DOI:
10.1039/C9RA08042B
(Paper)
RSC Adv., 2020,
10, 6491-6496
Biotransformation of α-terpineol by Alternaria alternata†
Received
4th October 2019
, Accepted 15th January 2020
First published on 11th February 2020
Abstract
α-Terpineol (1), the main volatile constituent in some traditional Chinese medicines, has been reported to be metabolized to 4R-oleuropeic acid by the larvae of common cutworms. The present study verified that α-terpineol could be converted to 4R-oleuropeic acid (2) and (1S,2R,4R)-p-menthane-1,2,8-triol (3) by Alternaria alternata fermentation. Using shortened fermentation times, 7-hydroxy-α-terpineol (2a) was identified as an oxidative intermediate, which was consistent with the hypothesis put forward by previous studies. Cytochrome P450 enzymes were also confirmed to catalyze this biotransformation. This is the first study on the biotransformation of α-terpineol by microbial fermentation.
1 Introduction
Biotransformation is superior to chemical synthesis due to superior regio- and stereo-selectivities,1 environmentally friendly procedures,2 and mild reaction conditions.3 Biocatalysts play an important role in regio- and stereo-selective reactions,4,5 including acetylation,6 hydroxylation,7 and epoxidation of steroids.8 Our previous studies have shown that many transformations have been achieved by microorganisms.9–12
α-Terpineol (1), a cyclic monoterpene alcohol, is widely present in many traditional Chinese medicines, such as Thymus vulgaris, Annona squamosa, and Magnolia denudata. α-Terpineol is also commonly used in industrial syntheses13,14 and certain pharmaceutical preparations.15–17 The in vivo metabolic pathways of α-terpineol were confirmed to be connected to the digestive system and the P450 enzyme system.18,19 It has been reported that α-terpineol is oxidized to oleuropeic acid by the larvae of common cutworms (Spodoptera litura).20 It is reasonable to speculate that microbes possessing P450 enzyme system might facilitate above transformation. As a key raw material, oleuropeic acid (2) can be used to synthesize a variety of active substances.21–25 Therefore, the investigation of the accumulation of oleuropeic acid (2) by microbial fermentation is important and prospective.
In the present study, the biotransformations of α-terpineol were screened in liquid fermentation using nine plant endophyte fungi to confirm its biological oxidation. The results showed that Alternaria alternata fermentation could facilitate the biotransformation of interest. Isolation of the fermentation broth led to the identification of two oxidative products 4R-oleuropeic acid (2) and (1S,2R,4R)-p-menthane-1,2,8-triol (3) (Fig. 1). 7-Hydroxy-α-terpineol (2a), isolated from the fermentation broth by shortening fermentation time, was confirmed to be an oxidative intermediate. The oxidation of α-terpinol (1) to compound 2 is consistent with larvae that reported in the literature.20 The conversion ratio of α-terpineol (1) to 4R-oleuropeic acid (2) was determined by high-performance liquid chromatography (HPLC) and the yield of compound 3 was determined by gas chromatography (GC). Additionally, cytochrome P450 enzymes were further confirmed to catalyze this biotransformation. Compared with feeding insects to collect their excreta, the method of liquid fermentation by fungi to convert α-terpinol is more potential for mass production. This is the first study to confirm the biotransformation of α-terpineol, and this study could serve as a reference for further studies on the internal metabolism of this compound.
