DOI:
10.1039/C9RA10089J
(Paper)
RSC Adv., 2020,
10, 6052-6062
iTRAQ-based proteomic and physiological analyses of mustard sprouts in response to heat stress†
Received
2nd December 2019
, Accepted 30th January 2020
First published on 6th February 2020
Abstract
Heat stress has been proved to increase the content of melatonin in plants. In the present study, a combination of methods including physiological and biochemical, gene transcription and proteomic were used to investigate the melatonin accumulation mechanisms in mustard sprouts under heat treatment during the germination period. It was revealed that the heat treatment can significantly affect sprout growth, antioxidant enzyme activity and melatonin content in mustard sprouts. Meanwhile, the expression of melatonin key synthase genes, such as tryptophan decarboxylase genes (BjTDC 1, BjTDC 2) and serotonin N-acetyltransferase genes (BjSNAT 1), were significantly induced by heat stress, which coincided with the trend of melatonin content. Under the heat stress, a total of 172 differential abundance proteins were confidently identified in mustard sprouts and participated in many physiological processes. Functional classification analysis showed that the defense/pressure, energy and nucleotide metabolism, protein biosynthesis, signal transduction and transcription etc. were largely induced. Heat treatment stimulated a defense response at the protein level by regulating the growth and physiological metabolism in mustard sprouts. The results in this work provided novel deep insights into the proteins' response to heat stress, which will certainly promote further understanding of the heat-tolerance mechanism of mustard sprouts.
1 Introduction
Melatonin (MT, N-acetyl-5-methoxytryptamine) is an indoleamine neurohormone secreted by human and animal pineal gland cells, and regulates circadian rhythms, relieves sleep disorders and enhances immunity.1–4 Due to the simple chemical structure and the realization of chemical synthesis of melatonin, a large number of health food and dietary supplements with melatonin as the main raw material have been supplied on the market. Although the green synthesis process of melatonin has been advanced, a variety of by-products could still be generated during the chemical process of melatonin synthesis, such as 1,2,3,4-tetrahydro-β-carboline-3-carboxylic acid, 1,1′-ethylidenebis-(tryptophan) etc. However, the physiological effect of these by-products on the human body is unknown.5–7
At present, the research studies on plant melatonin are going through a phase of exponential growth.8 In plants, melatonin synthesis from tryptophan involves the usage of a stepwise sequence of four enzymes, i.e., tryptophan decarboxylase (TDC), tryptamine 5-hydroxylase (T5H), serotonin N-acetyltransferase (SNAT), and N-acetylserotonin methyltransferase (ASMT).9 Melatonin has been found in a variety of seeds, fruits, vegetables, nuts and herbs, and its content is closely related to species, growth stages, tissues and environment.10,11 Melatonin biosynthesis activity is high in young tissues of plants and low in mature tissues. There are significant differences in melatonin content in plants under different environmental conditions, especially the adverse conditions.12 It has been reported that high temperature treatment could increase N-acetylserotonin methyltransferase (ASMT) activity, thus increasing melatonin formation in rice seedlings.13 Brassica juncea (L.) is one of the most important oil-seed crops in China.14 We studied the changes of melatonin in mustard sprouts and found that the melatonin content of the mustard sprouts was significantly increased under the heat stress. Therefore, in line with the future development direction, developing melatonin-enriched nutritious food is a more environmentally friendly and green way of health care through regulating plant growth to promote the accumulation of melatonin in the body.15 Studies have been reported to concentrate melatonin from cherries to develop related products.5 However, the molecular understanding of melatonin metabolic process is still unclear, and the parallel molecular metabolic network of mustard sprouts in response to heat stress is also unknown.
In the present study, an iTRAQ labeling technique was employed to characterize the protein changes in mustard sprouts under the heat stress treatment. The results of physiological assays, relative gene expression of melatonin key synthase and the comparative proteomic analyses would be conducive to understand the physiological and molecular mechanisms of melatonin enrichment in mustard sprouts in response to heat stress.
