DOI:
10.1039/C9RA09999A
(Paper)
RSC Adv., 2020,
10, 7432-7442
Simultaneous removal of multiple heavy metals from soil by washing with citric acid and ferric chloride†
Received
29th November 2019
, Accepted 29th January 2020
First published on 19th February 2020
Abstract
The remediation of soil contaminated with multiple heavy metals is a matter of great concern due to its serious threat to the ecosystem and human health. Batch and slurry reactor soil washing experiments were conducted to explore the removal of Cd, Cr, Pb and Zn using 7 agents. Citric acid (CA) and ferric chloride (FeCl3) exhibited an obvious synergistic effect on the removal of heavy metals. Furthermore, the concentration of heavy metals in different soil particle size fractions was closely related to the soil element concentrations. Fine sand (0.05–0.25 mm) had a strong adsorption capacity for Cr and Pb because of the high Mn concentration. Notably, heavy metals in smaller-size soil particles could be efficiently removed by CA and FeCl3. After remediation, the bioavailability of heavy metals in soil decreased. The potential ecological risk of heavy metals in soil reduced from an extremely high level to a low level. Moreover, some elements (e.g. Al, Mn and Fe) and organic matter in soil were dissolved by CA and FeCl3, which accelerated the desorption of heavy metals from the soil. In a slurry reactor experiment, the removal efficiencies of Cd, Cr, Pb and Zn were 94.8%, 79.5%, 92.7% and 97.2%, respectively. The combination of CA and FeCl3 is a feasible practice to remediate soil contaminated by multiple heavy metals.
1. Introduction
Soil contamination with heavy metals is one of the important environmental problems in the world. It is mainly derived from anthropogenic activities, including mining, electroplating, metallurgy and other industries.1,2 Multiple heavy metals (including Cd, Cr, Pb, Zn and Hg)3,4 represent a threat to the environment and human health due to their persistence in soil and potential uptake by crops.5 Therefore, the remediation of soil contaminated by heavy metals, particularly in combination with different pollutants, is of practical significance.6 Various techniques, including physical methods (soil replacement, thermal treatment), chemical methods (solidification/stabilization, soil washing) and biological methods (microbial remediation, phytoremediation) have been conducted for solving this environmental issue.7–11 Soil washing offers great advantages in soil remediation for its simple operation and permanent removal of heavy metals from soil.7,12
Various agents, including acids, bases, chelating agents, surfactants and other additives, have been employed to remove heavy metals from soil.13–15 Although acids could increase the solubility of metals, strong acids may destroy the soil structure, which is unsuitable for revegetation.16 Consequently, synthetic chelates, such as ethylenediamine tetraacetic acid (EDTA) and diethylene triamine pentaacetic acid (DTPA), have been used for soil remediation because of their strong chelating ability for different heavy metals.17 However, they have poor biodegradability and are unstable at high temperatures.18 In recent years, citric acid (CA), a tricarboxylic acid compound, has played an important role in removing heavy metals from soil via acid dissolution and chelation,19 and is more readily biodegradable than EDTA.20,21 Notably, CA leaches lower contaminants (e.g. Cd and Ni) than EDTA, except for Cr.22,23 Salt and chloride solutions (e.g. CaCl2 and FeCl3) are also promising washing agents, which have been verified to decrease heavy metal content, especially that of Cd, in soil and crops.24–26 FeCl3 can remove Cd through proton release and formation of soluble complexes with Cd.27 Different washing agents show affinity and selectivity for different heavy metals in soil. Studies have shown that the removal efficiencies of multiple heavy metals can be significantly improved by composite washing. The combination of ferric nitrate and EDDS was useful for the removal of Pb and Cu from soil.28 Combining washing reagents dramatically enhanced the removal efficiencies of Pb and Zn by using Na2EDTA and either tartaric acid or lactate.21 Hence, searching for efficient and environmentally friendly composite washing agents is a critical step for the remediation of co-contaminated soil.29
Batch and slurry reactor washing experiments were conducted to explore the removal of Cd, Cr, Pb and Zn from contaminated soil using 7 agents. The objectives of this study were as follows: (1) select efficient agents for soil remediation; (2) obtain the optimal conditions of washing with CA and FeCl3; (3) explore the concentrations and removal efficiencies of heavy metals in different soil particle-size fractions; (4) assess the pollution level and potential ecological risk of heavy metals in soil before and after remediation.
2. Materials and methods
2.1. Soil characteristics
Soil samples were collected from a demolished industrial site in Hangzhou City, Zhejiang Province, China. Samples were air-dried at room temperature, then ground and passed through a 2 mm sieve for analysis. The concentrations of Cd, Cr, Pb and Zn in the soil were determined by flame atomic absorption spectroscopy (FAAS, MKILM6) after triacid digestion (HNO3–HF–HClO4). The Cr(VI) concentration in soil was determined by the diphenylcarbazide spectrophotometric method30 after extraction with Na2CO3 and NaOH. The concentrations of Cd, Cr, Cr(VI), Pb and Zn were 646.7, 4802.0, 272.9, 9587.0 and 20
079.0 mg kg−1, respectively. The soil was neutral (pH 7.0 ± 0.1), the organic matter content was 8.7 ± 0.3% and the cation exchange capacity (CEC) of the soil was 14.5 ± 0.3 cmol kg−1. The soil was classified as sandy loam according to the USDA soil classification (6.8% clay, 33.6% silt and 59.6% sand).
2.2. Selection of promising agents for soil washing
In order to select efficient composite washing agents, the removal of heavy metals by washing with 7 agents (citric acid (CA), oxalic acid (OA), phosphoric acid (H3PO4), nitric acid (HNO3), ferrous chloride (FeCl2), ferric chloride (FeCl3) and disodium ethylenediaminetetraacetic acid (Na2EDTA)) was explored respectively in this study. The washing solution concentrations were 0.1, 0.5 and 1.0 mol L−1, respectively (washing time of 10 h, liquid
:
soil ratio of 10
:
1). Subsequently, CA, OA, HNO3, and FeCl3 (0.5 mol L−1) were combined in pairs for composite washing because of their relatively high removal efficiency. The volume ratios of agents were 8
:
2, 5
:
5 and 2
:
8, respectively. Each group was carried out at least in triplicate.
2.3. Determination of optimum washing conditions
Batch experiments were set to obtain the optimum washing conditions of CA and FeCl3 (v/v, 5
:
5). The explored washing solution concentrations were 0.25, 0.5, 0.75 and 1.0 mol L−1, respectively. Each group was carried out at least in triplicate. The optimum concentrations of agents would be used for further experiments. In addition, the optimum washing time and liquid
:
soil ratio were also investigated, with detailed experimental setups described in the ESI.†
Soil samples and washing agents were added into 50 mL centrifuge tubes, and mixed under 180 rpm at 25 °C. After washing, the tubes were centrifuged for 10 min at 5000g, and the supernatant was then filtered through a 0.45 μm membrane. The concentrations of Cd, Cr, Pb and Zn were determined by flame atomic absorption spectroscopy (FAAS, MKILM6).