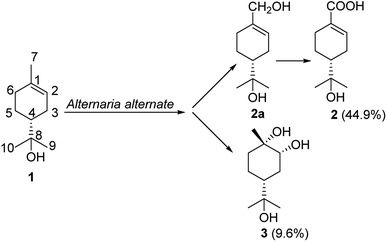 |
| Fig. 1 The biotransformation of 1 by Alternaria alternate. | |
2 Experimental
2.1 Chemicals
α-Terpineol (compound 1, CAS: 10482-56-1) was purchased from Aladdin Industrial Corporation. Silica gel (200–300 mesh) for column chromatography was purchased from Qingdao Marine Chemical Corporation, Qingdao, China. Acetonitrile and methanol (Hipure Chem, China) were of HPLC grade. 1-Aminobenzotriazole (ABT, 98%), piperonyl butoxide (PBO, 90%), and n-tetradecane (98%) were obtained from J&K Scientific Ltd. (Beijing, China). CDCl3 (99.9 atom% D, containing 0.03% TMS) was purchased from ARMAR Chemicals (Ningbo, China) and MeOD (99.9 atom% D, contains 0.03% TMS) was purchased from Sigma-Aldrich (Shanghai, China). The water used in this study was purified using a water purification system (Chengdu, China) and had a resistivity ≥18.25 MΩ cm−1. Other reagents were of analytical grade and were purchased from Tianjin Fuyu Fine Chemical Co. Ltd. (Tianjin, China).
2.2 Nuclear magnetic resonance (NMR) spectroscopy
The NMR spectra were obtained on a Bruker Avance 400 MHz spectrometer (400.00 MHz, 1H; 100.00 MHz, 13C) using tetramethylsilane (TMS) as the internal reference.
2.3 Polarimeter
Optical rotation was measured with a Jasco P-1020 digital polarimeter (Jasco, Tokyo, Japan). A Shimadzu UV-vis 2550.
2.4 HPLC
An Agilent Technologies 1200 Series HPLC equipped with an Agilent dual pump, a diode array detector (DAD), an Agilent ZORBAX SB-C18 (4.6 × 250 mm, 5 μm) and an Agilent Guard Column Hardware Kit was used for all HLPC analyses.
2.5 GC
An Agilent Technologies 7890B GC System with a flame ionization detector (FID), an Agilent 19091J-413 HP-5 (30 m × 320 μm × 0.25 μm) was used for all GC analyses.
2.6 Microorganisms and culture medium
A. alternata, Fusarium solani, Fusarium avenaceum, Aspergillus tubingensis, Fusarium oxysporum, Myrothecium inundatum, Aspergillus sydowii, Penicillium virgatum and Aspergillus versicolor were obtained from the Yunnan Institute of Microbiology, Yunnan Province, China. A. tubingensis, A. sydowii, P. virgatum and A. versicolor were separated from the root of Panax notoginseng. F. oxysporum, M. inundatum, A. alternata, F. solani, F. avenaceum were separated from the tuber of Gastrodia elata. Culture and biotransformation experiments used fungi grown in potato dextrose agar (PDA, 1 L of water, 200 g of potato, 20 g of dextrose and 15 g of agar) slant culture medium.
2.7 Screening of biotransformation
PDA slant culture medium was inoculated with the aforementioned fungi and kept in a constant temperature incubator at 28 °C for 7 days. Then, the mature slants were added to conical flasks (150 mL) that contained 20 mL of potato dextrose broth (PDB: 1 L of water, 200 g of potato starch, and 20 g of dextrose) seed culture medium and placed on a rotary shaker shaking at 120 rpm at 28 °C for 3 days. And then 150 mL of PDB was added to a 500 mL conical flask to serve as the fermentation culture medium. After the fermentation culture medium was sterilized in an autoclave at 121 °C and 1.06 kg cm−2 for 30 min, the seed cultures and 1 mL (5 μL mL−1) of substrate were added and the medium was incubated at room temperature for 7 days. Then, the PDB before and after fermentation was immersed in EtOAc (200 mL) and extracted with an ultrasonic cleaner three times (30 min each), extracts were analyzed with thin layer chromatography (TLC).
