DOI:
10.1039/C9RA09032K
(Paper)
RSC Adv., 2020,
10, 5351-5360
Lower Mg and S contents in solar salt used in kimchi enhances the taste and anticancer effects on HT-29 colon carcinoma cells
Received
1st November 2019
, Accepted 20th January 2020
First published on 3rd February 2020
Abstract
The anticancer effects of kimchi prepared with different kinds of solar salts were evaluated in an in vitro cellular system using HT-29 human colon carcinoma cells. Four kinds of kimchi samples were prepared, using different solar salts: conventionally manufactured solar salt (CS), filtered sea water solar salt (FS), dehydrated solar salt by centrifuging (DS), and washed-dehydrated solar salt (WDS). Prepared kimchi samples were presented as CSK, FSK, DSK, and WDSK, respectively. The pH values, acidity, and sensory evaluation were determined after 3 week fermentation at 5 °C (pH 4.3), and WDSK exhibited the best fermented characteristics and taste among the 4 samples examined. In the HT-29 cell growth inhibitory activity assay, all 4 kimchi samples exert dose-dependent cell growth inhibition, with WDSK showing significant growth inhibition of HT-29 cells. mRNA and protein expression levels of apoptosis and cell cycle arrest related factors reveals that WDSK significantly increases the mRNA expression levels of Bax, Bim, caspases-3, caspases-9, and p21 as compared to other kimchi samples, at a concentration of 4.0 mg mL−1. In addition, WDSK treatment strongly decreases the Bcl-2 protein expression (on western blot) in HT-29 cells, as compared to the control group (no kimchi treatment) and significantly increases the protein expression levels of Bax, caspases-3, caspases-9, and p53. Inductively coupled plasma atomic emission spectrometry (ICP-OES) reveals that WDS possesses a different mineral composition when compared to the other three solar salts; notably, the lower Mg (9.3 g kg−1) and S (4.7 g kg−1) content of WDS may cause better taste, fermented characteristcs, and functionality of WDSK. These results indicate WDS to be the ideal solar salt for kimchi preparation, which enhances the taste due to its lower Mg and S contents, and increases the anticancer effects by exerting better pro-apoptosis and cell cycle arrest abilities in HT-29 cells.
Introduction
Kimchi is a traditional Korean fermented food dating back more than 2000 years, generally prepared with Baechu cabbage and radish, along with other ingredients such as green onion, red pepper powder, garlic, ginger, etc.1 In recent decades, the functionalities of kimchi have been confirmed by several laboratory studies, and its beneficial effects include anti-aging,2 anti-obesity,3 anti-diabetic,4 anti-oxidative,5 and anti-cancer.6,7 Kimchi fermentation is initiated by lactic acid bacteria, which produce acids, ethanol, mannitol, and CO2 that affect the kimchi characteristics (pH, acidity, and bacterial community) and change the typical flavor profile of kimchi.8,9 Several factors influence the kimchi fermentation and characteristics including temperature, raw materials, starters, and salt; especially, the type of salt used has a great influence on the fermentation of kimchi.10,11
Salt is an essential condiment in daily diets and plays a vital role in maintaining body homeostasis. It is also an important seasoning for fermented foods, in food taste amelioration, and food shelf extension.10 Depending on the manufacturing process, different salts differ in their NaCl and mineral composition, and are chiefly categorized into four types for kimchi fermentation: purified salt, rock salt, recrystallized salt, and solar salt. In Korea, purified salt is used most widely in the preparation of kimchi as it is cheap and safe, since it contains no other compound except NaCl. However, it has been reported that kimchi brined with purified salt shows poor functionalities and taste compared to solar salt brined kimchi.12 Han et al.6 reported that kimchi brined with solar salt without bittern exhibit higher anticancer effect on HT-29 cells and AGS human gastric adenocarcinoma cells, as compared to purified salt brined kimchi. Moreover, based on sensory evaluation results, the taste and flavor scores of purified salt brined kimchi is also lower than solar salt brined kimchi.10
Solar salt is defined as “crystalline material obtained from sea water by natural evaporation in salt fields”, and contains various minerals, including potassium (K), magnesium (Mg), calcium (Ca), iron (Fe), zinc (Zn), and sulfur (S).13 Numerous studies have reported that Korean solar salt improves the characteristics of kimchi (pH, acidity, sensory evaluation indexes, yeast counts, proportion of lactic acid bacteria, and total bacteria communities)14 and enhances kimchi functionalities, such as anti-cancer15 and anti-obesity effects.16 However, fresh solar salt contains higher amounts of Mg and S compared to aged solar salt,16 which imparts a bitter taste to solar salt and further influences the taste of brined kimchi. Fortunately, these inorganic ion contents (Mg and S) are reduced during the solar salt aging period, which subsequently ameliorates the bitter taste in solar salt and its brined kimchi.14 Therefore, natural aging methods are generally used to further enhance the taste, flavor, and functionality of solar salt. Studies have determined that 1-, 3-, and 4-years aged Korean solar salt brined kimchi exhibit better fermentation characteristics, when compared to purified salt brined kimchi.10,11 These naturally aged solar salts (especially 3 years aged solar salt) impart lesser bitterness and have higher functionalities compared to conventionally manufactured solar salt (fresh solar salt), probably because of the lower contents of Mg and S.17 However, natural aging of solar salt takes a long time, and it is therefore necessary to develop and improve the processing method of solar salt to achieve taste and health functions similar to the natural aging solar salt. Hence, to identify the functional differences among natural aged solar salt and solar salt prepared by different processing methods, conventionally manufactured solar salt (CS), filtered sea water solar salt (FS), washed-dehydrated solar salt (WDS), and 3 years aged solar salt (3YS) were used to brine kimchi, and their anti-obesity effect was investigated. The study reported that WDS and 3YS brined kimchi have a better taste and significant anti-obesity effects, which may be due to decreased Mg content of WDS and 3YS.16 In a previous study at our laboratory, WDS exhibited similar or even higher anti-obesity effects than 3YS. However, the functionality of solar salt prepared by different processing methods requires further verification.
In the present research, kimchi samples were prepared using conventionally manufactured solar salt, filtered sea water solar salt, dehydrated solar salt, and washed-dehydrated solar salt, represented as CSK, FSK, DSK, and WDSK, respectively. This study aims to assess the anticancer effect of brined kimchi prepared using solar salts obtained from different processing methods. The anticancer efficacy was studied in HT-29 human colon carcinoma cancer cells through cell apoptosis and cell cycle arrest mechanisms. We further undertook to identify the possible mineral components in the solar salt that affects the anticancer activity of kimchi.