2.4. Composite washing combined with sieving pretreatment
Silty clay (<0.05 mm), fine sand (0.05–0.25 mm) and coarse sand (0.25–2 mm) were separated by 300, 60 and 10 mesh sieves, respectively. The removal of heavy metals in different treatments was explored with a washing time of 10 h and liquid
:
soil ratio of 10
:
1. Each group was carried out at least in triplicate. The other experimental steps were the same as Section 2.3. After washing, the soil was washed three times with deionized water and then freeze-dried for further analysis. The morphology and element distribution of soil samples were observed via a scanning electron microscopy (SEM, SU-8010) and an energy dispersive spectrometer (EDS, X-MaxN 80), respectively.
2.5. Sequential extraction procedure
Sequential extraction (Tessier's method)31 was used to investigate the speciation of heavy metals in soil. In short, heavy metal species were categorized into 5 fractions: exchangeable fraction (F1, extractable with 1 mol L−1 MgCl2 at pH 7.0), bound to carbonates fraction (F2, extractable with 1 mol L−1 NaAc–HAc at pH 5.0), bound to Fe–Mn oxides fraction (F3, extractable with 0.04 mol L−1 NH2OH·HCl), bound to organic matter fraction (F4, extractable with 0.02 mol L−1 HNO3 and 30% H2O2 at pH 2.0) and residual fraction (F5, digestible with HNO3–HF–HClO4).
2.6. Slurry reactor experiment
The polymethyl methacrylate column used in this study was of a 25 cm inner diameter and 25 cm height. The details of the slurry reactor are shown in the ESI (Fig. S1†). Contaminated soil (1 kg) and washing agents (CA and FeCl3, optimal washing conditions) were added into the reactor. The stirring speed was 180 rpm, and the temperature was 25 °C. The sampling intervals and other experimental steps were the same as Section 2.3. After washing, the soil was washed three times with deionized water and then freeze-dried for analysis. Molecular spectra of soil were recorded in the 4000–400 cm−1 region on a Fourier transform infrared spectrometer (FTIR, AVATAR370).
2.7. Statistical analysis
The Cd, Cr, Pb and Zn concentration data were reported as the mean value ± standard deviation (SD) of at least three replicates for each treatment. One-way ANOVA was performed to assess the statistical significance (P < 0.05) between different treated groups by SPSS 20.0.32 The response surface methodology (RSM) based central composite design (CCD) was used to establish the response surface maps and the optimum agent concentrations by Design Expert 8.0.33
3. Results and discussion
3.1. Selection of washing agents
The selection of efficient washing agents is the key to co-contaminated soil remediation. The removal of Cd, Cr, Pb and Zn from soil by 7 washing agents was investigated in this study. The removal of heavy metals was positively related to the agent concentration, except for Na2EDTA (Fig. S2†). The removal efficiencies of heavy metal washing by 7 agents were as follows (agent concentration of 0.5 mol L−1): for Cd, FeCl3 ≈ CA ≈ HNO3 > H3PO4 > Na2EDTA ≈ FeCl2 > OA; for Cr, OA > CA > HNO3 > H3PO4 > FeCl3 > Na2EDTA > FeCl2; for Pb, FeCl3 ≈ Na2EDTA > FeCl2 > CA > HNO3 ≈ OA ≈ H3PO4; for Zn, FeCl3 ≈ HNO3 ≈ CA ≈ H3PO4 > FeCl2 > Na2EDTA > OA. FeCl3, CA, HNO3 and H3PO4 were efficient agents for the removal of Cd and Zn, and OA and CA were efficient agents for the removal of Cr from soil.
Notably, FeCl3 exhibited a high extraction efficiency for Cd, Pb and Zn (Table S1†). The removal efficiencies of Cd, Cr, Pb and Zn were 67.7%, 15.6%, 98.2% and 69.4%, respectively. Studies have shown that the hydrolysis of FeCl3 results in the release of protons and the synthesis of hydroxides.34 Furthermore, the stable and soluble metal chloro-complexes were formed with Cl− in the solution, which prevented heavy metals from being re-absorbed into the soil.12 Similarly, FeCl3 has also proven to be the most stable washing agent for Pb removal, and is cheap and easy to handle.25,35 In addition, CA exhibited a high extraction efficiency for heavy metals, especially Cr. The removal efficiencies of Cd, Cr, Pb and Zn were 78.4%, 44.4%, 51.5% and 81.2%, respectively. Furthermore, CA has been considered to be the best for the remediation of Cr(VI) contaminated wastes and soils,22,36 and was more biodegradable and less toxic.37
Finally, the combination of CA and FeCl3 (5
:
5, v/v) was chosen for simultaneously removing multiple heavy metals from soil. The removal efficiencies of Cd, Cr, Pb and Zn (Table S1†) were 73.1%, 41.3%, 98.0% and 71.4%, respectively.
3.2. Optimization of composite washing conditions
To determine the optimum washing conditions, the effects of agent concentration, washing time and liquid
:
soil ratio on the removal of heavy metals were investigated in this study. Generally, the removal of heavy metals is positively related to the concentration of the agent.38 Differently, there was an obvious quadratic parabolic correlation between the CA concentration and the removal efficiencies of Cd and Zn (Fig. 1A and D). The removal efficiencies of Cd were 87.9%, 95.7% and 87.4%, and the removal efficiencies of Zn were 91.6%, 97.3% and 91.0%, at the FeCl3 concentration of 0.75 mol L−1 and CA concentrations of 0.50, 0.75 and 1.00 mol L−1, respectively. It was illustrated that a high agent concentration may not be conducive to the ion exchange of Cd and Zn.39 In addition, the removal of Cr and Pb (Fig. 1B and C) was positively related to the concentrations of CA and FeCl3. The removal efficiencies of Cr and Pb were respectively 77.0% and 97.7% at the CA and FeCl3 concentrations of 1.00 mol L−1. CA and FeCl3 exhibited an obvious synergistic effect on the removal of heavy metals from soil. Subsequently, the response surface analysis40 determined that the optimum concentrations of CA and FeCl3 were 0.86 and 1.00 mol L−1 (Fig. S3†), respectively. The predictive removal efficiencies of Cd, Cr, Pb and Zn in the soil were 96.3%, 72.5%, 97.6% and 98.6%, respectively.