2.8 Biotransformation process investigation
A. alternata was grown in shaking cultures at 28 °C for 3 days in 150 mL conical flasks that contained 50 mL of sterile PDB. The α-terpineol (10.0 μL each) dissolved in MeOH (0.5 mL) was evenly distributed between two flasks containing 50 mL of the previously mentioned sterile PDB and strains that had been cultured for 3 days. The α-terpineol mixtures were incubated for a further 1, 5, 7, or 9 days each. The broth was extracted with EtOAc (200 mL) three times to afford the extract. HPLC was used to determine the content of compound 2a and 2 in samples at different times.
2.9 Purification of metabolites
The extracts of strains that had been fermented for 5 days were subjected to a Sephadex LH-20 column (CHCl3–MeOH (1
:
1)) and silica gel CC (PE–EA, (15
:
1, 7
:
1, and 4
:
1)) to obtain compound 2a (7.8 mg). In addition, extracts of strains that had been fermented for 9 days were subjected to a Sephadex LH-20 column (CHCl3–MeOH (1
:
1)) and silica gel CC (PE–EA, (4
:
1)) to obtain compound 2 (14.5 mg), or the same extract was subjected to silica gel CC (PE–EA (2
:
1)) and a Sephadex LH-20 column (MeOH) to yield compound 3 (12.3 mg).
2.10 Spectroscopic data
7-Hydroxy-α-terpineol (2a). White oil; [α]25D −25.1 (c 0.020, CHCl3); EIMS m/z 170 M+; for 1H and 13C NMR spectral data, see Table 1.
Table 1 The NMR spectroscopic data for 2a, 3 in CDCl3 and 2 in MeOD
Position |
2a |
2 |
3 |
δC |
δH mult. (J in Hz) |
δC |
δH mult. (J in Hz) |
δC |
δH mult. (J in Hz) |
1 |
137.6 s |
|
131.7 s |
|
71.0 s |
|
2 |
122.6 d |
5.69 (m, 1H) |
140.8 d |
6.98 (t, 1H, 2.4) |
74.1 d |
3.61 (brs, 1H) |
3 |
23.7 t |
2.05 (m, 1H) |
24.6 t |
2.02 (m, 1H) |
30.0 t |
1.79 (m, 2H) |
1.24 (m, 1H) |
1.22 (m, 1H) |
4 |
45.2 d |
1.54 (m,1H) |
45.6 d |
1.53 (m, 1H) |
41.1 d |
1.63 (tt, 1H, 12.0, 6.0) |
5 |
26.5 t |
1.55 (m, 1H) |
26.4 t |
2.48 (br d, 1H, 16.0) |
22.3 t |
1.54 (t, 1H, 8.0) |
1.36 (m, 1H) |
2.08 (m, 1H) |
1.34 (td, 1H, 8.0, 4.0) |
6 |
26.7 t |
1.76 (m, 1H) |
28.5 t |
2.34 (br d, 1H, 16.0) |
33.7 t |
1.75 (t, 1H, 2.0) |
1.52 (m, 1H) |
2.01 (m, 1H) |
1.50 (t, 1H 3.6) |
7 |
67.2 t |
4.01 (t, 2H, 12.0) |
171.1 s |
|
27.8 q |
1.22 (s, 3H) |
8 |
72.9 s |
|
72.9 s |
|
72.8 s |
|
9 |
27.5 q |
1.19 (s, 3H) |
26.4 q |
1.17 (s, 3H) |
26.7 q |
1.18 (s, 3H) |
10 |
26.7 q |
1.24 (s, 3H) |
27.0 q |
1.17 (s, 3H) |
27.6 q |
1.14 (s, 3H) |
4R-Oleuropeic acid (2). White amorphous powder; [α]25D −38.9 (c 0.020, MeOH); EIMS m/z 184 M+; for 1H and 13C NMR spectral data, see Table 1.
(1S,2R,4R)-p-Menthane-1,2,8-triol (3). Amorphous powder; [α]25D +16.3 (c 0.020, CHCl3); EIMS m/z 188 M+; for 1H and 13C NMR spectral data, see Table 1.