Materials and methods
Preparation of kimchi sample
In the present study, kimchi was prepared using Baechu cabbages and sub-ingredients, which were purchased from Bongurichan kimchi (Namyang-ju, Korea). Four kinds of kimchi sample were brined with 4 types of solar salts (general method manufactured solar salt, filtered sea water solar salt, dehydrated solar salt, and washed-dehydrated solar salt), respectively, which were prepared at Taepyeong salt (Shinan, Korea). Briefly, Baechu cabbages were washed and cut in halves, followed by overnight salting with salt using the dry salting method. The brined cabbages were then washed 3 times gently and drained for 3 hours. In the dry salting method, solar salt equivalent to 4% of the cabbage weight was treated on the side of the cabbage stem. Subsequently, the brined cabbages and premixed sub-ingredients were mixed, stuffed in containers and kept at 5 °C for 4 weeks to obtain fermented kimchi. For every 100 g brined cabbages, sub-ingredients components are as follows: 8% radish, 4% seaweed soup, 4% glutinous rice paste, 4% red pepper powder, 3.4% anchovy powder, 2% green onion, 2% crushed garlic, 0.8% fermented shrimp, and 0.4% crushed ginger. The final salinity of kimchi is 1.8 ± 0.1 (%).16
Measurement of kimchi pH and acidity
The pH and acidity values of kimchi samples were measured using a pH meter (M220, Corning, MA, USA) at room temperature. Following the AOAC standard test method,18 kimchi juice was diluted 20 times to measure the amount of 0.1 N NaOH (in mL) consumed by titrating up to pH 8.4. The appropriate value was calculated as the percentage of lactic acid content:
Kimchi sensory evaluation
The kimchi sensory evaluation was performed according to the replicated randomized complete block design.19 In total 15 trained sensory personnel were allowed to evaluate these samples and repeated three times. Based on the quantitative descriptive analysis method, subjective items such as overall acceptability, appearance, smell and texture, and objective items including salty flavor, bitter flavor, moldy flavor, refresh flavor, and sour flavor, were evaluated. Values ranging from 1 to 9 indicate extremely poor to excellent for subjective factors, and weak to strong for objective factors. After molar mastication of the kimchi samples, the sensory perception was assessed by mouth and nose sensations.
Preparation of kimchi extract
Kimchi was dried using a freeze dryer (FD 5512, Ilshin BioBase CO., Korea) and frozen at −80 °C when the pH value reached 4.0–4.3 (optimum fermented period) in the 3rd week. Dried kimchi was ground to a powder (40 g) and 100% methanol was added to stir for 24 hours (800 mL × 3 times). The extracted methanol and kimchi mixtures were filtered, and the collected solutions were further concentrated using a vacuum rotary evaporator (EYELA; Tokyo Rikakikai Co., Tokyo, Japan). Dimethyl sulfoxide (DMSO) solution was added to the concentrated kimchi methanol extract to prepare a stock solution at a final concentration of 250 mg mL−1.20
Cell culture
The HT-29 human colon carcinoma cell line was purchased from the Korean Cell Line Bank (KCLB, Seoul, Korea) and cultured in RPMI-1640 medium (Gibco-BRL, Gaithersburg, MD, USA) supplemented with 10% heat-inactivated fetal bovine serum (FBS) and 1% penicillin–streptomycin (PS) solution. The HT-29 cells were grown as monolayers in 75T easy culture flasks (Nunc, Roskilde, Denmark) in a CO2 incubator with 5% CO2 and 37 °C humidified environment, with medium change two or three times per week.21
3-(4,5-Dimethylthiazol-2-yl)-2,5-diphenyltetrazolium bromide (MTT) assay
To evaluate the inhibitory activity of kimchi samples on HT-29 cells, the MTT assay was performed with minor modification.22 Briefly, HT-29 cells (1 × 105 cells per well) were incubated with varying concentrations of kimchi samples (2, 3, 4, and 5 mg mL−1) in a 96-well plate for 48 h; 500 μg mL−1 MTT solution dissolved in phosphate buffered saline (PBS) was subsequently added to each well. The plate was then incubated for 4 h at the same conditions, followed by addition of 100 μL DMSO per well to dissolve the purple formazan crystals. The plate optical density was then measured using a Wallac Victor3 1420 Multilabel Counter (PerkinElmer, Wellesley, MA) at 540 nm.
qRT-PCR analysis
Quantitative reverse transcription polymerase chain reaction (qRT-PCR) was performed to determine the mRNA expression levels of cancer related genes in human colon carcinoma cells.23 Briefly, cultured HT-29 cells were plated in 6-well plates at a density of 1 × 106 cells per mL, as counted by a cell counter (Luna automated cell counter; Logos Biosystems, Gyunggi, Korea). After 24 h incubation, 4 mg mL−1 of kimchi extracts were added to the cell plate, and further incubated for 48 h under the same culture conditions. The cell total RNA was isolated using TRIzol reagent (Invitrogen, Carlsbad, CA, USA), followed by dissolving in 0.1% diethylpyrocarbonate (DEPC) water. The isolated total RNA was quantified using NanoDrop ND-1000 (NanoDrop Technologies Inc., Wilmington, DE). Subsequently, the total RNA was converted to cDNA using a thermal cycler, BioRad CFX-96 real-time system (BioRad, USA). The synthesized cDNA was subsequently amplified, and the amplified products were detected and measured by fluorescence using the FIREPol PCR Mix (Solis BioDyne, Tartu, Estonia).
The primers evaluated were purchased from Bioneer Corporation (Daejeon, Korea): 18s rRNA, forward 5′-CAG CCA CCC GAG ATT GAG CA-3′ and reverse 5′-TAG TAG CGA CGG GCG GTGT G-3′; Bax, forward 5′-TGC TTC AGG GTT TCA TCC AG-3′ and reverse 5′-GGC GGC AAT CAT CCT CTG-3′; Bim, forward 5′-AGA TCC CCG CTT TTC ATC TT-3′ and reverse 5′-TCT TGG GCG ATC CAT ATC TC-3′; Bcl-2, forward 5′-CAG CTG CAC CTG ACG CCC TT-3′ and reverse 5′-GCC TCC GTT ATC CTG GAT CC-3′; caspase-3, forward 5′-TTT TTC AGA GGG GAT CGT TG-3′ and reverse 5′-CGG CCT CCA CTG GTA TTT TA-3′; caspase-9, forward 5′-CTA GTT TGC CCA CAC CCA GT-3′ and reverse 5′-CTG CTC AAA GAT GTC GTC CA-3′; p21, forward 5′-ATG TCA GAA CCG GCT GGG G-3′ and reverse 5′-GCC GGG GCC CCG TGG GA-3′; p53, forward 5′-ATG GAG GAG CCG CAG TCA GA-3′ and reverse 5′-TGC AGG GGC CGC CGG TGT AG-3′.