2 Experimental
2.1 Plant growth and heat treatment
The mustard seeds (Brassica juncea L.) were surface sterilized by soaking in 1.5% (v/v) sodium hypochlorite for 15 min and then steeped in distilled water at 30 °C for 4 h. The soaked seeds were spread evenly on transparent square case (17.5 × 17.5 × 8.8 cm) filled with vermiculite and were irrigated with distilled water. The cases were transferred to a controlled environment chamber with a 16 h light/8 h dark cycle at an air temperature of 30 °C. After 2 days of germination, the treatments were carried out from the third day as follows: (1) control check (CK): the sprouts were sprayed with 20 mL distilled water; (2) heat stress (heat): the mustard sprouts were treated at 55 °C for 30 min every 24 h and sprayed with 20 mL distilled water started from third day. Mustard sprouts were randomly sampled on the fourth and seventh days for further biochemical measurements.
2.2 Measurement of sprout length, cellular damage indicators, and antioxidant activities
Thirty sprouts of each treatment and measure their length with micrometer. Membrane damage of mustard sprouts was assessed by electrolyte leakage as described by Dionisio-Sese and Tobita,16 and free amino acids were measured according to the method of Moore.17 The glutathione peroxidase (GSH-PX), and total antioxidant capacity (T-AOC) were determined using a GSH-PX Assay Kit (A005-1-2, Nanjing Jiancheng Bioengineering Institute, China), and a T-AOC Assay Kit (A015-1-2, Nanjing Jiancheng Bioengineering Institute, China) respectively, according to the manufacturer's instructions. The protein concentration of plant protein extraction was determined according to Bradford18 using bovine serum albumin as a standard.
2.3 Melatonin measurements
Melatonin in mustard sprouts was measured according to Arnao and Hernández-Ruiz.19 In detail, a 0.2 g fresh weight (FW) of tissue was cut into sections (3–5 mm) and without homogenization, placed in vials containing 3 mL of chloroform/methanol (30
:
1), overnight (15 h) at 4 °C in darkness with shaking. After centrifugation at 12
000g for 10 min, the supernatant was collected, evaporated to dryness, and then dissolved in 100 μL of 35% methanol. Then, 20 μL of the extraction was analyzed using an Agilent 1200 HPLC system (Agilent Technologies Co. Ltd., USA) coupled with fluorescence detection G1321A (Agilent Technologies Co. Ltd., USA) to determine melatonin. The sample was separated on a ZORBAX SB-C18 column (5 μm particle size, 4.6 × 250 mm; Agilent Technologies Co. Ltd., USA), gradient elution (42% MeOH to 50% MeOH gradient eluted for 27 min, then separation was followed by 18 min of isocratic elution with 50% MeOH.). The flow rate was 0.3 mL min−1 and the column temperature was set as 30 °C. The melatonin content was measured at excitation/emission wavelength of 280 nm/348 nm.
2.4 Determination of intracellular free calcium
The visualization of endogenous [Ca2+]cyt in mustard root tip was monitored with 10 μmol L−1 of Fluo-4 AM (visible wavelength calcium probe). The samples were loaded with Fluo-4 AM for 2 h at 4 °C in 20 mM HBSS buffer (pH 7.2), washed 3 times in HBSS buffer and then incubated at 25 °C without light for 2 h. The incubated samples were placed on a slide-dried slide and observed using LSM 880 NLO confocal laser scanning microscope (Leica Lasertechnik GmbH, Heidelberg, Germany) under an excitation wavelength of 488 nm (maximum absorption wavelength 512 nm, maximum emission wavelength 520 nm) with an argon ion laser to record fluorescence intensity.
2.5 Identification of the key genes of melatonin synthetic enzymes in mustard
The key genes of melatonin synthetic enzymes, including tryptophan decarboxylase (TDC), tryptamine 5-hydroxylase (T5H), serotonin N-acetyltransferase (SNAT), N-acetylserotonin methyltransferase (ASMT) genes sequences, were isolated according to the method developed by Lei, et al.20 The melatonin synthase homologous genes in rice, camptotheca, yew, laver, corn, arabidopsis and begonia as the reference were compared with the mustard genome database (http://brassicadb.org/brad/index.php). The candidate genes were acquired from the mustard genome, and their amino acid sequences were compared with the sequence of TDC (GenBank: AK065830, CAU73656), T5H (GenBank: AK071599.1, JQ618851), SNAT (GenBank: AK059369.1, NC007932, NC007982), and ASMT (GenBank: AK072740.1, KJ123721, At5g54160) using DNAMAN software respectively. Compared with melatonin synthase genes in other plants, selected candidate genes have conserved functional domains and over 30% homology (Table S1†).