2.11 Determining reaction yield by HPLC and GC analysis
Standards and samples were dissolved in MeOH (1.000 mL) and filtered through a 0.45 μm filter prior to injection into the HPLC and GC. Different concentrations of compound 2 and 3 methanol solution were injected into HPLC or GC and integrate the peak area to draw a standard working curve. Each sample was analyzed in triplicate. The contents of products were determined according to the corresponding calibration curves; further conversions were calculated on the basis of these contents.
A gradient elution system consisting of solvents A (0.1% HAc) and B (acetonitrile) was used for the HPLC analysis. The gradient program was as follows: 0–10 min, 20–70% solvent B; 10–40 min, 70–80% solvent B; 40–45 min, 80–100% solvent B; and 45–46 min, 100–20% solvent B and then held for 10 min. The detecting wavelength of the diode array detector was set to 225 nm. The flow rate was 1.0 mL min−1, the column temperature was set at 25 °C, and the injection volume was 10 μL.
Argon was used in the mobile phase of GC analysis, argon at a flow rate of 1 mL min−1 was used as a carrier gas. The oven temperature was programmed from 200 to 250 °C at 4 °C min−1. The injector and detector temperatures were 270 and 280 °C, respectively.
2.12 Effects of the inhibitors ABT and PBO and of the inducer n-tetradecane
The inhibitors ABT and PBO (40 μg mL−1, final concentration) and the inducer n-tetradecane (2%, v/v, final concentration) were added to the spore suspension after it had been incubated for 24 h. After an additional 48 h of culturing, 1 mL of the substrate (10 μL mL−1) was added. The spore suspension without the inhibitors and inducer was used as the positive control. The concentrations of the product and reactant were determined by HPLC and GC analysis after 6 h, 24 h, 48 h, 72 h and 96 h. Each sample was repeated in triplicate.
3 Results and discussion
3.1 Screening of microorganism
Oxidation reactions caused by microbes are commonly reported in the literature;26,27 thus it is reasonable to propose that α-terpineol was oxidized to 4R-oleuropeic acid by the enteric microorganism in the larvae of common cutworms. Therefore, in the present study, the biotransformation of α-terpineol was screened in liquid fermentation using nine plant pathogenic fungi to confirm its biological oxidation process. TLC was used to monitor the transformation process during fermentation. The results showed that A. alternata fermentation could facilitate the biotransformation of interest.
3.2 Identification of compounds
All 1H and 13C NMR spectral data of the known compounds 2a, 2 and 3 agreed with spectral data reported in the literature.20,28,29 The absolute configuration of compound 3 was determined based on the ROESY spectrum. As mentioned above, chiral center at C-4 remained unchanged during the fermentation process. The α-orientation of OH-2 was assigned on the basis of NOE correlations between H-4β [δH 1.63 (tt, J = 12.0, 6.0 Hz)] and H-2 [δH 3.61 (brs, 1H)]. The NOE correlation (Fig. 2) between CH3-7 [δH 1.22 (s, 3H)] and H-2 indicated that they were oriented in the same direction. Thus, the chiral centers in 3 were assigned as 1S, 2R and 4R. Compound 3 was chemically synthesized by a previously reported method,30 and this is the first report of its synthesis via microbial fermentation.