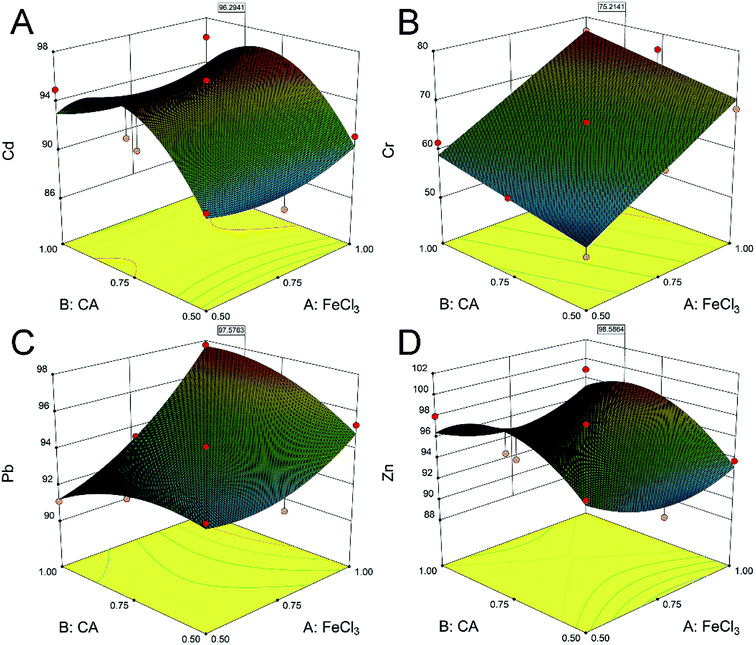 |
| Fig. 1 Response surface maps of the effects of different CA and FeCl3 concentrations on the removal efficiencies of Cd (A), Cr (B), Pb (C) and Zn (D) in contaminated soil. | |
Another crucial factor is the washing time. The removal of heavy metals proceeds until a dynamic equilibrium between adsorption and desorption is reached.32 At first, heavy metals on the soil surface were quickly desorbed into solution.41 The removal efficiencies of heavy metals increased dramatically over time (Fig. 2A) in the early stage (<2 h). The removal efficiencies of Cd, Cr, Pb and Zn after washing for 2 h were 93.2%, 48.9%, 92.9% and 86.8%, respectively. The desorption of heavy metals became slow and reached a plateau after washing for 10 h. Notably, the removal of heavy metals was unaltered, or even decreased over time (10–24 h). Therefore, the washing time was determined to be 10 h.
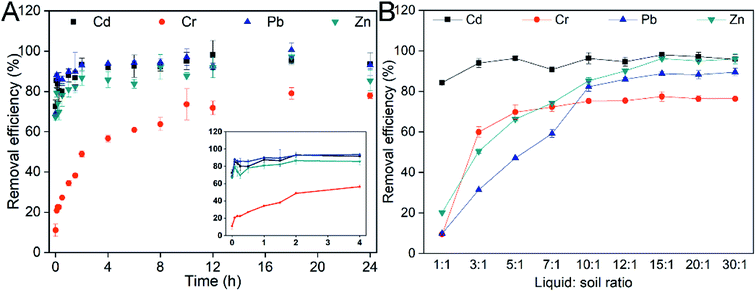 |
| Fig. 2 Removal efficiencies of Cd, Cr, Pb and Zn in contaminated soil over time (0–24 h) by washing with CA and FeCl3(A); removal efficiencies of Cd, Cr, Pb and Zn in contaminated soil at the first 4 hours (A, the inset graphic); effects of liquid : soil ratios on the removal efficiencies of heavy metals during composite washing (B). | |
The liquid
:
soil ratio is also an important factor affecting the desorption of heavy metals from soil.42 A lower concentration or higher liquid
:
soil ratio could avoid the clogging of soil but increase costs.43 In this study, there was no significant difference in Cd removal efficiency (94.2 ± 4.3%) under different liquid
:
soil ratio conditions (Fig. 2B). This indicated that Cd was more easily desorbed from soil, because Cd mostly exists as an exchangeable fraction and is very active in nature.44 The removal of Cr, Pb and Zn was increased dramatically as the liquid
:
soil ratio increased from 1
:
1 to 10
:
1. The removal efficiencies of Cr, Pb and Zn reached 75.2%, 88.8% and 96.2%, respectively. Notably, the removal of heavy metals was unaltered as the liquid soil ratio increased from 10
:
1 to 30
:
1. Hence, the optimal liquid
:
soil ratio was determined to be 10
:
1 considering the economics of soil remediation.
3.3. Removal of heavy metals from different particle-size fractions of soil
Soils are characterized by the distribution of different soil particle-size fractions.45 Generally, fine sand exhibits a higher affinity for heavy metals than that of coarse sand, due to its higher specific surface area and natural organic content.16 Here, coarse sand (0.25–2 mm), fine sand (0.05–0.25 mm) and silty clay (<0.05 mm) were separated by sieving. The results (Table 1) showed that Cd and Zn were more easily accumulated in the soil of small particle-size fractions. The concentrations of Cd and Zn in silty clay were increased by 11.3% and 55.6% of those in the coarse sand, respectively. Differently, the concentrations of Cr and Pb were highest in the fine sand, which were respectively increased by 15.0% and 90.5% of those in the coarse sand.
Table 1 Concentrations of Cd, Cr, Pb, Zn, Ca, Mg, Al, Fe and Mn in different soil particle-size fractionsa
Heavy metal |
Concentration of metals (mg kg−1) |
Original |
Coarse sand |
Fine sand |
Silty clay |
Different letters (a–d) behind the data indicate a significant difference among different particle-size fractions of soil (one-way ANOVA, P < 0.05). |
Cd |
646.7 ± 46.0ab |
629.3 ± 34.9b |
665.7 ± 4.3 ab |
700.6 ± 2.1a |
Cr |
4802 ± 161.1b |
4930 ± 67.7 ab |
5671 ± 46.3a |
4213 ± 94.2b |
Pb |
9587 ± 654.9c |
6884 ± 45.0d |
13 115 ± 26.7a |
10 625 ± 565.7b |
Zn |
20 079 ± 615.5b |
15 508 ± 596.5d |
18 967 ± 96.6c |
24 137 ± 219.9a |
Ca |
22 007 ± 565.7bc |
32 587 ± 1330.1a |
22 940 ± 433.0b |
21 105 ± 257.2c |
Mg |
3641.7 ± 85.6b |
3085.8 ± 69.7d |
3244.2 ± 27.7c |
4559.2 ± 65.1a |
Al |
41 775 ± 346.4c |
50 813 ± 1339.9a |
39 100 ± 229.1d |
45 450 ± 1039.5b |
Fe |
44 942 ± 1410.3b |
23 046 ± 1545.5d |
41 275 ± 125.0c |
60 950 ± 1477.3a |
Mn |
63 583 ± 1418.0b |
38 281 ± 1962.6c |
73 258 ± 262.6a |
62 550 ± 433.7b |
To explore the interaction between heavy metal concentrations and the element contents of soil, the concentrations of Ca, Mg, Al, Fe and Mn in different treatments were further investigated (Table 1). The correlation analysis showed that the distributions of Cd and Zn were closely related to the Fe and Mg concentrations (Fig. S4†), followed by the concentration of Mn. Additionally, the Pb concentration was related to the concentrations of Mn and Fe, which indicated that high contents of Fe and Mn oxides in soil could adsorb more Pb.46 Conversely, the distribution of Cr in soil showed an inverse correlation with the concentrations of Mg, Al and Fe, apart from Mn and Ca. Shehzad et al. also found that Cr was more likely to be adsorbed to soils with high Mn concentrations.47 Besides, Mn oxides were proven to be the only naturally occurring oxidant of Cr(III).48 Soil elements (e.g. Al, Mn and Fe) play an important role in the concentration of heavy metals in different treatments.