Western blotting
Levels of cancer related proteins of HT-29 cells were investigated by subjecting the cells to western blotting analysis.21 Briefly, HT-29 cells were seeded in 6-well plate at a density of 1 × 106 cells per mL and incubated for 24 h. Cells were subsequently treated with the different kimchi extracts at a concentration of 4 mg mL−1 for 48 h, and total lysates were isolated using radioimmunoprecipitation (RIPA) assay buffer (Invitrogen, Carlsbad, CA, USA). The cell lysates were separated by sodium dodecyl sulfate-polyacrylamide gel electrophoresis, and transferred to polyvinylidene fluoride (PVDF, BioRad, USA) membranes. PVDF membranes were blocked with 5% skim milk for 1 hour at room temperature (to block non-specific proteins) followed by overnight hybridization with primary antibody at 4 °C. Probed membranes were then incubated with horseradish peroxidase-conjugated secondary antibody at room temperature, and chemiluminescent enhanced chemiluminescence assay kit was used to detect immunoreactive proteins. The western blot bands were quantified using the LAS-4000 luminescent image analyzer (Fujifilm Life Science, Tokyo, Japan).
Mineral composition analysis of solar salts (ICP-OES)
An inductively coupled plasma atomic emission spectrometer (ICP-OES, Optima 8300, PerkinElmer, Waltham, MA, USA) was used for mineral analysis. The mineral measurement conditions were adjusted according to the concentration of the sample and the condition of the equipment.24 The operating power was 1.5 kW, nebulizer used was mira mist, plasma gas flow was 12.0 L min−1, auxiliary gas flow was 0.2 L min−1, and nebulizer gas flow was 0.55 L min−1.
Statistical analysis
In order to assess the significance of the experimental results obtained from the control group and each sample in the present study, Duncan's multiple range test was performed at the level of p < 0.05 after ANOVA, and the results are expressed as mean ± standard deviation (SD). Western blotting and qPCR results are expressed as mean ± standard error (SE). All statistical analysis were processed using the SPSS (v23.0, SPSS Inc., Chicago, IL, USA) statistical program. In addition, significance is determined at p value less than 5%.25
Results
Physicochemical characteristics of kimchi
Physicochemical characteristics of kimchi samples were determined by analyzing the pH, acidity and sensory attributes. The major components of kimchi are decomposed and re-synthesized to produce various organic acids during the fermentation process, which impart the characteristics to kimchi. pH and acidity values are the main fermentation quality indicators that indicate the mature stage of kimchi. As shown in Fig. 1, the pH of all kimchi samples decrease with progressing fermentation. The pH obtained at 0 week for the various fermented kimchi were: CSK 6.44 ± 0.01, FSK 6.34 ± 0.01, DSK 6.29 ± 0.06, and WDSK 6.27 ± 0.01, whereas the pH values acquired at the end of the 3 week fermentation were 4.52 ± 0.03, 4.30 ± 0.03, 4.29 ± 0.01, and 4.20 ± 0.02, respectively. Generally, the kimchi taste better when the pH is in the range of 4.2–4.3.1 Moreover, the acidity values of CSK, FSK, DSK, and WDSK obtained at the 0 week fermentation were 0.23 ± 0.03, 0.26 ± 0.01, 0.26 ± 0.03, and 0.28 ± 0.01%, respectively, which increased to 0.85 ± 0.05, 0.81 ± 0.03, 0.81 ± 0.01, and 0.84 ± 0.02%, respectively, at the 3 week fermentation period. According to the codex standard for kimchi,26 the acidity value of kimchi at the optimally ripened stage is 0.4–0.9%.
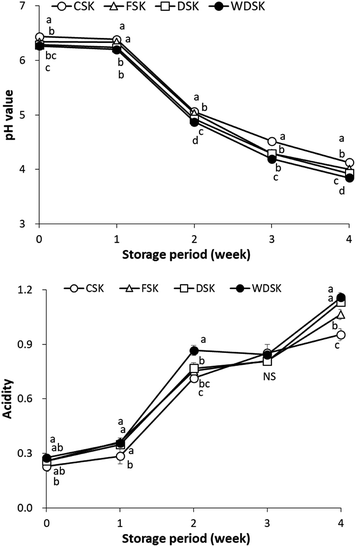 |
| Fig. 1 Changes of pH and acidity in kimchi brined with a variety of salts during fermentation at 5 °C for 4 weeks. CSK: conventional manufactured solar salt kimchi; FSK: filtrated solar salt kimchi; DSK: dehydrated solar salt kimchi; WDSK: wash and dehydrated solar salt kimchi. (a–d) Different letters at the same storage period indicate statistically significant difference (p < 0.05) by Duncan's multiple range tests. NS: no significance. | |
Sensory evaluation of the 3 week fermented CSK, FSK, DSK, and WDSK kimchi was performed using the 9-point scale method (Fig. 2). The main component of Baechu cabbage (such as pectin) decomposes and dissolves the water-soluble vitamins, sugars, sulfur-containing substances, free amino acids, etc., and the taste imparted to kimchi is related to the presence of these substances. In terms of appearance, WDSK scored the highest (6.82 ± 1.20 point), followed by DSK (5.71 ± 1.16 point), FSK (4.94 ± 1.13 point), and CSK (4.12 ± 1.02 point). Although all kimchi samples obtained appropriate scores for sour smell, green smell, mold smell, mold flavor, and hardness, WDSK had the lowest score for bitter flavor, which may be due to the lower Mg content of WDS. In the overall acceptability (comprehensive evaluation), WDSK obtained the highest score (5.65 ± 0.09), followed by DSK (5.24 ± 1.71), FSK (4.94 ± 1.27), and CSK (4.71 ± 1.26). Based on the sensory evaluation scores, WDSK would undoubtedly be more popular among consumers; hence, we propose that WDS is the better selection for brining kimchi, under the same cabbage raw materials, seasoning and fermentation conditions.