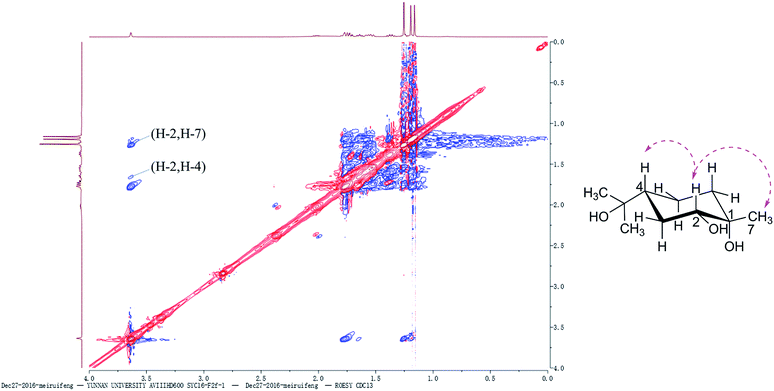 |
| Fig. 2 ROESY spectrum and Key NOE ( ) correlations of 3. | |
3.3 A. alternata fermentation of α-terpineol
Fermentation of α-terpineol by A. alternata for seven days allowed the isolation of two oxidative products, 4R-oleuropeic acid (2) and (1S,2R,4R)-p-menthane-1,2,8-triol (3). After reducing fermentation time to 5 days, we isolated 7-hydroxy-α-terpineol (2a) as a key oxidative intermediate, which confirmed the oxidation process of α-terpineol (1) to oleuropeic acid (2). The fermentation itself process was also investigated. As shown in Fig. 3, no absorption peak was detected at the beginning (Fig. 3a), when the fermentation time increased, compound 2a gradually appeared, reached a peak at 5 days (Fig. 3b), then decreased quickly (Fig. 3c) and finally disappeared (Fig. 3d). In addition, the content of compound 2 gradually increased and achieved its highest level after 9 days of fermentation (Fig. 3d), corresponding to a maximum conversion ratio of 44.9% ± 2.4%. This biotransformation facilitates the possibility of mass production of 4R-oleuropeic acid (2). The isolation of 3 indicated that A. alternata fermentation could facilitate cis-dihydroxylation of the C(1)–C(2) double bond in α-terpineol, which is the same as the reported in the literature.31–33 Compound 3 could not be detected by FID until four days fermentation. The yield of compound 3 after 9 days of fermentation was 9.6% ± 0.8%.
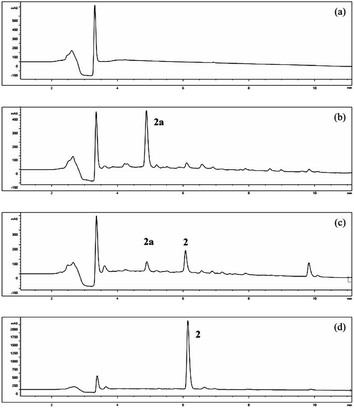 |
| Fig. 3 Detection of the biotransformation process of compound 2a to 2 by HPLC. Figure (a–d) shows the fermentation time: 1, 5, 7, 9 days. α-Terpineol was first oxidized to compound 2a then decreased quickly and finally oxidized to 2. | |
3.4 Effects of the inhibitors ABT and PBO and the inducer n-tetradecane
Cytochrome P450 monooxygenases catalyze the oxidation of a wide variety of endogenous and exogenous organic substrates typically by inserting an oxygen atom from atmospheric dioxygen into a carbon–hydrogen bond to produce the corresponding alcohol34,35 or carboxylic acid.36 In this study, compound 2 was proposed to be derived from α-terpineol via cytochrome P450 oxidative catalysis.
To determine the enzyme system involved in this biotransformation, special inhibition and induction experiments were performed. PBO (piperonyl butoxide, which contains a methylenedioxyphenyl (MDP) or benzodioxole moiety) is used to estimate the inhibition and induction of reactions mediated by cytochrome P450.37,38 ABT is a triazole P450-14DM inhibitor that is widely used as an inactivator of many P450 isozymes.39 n-Tetradecane is an effective inducer of cytochrome P450 enzymes.40 As shown in Fig. 4a and b, ABT and PBO exhibited a significant inhibitory effect on the conversion of α-terpineol to compound 2a at a concentration of 40 μg mL−1. Under such conditions, the production of compound 2 was very slow and we did not detect compound 2 by HPLC in these cases. In contrast, this transformation could be significantly induced by 2% (v/v) n-tetradecane (Fig. 4c). In the early stage of the fermentation, compound 2a was produced much more quickly than it was in the absence of the inducer. After 90 hours of fermentation, the content of 2a decreased quickly to produce the final oxidation product 2, indicating that n-tetradecane could promote the biotransformation from α-terpineol to 2. The results of the inhibition and induction experiments indicated that cytochrome P450 enzymes serve as the catalysis for this biotransformation. The same approach was tried to accumulate compound 3, but the results of the experiment showed that the yield of compound 3 had no relationship with P450 enzyme inhibitors and inducers. Through literature review,41–44 compound 3 is more likely to be catalyzed by dioxygenase.
