DOI:
10.1039/C9RA10014H
(Paper)
RSC Adv., 2020,
10, 10848-10853
Enzymatic approach to cascade synthesis of bis(indolyl)methanes in pure water†
Received
29th November 2019
, Accepted 10th March 2020
First published on 16th March 2020
Abstract
A mild, efficient, and green protocol was developed for the synthesis of bis(indolyl)methanes catalyzed by lipase TLIM through the cascade reactions of indole with aldehydes in pure water. This methodology offers many superiorities such as excellent yields, wide substrate range, simple procedure, reusable and minimal amount of catalyst, and the ability to be scaled up.
1. Introduction
Bis(indolyl)methanes make great contributions in the fields of pharmacological industry and material science. They may act as agonists of the immunostimulatory orphan G protein-coupled receptor GPR84,1 as antagonists of Leishmania donovani promastigotes as well as axenic amastigotes,2 and also as probes for monitoring the interactions of related molecules with transport and response-mediating proteins.3 In addition, bis(indolyl)methanes play a brilliant role in the treatment of prostate cancer,4 colon cancer,5 pancreatic cancer6 and breast cancer.7 Bis(indolyl)methanes are generally obtained from cascade reactions of indole with carbonyl compounds catalyzed by protic or Lewis acids, which may be easily captured by nitrogenous compounds so as to bring some problems for the synthesis of bis(indolyl)methanes.8 Recently, various novel catalysts have been reported to synthesize bis(indolyl)methanes, such as aza-crown ether ionic liquids supported on magnetic Fe3O4@SiO2 core–shell particles,9 nanocomposites ZrO2–Al2O3–Fe3O4,10 1-hexenesulphonic acid sodium salt under ultrasound irradiation,11 SDS,12 zeolite,13 graphene oxide,14 AgOTf,15 LASSC catalytic system,16 meglumine catalyst,17 NADES,18 etc. However, there are some shortcomings in the most of catalytic methods mentioned above including expensive or complicated catalyst used, relative high temperature and excessive substrate needed, volatile organic solvent employed, etc. Therefore, it is meaningful to develop a green and efficient method to synthesize bis(indolyl)methanes.
The application of lipase in C–C bond formation reaction is attracting more and more attention due to its mild reaction conditions, lack of coenzyme requirements and the ability to work not only in aqueous systems but also in organic solvents.19 PPL was employed in aqueous 1,4-dioxane to catalyze the cascade reactions of indole with aromatic aldehydes to synthesize bis(indolyl)methanes.20 Le et al.21 found that α-chymotrypsin in aqueous ethanol exhibited prominent promiscuity for the synthesis of bis(indolyl)methanes. However, both of them could only obtain low to moderate yields for reactions of indole with aromatic aldehydes bearing electron-donating substituents as well as aliphatic aldehydes, and large amount of non-recyclable lipase was needed. In continuation of our interest in lipase-catalyzed C–C bond forming reactions,22 we herein report that cascade reactions of indole with aromatic aldehydes can be catalyzed by lipase TLIM in pure water to synthesize bis(indolyl)methanes effectively.
2. Materials and methods
2.1 Materials
Porcine pancreas lipase (PPL), Amano Lipase PS from Burkholderia cepacia (BCL) and Candida rugosa lipase (CRL) were purchased from Sigma. Lipase from Thermomyces lanuginosus immobilized on particle silica gel (TLIM), lipase from Rhizomucor miehei immobilized on anion exchange resin (RMIM), lipase B from Candida antarctica immobilized on a macroporous acrylic resin (Novozym 435) and papain were purchased from Novo Nordisk. Bovine serum albumin (BSA) was purchased from Shanghai Huixing. Other reagents were commercially available and were used without further purification.
2.2 Characterization
The melting points were determined on a WRS-1B digital melting point instrument and were not corrected. The 1H NMR and 13C NMR spectra were measured on a Bruker Advance 2B 300 MHz instrument with CDCl3 as solvent and TMS as internal standard. The HRMS were measured on Agilent LC/MS mass spectrometer. The progress of the reaction was monitored by TLC using pre-coated Haiyang GF254 silica gel plates. HPLC data was obtained using Dionex Liquid Chromatography (Diamonsil C18(2) (4.6 × 250 mm, 5 μm), water with 0.1% formic acid/methanol (v/v = 1/4, 20 min, radiant elution), 40 °C, 254 nm).