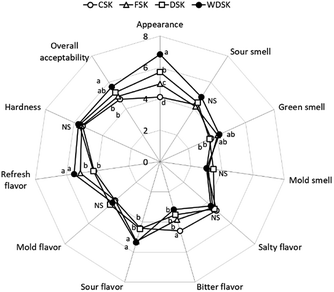 |
| Fig. 2 Sensory evaluation of 4 kinds of kimchi fermented at 5 °C for 4 weeks. CSK: conventional manufactured solar salt kimchi; FSK: filtrated solar salt kimchi; DSK: dehydrated solar salt kimchi; WDSK: wash and dehydrated solar salt kimchi. (a–d) Different letters at the same storage period indicate statistically significant difference (p < 0.05) by Duncan's multiple range tests. NS: no significance. | |
Antiproliferative effect of kimchi (MTT assay)
To assess the antiproliferative effect of kimchi, MTT assay was applied to measure the growth rate of HT-29 human colon carcinoma cells. As shown in Fig. 3, the antiproliferative effect of all kimchi extracts increased in a dose-dependent manner, but WDSK exhibited significant cell growth inhibition at all tested concentrations (2–5 mg mL−1), compared to other groups. There are obvious differences of cell growth inhibition observed between CSK, FSK, DSK, and WDSK at 4 mg mL−1, which demonstrate cell inhibition rates of 19.85 ± 2.03, 24.03 ± 1.21, 28.81 ± 1.40, and 34.94 ± 0.50%, respectively, thus 4 mg mL−1 concentration was selected to perform further studies. Taken together, the results indicate that CSK, FSK, DSK, and WDSK inhibit the growth of HT-29 colon carcinoma cells, and might possess anticancer properties.
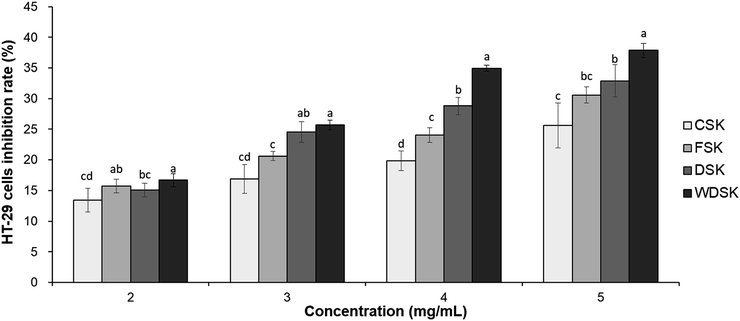 |
| Fig. 3 Analysis of cell growth inhibition rate in HT-29 human colon carcinoma cells. CSK: cells treated with conventional manufactured solar salt kimchi extract; FSK: cells treated with filtrated solar salt kimchi extract; DSK: cells treated with dehydrated solar salt kimchi extract; WDSK: cells treated with wash and dehydrated solar salt kimchi extract. (a–d) Means the different letters above the bars are significantly different (p < 0.05) by Duncan's multiple range test. | |
Gene expression analysis of apoptosis related factors in HT-29 cells
To investigate the anticancer effect of kimchi samples, expression levels of cell apoptosis related factors were measured in HT-29 cells via qRT-PCR and western blot assay. As a process of programmed cell death, apoptosis is considered a vital component of various processes, and the defects in apoptotic cell death regulation contribute to several diseases.27,28 In the present study, we measured the cell apoptosis related gene expression levels, viz., Bax, Bim, Bcl-2, caspase-3, and caspase-9. As presented in Fig. 4 and 5, the mRNA expression levels of Bax, Bim, Caspase-3, and Caspase-9 increased significantly with WDSK treatment as compared to control group. Levels of Bim expression significantly increased 3-fold, and Bcl-2 mRNA expression was reduced in WDSK, compared to the control group. Furthermore, the Bax, Bcl-2, Caspase-3, and Caspase-9 protein expressions were consistent with their mRNA expression levels which exhibited obvious differences between WDSK and control groups. The present qPCR and western blot results of apoptosis related genes indicate that WDSK exerts excellent pro-apoptosis activity and possibly contributes to the anticancer effect.
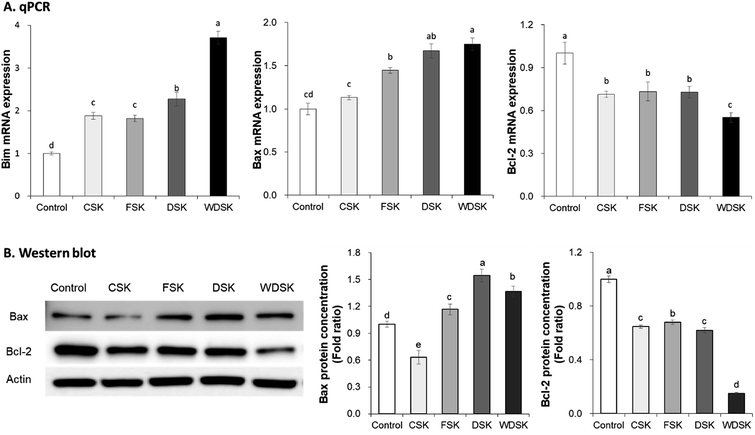 |
| Fig. 4 mRNA (A) and protein (B) expression levels of apoptosis related genes in HT-29 human colon carcinoma cells. Control: cells treated with media; CSK: cells treated with conventional manufactured solar salt kimchi extract; FSK: cells treated with filtrated solar salt kimchi extract; DSK: cells treated with dehydrated solar salt kimchi extract; WDSK: cells treated with wash and dehydrated solar salt kimchi extract. (a–e) Means with the different letters above the bars are significantly different (p < 0.05) by Duncan's multiple range tests. | |
