walls in congenital heart disease: light and electron microscopic analyses, Circulation, 2001, 103, 393–400 CrossRef CAS PubMed.
- L. Xie, P. Lin, H. Xie and C. Xu, Effects of atorvastatin and losartan on monocrotaline-induced pulmonary artery remodeling in rats, Clin. Exp. Hypertens., 2010, 32, 547–554 CrossRef CAS PubMed.
- D. K. Sharma, A. Manral and V. Saini,
et al., Novel diallyldisulfide analogs ameliorate cardiovascular remodeling in rats with L-NAME-induced hypertension, Eur. J. Pharmacol., 2012, 691, 198–208 CrossRef PubMed.
- Y. M. Barri, Hypertension and kidney disease: a deadly connection, Curr. Hypertens. Rep., 2008, 10, 39–45 CrossRef PubMed.
- J. M. Krzesinski and E. P. Cohen, Hypertension and the kidney, Acta Clin. Belg., 2007, 62, 5–14 CrossRef CAS PubMed.
- A. L. Olson and J. E. Pessin, Structure, function, and regulation of the mammalian facilitative glucose transporter gene family, Annu. Rev. Nutr., 1996, 16, 235–256 CrossRef CAS PubMed.
- P. L. Huang, Z. Huang and H. Mashimo,
et al., Hypertension in mice lacking the gene for endothelial nitric oxide synthase, Nature, 1995, 377, 239–242 CrossRef CAS PubMed.
- V. A. Morrow, F. Foufelle and J. M. Connell,
et al., Direct activation of AMP-activated protein kinase stimulates nitric-oxide synthesis in human aortic endothelial cells, J. Biol. Chem., 2003, 278, 31629–31639 CrossRef CAS PubMed.
- X. Li, J. Li and Z. Li,
et al., Fucoidan from Undaria pinnatifida prevents vascular dysfunction through PI3K/Akt/eNOS-dependent mechanisms in the L-NAME-induced hypertensive rat model, Food Funct., 2016, 7, 2398–2408 RSC.
- K. Y. Hsin, S. Ghosh and H. Kitano, Combining machine learning systems and multiple docking simulation packages to improve docking prediction reliability for network pharmacology, PLoS One, 2013, 8, e83922 CrossRef PubMed.
- G. Liu, Y. Li and X. R. Huang,
et al., Smad7 inhibits AngII-mediated hypertensive nephropathy in a mouse model of hypertension, Clin. Sci., 2014, 127, 195–208 CrossRef CAS PubMed.
- T. Okura, M. Jotoku and J. Irita,
et al., Association between cystatin C and inflammation in patients with essential hypertension, Clin. Exp. Nephrol., 2010, 14, 584–588 CrossRef CAS PubMed.
- I. D. Bespalova, N. V. Riazantseva and V. V. Kaliuzhin,
et al., Effect of atorvastatin on pro-inflammatory status (in vivo and in vitro) in patients with essential hypertension and metabolic syndrome, Kardiologiia, 2014, 54, 37–43 CAS.
- R. C. Moorhouse, D. J. Webb and D. C. Kluth,
et al., Endothelin antagonism and its role in the treatment of hypertension, Curr. Hypertens. Rep., 2013, 15, 489–496 CrossRef CAS PubMed.
- J. Seo, J. Y. Lee and M. S. Sung,
et al., Arsenite Acutely Decreases Nitric Oxide Production via the ROS-Protein Phosphatase 1-Endothelial Nitric Oxide Synthase-Thr(497) Signaling Cascade, Biomol. Ther., 2014, 22, 510–518 CrossRef CAS PubMed.
- J. Davignon and P. Ganz, Role of endothelial dysfunction in atherosclerosis, Circulation, 2004, 109, I27–I32 Search PubMed.
- D. S. Majid, S. A. Omoro and S. Y. Chin,
et al., Intrarenal nitric oxide activity and pressure natriuresis in anesthetized dogs, Hypertension, 1998, 32, 266–272 CrossRef CAS PubMed.
- K. M. Pereira, F. N. Chaves and T. S. Viana,
et al., Oxygen metabolism in oral cancer: HIF and GLUTs (review), Oncol. Lett., 2013, 6, 311–316 CrossRef PubMed.
- B. P. Marriott, N. Cole and E. Lee, National estimates of dietary fructose intake increased from 1977 to 2004 in the United States, J. Nutr., 2009, 139, 1228S–1235S CrossRef CAS PubMed.
- F. Maher, S. J. Vannucci and I. A. Simpson, Glucose transporter proteins in brain, FASEB J., 1994, 8, 1003–1011 CrossRef CAS PubMed.
- J. A. Panza, P. R. Casino and D. M. Badar,
et al., Effect of increased availability of endothelium-derived nitric oxide precursor on endothelium-dependent vascular relaxation in normal subjects and in patients with essential hypertension, Circulation, 1993, 87, 1475–1481 CrossRef CAS PubMed.
- Z. Shah, C. Pineda and T. Kampfrath,
et al., Acute DPP-4 inhibition modulates vascular tone through GLP-1 independent pathways, Vasc. Pharmacol., 2011, 55, 2–9 CrossRef CAS PubMed.
- S. Li, Q. Li and X. Lv,
et al., Aurantio-obtusin relaxes systemic arteries through endothelial PI3K/AKT/eNOS-dependent signaling pathway in rats, J. Pharmacol. Sci., 2015, 128, 108–115 CrossRef CAS PubMed.
- C. F. Garcia-Prieto, F. Hernandez-Nuno and D. D. Rio,
et al., High-fat diet induces endothelial dysfunction through a down-regulation of the endothelial AMPK-PI3K-Akt-eNOS pathway, Mol. Nutr. Food Res., 2015, 59, 520–532 CrossRef CAS PubMed.
- Z. Ying, X. Xie, M. Chen and K. Yi,
et al., Alpha-lipoic acid activates eNOS through activation of PI3-kinase/Akt signaling pathway, Vasc. Pharmacol., 2015, 64, 28–35 CrossRef CAS PubMed.
Footnotes |
† Electronic supplementary information (ESI) available: Chemical structures of main components identified in the extract of Myrica rubra. Proposed fragmentation patterns pathway of quercetin-3-O-2′,6′-dirhamnosylglucoside. The cellular viability of H2O2-induced HUVECs injury with compounds 1, 3, 4, 7, 8, 9, 11, 12, 19, 21, 23, 27 at different concentrations. See DOI: 10.1039/c9ra05895h |
‡ These authors contributed equally. |
|
This journal is © The Royal Society of Chemistry 2020 |
Click here to see how this site uses Cookies. View our privacy policy here.