2.6 RNA extraction and quantitative real-time PCR analysis (qRT-PCR)
Total RNA was isolated from mustard sprouts using a E.Z.N.A.™ Plant RNA Kit (R6827-01, OMEGA, USA). The RNA samples were reverse transcribed into cDNA. Triplicate quantitative assays were performed on each cDNA using SYBRR Premix Ex-Taq™ (RR420A, Takara, Japan) and the ABI 7500 sequence detection system (Applied Biosystems, Calif., USA) according to the manufacturer's protocol. The PCR amplification was performed using three-step cycling conditions of 95 °C for 30 s, followed by 40 cycles of 95 °C for 5 s and 60 °C for 30 s, respectively. The sequence-specific primers used were listed in Table S1.† The gene relative expression was analyzed by the 2−ΔΔCt method.21
2.7 Protein extraction, digestion, and iTRAQ labeling
Total protein in mustard sprouts was extracted using a Plant Total Protein Extraction Kit (PE0230, Sigma, USA) supplied with protease inhibitor. Protein concentration was determined by Pierce™ Coomassie Protein Assay Kit (23
200, Thermo Scientific, USA) using bovine serum albumin as the standard. The sample was reduced, alkylated, and then submitted to digestion with trypsin according to the method developed by Yin et al.22 Afterwards, each sample were labeled separately using the iTRAQ 8-plex kit (4381662, Sigma-Aldrich, USA) according to the manufacturer's instructions. Finally, all samples were combined and lyophilized.
2.8 LC-MS/MS and data analysis
The labeled samples were fractionated using a Thermo UHPLC U3000 Pump system (Thermo Fisher Scientific, CA, USA) by using an ACQUITY UPLC BEH C18 RP column (1.7 μm particle size, 2.1 × 100 mm; Waters, USA). The peptides were dissolved in solvent buffer A (20 mM ammonium formate, pH 10.0), and then separated at a flow rate of 0.2 mL min−1 with a gradient of 5–15% B (20 mM ammonium formate in 90% acetonitrile, pH 10.0) in 15 min, 15–45% B in 30 min and 90% B hold for 10 min. The absorbance at 214 nm was measured, a total of 10 fractions were collected every 1 min and dried in vacuum concentrator.
Each of the fractions was lyophilized and dissolved in buffer A (0.1% formic acid, 2% acetonitrile) and centrifuged at 14
000g for 20 min. Load 5 μL of the supernatant onto the trap column (Acclaim PepMap100C18, 75 μm × 2 cm, 3 μm, 100 Å, Thermo Scientific, USA) and then eluted on the analytical column (Acclaim PepMap® RSLC, C18, 75 μm × 15 cm, 3 μm, 100 Å, Thermo Scientific, CA, USA). Under a gradient program from 3 to 45% B (0.1% formate and 80% acetonitrile) at a flow rate of 250 nL min−1 over 112 min. The tandem mass spectrometry (MS/MS) analysis was performed using a LTQ-Orbitrap XL mass spectrometer, equipped with a nano-electrospray ion source (both from Thermo Fisher Scientific, CA, USA) according to Jin et al.23 The complete peptide was detected by Orbitrap with a resolution of 60
000. Using the high-energy collision dissociation (HCD) operating mode for MS/MS to select peptides, normalized collision energy is set to 40.0%; the resolution of ion fragments detected in the orbital trap was 7500. In the MS survey scan, a data-dependent program was applied to the 5 richest precursor ions (exceeding the threshold ion count 5000), which alternated between one MS scan and the subsequent 5 MS/MS scans for a duration of 60 s. The applied separation voltage was 2.4 kV, automatic gain control targets were 1 × 106 for MS1 and 1 × 105 for MS2. The m/z scan ranges were 350–1800 and 100–1800 Da for MS scans and MS2 scans, respectively.