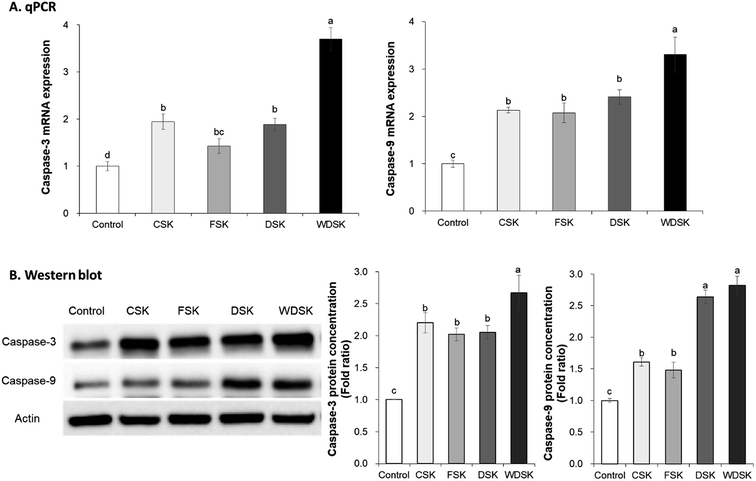 |
| Fig. 5 mRNA (A) and protein (B) expression levels of apoptosis related genes in HT-29 human colon carcinoma cells. Control: cells treated with media; CSK: cells treated with conventional manufactured solar salt kimchi extract; FSK: cells treated with filtrated solar salt kimchi extract; DSK: cells treated with dehydrated solar salt kimchi extract; WDSK: cells treated with wash and dehydrated solar salt kimchi extract. (a–e) Means with the different above the bars are significantly different (p < 0.05) by Duncan's multiple range tests. | |
Gene expression analysis of cell cycle arrest related factors in HT-29 cells
The standard cell cycle comprises the G1, S, G2, and M phases. The preparation of DNA synthesis occurs in the G1 phase, and its replication occurs in the S phase. Numerous studies have established that the tumor suppressor gene (p53) and cyclin-dependent kinase inhibitor (p21) are cell cycle inhibitors, which contribute to tumor cell growth inhibiton.29,30 Therefore, mRNA and protein expression levels of p53 and p21 in HT-29 cells were evaluated in this study. As shown in Fig. 6, p53 mRNA expression levels were doubled in the WDSK group as compared to control group, followed by DSK fermentation. Similarly, the protein expression of p53 was also significantly increased by WDSK, which is consistent with qRT-PCR result. Additionally, El-Deiry et al.31 reported that the p21 mRNA is highly induced after p53 activation, which is similar to results obtained in our present study. HT-29 cells exhibit significantly increased p21 mRNA expression after treatment with WDSK, followed by DSK, CSK, and FSK. Taken together, these results indicate that WDSK has potential activity on cell cycle arrest and contributes to HT-29 colon carcinoma cells growth inhibition.
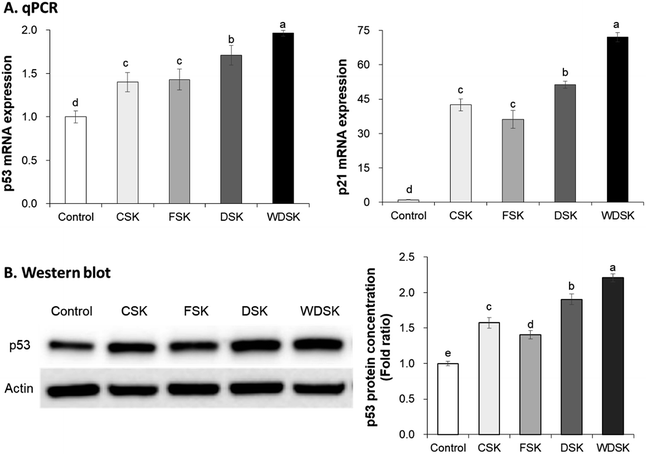 |
| Fig. 6 mRNA (A) and protein (B) expression levels of cell cycle arrest related genes in HT-29 human colon carcinoma cells. Control: cells treated with media; CSK: cells treated with conventional manufactured solar salt kimchi extract; FSK: cells treated with filtrated solar salt kimchi extract; DSK: cells treated with dehydrated solar salt kimchi extract; WDSK: cells treated with wash and dehydrated solar salt kimchi extract. (a–e) Means with the different letters above the bars are significantly different (p < 0.05) by Duncan's multiple range tests. | |
Solar salt mineral composition analysis (ICP-OES)
Mineral component determination of solar salts used for brining kimchi in the present study was performed by ICP-OES. Totally, four kinds of solar salt were evaluated: conventionally manufactured solar salt (CS), filtered sea water solar salt (FS), dehydrated solar salt (DS), and washed-dehydrated solar salt (WDS). As presented in Table 1, based on the different processing methods of solar salt, the mineral contents of kimchi were also different. Although there were no significant differences in the mineral composition between CS and FS for Na, Mg, S, Ca, and Zn, the DS and WDS extracts contained higher Na contents (360.3 ± 1.0 g kg−1 and 372.7 ± 0.5 g kg−1, respectively), compared to the Na content of 325.3 ± 0.8 g kg−1 in CS and 324.3 ± 0.9 g kg−1 in FS. In addition, Mg content of DS and WDS were significantly reduced (9.3 ± 0.0 and 5.8 ± 0.0 g kg−1, respectively) compared to Mg content of CS (17.8 ± 0.1 g kg−1) and FS (17.8 ± 0.2 g kg−1). Moreover, the lowest content of S was found in DS (2.9 ± 0.0 g kg−1), followed by WDS (4.7 ± 0.0 g kg−1), which were 2-fold less as compared to CS (6.8 ± 0.0 g kg−1) and FS (7.2 ± 0.0 g kg−1).