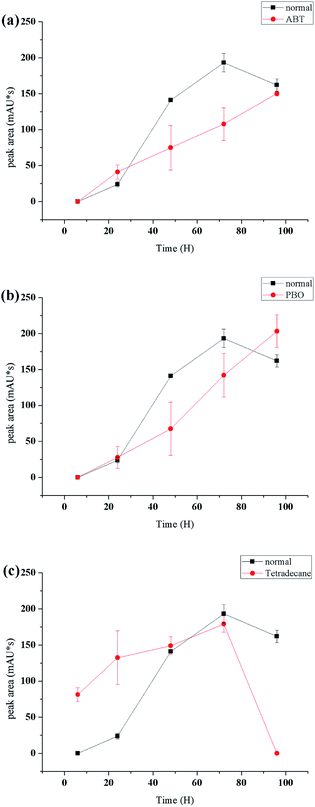 |
| Fig. 4 Effects of ABT ((a), 40 μg mL−1), PBO ((b), 40 μg mL−1) and n-tetradecane ((c), 2% v/v) on the biotransformation of 1 to 2a. | |
4 Conclusions
In summary, the present study verified that α-terpineol could be converted to 4R-oleuropeic acid (2) and a cis-dihydroxyl derivative (3) by A. alternata. 7-Hydroxy-α-terpineol (2a) was shown to be an intermediate in the biotransformation of α-terpineol to 2. Cytochrome P450 enzymes were confirmed to catalyse this biotransformation. This is the first study to clarify the biotransformation of α-terpineol, and this study could serve as a reference for further investigations on the internal metabolism of this compound.
Conflicts of interest
There are no conflicts to declare.
Acknowledgements
This work was financially supported by a grant from the Natural Science Foundation of China (No. 81960760), Program for Changjiang Scholars and Innovative Research Team in University (IRT_17R94), the project of Yunling Scholars of Yunnan province, a Program for Excellent Young Talents, and the grant from Yunnan University’s Research Innovation Fund for Graduate Students (No. 2018184).
Notes and references
- X. Chen, Y.-G. Zheng, Z.-Q. Liu and L.-H. Sun, J. Chromatogr. B: Anal. Technol. Biomed. Life Sci., 2015, 974, 57–64 CrossRef CAS PubMed.
- K. Pazdzior, J. Wrebiak, A. Klepacz-Smolka, M. Gmurek, L. Bilinska, L. Kos, J. Sojka-Ledakowicz and S. Ledakowicz, J. Environ. Manage., 2017, 195, 166–173 CrossRef CAS PubMed.
- Y. Simeo and J. V. Sinisterra, Cheminform, 2010, 41, 128–134 CrossRef.
- D. Romano, F. Bonomi, M. C. de Mattos, S. F. T. De, O. M. C. De and F. Molinari, Biotechnol. Adv., 2015, 33, 547–565 CrossRef CAS PubMed.
- M. V. Donova and O. V. Egorova, Appl. Microbiol. Biotechnol., 2012, 94, 1423 CrossRef CAS PubMed.
- J. Lu, S. Deng, H. Chen, J. Hou, B. Zhang, Y. Tian, C. Wang and X. Ma, J. Mol. Catal. B: Enzym., 2013, 89, 102–107 CrossRef CAS.