In addition, the combination of CA and FeCl3 exhibited an obvious synergistic effect on the removal of heavy metals, especially for Cr, in different treatments (Table 2). Notably, there was no significant difference in the removal of Cd and Zn in different soil particle-size fractions (Table 2). It was illustrated that Cd and Zn in smaller soil particle sizes could be also effectively removed by CA and FeCl3. However, the removal efficiencies of Cr and Pb in fine sand were lower than the other groups, which was consistent with the above results. It was elucidated that fine sand had a strong adsorption capacity for Cr and Pb.49 In short, heavy metals in smaller soil particle-size fractions could be removed efficiently by composite washing.
Table 2 Removal efficiencies of Cd, Cr, Pb and Zn in different soil particle-size fractions after washinga
Washing agent |
|
Removal efficiency (%) |
Cd |
Cr |
Pb |
Zn |
Different letters (a–d) behind the data indicate a significant difference among different soil particle size fractions (one-way ANOVA, P < 0.05). |
FeCl3 |
Original |
95.57 ± 1.06a |
23.68 ± 0.32b |
96.96 ± 1.26b |
89.87 ± 0.89b |
Coarse sand |
99.21 ± 2.31a |
19.62 ± 0.15c |
70.39 ± 3.05c |
96.17 ± 2.54a |
Fine sand |
93.75 ± 0.36a |
16.75 ± 0.38d |
63.76 ± 0.83d |
90.06 ± 0.27b |
Silty clay |
97.09 ± 0.71a |
27.13 ± 0.56a |
99.64 ± 0.07a |
87.19 ± 0.31b |
CA |
Original |
94.23 ± 2.98a |
68.24 ± 0.94a |
42.32 ± 0.62a |
95.44 ± 0.73bc |
Coarse sand |
96.23 ± 2.62a |
57.29 ± 2.74b |
44.69 ± 1.95a |
98.98 ± 2.66a |
Fine sand |
92.65 ± 1.06a |
46.66 ± 0.67c |
24.87 ± 0.41b |
98.10 ± 0.53ab |
Silty clay |
95.79 ± 0.83a |
68.73 ± 0.89a |
42.22 ± 0.28a |
93.93 ± 0.39c |
CA+ |
Original |
94.85 ± 3.08a |
75.48 ± 4.20a |
91.23 ± 0.84a |
94.74 ± 0.76a |
FeCl3 |
Coarse sand |
93.11 ± 1.58a |
74.39 ± 3.80ab |
77.71 ± 1.33b |
95.16 ± 2.60a |
Fine sand |
93.30 ± 1.53a |
65.37 ± 2.90b |
68.28 ± 1.12c |
92.50 ± 0.46a |
Silty clay |
85.87 ± 1.87a |
79.86 ± 0.72a |
94.83 ± 0.70a |
95.02 ± 0.73a |
3.4. Speciation of heavy metals in different soil particle-size fractions
Five chemical fractions of heavy metals were extracted by Tessier's method: exchangeable (F1), bound to carbonates (F2), bound to Fe–Mn oxides (F3), bound to organic matter (F4) and residual fractions (F5).50 Our results showed that the bound to carbonates (36.0–41.7%) and Fe–Mn oxides (30.4–39.2%) fractions were the main speciation options for Cd (Fig. 3A). A large amount of labile fractions (F1 + F2 + F3, 93.4–96.2%) in the soil resulted in the high removal of Cd from the soil.51 After remediation, most of the labile fraction of Cd in the soil was removed by CA and FeCl3, and the residual fraction was predominant in the soil (54.7–60.7%). Similarly, the bound to Fe–Mn oxides (49.3–54.7%) and carbonates (37.8–46.3%) fractions were the main speciation sources for Zn (Fig. 3D), which were removed effectively by washing with CA and FeCl3.
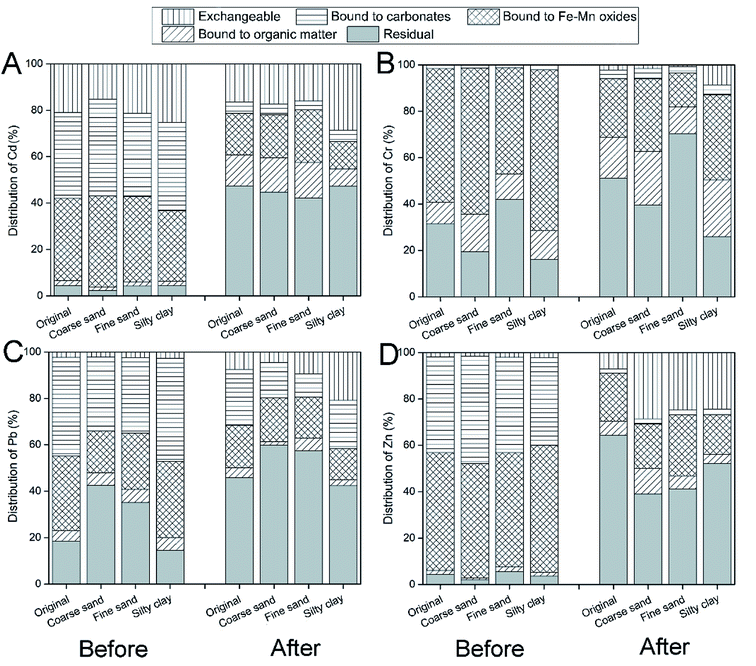 |
| Fig. 3 The speciation of Cd (A), Cr (B), Pb (C) and Zn (D) in different particle-size fractions of soil before and after washing. | |
In addition, the bound to Fe–Mn oxides fraction was the main Cr speciation option (45.8–69.4%), followed by the residual fraction (Fig. 3B). Notably, a large amount of the residual fraction existed in different soil particle-size fractions, especially in fine sand (42.0%), which resulted in the lower Cr removal efficiency.22 In addition, the bound to carbonates (31.7–44.4%) and Fe–Mn oxides (28.1–32.7%) fractions were the main speciation source of Pb (Fig. 3C). Carbonates and Fe–Mn oxides showed a high affinity to Pb in soil, which was consistent with the previous correlation analysis and studies.46 After washing, the labile fraction of Cr and Pb was removed significantly by CA and FeCl3, and the residual fraction was the main speciation source for heavy metals.
In summary, the labile fraction (F1 + F2 + F3) of heavy metals was removed effectively by composite washing, and residual fraction was predominant in soil after remediation. The bioavailability of Cd, Cr, Pb and Zn in soil was reduced by washing with CA and FeCl3.
3.5. Risk assessment of heavy metals in soil
The geo-accumulation index (Igeo) was used to evaluate the pollution level of heavy metals in contaminated soil. The calculation equation for Igeo index is presented as follows:
where Cn and Bn are the concentrations of heavy metals in samples and background soil, respectively. The factor 1.5 is applied as the background matrix correction value.52 The geochemical background concentrations of Cd, Cr, Pb and Zn in soil in Hangzhou City, Zhejiang Province, China are 0.11, 79.0, 26.5 and 83.3 mg kg−1, respectively.53
There was no significant difference in the Igeo index of Cd in different particle-size fractions of soil before washing (11.9–12.0) (Fig. 4A). The highest Igeo indexes of Cr and Pb respectively were 5.5 and 8.3 in the fine sand, which was consistent with the above results. Also, the Igeo index of Zn was increased with the decreasing soil particle size. The indexes of Zn in coarse sand, fine sand and silty clay were 7.0, 7.2 and 7.5, respectively. The soil was very strongly polluted by Cd, Cr, Pb and Zn (Igeo ≥ 5). After composite washing, the Igeo index of the heavy metals was decreased in all treatments, indicating that the polluted level of heavy metals in soil was reduced by CA and FeCl3. The polluted level of Cr and Zn was moderately to strongly polluted (3 > Igeo ≥ 2) after remediation.