Raw data were processed by a Proteome Discoverer Software 1.4 (Thermo Scientific). The protein identification was performed using Sequest HT engine against the uniprot Arabidopsis thaliana database. The searching parameters were as follows: trypsin was chosen as the enzyme used, allowance at most two missed cleavage; potential variable modifications were Gln → pyro-Glu (N-term Q), oxidation (M), deamidated (NQ), and the fixed modifications were carbamidomethyl (C), iTRAQ 8plex (K), iTRAQ 8plex (N-term). A peptide mass tolerance of 10 ppm and fragment mass tolerance of 0.02 Da were allowed. In order to be identified as important DAP, a protein must contain at least one unique peptide with a p-value less than 0.05 and fold change greater than 1.5 or less than 0.67. Biological functions of identified proteins were annotated according to KEGG (http://www.kegg.jp/kegg/pathway.html) and literature.23,24 Information of these proteins was acquired from the Universal Protein Resource (http://www.uniprot.org/). Moreover, KOBAS 3.0 (http://kobas.cbi.pku.edu.cn/) was used for pathway enrichment analysis.
2.9 Statistical analysis
Average values and standard deviation were computed according to the experimental data. Each data point was the mean of three biological replicates. Data obtained were subjected to analysis of variance (ANOVA), and the mean differences were compared with Tukey's test with a p value < 0.05 was considered significant.
3 Results and discussion
3.1 Physiological and biochemical indexes of mustard sprouts under heat treatment
As shown in Fig. 1A and B, heat treatment can significantly inhibit the growth and development of mustard sprouts compared with the control. As indicators of membrane damage, electrolyte leakage and free amino acid content dramatically enhanced in mustard sprouts under heat treatment (p < 0.05) (Fig. 1C and D). Furthermore, the activities of antioxidant enzymes GSH-PX and total antioxidant capacity T-AOC in mustard sprouts were dramatically induced by heat stress (p < 0.05) (Fig. 1E and F).
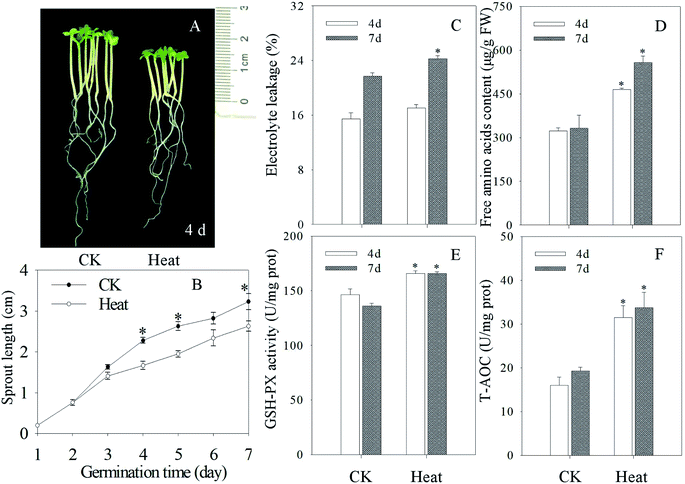 |
| Fig. 1 Effect of heat stress treatment on the growth performance and biochemical indexes of mustard sprouts. The (A) photograph, (B) sprout length, (C) electrolyte leakage, (D) free amino acid content, (E) GSH-PX activity, (F) T-AOC of mustard seeding subjected to heat stress were taken four days after germination. Each data point represents the average of three independent biological replications (average ± SE). An asterisk indicates that there is a significant difference between heat stress and control when the Tukey test is used at p < 0.05. | |
3.2 Effects of heat stress treatment on melatonin content
As shown in Fig. 2, after germinating for 4 days under heat treatment, the endogenous melatonin content increased significantly in mustard sprouts by 1.89 times higher than the control. The melatonin content in sprouts declined dramatically with sprouts growth, and the melatonin level at 7 days-old decreased 143.75% in comparison to 4 days-old sprouts under heat treatment.