- A. V. Basso, V. E. Nicotra, A. Parra, A. Martínez and A. Fernándezvivas, J. Nat. Prod., 2016, 79, 1658–1667 CrossRef CAS PubMed.
- L. H. Huang, J. Li, G. Xu, X. H. Zhang, Y. G. Wang, Y. L. Yin and H. M. Liu, Steroids, 2010, 75, 1039 CrossRef CAS PubMed.
- J. Dong, L. Zhao, L. Cai, H. Fang, X. Chen and Z. Ding, J. Biosci. Bioeng., 2014, 118, 396–399 CrossRef CAS PubMed.
- Y. Li, L. Cai, J. W. Dong, Y. Xing, W. H. Duan, H. Zhou and Z. T. Ding, J. Agric. Food Chem., 2015, 63, 6596 CrossRef CAS PubMed.
- J. W. Dong, L. Cai, J. Xiong, X. H. Chen, W. Y. Wang, N. Shen, B. L. Liu and Z. T. Ding, Process Biochem., 2015, 50, 8–13 CrossRef CAS.
- L. Cai, J. W. Dong, L. X. Zhao, H. Zhou, Y. Xing, Y. Li, Z. J. Li, W. H. Duan, X. J. Li and Z. T. Ding, Process Biochem., 2016, 51, 933–940 CrossRef CAS.
- R. Vallinayagam, S. Vedharaj, W. L. Roberts, R. W. Dibble and S. M. Sarathy, Renewable Energy, 2017, 101, 1087–1093 CrossRef CAS.
- N. Negishi and K. Takeuchi, Thin Solid Films, 2001, 392, 249–253 CrossRef CAS.
- S. N. Park, Y. K. Lim, M. O. Freire, E. Cho, D. Jin and J. K. Kook, Anaerobe, 2012, 18, 369–372 CrossRef CAS PubMed.
- M. G. B. Oliveira, R. G. Brito, P. L. Santos, H. G. Araújo-Filho, J. S. S. Quintans, P. P. Menezes, M. R. Serafini, Y. M. B. G. Carvalho, J. C. Silva and J. R. G. S. Almeida, Chem.-Biol. Interact., 2016, 254, 54–62 CrossRef CAS PubMed.
- K. M. Madyastha and V. Renganathan, Can. J. Microbiol., 1984, 30, 1429 CrossRef CAS PubMed.
- K. M. Madyastha and V. Srivatsan, Bull. Environ. Contam. Toxicol., 1988, 41, 17–25 CrossRef CAS PubMed.
- Y. J. Choi, W. C. Sim, H. K. Choi, S. H. Lee and B. H. Lee, Food Chem. Toxicol., 2013, 55, 129–136 CrossRef CAS PubMed.
- M. Miyazawa and M. Ohsawa, J. Agric. Food Chem., 2002, 50, 4916–4918 CrossRef CAS PubMed.
- L. W. Tian, M. Xu, Y. Li, X. Y. Li, D. Wang, H. T. Zhu, C. R. Yang and Y. J. Zhang, Chem. Biodiversity, 2012, 9, 123–130 CrossRef CAS PubMed.
- J. Q. Goodger and I. E. Woodrow, Phytochemistry, 2011, 72, 2259–2266 CrossRef CAS PubMed.
- L. W. Tian, Y. J. Zhang, Y. F. Wang, C. C. Lai and C. R. Yang, J. Nat. Prod., 2009, 72, 1608–1611 CrossRef CAS PubMed.
- A. M. Heskes, C. N. Lincoln, J. Q. Goodger, I. E. Woodrow and T. A. Smith, J. Microsc., 2012, 247, 33 CrossRef CAS PubMed.
- J. Q. D. Goodger, B. Cao, I. Jayadi, S. J. Williams and I. E. Woodrow, Phytochemistry, 2009, 70, 1187–1194 CrossRef CAS PubMed.