2.3 General procedure for the synthesis of bis(indolyl)methanes
A mixture of indole (2 mmol), aldehyde (1 mmol) and lipase (10 mg) in pure water (5 mL) was stirred at 55 °C. The progress of the reaction was monitored by TLC (ethyl acetate–petroleum ether, 1/2). Upon completion of the reaction, the reaction mixture was filtered, and the residue achieved was then dissolved in 1,4-dioxane (10 mL) to separate the product and lipase. By simple filtration, lipase was recovered and applied to the next run directly. After evaporation, to recover 1,4-dioxane, the crude products could be obtained and further purified by column chromatography (eluent, ethyl acetate–petroleum ether, 1/3) on silica gel (200–300 mesh).
3. Results and discussion
3.1 Screening of enzyme sources for the cascade synthesis of bis(indolyl)methanes
In our previous research,22d lipase RMIM was successfully used in pure water to catalyze the Knoevenagel–Michael cascade reactions of 4-hydroxycoumarin with aromatic, heterocyclic or aliphatic aldehydes to synthesize dicoumarol derivatives (81–98%). In our initial research, the cascade reaction of 1H-indole with 4-chlorobenzaldehyde was catalyzed by lipase RMIM in pure water (Table 1, entry 1). However, the result was not as satisfactory as we expected and only 51% of product was obtained. Subsequently, we tried other lipases to screen more effective catalysts. As showed in Table 1, lipases CRL, BCL, Novozym 435, PPL, TLIM (Table 1, entries 3–7) as well as papain (Table 1, entry 9) and BSA (Table 1, entry 10) can promote the model reaction (Scheme 1). Lipase TLIM (lipase from Thermomyces lanuginosus, immobilized on particle silica gel, the catalyst dosage of lipase on particle silica gel is 7%) showed the best catalytic activity and gave the corresponding product in 56% yield. As for the denatured TLIM (Table 1, entry 8), it demonstrated little catalytic activity and presented similar result with the blank control reaction (Table 1, entry 2). We supposed that the specific tertiary structure of enzymes makes great contributions in this enzyme-catalyzed reaction. The immobilized lipase TLIM is relatively efficient and has the possibility of reuse since it worked as a heterogeneous catalyst. As a result, lipase TLIM was chosen as the promoter (Table 2).
Table 1 Effect of enzyme sources on the yield of 3a
Entry |
Enzymea |
Yieldb (%) |
Entry |
Enzymea |
Yieldb (%) |
Reaction conditions: lipase (50 mg), 4-chlorobenzaldehyde (1 mmol), 1H-indole (2 mmol), water (5 mL), 45 °C, 18 h. HPLC yield. Denatured TLIM was obtained by treating with acetone for 24 h. |
1 |
RMIM |
51 |
6 |
PPL |
49 |
2 |
No enzyme |
3 |
7 |
TLIM |
56 |
3 |
CRL |
37 |
8 |
TLIMc |
7 |
4 |
BCL |
40 |
9 |
Papain |
40 |
5 |
Novozym 435 |
43 |
10 |
BSA |
41 |
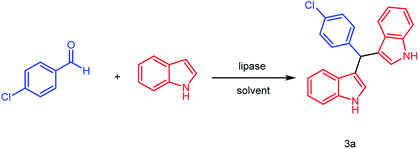 |
| Scheme 1 Enzymatic reaction of p-chlorobenzaldehyde with 1H-indole. | |
Table 2 Effect of solvent source on the yield of 3a
Entry |
Solventa |
Yieldb (%) |
Reaction conditions: TLIM (50 mg), 4-chlorobenzaldehyde (1 mmol), 1H-indole (2 mmol), solvent (5 mL), 45 °C, 18 h. HPLC yield. |
1 |
n-Hexane |
12 |
2 |
Toluene |
51 |
3 |
Water |
56 |
4 |
2-Propanol |
0 |
5 |
Acetonitrile |
0 |
6 |
1,4-Dioxane |
0 |
7 |
DMF |
0 |
8 |
THF |
0 |
9 |
DMSO |
0 |
10 |
Ethanol |
Trace |
3.2 Screening of solvent for the cascade synthesis of bis(indolyl)methanes
In our previous research,22c lipase TLIM showed the best result in n-hexane to catalyze the Knoevenagel–Michael cascade reactions of 1,3-diketones with aromatic aldehydes to generate 80–97% yields of xanthone derivatives. While in this study, TLIM showed better catalytic activity in water, and only 12% yield could be obtained n-hexane. Additionally, comparable yield also could be obtained when toluene was used as reaction media. Surprisingly, no product could be detected in solvents having a good solubility for the substrates and products like 2-propanol, acetonitrile, DMF, THF and DMSO. PPL was employed in aqueous 1,4-dioxane to synthesize bis(indolyl)methanes.20 Le et al.21 found that α-chymotrypsin in aqueous ethanol exhibited prominent promiscuity for the synthesis of bis(indolyl)methanes. However, no product in 1,4-dioxane and trace product in ethanol were detected in this research. Water has the worst solubility for the substrates and products, which may contribute to the progress of the reaction.