Table 1 Mineral composition of different solar saltsa,b
|
CS |
FS |
DS |
WDS |
CS: conventional manufactured solar salt; FS: filtrated solar salt; DS: dehydrated solar salt; WDS: wash and dehydrated solar salt. a–dMeans with the different letters in each column are significantly different (p < 0.05) by Duncan's multiple range tests. |
Na (g kg−1) |
325.3 ± 0.8c |
324.5 ± 0.9c |
372.7 ± 0.5a |
360.3 ± 1.0b |
Mg (g kg−1) |
17.7 ± 0.1a |
18.0 ± 0.2a |
5.8 ± 0.0c |
9.3 ± 0.0b |
S (g kg−1) |
6.8 ± 0.0b |
7.2 ± 0.0a |
2.9 ± 0.0d |
4.7 ± 0.0c |
K (g kg−1) |
4.1 ± 0.0b |
5.0 ± 0.0a |
1.5 ± 0.0d |
2.4 ± 0.0c |
Ca (g kg−1) |
3.9 ± 0.0a |
4.0 ± 0.0a |
2.3 ± 0.0c |
3.1 ± 0.0b |
Fe (mg kg−1) |
0.5 ± 0.0d |
1.3 ± 0.0b |
0.9 ± 0.0c |
1.4 ± 0.1a |
Zn (mg kg−1) |
0.1 ± 0.0a |
0.1 ± 0.0b |
0.1 ± 0.0a |
0.1 ± 0.0c |
P (mg kg−1) |
Not detected |
Not detected |
Not detected |
Not detected |
Cu (mg kg−1) |
Not detected |
Not detected |
Not detected |
Not detected |
Discussion
In the present study, four types of solar salts made from distinct processing methods were used to brine kimchi samples, which displayed different anticancer activities in HT-29 human colon carcinoma cells. Kimchi is a fermented health food having probiotic, prebiotic, and biogenic (fermentation products) properties. The fermentation conditions are affected by sub-ingredients, fermented temperature, salt types, and salt concentration, and these conditions directly affect its edible value.14 The use of salt in kimchi first kills the spoilage bacteria and acts as a starter by utilizing lactic acid bacteria.32 It was reported that different salt treatments result in the changes of bacterial profiles, which in turn lead to changes in kimchi metabolites associated with sensory and nutritional qualities.33 Various bioactive compounds have been found in kimchi, such as isothiocyanates, indole-3-carbinol, allyl sulfur compounds, beta-sitosterol, ascorbic acid, carotenoids, flavonoids, tocopherol, selenium, dietary fibers, polyunsaturated fatty acids, etc.1 In the past, although solar salts were used for brining, the resultant kimchi had a bitterness due to the high content of Mg contained in the solar salt. Thus, the 3 years aged solar salt was prepared via natural dehydration and applied to make kimchi. In recent years, only 50% kimchi is brined at home, while the rest is produced and sold by the kimchi factory. Considering the hygiene of microorganisms and the low price, purified salt is preferred for production. However, not only is the kimchi taste sub-standard, but with increasing fermentation times, the cabbage becomes softer with increased yeast growth.6 Furthermore, Lee et al. (2018) have reported that yeasts are detected from purified salt brined kimchi at 10 weeks but not detected until 18 weeks from solar salt brined kimchi.10 This result prove that solar salt helps to inhibit the growth of yeast during kimchi fermentation, thereby improving the taste of kimchi. Considering the poor quality, kimchi factories started using solar salts, and the 3 years aged solar salt possesses the best ferment performance. However, executing natural dehydration for up to 3 years is unfavorable with respect to space, price, time consuming, etc. Salt field washes the impurities of the solar salt from the saturated salt, and is dehydrated to produce the eluting salt of the processed solar salt. During the dehydration process, Mg and S are naturally eluted with water, which makes the taste and functionality of the solar salt better. This study therefore investigated whether the use of WDS in the production of kimchi can remove bitterness and increase the anticancer effect for healthy functionality.
Lactic acid bacteria (LAB) produce organic acids, ethanol, and carbon dioxide during the fermentation process of kimchi, which closely affects the pH and acidity, and finally the taste, nutritional values, and functionality of kimchi.9 As the fermentation time increases, LAB dominate the fermentation, and an increase in the acid production results in a decreased pH and elevated acidity values. In turn, the environment with low pH and high acidity further promotes the growth of lactic acid bacteria, and at the same time inhibits spoilage bacteria, which is conducive to the fermentation and functional improvement of kimchi.10 Our present study reveals that WDS fermented kimchi exerts a better fermentation effect, which can be seen from the fermentation characteristics (pH and acidity).
Especially in the sensory evaluation, WDS enhances the comprehensive taste of kimchi, and the bitterness was also reduced to an acceptable range. We determined that WDS has a similar effect as the 3 year aged solar salt.16
Several studies have identified the HT-29 cells inhibition activity of kimchi via MTT assay. It has been reported that chitosan-added kimchi exhibits a high anti-proliferative effect against HT-29 cells.21 Moreover, Kim et al.7 reported the anti-proliferative effect of kimchi fermented for different times, on HT-29 cells. Similar to these reports, our present results indicate that kimchi exerts its anticancer effects via inhibition of HT-29 cell growth.
The Bcl-2 family proteins, located predominantly on the mitochondria, play critical roles in cell apoptosis. Depending on their distinct functions, these proteins are categorized into anti-apoptotic proteins and pro-apoptotic proteins. The pro-apoptotic proteins are further to divided into Bax/Bak-like proteins (including Bak, Bax, Bcl-xs, and Bok) and BH-3 only proteins (such as Bim, Bad, and Bid).34 It is reported that cell death stimulus activates the BH-3 only proteins, which initiate Bax/Bak-like proteins and suppress the pro-survival proteins, although anti-apoptotic proteins can also promote cell survival; the BH-3 only proteins and pro-apoptosis Bax/Bak-like proteins inhibit the anti-apoptotic proteins, ultimately leading to cell apoptosis.35 In addition, a recent study indicated that Korean solar salt exerts its anticancer effects through regulation of the apoptosis related factors (namely, Bax and Bcl-2 expression levels) in the AOM/DSS-induced C57BL/6 mouse model.25 Furthermore, it is reported that bittern-removed natural solar salt and baked salt are a better choice to improve kimchi quality and anticancer effect, suggesting that salt varieties are genuinely important for kimchi preparation.6 Our present results reveal the cancer cell growth inhibitory effects of kimchi samples prepared with CS, FS, DS, and WDS, and strongly suggest that WDSK significantly suppresses the development of colon cancer by regulating the apoptotic factors (Bax, Bim, and Bcl-2).
According to the functions, mammalian caspases are generally separated into inflammatory caspases and apoptotic caspases, and apoptotic caspases are composed of initiator caspases (such as caspase-9) and effector caspases (such as caspase-3).36 A study indicated that death stimulus activates p53 and pro-apoptotic proteins (Bax and Bak), which lead to the activation of apoptosome; the activated apoptosome further actives caspase-9 and caspase-3, and finally the activated caspase-9 and caspase-3 induce cell apoptosis.37 Kim et al.7 also reported that optimally-ripened anticancer kimchi increased the expressions of caspase-3 and caspase-9 to promote cell apoptosis in HT-29 cells. Similarly, our present study demonstrates that DSK and WDSK considerably improve the expression levels of caspase-9, caspase-3, and Bax in HT-29 cells as compared to the control group, thereby indicating that the varying extent of anticancer activity of these kimchi samples may be due to the different solar salts, with WDS exhibiting the best functional ability among the four tested Korean solar salts.