- P. Soares-Castro, P. Montenegro-Silva, H. Heipieper and P. Santos, Appl. Environ. Microbiol., 2017, 83, 19 CrossRef PubMed.
- S.-H. Xu, W.-W. Wang, C. Zhang, X.-F. Liu, B.-Y. Yu and J. Zhang, Tetrahedron, 2017, 73, 3086–3092 CrossRef CAS.
- R. X. Tan, J. Jakupovic, F. Bohlmann, Z. J. Jia and S. Huneck, Phytochemistry, 1991, 30, 583–587 CrossRef CAS.
- T. Ishikawa, Y. Sega and J. Kitajima, Chem. Pharm. Bull., 2001, 49, 584 CrossRef CAS PubMed.
- R. M. Carman, M. T. Fletcher, R. M. Carman and M. T. Fletcher, Aust. J. Chem., 1984, 37, 2129–2136 CrossRef CAS.
- M. F. Zia, Á. G. Vasko, Z. Riedl, C. Hametner, G. Hajós, K. Mereiter and M. D. Mihovilovic, Tetrahedron, 2016, 72, 7348–7355 CrossRef CAS.
- D. R. Boyd, N. D. Sharma, J. G. Carroll, P. L. Loke, C. R. O'Dowd and C. C. R. Allen, RSC Adv., 2013, 3, 10944 RSC.
- D. R. Boyd, N. D. Sharma, I. N. Brannigan, T. A. Evans, S. A. Haughey, B. T. McMurray, J. F. Malone, P. B. McIntyre, P. J. Stevenson and C. C. Allen, Org. Biomol. Chem., 2012, 10, 7292–7304 RSC.
- W. Adam, Z. Lukacs, D. Harmsen, C. R. Sahamoller and P. Schreier, J. Org. Chem., 2000, 65, 878–882 CrossRef CAS PubMed.
- C. J. Whitehouse, S. G. Bell and L. L. Wong, Chemistry, 2008, 14, 10905 CrossRef CAS PubMed.
- E. Widemann, B. Grausem, H. Renault, E. Pineau, C. Heinrich, R. Lugan, P. Ullmann, L. Miesch, Y. Aubert, M. Miesch, T. Heitz and F. Pinot, Phytochemistry, 2015, 117, 388–399 CrossRef CAS PubMed.
- A. Rasool, N. Joußen, S. Lorenz, R. Ellinger, B. Schneider, S. A. Khan, M. Ashfaq and D. G. Heckel, Insect Biochem. Mol. Biol., 2014, 53, 54 CrossRef CAS PubMed.
- A. C. Kotze, Int. J. Parasitol., 1999, 29, 389 CrossRef CAS PubMed.
- C. Linder and N. Renaud, Drug Metab. Dispos., 2009, 37, 10–13 CrossRef CAS PubMed.
- D. Sanglard and A. Fiechter, FEBS Lett., 1989, 256, 128–134 CrossRef CAS.
- Y. Qu, B. Xu, X. Zhang, Q. Ma, H. Zhou, C. Kong, Z. Zhang and J. Zhou, Biochem. Eng. J., 2013, 72, 54–60 CrossRef CAS.
- W. Hüttel, Chem. Ing. Tech., 2013, 85, 809–817 CrossRef.
- H. Overwin, M. Gonzalez, V. Mendez, M. Seeger, V. Wray and B. Hofer, Appl. Microbiol. Biotechnol., 2016, 100, 8053–8061 CrossRef CAS PubMed.
- H. Overwin, M. Gonzalez, V. Mendez, F. Cardenas, M. Seeger and B. Hofer, Enzyme Microb. Technol., 2015, 81, 63–71 CrossRef CAS PubMed.
Footnotes |
† Electronic supplementary information (ESI) available. See DOI: 10.1039/c9ra08042b |
‡ The first two authors contributed equally to this work. |
|
This journal is © The Royal Society of Chemistry 2020 |
Click here to see how this site uses Cookies. View our privacy policy here.