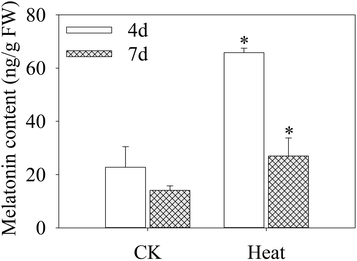 |
| Fig. 2 Effect of heat stress treatment on melatonin content in mustard sprouts. The asterisk indicates that there is a significant difference between heat stress and control at p < 0.05 using Tukey's test. | |
3.3 Intracellular free calcium
Root tips of mustard sprouts cultured in vermiculite for 4 days were cut into 4 mm and then put into a HBSS buffer solution in the presence (Fig. 3B and C) and in the absence of Fluo-4 AM (Fig. 3A), respectively. The root tips were observed after incubation. The cell wall of mustard sprout's root tip without the treatment by Fluo-4 AM showed faint spontaneous fluorescence, while almost no fluorescence in its cytoplasm was observed. However, under the heat stress treatment, the fluorescence intensity of mustard sprout root tips cell wall treated by Fluo-4 AM was significantly improved than control, exhibiting a brighter green fluorescence.
 |
| Fig. 3 Changes of calcium ions (Ca2+) in root tip cells of mustard sprouts after heat stress treatment for 4 d. (B) CK; (C) heat; (A) was treated with distilled water without addition of probe before observation under a laser scanning confocal microscope. (A–C) were pseudocolor images of Ca2+ in mustard seeding root tip, and (a–c) were the corresponding pictures taken under bright field. Color bar in the right side shows where dark is minimum (0) and white (or green) is maximum intensity (255). Scale bar = 50 μm. | |
3.4 Changes in gene expression of melatonin synthesis key enzyme in mustard sprouts
TDC, T5H, SNAT and ASMT are major enzymes in melatonin biosynthesis pathway. As shown in Fig. 4, compared with the control, the expression levels of genes involved in melatonin biosynthesis in mustard sprouts were significantly different during germination under heat treatment (p < 0.05). The gene expression levels of BjTDC 1 and BjTDC 2 in mustard sprouts by heat stress treatment were significantly increased compared with the control (Fig. 4A and B), and BjTDC 2 was coincided with the trend of melatonin content. The expression levels of BjT5H 1 and BjT5H 2 at 4 d were significantly higher than control group while the expression levels of BjT5H 1 and BjT5H 2 at 7 d were decreased (p < 0.05) (Fig. 4C and D). Under heat stress, the transcriptional levels of BjSNAT 1 and BjSNAT 4 in 4 d sprouts increased while the expressions of BjSNAT 2 and BjSNAT 3 were negatively correlated with the melatonin content (Fig. 4E–H). Furthermore, BjASMT 1 gene expression was opposite to that of BjASMT 2 and BjASMT 3 during germination. At 4 d, the expression of BjASMT 1 was 1.99 times higher than control group, while the expression of BjASMT 2 and BjASMT 3 decreased by 57.3% and 76.5% respectively.