The tumor suppressor gene, p53, is involved in multiple signaling pathways and cellular process regulations, including cell cycle arrest, antioxidant response, DNA repair, and apoptosis.38,39 The cyclin-dependent kinase inhibitor, p21, suppresses the synthesis of DNA through binding to and inhibiting the proliferating cell nuclear antigen.29 Additionally, p21 also correlates highly with p53 levels. Waldman et al.40 have reported that p21 is required for the p53-mediated G1 arrest in human cancer cells. Previous reports state that Korean solar salt and 9-time-baked bamboo salt exhibit anti-cancer activity by promoting the colon mRNA expression of p21 in AOM/DSS colon cancer mouse.25 Furthermore, Kim et al.41 demonstrated that kimchi treatment increases the mRNA and protein levels of p53 and p21 in colon tissues of AOM/DSS induced colon cancer mouse. Similarly, the present study results reveal that WDSK greatly increases the expression level of p21 with simultaneous increase in p53 levels, and the highest p53 protein expression level was observed in WDSK treated group. Therefore, our present study indicates that kimchi prepared with Korean solar salt (especially WDS) inhibits carcinogenesis by increasing the cell cycle arrest relative factors, p21 and p53.
To further identify the possible reason why solar salts exert different bioactivity, the mineral composition analysis was performed using ICP-OES. According to previous reports, Korea solar salts contain considerable amounts of minerals (such as Mg, K, and Ca) compared to general salt.42,43 Additionally, Chang et al.14 demonstrated that Na, Mg, K, and Ca are the main cations in 1 year aged and 4 years aged Korean solar salts, suggesting that Mg, K, and Ca are the major minerals involved in Korean solar salts. Furthermore, Kim et al.33 demonstrated that mineral-rich sea salt treatment had positively affected the changes of the kimchi bacterial growth, and metabolite profiles, which are linked to kimchi quality. Moreover, a previous study indicated that kimchi prepared with natural sea salt without bittern (NS-B) and baked salt (G) display better taste, color and overall acceptability, as compared to purified salt and natural sea salt brined kimchi. NS-B and G kimchi also significantly inhibit the growth of AGS and HT-29 cells compared to other kimchi samples, suggesting that salt varieties and salt processing method are very important for kimchi preparation.6
Considering these researches, we can reasonably infer that the different activities of kimchi are affected by their mineral composition due to different solar salts used, and the difference in mineral composition of various solar salts may be due to the marine environment, production and processing methods. To improve the functional value of kimchi, the present study therefore focused on the amelioration of solar salt processing method used in kimchi preparation. The ICP-OES data demonstrates that the WDS Mg and S contents were 3-times lower than in CS and FS. Studies evaluating the numerous mineral contents of solar salts report that Mg and K impart a bitter taste,14 although their concentrations reduce as the salt matures.13 However, the natural ripening of solar salt requires a lot of time, which increases the production cost of the factory. Our present study used solar salts produced by conventionally manufactured, filtered, dehydrated, and washed-dehydrated methods, respectively. Our data therefore demonstrates that kimchi prepared with WDS exhibits significant in vitro anticancer effect compared to the other three kimchi samples, thereby strongly suggesting that WDS significantly increases the anticancer activity of kimchi. Moreover, the mineral composition analysis (Table 1) indicated that the Mg and S contents of the four types of solar salt are significantly different depending on the processing methods. The Mg and S contents in WDS are reduced by 47.5% and 30.9%, DS reduced by 67.2% and 37.4%, respectively, compared with those in CS. The Mg and S contents of WDS are at intermediate levels. Meanwhile, our previous study (data not shown) revealed that solar salts exhibit higher anti-obesity effect in vitro and in vivo when the Mg content is maintained at an optimal level, which showed a bell type curve. In view of the above, the Mg content in solar salt should not be at a minimal level, but should be maintained at an appropriate level. As a result, kimchi taste and healthy functionality can be improved, depending on the type of salt used in kimchi fermentation. Compared with too low or too high Mg content in the salt, the proper concentration enhances the functionality and anti-obesity effects. Depending on our researches, we may recommend that the effective Mg contents ranged at 7.6–9.3 g kg−1 and S contents ranged at 4.2–4.7 g kg−1. Kimchi is a health food accepted worldwide, its taste, lactic acid bacteria, and fermentation products are influenced by various factors on the fermentation of kimchi, especially the solar salt used.
Conclusions
In summary, our present study evaluated the anticancer effect of kimchi in HT-29 human colon carcinoma cells, prepared with various solar salts. Our results reveal significant cancer cell growth inhibitory effect of WDSK, indicating that appropriate Mg and S content of WDS may contribute to the anticancer effect of WDSK, which further suggests that salt varieties play an important role in kimchi fermentation and its functionalities, such as anticancer function enhancement.
Conflicts of interest
The authors declare that there are no conflicts to declare.
Acknowledgements
This study was supported by the support project of the Fisheries Research Center of the Ministry of Maritime Affairs and Fisheries (National Institute of Mokpo University, Gyeongsang National University, Project (No. 20130290)).
References
- K. Y. Park and J. H. Ju, in Korean Functional Foods: Composition, Processing and Health Benefits, ed. K. Y. Park, D. Y. Kwon, K. W. Lee and S. M. Park, CRC Press, Florida, 1st edn, 2018, ch. 3, pp. 43–73 Search PubMed.
- B. Kim, K. Y. Park, H. Y. Kim, S. C. Ahn and E. J. Cho, Food Sci. Biotechnol., 2011, 20, 643–649 CrossRef.
- K. H. Lee, J. L. Song, E. S. Park, J. Ju, H. Y. Kim and K. Y. Park, Prev. Nutr. Food Sci., 2015, 20, 298 CrossRef CAS PubMed.
- M. S. Islam and H. Choi, J. Med. Food, 2009, 12, 292–297 CrossRef CAS PubMed.
- B. K. Kim, J. M. Choi, S. A. Kang, K. Y. Park and E. J. Cho, Nutr. Res. Pract., 2014, 8, 638–643 CrossRef CAS PubMed.