3.3 Screening of temperature, enzyme amount and reaction time for the cascade synthesis of bis(indolyl)methanes
The activity of enzymes and the rate of reactions are strongly associated with the temperature of reactions. The most suitable reaction temperature for TLIM in pure water to produce bis(indolyl)methanes is 55 °C (Fig. 1). Subsequently, we investigated the effect of enzyme loading on this cascade reaction. The results seem to be equivalent when the enzyme amount is more than 10 mg (Table 3, entries 2–4). As a result, 10 mg was chosen to be the optimized enzyme loading for 1 mmol 4-chlorobenzaldehyde substrate of this reaction, which is noteworthy that the enzyme amount is much lesser than other enzymatic reactions.20,21 With the optimized conditions in hand, high yields (93%) of 3a could be obtained after 36 h (Table 3, entry 7).
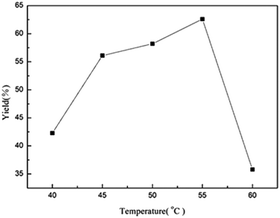 |
| Fig. 1 Effects of temperature on the yield of 3a. Reaction conditions: TLIM (50 mg), 4-chlorobenzaldehyde (1 mmol), 1H-indole (2 mmol), water (5 mL), 18 h, HPLC yield. | |
Table 3 Effects of enzyme amount and reaction time on the yield of 3a
Entrya |
Enzyme amount (mg) |
Time (h) |
Yieldb (%) |
Reaction conditions: TLIM, 4-chlorobenzaldehyde (1 mmol), 1H-indole (2 mmol), water (5 mL), 55 °C. HPLC yield. |
1 |
5 |
18 |
57 |
2 |
10 |
18 |
73 |
3 |
20 |
18 |
68 |
4 |
30 |
18 |
70 |
5 |
10 |
24 |
82 |
6 |
10 |
30 |
88 |
7 |
10 |
36 |
93 |
8 |
10 |
48 |
92 |
3.4 TLIM-catalyzed synthesis of bis(indolyl)methanes
Considering the overall effects of catalyst, solvents, temperature and catalyst loading were investigated, further studies on the exploration of substrate scopes and limitations were carried out (Scheme 2). As can be seen in Table 4, aromatic aldehydes with electron-withdrawing substituents (Table 4, entries 1–5) as well as aromatic aldehydes with electron-donating substituents (Table 4, entries 6–12) can both react easily with 1H-indole to give corresponding products with 75–99% yields. It is worth noting that the reported enzymatic cascade reactions couldn't get satisfactory yields when aliphatic aldehydes and aromatic aldehydes with electron-donating substituents react with 1H-indole.20,21 It is extraordinary that excellent result was obtained for aromatic aldehydes having large steric hindrance such as 4-tert-butylbenzaldehyde (Table 4, entry 15) with 1H-indole after 72 h. Satisfactorily, heterocyclic aldehydes which haven't been investigated in the previous enzymatic synthesis20,21 like 2-thiophenealdehyde (Table 4, entry 13) and pyridine-2-carbaldehyde (Table 4, entry 14) could react easily with 1H-indole to generate corresponding products in good yields after 72 h. In addition, indole bearing different substituents (Table 4, entries 16–20) also could react smoothly with aldehydes under the reaction conditions with excellent yields obtained. Practically, this protocol could be applied to a gram-scale synthesis and high yield was obtained (Table 4, entry 21). Finally, recyclability and reusability of TLIM were investigated for the synthesis of 3a in a good yields (Table 4, entries 22–23). In summary, the method developed in this paper has a wider substrate scopes than the reported enzymatic cascade reactions and lipase TLIM could be reused with little loss of activity.