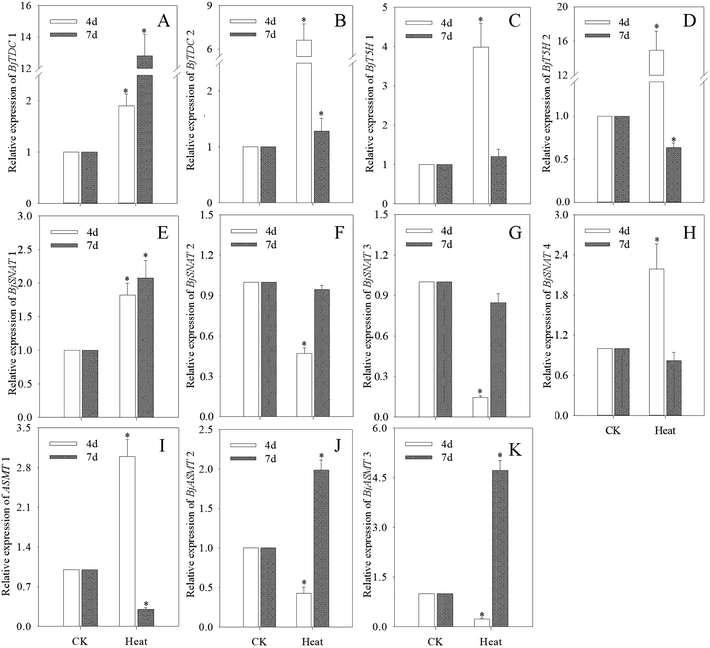 |
| Fig. 4 Changes of BjTDC 1 (A), BjTDC 2 (B), BjT5H 1 (C), BjT5H 2 (D), BjSNAT 1 (E), BjSNAT 2 (F), BjSNAT 3 (G), BjSNAT 4 (H), ASMT 1 (I), BjASMT 2 (J) and BjASMT 3 (K) relative expression in mustard sprouts under heat stress treatment. The asterisk indicates that there is a significant difference between heat stress and control at p < 0.05 using Tukey's test. | |
3.5 iTRAQ analysis and identification of differentially expressed protein (DAPs)
According to previous study,23 both an expression ratio >1.50 or <0.67 and a p-value of less than 0.05 were defined as DAPs. In the present study, a total of 172 DAPs were identified in all three replicates (Table S2†). These proteins were divided into fourteen functional classes according to UniProt and KEGG websites, based on the number of total DAPs, i.e., defense/pressure (10.5%), carbohydrate metabolism (4.7%), energy (15.7%), nucleotide metabolism (11.0%), amino acid metabolism (11.0%), secondary metabolism (7.0%), protein biosynthesis (10.5%), protein transport and storage (4.1%), protein folding and degradation (4.7%), transport (7.6%), signal transduction and transcription (2.3%), cell growth/splitting (6.4%), etc. (Fig. 5).
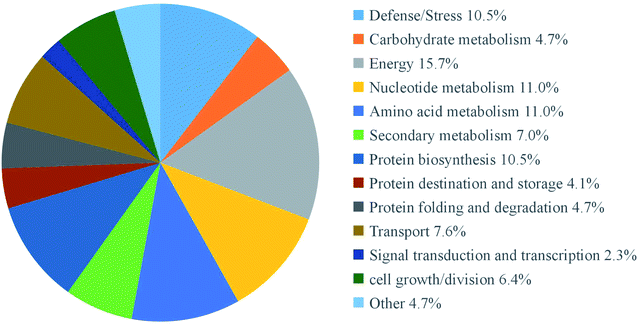 |
| Fig. 5 Functional classifications of differentially expressed proteins in mustard sprouts treated with under heat stress. | |
After germinating for 4 days under heat treatment, the abundance of 140 DAPs showed a significantly increased while 32 DAPs significantly decreased in mustard sprouts in comparison to the control (Fig. 6 and 7). These DAPs were analyzed by bioinformatics approaches to obtain relevant pathways information. All the identified peptides and DAPs under heat treatment were both classified into three major GO categories: biological processes (BP), cellular components (CC), and molecular functions (MF). Fig. 8 showed that in CC analysis, cytoplasm process count 54 DAPs was the most representative term (p-value: 2.22 × 10−11). Five DAPs in tryptophan biosynthesis pathway related to melatonin synthesis, and it is positively correlated with melatonin content.