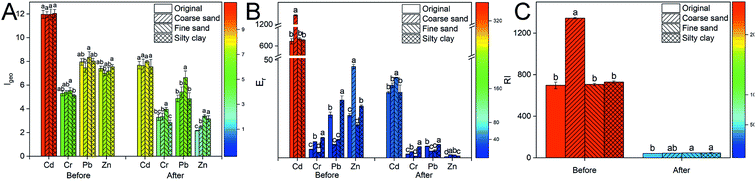 |
| Fig. 4 The geo-accumulation index (Igeo) (A), ecological risk coefficient (Er) (B) and potential ecological risk index (RI) (C) of heavy metals in soil in different treatments. Different letters (a–d) on the top of the error bars indicate a significant difference among different treatments (one-way ANOVA, P < 0.05). | |
The ecological risk coefficient (Er) and potential ecological risk index (RI) are used for the ecological risk evaluation of heavy metals in soil.54 The calculation equations for RI and Er are presented as follows:
where,
Cri is the contamination factor of heavy metals.
F1,
F2,
F3,
F4 and
F5 are the concentrations of heavy metals of different chemical fractions extracted by Tessier's method.
F1, exchangeable;
F2, bound to carbonates;
F3, bound to Fe–Mn oxides;
F4, bound to organic matter;
F5, residual.
Tri is the toxicity coefficient of heavy metals (Cd(30), Cr(2), Pb(5), and Zn(1)).
55
Before washing, the ecological risk coefficient (Er) of Cd (Fig. 4B) was extremely high in the coarse sand, which was related to the higher labile fractions of Cd in soil. The risk level of soil polluted by Cd was very high (Er ≥ 320) in all treatments. Similarly, the risk level of soil polluted by Zn was medium (80 > Er ≥ 40) in coarse sand, which was higher than that in the other groups. Although the Igeo index of heavy metals showed that the soil was very strongly polluted by Cr and Pb, the potential ecological risk level was low (40 > Er). After washing with CA and FeCl3, the potential ecological risk of soil polluted by heavy metals was reduced from an extremely high level (RI ≥ 600) to a low level (150 > RI) (Fig. 4C).
3.6. Surface morphology of soil particles
The surface morphology and elemental distribution of soil particles were further explored by SEM-EDS. Micro-aggregates were found on the soil surface, especially on the fine sand and silty clay (Fig. 5B and C), which may contribute to the adsorption of heavy metals.49 No micro-aggregates were observed and the soil surface became smoother after washing with CA and FeCl3 (Fig. 5D–F). It could be inferred that the removal of heavy metals was related to the disappearance of those micro-aggregates.56
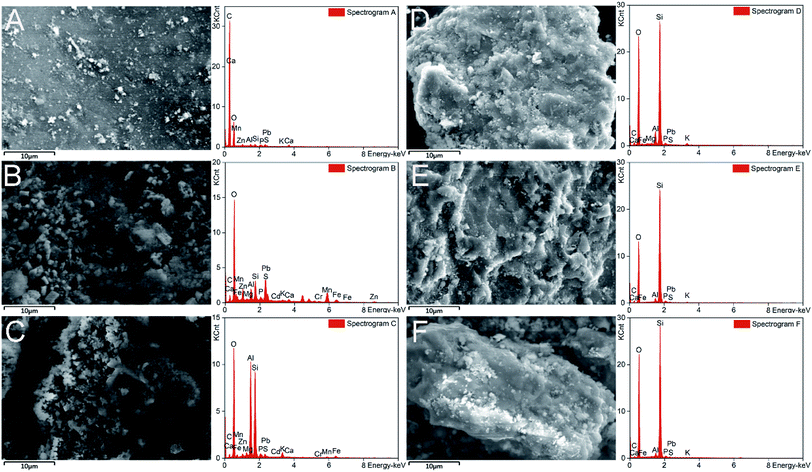 |
| Fig. 5 SEM and EDS images of soil before (A–C) and after (D–F) washing. A(D), B(E), and C(F) are images of the coarse sand, fine sand and silty clay, respectively. | |
The EDS analysis showed that O, Si, Al and C were predominant elements in the soil (Fig. 5). The contents of elements (e.g. Al, Mn and Fe) in the soil were decreased after washing. In addition, FTIR analysis was used to identify the functional groups of the soil.57 Hydroxyl and carboxyl groups were proven to be important in the adsorption of heavy metals in soil.58 In this study, the disappearance of carboxylic groups (1427 cm−1) and hydroxyl groups (1036 cm−1) illustrated the dissolution of organic matter after washing (Fig. S5†). These results revealed that soil elements (e.g. Al, Mn and Fe) and organic matter were dissolved by CA and FeCl3, which promoted the desorption of heavy metals from the soil.
3.7. Removal of heavy metals in a slurry reactor
In the lab, most of the experiments were small-scale washing tests in centrifuge tubes.3,59,60 Subsequently, large-scale stirring and washing equipment were used.61,62 Here, the removal of heavy metals in a slurry reactor was investigated to explore the application of washing with CA and FeCl3. After washing, the soil organic matter content decreased from 8.7% to 7.7%, and the pH decreased from 7.0 to 3.5. It was illustrated that acidification was one of the important mechanisms for the removal of heavy metals. Meanwhile, most of the Cd, Pb and Zn in the soil (>90%) were removed within 1.5 h (Fig. 6). Conversely, only 27.4% of Cr was removed from the soil after washing for 1.5 h. Then, the desorption of heavy metals achieved a dynamic equilibrium gradually. Finally, the removal efficiencies of Cd, Cr, Pb and Zn increased slowly and reached 94.8%, 79.5%, 92.7% and 97.2%, respectively.
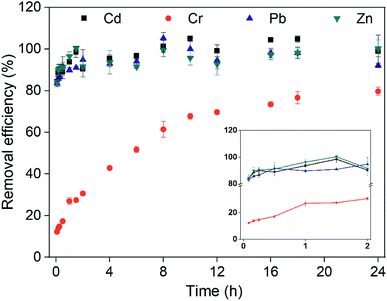 |
| Fig. 6 Removal efficiencies of Cd, Cr, Pb and Zn in contaminated soil by washing with CA and FeCl3 in the slurry reactor system; the removal efficiencies of Cd, Cr, Pb and Zn at the first 2 hours (the inset graphic). | |
After remediation, the concentrations of Cd, Pb and Zn in the soil were 33.8, 700.2 and 559.3 mg kg−1, which were lower than the risk screening values in the environmental protection administration guidelines of China.63 Additionally, the Cr(VI) concentration in soil (65.2 mg kg−1) was lower than the risk intervention value in the environmental protection administration guidelines of China.63 It was determined that column washing with CA and FeCl3 could be used at full scale.