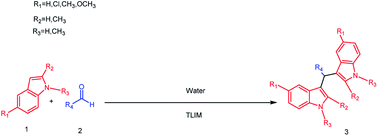 |
| Scheme 2 Enzymatic cascade reaction of aldehydes with indole. | |
Table 4 TLIM-catalyzed synthesis of bis(indolyl)methanes
Entrya |
R1 |
R2 |
R3 |
R4 |
Product |
Time/h |
Yieldb/% |
Reaction conditions: TLIM (10 mg), aldehyde (1 mmol), indole (2 mmol), water (5 mL), 55 °C. HPLC yield. Reaction conditions: TLIM (10 mg), formaldehyde aqueous solution (37%, 3 mmol), indole (2 mmol), water (5 mL), 55 °C. Isolated yield. Reaction conditions: TLIM (100 mg), 4-chlorobenzaldehyde (10 mmol), 1H-indole (20 mmol), water (50 mL), 55 °C, HPLC yield. HPLC yield of 3a (run 2). HPLC yield of 3a (run 3). HPLC yield of 3a (run 4). HPLC yield of 3a (run 5). |
1 |
H |
H |
H |
4-ClC6H5 |
3a |
36 |
93(90d) |
2 |
H |
H |
H |
3-NO2-C6H5 |
3b |
36 |
85 |
3 |
H |
H |
H |
4-NO2-C6H5 |
3c |
36 |
87 |
4 |
H |
H |
H |
2-F-C6H5 |
3d |
36 |
99 |
5 |
H |
H |
H |
4-CN-C6H5 |
3e |
36 |
98 |
6 |
H |
H |
H |
C6H6 |
3f |
36 |
98 |
7 |
H |
H |
H |
4-CH3-C6H5 |
3g |
36 |
95 |
8 |
H |
H |
H |
4-OCH3-C6H5 |
3h |
36 |
95 |
9 |
H |
H |
H |
4-OH-3-OCH3-C6H4 |
3i |
36 |
88 |
10 |
H |
H |
H |
2-OH-3-OCH3-C6H4 |
3j |
36 |
88 |
11 |
H |
H |
H |
C3H7 |
3k |
72 |
87 |
12 |
H |
H |
H |
Hc |
3l |
36 |
75 |
13 |
H |
H |
H |
2-Thienyl |
3m |
72 |
89 |
14 |
H |
H |
H |
2-Pyridyl |
3n |
72 |
72 |
15 |
H |
H |
H |
4-t-C4H9-C6H5 |
3o |
72 |
95 |
16 |
H |
CH3 |
H |
4-Cl-C6H5 |
3p |
36 |
96d |
17 |
CH3 |
H |
H |
4-Cl-C6H5 |
3q |
36 |
98d |
18 |
OCH3 |
H |
H |
4-Cl-C6H5 |
3r |
36 |
88d |
19 |
Cl |
H |
H |
4-Cl-C6H5 |
3s |
36 |
90d |
20 |
H |
H |
CH3 |
4-Cl-C6H5 |
3t |
36 |
93d |
21 |
H |
H |
H |
4-Cl-C6H5 |
3a |
36 |
94e |
22 |
H |
H |
H |
4-Cl-C6H5 |
3a |
36 |
86f |
23 |
H |
H |
H |
4-Cl-C6H5 |
3a |
36 |
81g |
24 |
H |
H |
H |
4-Cl-C6H5 |
3a |
36 |
77h |
25 |
H |
H |
H |
4-Cl-C6H5 |
3a |
36 |
74i |
3.5 Possible mechanism for the synthesis of bis(indolyl)methanes catalyzed by TLIM
Enzymatic synthesis of bis(indolyl)methanes was carried out using lipase TLIM as catalyst. Based on literatures,18,20 it can be explained by a proposed mechanism depicted in Scheme 3. We supposed that the catalytic active site of lipase TLIM plays a vital role in the cascade reaction. Firstly, the Gly residues and Ser residues interacted with the carbonyl of aromatic aldehyde to form the oxyanion hole, which could stabilized the activated aromatic aldehyde. Then the highly nucleophilic 3-position of indole attacked the activated aromatic aldehyde to form the intermediate product, which interacted with His residues. Finally, the intermediate product contacted with another molecule of indole and joined together to form the final product and released a molecule of water, and the catalyst was set free for the next cycle.