- G. J. Han, A. Son, S. M. Lee, J. K. Jung, S. H. Kim and K. Y. Park, J. Korean Soc. Food Sci. Nutr., 2009, 38, 996–1002 CrossRef.
- B. Kim, J. L. Song, J. H. Ju, S. A. Kang and K. Y. Park, Food Sci. Biotechnol., 2015, 24, 629–633 CrossRef CAS.
- J. Y. Yun, J. K. Jeong, S. H. Moon and K. Y. Park, J. Korean Soc. Food Sci. Nutr., 2014, 43, 1081–1087 CrossRef.
- Y. Hong, J. Microbiol. Biotechnol., 2013, 23, 76–84 CrossRef CAS PubMed.
- K. W. Lee, J. M. Shim, D. W. Kim, Z. Yao, J. A. Kim, H. J. Kim and J. H. Kim, Food Sci. Biotechnol., 2018, 27, 489–498 CAS.
- T. I. Mheen, Japanese Journal of Lactic Acid Bacteria, 2003, 14, 56–71 CrossRef.
- J. Ju, J. L. Song, E. S. Park, M. S. Do and K. Y. Park, Nutr. Res. Pract., 2016, 10, 629–634 CrossRef CAS PubMed.
- J. O. Ha and K. Y. Park, J. Korean Soc. Food Sci. Nutr., 1998, 27, 413–418 CAS.
- J. Y. Chang, I. C. Kim and H. C. Chang, Korean Journal of Food Preservation, 2011, 18, 256–265 CrossRef.
- X. Zhao, S. H. Kim, Y. Qi, S. Y. Kim and K. Y. Park, J. Korean Soc. Food Sci. Nutr., 2012, 41, 26–32 CrossRef CAS.
- E. S. Park, S. M. Lee and K. Y. Park, J. Korean Soc. Food Sci. Nutr., 2018, 47, 229–234 CrossRef.
- E. S. Park, S. M. Lee and K. Y. Park, Tang Humanitas Medicine, 2018, 8, 1–6 CAS.
- AOAC, in Official methods of analysis: Association of official analytical chemistry, AOAC international, Washington D. C., 1995 Search PubMed.
- G. J. Han, H. S. Choi, S. M. Lee, E. J. Lee, S. E. Park and K. Y. Park, J. Korean Soc. Food Sci. Nutr., 2011, 40, 110–115 CrossRef.
- G. H. Song, E. S. Park, S. M. Lee, D. B. Park and K. Y. Park, J. Environ. Pathol., Toxicol. Oncol., 2018, 37, 151–161 CrossRef PubMed.
- C. S. Kong and K. Y. Park, J. Med. Food, 2010, 13, 6–12 CrossRef CAS PubMed.
- T. Mosmann, J. Immunol. Methods, 1983, 65, 55–63 CrossRef CAS PubMed.
- K. J. Livak and T. D. Schmittgen, Methods, 2001, 25, 402–408 CrossRef CAS PubMed.
- H. J. Ahn and H. S. Son, Korean J. Food Sci. Technol., 2012, 44, 280–286 CrossRef.
- J. Ju, Y. J. Kim, E. S. Park and K. Y. Park, Prev. Nutr. Food Sci., 2017, 22, 149–155 CAS.
- CODEX (Codex Alimentarius Commission), Codex standard for kimchi, Codex Stan 223, Food and Agriculture Organization of the United Nations, Rome, Italy, 2001 Search PubMed.
- J. C. Reed, Am. J. Pathol., 2000, 157, 1415–1430 CrossRef CAS PubMed.
- S. Elmore, Toxicol. Pathol., 2007, 35, 495–516 CrossRef CAS PubMed.
- K. Vermeulen, D. R. Van Bockstaele and Z. N. Berneman, Cell Proliferation, 2003, 36, 131–149 CrossRef CAS PubMed.
- M. Malumbres and M. Barbacid, Nat. Rev. Cancer, 2009, 9, 153 CrossRef CAS PubMed.
- W. S. El-Deiry, T. Tokino, V. E. Velculescu, D. B. Levy, R. Parsons, J. M. Trent, D. Lin, W. E. Mercer, K. W. Kinzler and B. Vogelstein, Cell, 1993, 75, 817–825 CrossRef CAS PubMed.
- K. Y. Park, J. K. Jeong, Y. E. Lee and J. W. Daily 3rd, J. Med. Food, 2014, 17, 6–20 CrossRef CAS PubMed.
- D. W. Kim, B. M. Kim, H. J. Lee, G. J. Jang, S. H. Song, J. I. Lee, S. B. Lee, J. M. Shim, K. W. Lee, J. H. Kim, K. S. Ham, F. Chen and H. J. Kim, J. Food Sci., 2017, 82, 1124–1131 CrossRef CAS PubMed.
- J. T. Opferman and A. Kothari, Cell Death Differ., 2018, 25, 37–45 CrossRef CAS PubMed.
- P. N. Kelly and A. Strasser, Cell Death Differ., 2011, 18, 1414–1424 CrossRef CAS PubMed.
- S. M. Man and T. D. Kanneganti, Nat. Rev. Immunol., 2016, 16, 7–21 CrossRef CAS PubMed.
- S. Shalini, L. Dorstyn, S. Dawar and S. Kumar, Cell Death Differ., 2015, 22, 526–539 CrossRef CAS PubMed.
- J. Chen, Cold Spring Harbor Perspect. Med., 2016, 6, a026104 CrossRef PubMed.
- D. Michael and M. Oren, Semin. Cancer Biol., 2003, 13, 49–58 CrossRef CAS PubMed.
- T. Waldman, K. W. Kinzler and B. Vogelstein, Cancer Res., 1995, 55, 5187–5190 CAS.
- H. Y. Kim, J. L. Song, H. K. Chang, S. A. Kang and K. Y. Park, J. Med. Food, 2014, 17, 833–841 CrossRef PubMed.
- T. C. Gao, J. Y. Cho, L. Y. Feng, S. Chanmuang, S. Y. Park, K. S. Ham, C. K. Auh and T. K. Pai, Food Sci. Biotechnol., 2014, 23, 951–956 CrossRef CAS.
- M. M. Tan, S. Cui, J. Yoo, S. H. Han, K. S. Ham, S. H. Nam and Y. Lee, Appl. Spectrosc., 2012, 66, 262–271 CrossRef CAS PubMed.
|
This journal is © The Royal Society of Chemistry 2020 |
Click here to see how this site uses Cookies. View our privacy policy here.