4. Conclusions
In conclusion, CA and FeCl3 were selected from 7 washing agents for co-contaminated soil remediation. The optimum washing conditions were determined to be: CA (0.86 mol L−1), FeCl3 (1.00 mol L−1), washing time (10 h) and liquid
:
soil ratio (10
:
1). Heavy metals in smaller-size soil particles could be efficiently removed by washing. The bioavailability of heavy metals in soil was decreased after washing. The potential ecological risk of heavy metals in different treatments was reduced from an extremely high level to a low level. Furthermore, soil elements (e.g. Al, Mn and Fe) and organic matter were dissolved by CA and FeCl3, which promoted the desorption of heavy metals from the soil. In addition, washing with CA and FeCl3 could be used at full scale, resulting in the effective reduction of health risks from heavy metals in soil after washing.
Conflicts of interest
There are no conflicts to declare.
Abbreviations
CA | Citric acid |
OA | Oxalic acid |
FeCl3 | Ferric chloride |
Acknowledgements
The work was supported by the National Key Research and Development Program of China (2016YFD0800401), the National Natural Science Foundation of China (41721001). We would like to express our gratitude to Nianhang Rong and Xi Zheng at the analysis center of agrobiology and environmental sciences for their generous help in the observation of the surface morphology of soil particles.
References
- H. Hu, Q. Jin and P. Kavan, A study of heavy metal pollution in China: current status, pollution-control policies and countermeasures, Sustainability, 2014, 6, 5820–5838, DOI:10.3390/su6095820.
- Z. M. Gusiatin and E. Klimiuk, Metal (Cu, Cd and Zn) removal and stabilization during multiple soil washing by saponin, Chemosphere, 2012, 86, 383–391, DOI:10.1016/j.chemosphere.2011.10.027.
- A. Ferraro, M. Fabbricino, E. D. van Hullebusch, G. Esposito and F. Pirozzi, Effect of soil/contamination characteristics and process operational conditions on aminopolycarboxylates enhanced soil washing for heavy metals removal: a review, Rev. Environ. Sci. Bio/Technol., 2015, 15, 111–145, DOI:10.1007/s11157-015-9378-2.
- D. H. Rui, Z. P. Wu, M. C. Ji, J. F. Liu, S. R. Wang and Y. Ito, Remediation of Cd- and Pb- contaminated clay soils through combined freeze-thaw and soil washing, J. Hazard. Mater., 2019, 369, 87–95, DOI:10.1016/j.jhazmat.2019.02.038.
- A. Piccolo, R. Spaccini, A. De Martino, F. Scognamiglio and V. di Meo, Soil washing with solutions of humic substances from manure compost removes heavy metal contaminants as a function of humic molecular composition, Chemosphere, 2019, 225, 150–156, DOI:10.1016/j.chemosphere.2019.03.019.
- S. J. Ye, G. M. Zeng, H. P. Hu, C. Zhang, J. Liang, J. Dai, Z. F. Liu, W. P. Xiong, J. Wan, P. Xu and M. Cheng, Co-occurrence and interactions of pollutants, and their impacts on soil remediation—a review, Crit. Rev. Environ. Sci. Technol., 2017, 47, 1528–1553, DOI:10.1080/10643389.2017.1386951.
- Y. Y. Gong, D. Y. Zhao and Q. L. Wang, An overview of field-scale studies on remediation of soil contaminated with heavy metals and metalloids: Technical progress over the last decade, Water Res., 2018, 147, 440–460, DOI:10.1016/j.watres.2018.10.024.
- S. J. Ye, G. M. Zeng, H. P. Wu, C. Zhang, J. Dai, J. Liang, J. G. Yu, X. Y. Ren, H. Yi, M. Cheng and C. Zhang, Biological technologies for the remediation of co-contaminated soil, Crit. Rev. Biotechnol., 2017, 37, 1062–1076, DOI:10.1080/07388551.2017.1304357.
- S. J. Ye, M. Yan, X. F. Tan, J. Liang, G. M. Zeng, H. P. Wu, B. Song, C. Y. Zhou, Y. Yang and H. Wang, Facile assembled biochar-based nanocomposite with improved graphitization for efficient photocatalytic activity driven by visible light, Appl. Catal., B, 2019, 250, 78–88, DOI:10.1016/j.apcatb.2019.03.004.
- S. J. Ye, G. M. Zeng, H. P. Wu, J. Liang, C. Zhang, J. Dai, W. P. Xiong, B. Song, S. H. Wu and J. F. Yu, The effects of activated biochar addition on remediation efficiency of co-composting with contaminated wetland soil, Resour., Conserv. Recycl., 2019, 140, 278–285, DOI:10.1016/j.resconrec.2018.10.004.
- L. W. Liu, W. Li, W. P. Song and M. X. Guo, Remediation techniques for heavy metal-contaminated soils: principles and applicability, Sci. Total Environ., 2018, 633, 206–219, DOI:10.1016/j.scitotenv.2018.03.161.
- G. Dermont, M. Bergeron, G. Mercier and M. Richer-Lafleche, Soil washing for metal removal: a review of physical/chemical technologies and field applications, J. Hazard. Mater., 2018, 152, 1–31, DOI:10.1016/j.jhazmat.2007.10.043.
- T. Yang and M. E. Hodson, Investigating the use of synthetic humic-like acid as a soil washing treatment for metal contaminated soil, Sci. Total Environ., 2019, 647, 290–300, DOI:10.1016/j.scitotenv.2018.07.457.
- N. Bolan, A. Kunhikrishnan, R. Thangarajan, J. Kumpiene, J. Park, T. Makino, M. B. Kirkham and K. Scheckel, Remediation of heavy metal
(loid)s contaminated soils – to mobilize or to immobilize?, J. Hazard. Mater., 2014, 266, 141–166, DOI:10.1016/j.jhazmat.2013.12.018.
- Z. A. Begum, I. M. Rahman, Y. Tate, H. Sawai, T. Maki and H. Hasegawa, Remediation of toxic metal contaminated soil by washing with biodegradable aminopolycarboxylate chelants, Chemosphere, 2012, 87, 1161–1170, DOI:10.1016/j.chemosphere.2012.02.032.
- R. W. Peters, Chelant extraction of heavy metals from contaminated soils, J. Hazard. Mater., 1999, 66, 151–210, DOI:10.1016/S0304-3894(99)00010-2.
- P. P. Falciglia, D. Malarbì, R. Maddalena, V. Greco and F. G. A. Vagliasindi, Remediation of Hg-contaminated marine sediments by simultaneous application of enhancing agents and microwave heating (MWH), Chem. Eng. J., 2017, 321, 1–10, DOI:10.1016/j.cej.2017.03.097.
- I. Gonzalez, A. Cortes, A. Neaman and P. Rubio, Biodegradable chelate enhances the phytoextraction of copper by Oenothera picensis grown in copper-contaminated acid soils, Chemosphere, 2011, 84, 490–496, DOI:10.1016/j.chemosphere.2011.03.015.