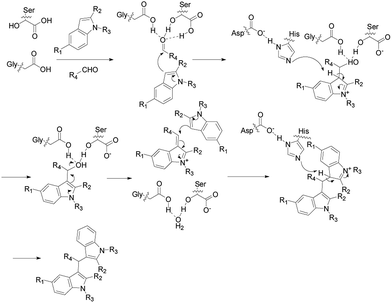 |
| Scheme 3 Possible mechanism for the synthesis of bis(indolyl)methanes catalyzed by TLIM. | |
4. Comparison of the catalytic efficiency of TLIM with some reported catalysts
Finally, we selected the reaction of 4-methoxybenzaldehyde with indole for the synthesis of 3h as a model reaction and compared the catalytic efficiency of TLIM with other reported catalysts in terms of reaction time, temperature, solvent and yields (Table 5). As can be seen in Table 5, this work could generate higher yield of corresponding product than most of reported methods. Moreover, TLIM was used in pure water instead of volatile, toxic organic solvents. Compared with chemical catalysis methods, TLIM-catalyzed synthesis of bis(3-indolyl)methane has several advantages such as environmental begin, simple, commercially-available and recyclable catalyst.
Table 5 Comparison of the catalytic efficiency of TLIM with some reported catalysts
Entry |
Catalyst |
Time/h |
Temperature/°C |
Solvent |
Yield/% |
Ref. |
1 |
Lipase TLIM |
36 |
55 |
Water |
95 |
This work |
2 |
SDS |
18 |
rt |
MeOH |
78 |
12 |
3 |
Zeolites |
1.5 |
rt |
CH2Cl2 |
85 |
13 |
4 |
Lipase PPL |
72 |
50 |
Aqueous 1,4-dioxane |
50 |
20 |
5 |
α-Chymotrypsin |
32 |
50 |
Aqueous ethanol |
75 |
21 |
6 |
MoS2–RGO |
2 |
rt |
Water |
89 |
23 |
7 |
[DABCO-H][HSO4] |
2 |
90 |
— |
74 |
24 |
5. Spectroscopic data for new products
5.1 3,3′-((2-Fluorophenyl)methylene)bis(1H-indole) (3d)
Yield: 0.99 mmol (99%); red solid; mp 76–77 °C. 1H NMR (300 MHz, CDCl3) δ 7.93 (s, 2H), 7.38 (dd, J = 13.0, 8.1 Hz, 4H), 7.24–7.13 (m, 4H), 7.09 (d, J = 9.6 Hz, 1H), 7.00 (q, J = 6.9, 6.1 Hz, 3H), 6.73 (s, 2H), 6.23 (s, 1H). 13C NMR (75 MHz, CDCl3) δ 160.2, 158.2, 137.5, 129.8, 129.6, 128.6, 127.4, 125.6, 122.7, 121.2, 119.2, 115.4, 114.3, 111.6, 30.8. HRMS (EI-TOF): m/z calcd for C23H17N2F [M + Na]+: 340.3921, found 340.3930.
5.2 3,3′-((4-Chlorophenyl)methylene)bis(2-methyl-1H-indole) (3p)
Yield: 0.3686 g (96%); red solid; mp 162–163 °C. 1H NMR (300 MHz, CDCl3) δ 7.78 (s, 2H), 7.28 (s, 2H), 7.24 (d, J = 8.9 Hz, 4H), 7.07 (t, J = 7.4 Hz, 2H), 7.00 (d, J = 7.8 Hz, 2H), 6.94–6.86 (m, 2H), 5.98 (s, 1H), 2.07 (s, 6H). 13C NMR (75 MHz, CDCl3) δ 135.0, 131.8, 130.4, 129.3, 128.2, 120.7, 119.2, 112.9, 110.0, 104.6, 38.7, 12.4. HRMS (EI-TOF): m/z calcd for C25H21N2Cl[M + Na]+: 384.1400, found 384.1389.
5.3 3,3′-((4-Chlorophenyl)methylene)bis(5-chloro-1H-indole) (3s)
Yield: 0.3816 g (90%); pink solid; mp 175–176 °C. 1H NMR (300 MHz, CDCl3) δ 7.96 (s, 2H), 7.30 (d, J = 7.2 Hz, 4H), 7.26 (s, 2H), 7.22 (d, J = 8.5 Hz, 2H), 7.19–7.06 (m, 2H), 6.65 (s, 2H), 5.74 (s, 1H). 13C NMR (75 MHz, CDCl3) δ 141.3, 136.8, 128.70, 128.6, 127.4, 127.2, 124.2, 121.2, 120.8, 119.6, 113.7, 112.2, 34.8. HRMS (EI-TOF): m/z calcd for C23H15N2Cl3[M + Na]+: 424.0311 found 424.0315.
6. Conclusions
In summary, we have successfully developed an environmentally friendly and highly efficient method to synthesize bis(indolyl)methanes, which could make for some drawbacks of the reported enzyme-catalyzed synthesis of bis(indolyl)methanes. This protocol can generate bis(indolyl)methanes with wide substrate range in excellent yields in pure water using very small amount of recyclable lipase. What's more, this enzymatic approach to cascade synthesis of bis(indolyl)methanes could be scaled up.
Conflicts of interest
There are no conflicts to declare.
Acknowledgements
This research was financially supported by the National Natural Science Foundation of China (No. 21676143), the Self-owned Research Project from Key Laboratory of Materials-Oriented Chemical Engineering (No. ZK201603), the Jiangsu Synergetic Innovation Center for Advanced Bio-Manufacture, and the Qing Lan Project of Jiangsu Province.
Notes and references
- T. Pillaiyar, M. Kose, K. Sylvester, H. Weighardt, D. Thimm, G. Borges, I. Forster, I. von Kugelgen and C. E. Muller, Diindolylmethane Derivatives: Potent Agonists of the Immunostimulatory Orphan G Protein-Coupled Receptor GPR84, J. Med. Chem., 2017, 60(9), 3636–3655 CrossRef CAS PubMed.
- S. B. Bharate, J. B. Bharate, S. I. Khan, B. L. Tekwani, M. R. Jacob, R. Mudududdla, R. R. Yadav, B. Singh, P. R. Sharma, S. Maity, B. Singh, I. A. Khan and R. A. Vishwakarma, Discovery of 3,3'-diindolylmethanes as potent antileishmanial agents, Eur. J. Med. Chem., 2013, 63, 435–443 CrossRef CAS PubMed.
- K. K.-W. Lo, K. H.-K. Tsang, W.-K. Hui and N. Zhu, Luminescent rhenium(i) diimine indole conjugates – photophysical, electrochemical and protein-binding properties, Chem. Commun., 2003,(21), 2704–2705 RSC.
- K. Abdelbaqi, N. Lack, E. T. Guns, L. Kotha, S. Safe and J. T. Sanderson, Antiandrogenic and Growth Inhibitory Effects of Ring-Substituted Analogs of 3,3'-Diindolylmethane (Ring-DIMs) in Hormone-Responsive LNCaP Human Prostate Cancer Cells, Prostate, 2011, 71(13), 1401–1412 CrossRef CAS PubMed.
- A. Lerner, M. Grafi-Cohen, T. Napso, N. Azzam and F. Fares, The Indolic Diet-Derivative, 3,3'-Diindolylmethane, Induced Apoptosis in Human Colon Cancer Cells through Upregulation of NDRG1, J. Biomed. Biotechnol., 2012, 2012, 256178–256183 CAS.
- K. Yoon, S.-O. Lee, S.-D. Cho, K. Kim, S. Khan and S. Safe, Activation of nuclear TR3 (NR4A1) by a diindolylmethane analog induces apoptosis and proapoptotic genes in pancreatic cancer cells and tumors, Carcinogenesis, 2011, 32(6), 836–842 CrossRef CAS PubMed.
- Y. S. Kim and J. A. Milner, Targets for indole-3-carbinol in cancer prevention, J. Nutr. Biochem., 2005, 16(2), 65–73 CrossRef CAS PubMed.