- H. J. Zhang, Y. T. Gao and H. B. Xiong, Removal of heavy metals from polluted soil using the citric acid fermentation broth: a promising washing agent, Environ. Sci. Pollut. Res., 2017, 24, 9506–9514, DOI:10.1007/s11356-017-8660-y.
- G. Li, X. L. Yang, L. L. Liang and S. H. Guo, Evaluation of the potential redistribution of chromium fractionation in contaminated soil by citric acid/sodium citrate washing, Arabian J. Chem., 2017, 10, S539–S545, DOI:10.1016/j.arabjc.2012.10.016.
- J. M. Wang, J. G. Jiang, D. Li, T. R. Li, K. M. Li and S. C. Tian, Removal of Pb and Zn from contaminated soil by different washing methods: the influence of reagents and ultrasound, Environ. Sci. Pollut. Res., 2015, 22, 20084–20091, DOI:10.1007/s11356-015-5219-7.
- L. Jean-Soro, F. Bordas and J. C. Bollinger, Column leaching of chromium and nickel from a contaminated soil using EDTA and citric acid, Environ. Pollut., 2012, 164, 175–181, DOI:10.1016/j.envpol.2012.01.022.
- C. Turgut, M. Katie Pepe and T. J. Cutright, The effect of EDTA and citric acid on phytoremediation of Cd, Cr, and Ni from soil using Helianthus annuus, Environ. Pollut., 2004, 131, 147–154, DOI:10.1016/j.envpol.2004.01.017.
- T. Makino, T. Kamiya, H. Takano, T. Itou, N. Sekiya, K. Sasaki, Y. Maejima and K. Sugahara, Remediation of cadmium-contaminated paddy soils by washing with calcium chloride: verification of on-site washing, Environ. Pollut., 2007, 147, 112–119, DOI:10.1016/j.envpol.2006.08.037.
- T. Makino, K. Sugahara, Y. Sakurai, H. Takano, T. Kamiya, K. Sasaki, T. Itou and N. Sekiya, Remediation of cadmium contamination in paddy soils by washing with chemicals: selection of washing chemicals, Environ. Pollut., 2006, 144, 2–10, DOI:10.1016/j.envpol.2006.01.017.
- X. F. Guo, Z. B. Wei, Q. T. Wu, C. P. Li, T. W. Qian and W. Zheng, Effect of soil washing with only chelators or combining with ferric chloride on soil heavy metal removal and phytoavailability: field experiments, Chemosphere, 2016, 147, 412–419, DOI:10.1016/j.chemosphere.2015.12.087.
- X. Q. Zhai, Z. W. Li, B. Huang, N. L. Luo, M. Huang, Q. Zhang and G. M. Zeng, Remediation of multiple heavy metal-contaminated soil through the combination of soil washing and in situ immobilization, Sci. Total Environ., 2018, 635, 92–99, DOI:10.1016/j.scitotenv.2018.04.119.
- J. C. Yoo, J. Beiyuan, L. Wang, D. C. W. Tsang, K. Baek, N. S. Bolan, Y. S. Ok and X. D. Li, A combination of ferric nitrate/EDDS-enhanced washing and sludge-derived biochar stabilization of metal-contaminated soils, Sci. Total Environ., 2018, 616–617, 572–582, DOI:10.1016/j.scitotenv.2017.10.310.
- M. Rivero-Huguet and W. D. Marshall, Scaling up a treatment to simultaneously remove persistent organic pollutants and heavy metals from contaminated soils, Chemosphere, 2011, 83, 668–673, DOI:10.1016/j.chemosphere.2011.02.007.
- Y. T. Luo, B. H. Ye, J. E. Ye, J. L. Pang, Q. Xu, J. X. Shi, B. B. Long and J. Y. Shi, Ca2+ and SO42− accelerate the reduction of Cr(VI) by Penicillium oxalicum SL2, J. Hazard. Mater., 2019, 382, 121072, DOI:10.1016/j.jhazmat.2019.121072.
- A. Tessier, P. G. C. Campbell and M. Bisson, Sequential extraction procedure for the speciation of particulate trace-metals, Anal. Chem., 1979, 51, 844–851, DOI:10.1021/ac50043a017.
- C. Peng, H. Zhang, H. X. Fang, C. Xu, H. M. Huang, Y. Wang, L. J. Sun, X. F. Yuan, Y. X. Chen and J. Y. Shi, Natural organic matter–induced alleviation of the phytotoxicity to rice (Oryza sativa L.) caused by copper oxide nanoparticles, Environ. Toxicol. Chem., 2015, 34, 1996–2003, DOI:10.1002/etc.3016.
- F. Asadzadeh, M. Maleki-Kaklar, N. Soiltanalinejad and F. Shabani, Central composite design optimization of zinc removal from contaminated soil, using citric acid as biodegradable chelant, Sci. Rep., 2018, 8, 2633, DOI:10.1038/s41598-018-20942-9.
- T. Makino, H. Takano, T. Kamiya, T. Itou, N. Sekiya, M. Inahara and Y. Sakurai, Restoration of cadmium-contaminated paddy soils by washing with ferric chloride: Cd extraction mechanism and bench-scale verification, Chemosphere, 2008, 70, 1035–1043, DOI:10.1016/j.chemosphere.2007.07.080.
- C. L. Chen, T. Tian, M. K. Wang and G. Wang, Release of Pb in soils washed with various extractants, Geoderma, 2016, 275, 74–81, DOI:10.1016/j.geoderma.2016.04.015.
- X. R. Wang, Y. X. Zhang, Q. Wang and J. M. Shu, Effect and removal mechanisms of 6 different washing agents for building wastes containing chromium, Sci. World J., 2012, 298407, 2012, DOI:10.1100/2012/298407.
- C. C. Liu and Y. C. Lin, Reclamation of copper-contaminated soil using EDTA or citric acid coupled with dissolved organic matter solution extracted from distillery sludge, Environ. Pollut., 2013, 178, 97–101, DOI:10.1016/j.envpol.2013.02.034.
- D. Naghipour, H. Gharibi, K. Taghavi and J. Jaafari, Influence of EDTA and NTA on heavy metal extraction from sandy-loam contaminated soils, J. Environ. Chem. Eng., 2016, 4, 3512–3518, DOI:10.1016/j.jece.2016.07.034.
- Y. R. Cao, S. R. Zhang, Q. M. Zhong, G. Y. Wang, X. X. Xu and T. Li, Feasibility of nanoscale zero-valent iron to enhance the removal efficiencies of heavy metals from polluted soils by organic acids, Ecotoxicol. Environ. Saf., 2018, 162, 464–473, DOI:10.1016/j.ecoenv.2018.07.036.
- S. M. J. Mirazimi, F. Rashchi and M. Saba, Vanadium removal from roasted LD converter slag: optimization of parameters by response surface methodology (RSM), Sep. Purif. Technol., 2013, 116, 175–183, DOI:10.1016/j.seppur.2013.05.032.
- H. J. Zhang, Z. W. Wang and Y. T. Gao, Compound washing remediation and response surface analysis of lead-contaminated soil in mining area by fermentation broth and saponin, Environ. Sci. Pollut. Res., 2018, 25, 6899–6908, DOI:10.1007/s11356-017-0971-5.