-
(a) Y. T. Reddy, P. N. Reddy, B. S. Kumar and B. Rajitha, Efficient synthesis of bis(indolyl)methanes catalyzed by TiCl4, Indian J. Chem., Sect. B: Org. Chem. Incl. Med. Chem., 2005, 44(11), 2393–2395 Search PubMed;
(b) W. J. Li, X. F. Lin, J. Wang, G. L. Li and Y. G. Wang, A Mild and Efficient Synthesis of bis-Indolylmethanes Catalyzed by Sulfamic Acid, Synth. Commun., 2006, 35(21), 2765–2769 CrossRef;
(c) M. Xia, S. h. Wang and W. b. Yuan, Lewis Acid Catalyzed Electrophilic Substitution of Indole with Aldehydes and Schiff's Bases Under Microwave Solvent-Free Irradiation, Synth. Commun., 2004, 34(17), 3175–3182 CrossRef CAS;
(d) J. Kothandapani, A. Ganesan, P. Vairaprakash and S. S. Ganesan, Copper(II) chloride assisted aryl exchange in arylmethanes: a simple and efficient route to triarylmethane derivatives, Tetrahedron Lett., 2015, 56(17), 2238–2242 CrossRef CAS;
(e) G. Babu, N. Sridhar and P. T. Perumal, A Convenient Method of Synthesis of Bis-Indolylmethanes: Indium Trichloride Catalyzed Reactions of Indole with Aldehydes and Schiff's Bases, Synth. Commun., 2000, 30(9), 1609–1614 CrossRef CAS.
- D. Li, J. Wang, F. Chen and H. Jing, Fe3O4@SiO2 supported aza-crown ether complex cation ionic liquids: preparation and applications in organic reactions, RSC Adv., 2017, 7(8), 4237–4242 RSC.
- A. Wang, X. Liu, Z. Su and H. Jing, New magnetic nanocomposites of ZrO2–Al2O3–Fe3O4 as green solid acid catalysts in organic reactions, Catal. Sci. Technol., 2014, 4(1), 71–80 RSC.
- R. S. Joshi, P. G. Mandhane, S. D. Diwakar and C. H. Gill, Ultrasound assisted green synthesis of bis(indol-3-yl)methanes catalyzed by 1-hexenesulphonic acid sodium salt, Ultrason. Sonochem., 2010, 17(2), 298–300 CrossRef CAS PubMed.
- M. L. Deb and P. J. Bhuyan, An efficient and clean synthesis of bis(indolyl)methanes in a protic solvent at room temperature, Tetrahedron Lett., 2006, 47(9), 1441–1443 CrossRef CAS.
- M. Karthik, A. Tripathi, N. Gupta, M. Palanichamy and V. Murugesan, Zeolite catalyzed electrophilic substitution reaction of indoles with aldehydes: synthesis of bis(indolyl)methanes, Catal. Commun., 2004, 5(7), 371–375 CrossRef CAS.
- Y. Wang, R. Sang, Y. Zheng, L. Guo, M. Guan and Y. Wu, Graphene oxide: An efficient recyclable solid acid for the synthesis of bis(indolyl)methanes from aldehydes and indoles in water, Catal. Commun., 2017, 89, 138–142 CrossRef CAS.
- J. Beltra, M. C. Gimeno and R. P. Herrera, A new approach for the synthesis of bisindoles through AgOTf as catalyst, Beilstein J. Org. Chem., 2014, 10, 2206–2214 CrossRef PubMed.
- Z. Wu, G. Wang, S. Yuan, D. Wu, W. Liu, B. Ma, S. Bi, H. Zhan and X. Chen, Synthesis of bis(indolyl)methanes under dry grinding conditions, promoted by a Lewis acid–surfactant–SiO2-combined nanocatalyst, Green Chem., 2019, 21(13), 3542–3546 RSC.
- B. R. Nemallapudi, G. V. Zyryanov, B. Avula, M. R. Guda and S. Gundala, An effective green and ecofriendly catalyst for synthesis of bis(indolyl)methanes as promising antimicrobial agents, J. Heterocycl. Chem., 2019, 56(12), 3324–3332 CrossRef CAS.
- C. Grosso, A. Brigas, J. M. de los Santos, F. Palacios, A. Lemos and T. M. V. D. Pinho e Melo, Natural deep eutectic solvents in the hetero-Diels–Alder approach to bis(indolyl)methanes, Monatsh. Chem., 2019, 150(7), 1275–1288 CrossRef CAS.