- M. D. Andrade, S. O. Prasher and W. H. Hendershot, Optimizing the molarity of a EDTA washing solution for saturated-soil remediation of trace metal contaminated soils, Environ. Pollut., 2007, 147, 781–790, DOI:10.1016/j.envpol.2006.07.010.
- M. A. M. Kedziorek, A. Dupuy, A. C. M. Bourg and F. Compere, Leaching of Cd and Pb from a polluted soil during the percolation of EDTA: laboratory column experiments modeled with a non-equilibrium solubilization step, Environ. Sci. Technol., 1998, 32, 1609–1614, DOI:10.1021/es970708m.
- S. Y. Zhang, J. Wen, Y. Hu, Y. Fang, H. B. Zhang, L. Xing, Y. Wang and G. Zeng, Humic substances from green waste compost: an effective washing agent for heavy metal (Cd, Ni) removal from contaminated sediments, J. Hazard. Mater., 2019, 366, 210–218, DOI:10.1016/j.jhazmat.2018.11.103.
- Y. Li, X. Y. Liao and W. A. Li, Combined sieving and washing of multi-metal-contaminated soils using remediation equipment: a pilot-scale demonstration, J. Cleaner Prod., 2019, 212, 81–89, DOI:10.1016/j.jclepro.2018.11.294.
- C. Waterlot, G. Bidar, C. Pruvot and F. Douay, Effects of grinding and shaking on Cd, Pb and Zn distribution in anthropogenically impacted soils, Talanta, 2012, 98, 185–196, DOI:10.1016/j.talanta.2012.06.068.
- M. T. Shehzad, G. Murtaza, M. Shafeeque, M. Sabir, H. Nawaz and M. J. Khan, Assessment of trace elements in urban topsoils of Rawalpindi-Pakistan: a principal component analysis approach, Environ. Monit. Assess., 2019, 191, 65, DOI:10.1007/s10661-019-7212-y.
- S. E. Fendorf, Surface reactions of chromium in soils and waters, Geoderma, 1995, 67, 55–71, DOI:10.1016/0016-7061(94)00062-F.
- X. Y. Liao, Y. Li and X. L. Yan, Removal of heavy metals and arsenic from a co-contaminated soil by sieving combined with washing process, J. Environ. Sci., 2016, 41, 202–210, DOI:10.1016/j.jes.2015.06.017.
- S. J. Liu, J. Y. Jiang, S. Wang, Y. P. Guo and H. Ding, Assessment of water-soluble thiourea-formaldehyde (WTF) resin for stabilization/solidification (S/S) of heavy metal contaminated soils, J. Hazard. Mater., 2018, 346, 167–173, DOI:10.1016/j.jhazmat.2017.12.022.
- L. Q. Ma and G. N. Rao, Chemical fractionation of cadmium, copper, nickel, and zinc in contaminated soils, J. Environ. Qual., 1997, 26, 259–264, DOI:10.2134/jeq1997.00472425002600010036x.
- X. Zhang, K. Zhang, W. L. Lv, B. Liu, M. Aikawa and J. H. Wang, Characteristics and risk assessments of heavy metals in fine and coarse particles in an industrial area of central China, Ecotoxicol. Environ. Saf., 2019, 179, 1–8, DOI:10.1016/j.ecoenv.2019.04.024.
- W. C. Ji, T. T. Yang, S. Ma and W. Z. Ni, Heavy metal pollution of soils in the site of a retired paint and ink factory, Energy Procedia, 2012, 16, 21–26, DOI:10.1016/j.egypro.2012.01.005.
- J. W. Jin, M. Y. Wang, Y. C. Cao, S. C. Wu, P. Liang, Y. N. Li, J. Zhang, J. Zhang, M. H. Wong, S. Shan and P. Christie, Cumulative effects of bamboo sawdust addition on pyrolysis of sewage sludge: Biochar properties and environmental risk from metals, Bioresour. Technol., 2017, 228, 218–226, DOI:10.1016/j.biortech.2016.12.103.
- Q. X. Dai, L. P. Ma, N. Q. Ren, P. Ning, Z. Y. Guo and L. G. Xie, Research on the variations of organics and heavy metals in municipal sludge with additive acetic acid and modified phosphogypsum, Water Res., 2019, 155, 42–55, DOI:10.1016/j.watres.2019.02.015.
- Y. H. Sun, F. Guan, W. W. Yang and F. Y. Wang, Removal of chromium from a contaminated soil using oxalic acid, citric acid, and hydrochloric acid: dynamics, mechanisms, and concomitant removal of non-targeted metals, Int. J. Environ. Res. Public Health, 2019, 16, 2771, DOI:10.3390/ijerph16152771.
- Y. R. Cao, S. Zhang, G. Wang, Q. Huang, T. Li and X. Xu, Removal of Pb, Zn, and Cd from contaminated soil by new washing agent from plant material, Environ. Sci. Pollut. Res., 2017, 24, 8525–8533, DOI:10.1007/s11356-017-8542-3.
- J. Y. Shi, Q. H. Wu, C. Q. Zheng and J. J. Yang, The interaction between particulate organic matter and copper, zinc in paddy soil, Environ. Pollut., 2018, 243, 1394–1402, DOI:10.1016/j.envpol.2018.09.085.
- R. A. Wuana, F. E. Okieimen and J. A. Imborvungu, Removal of heavy metals from a contaminated soil using organic chelating acids, Int. J. Environ. Sci. Technol., 2010, 7, 485–496, DOI:10.1007/bf03326158.
- S. H. Yuan, X. F. Wu, J. Z. Wan, H. Y. Long, X. H. Lu and X. H. Wu, Enhanced washing of HCB and Zn from aged sediments by TX-100 and EDTA mixed solutions, Geoderma, 2010, 156, 119–125, DOI:10.1016/j.geoderma.2010.02.006.
- J. B. Qiao, H. M. Sun, X. H. Luo, W. Zhang, S. Mathews and X. Q. Yin, EDTA-assisted leaching of Pb and Cd from contaminated soil, Chemosphere, 2017, 167, 422–428, DOI:10.1016/j.chemosphere.2016.10.034.
- Z. Arwidsson, K. Elgh-Dalgren, T. von Kronhelm, R. Sjoberg, B. Allard and P. van Hees, Remediation of heavy metal contaminated soil washing residues with amino polycarboxylic acids, J. Hazard. Mater., 2010, 173, 697–704, DOI:10.1016/j.jhazmat.2009.08.141.
- CEPA, The soil environmental quality risk control standard for soil contamination of development land (GB36600–2018) (in Chinese), CEPA (Chinese Environmental Protection Adiministration), Beijing (in Chinese), 2018, http://kjs.mee.gov.cn/hjbhbz/bzwb/trhj/trhjzlbz/201807/t20180703_446027.shtml Search PubMed.
Footnote |
† Electronic supplementary information (ESI) available. See DOI: 10.1039/c9ra09999a |
|
This journal is © The Royal Society of Chemistry 2020 |
Click here to see how this site uses Cookies. View our privacy policy here.