-
(a) Y. Ding, H. Huang and Y. Hu, New Progress on Lipases Catalyzed C-C Bond Formation Reactions, Chin. J. Org. Chem., 2013, 33(5), 905–914 CrossRef CAS;
(b) Y. Miao, M. Rahimi, E. M. Geertsema and G. J. Poelarends, Recent developments in enzyme promiscuity for carbon-carbon bond-forming reactions, Curr. Opin. Chem. Biol., 2015, 25, 115–123 CrossRef CAS PubMed;
(c) M. Lopez-Iglesias and V. Gotor-Fernandez, Recent Advances in Biocatalytic Promiscuity: Hydrolase-Catalyzed Reactions for Nonconventional Transformations, Chem. Rec., 2015, 15(4), 743–759 CrossRef CAS PubMed;
(d) D. Koszelewski, D. Paprocki, A. Madej, F. Borys, A. Brodzka and R. Ostaszewski, Enzymatic Tandem Approach to Knoevenagel Condensation of Acetaldehyde with Acidic Methylene Compounds in Organic Media, Eur. J. Org. Chem., 2017, 2017(31), 4572–4579 CrossRef CAS;
(e) M. Svedendahl, K. Hult and P. Berglund, Fast carbon-carbon bond formation by a promiscuous lipase, J. Am. Chem. Soc., 2005, 127(51), 17988–17989 CrossRef CAS PubMed.
- Z. Xiang, Z. Liu, X. Chen, Q. Wu and X. Lin, Biocatalysts for cascade reaction: porcine pancreas lipase (PPL)-catalyzed synthesis of bis(indolyl)alkanes, Amino Acids, 2013, 45(4), 937–945 CrossRef CAS PubMed.
- Z. B. Xie, D. Z. Sun, G. F. Jiang and Z. G. Le, Facile synthesis of bis(indolyl)methanes catalyzed by alpha-chymotrypsin, Molecules, 2014, 19(12), 19665–19677 CrossRef PubMed.
-
(a) Y. Ding, X. Ni, M. Gu, S. Li, H. Huang and Y. Hu, Knoevenagel condensation of aromatic aldehydes with active methylene compounds catalyzed by lipoprotein lipase, Catal. Commun., 2015, 64, 101–104 CrossRef CAS;
(b) Y. Ding, X. Xiang, M. Gu, H. Xu, H. Huang and Y. Hu, Efficient lipase-catalyzed Knoevenagel condensation: utilization of biocatalytic promiscuity for synthesis of benzylidene-indolin-2-ones, Bioprocess Biosyst. Eng., 2016, 39(1), 125–131 CrossRef CAS PubMed;
(c) Y. Fu, B. Fan, H. Chen, H. Huang and Y. Hu, Promiscuous enzyme-catalyzed cascade reaction: Synthesis of xanthone derivatives, Bioorg. Chem., 2018, 80, 555–559 CrossRef CAS PubMed;
(d) Y. Fu, Z. Lu, K. Fang, X. He, H. Huang and Y. Hu, Promiscuous enzyme-catalyzed cascade reaction in water: Synthesis of dicoumarol derivatives, Bioorg. Med. Chem. Lett., 2019, 29(10), 1236–1240 CrossRef CAS PubMed.
- A. Bahuguna, S. Kumar, V. Sharma, K. L. Reddy, K. Bhattacharyya, P. C. Ravikumar and V. Krishnan, Nanocomposite of MoS2-RGO as Facile, Heterogeneous, Recyclable, and Highly Efficient Green Catalyst for One-Pot Synthesis of Indole Alkaloids, ACS Sustainable Chem. Eng., 2017, 5(10), 8551–8567 CrossRef CAS.
- D.-z. Xu, J. Tong and C. Yang, Ionic Liquid [DABCO-H][HSO4] as a Highly Efficient and Recyclable Catalyst for Friedel–Crafts Alkylation in the Synthesis of Bis(naphthol)methane and Bis(indolyl)methane Derivatives, Synthesis, 2016, 48(20), 3559–3566 CrossRef.
Footnote |
† Electronic supplementary information (ESI) available. See DOI: 10.1039/c9ra10014h |
|
This journal is © The Royal Society of Chemistry 2020 |