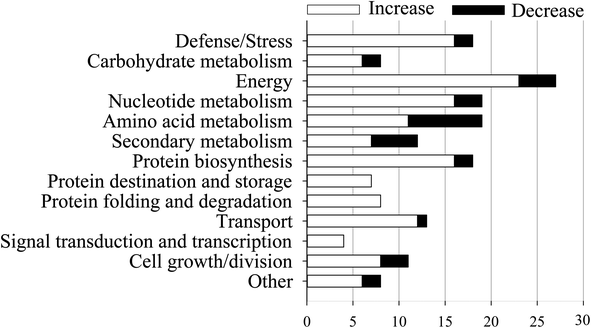 |
| Fig. 6 Change of DAPs abundances in each functional classification. The X-axis represents the numbers of DAPs enriched in each functional classification. The Y-axis represents DAPs functional classification. | |
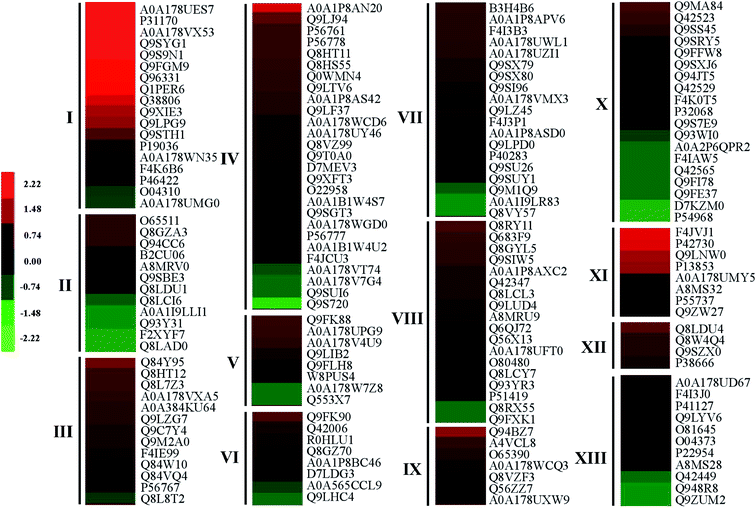 |
| Fig. 7 Proteins differentially expressed in 4 day mustard sprouts under heat stress. Expression changes were made based on the log 2 conversion expression ratios of the proteins applying Gene Cluster 3.0 software. Visualize results using JAVA Treeview software. Proteins were involved in defense/stress (I), secondary metabolism (II), transporters (III), energy (IV), carbohydrate metabolism (V), other (XI), nucleotide metabolism (XII), protein synthesis (XIII), protein destination and storage (IX), amino acid metabolism (X), protein folding and degradation (XI), signal transduction and transcription (XII), cell growth/division (XIII). | |
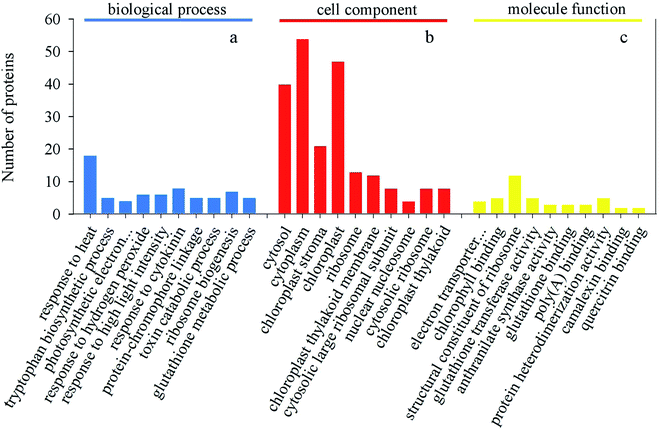 |
| Fig. 8 Gene Ontology classification of all DAPs. (a) Number of proteins in each classification of biological process. (b) Number of proteins in each classification of cellular component. (c) Number of proteins in each classification of molecular function. | |
In order to analyze the metabolic pathways that responded to heat stress, DAPs were further researched using the KEGG database. The pathway enrichment analysis demonstrated that 10 KEGG pathways were identified under heat stress treatment with a p-value less than 0.05 as the threshold (Table 1). The top three enrichments of pathways were for metabolic pathways, protein processing and secondary metabolites pathways, and 21, 13 and 12 DAPs were found to be enriched in these pathways, respectively.
Table 1 Pathway enrichment analysis of differentially rich proteins in mustard sprouts under heat stress
Pathway |
Pathway ID |
Input number |
p-value |
Protein processing in endoplasmic reticulum |
ath04141 |
12 |
4.25 × 10−11 |
Photosynthesis |
ath00195 |
7 |
2.67 × 10−8 |
Phenylalanine, tyrosine and tryptophan biosynthesis |
ath00400 |
6 |
1.53 × 10−7 |
Metabolic pathways |
ath01100 |
21 |
8.56 × 10−6 |
Glutathione metabolism |
ath00480 |
5 |
3.01 × 10−5 |
Biosynthesis of amino acids |
ath01230 |
7 |
5.83 × 10−5 |
Ribosome |
ath03010 |
8 |
0.0001 |
Biosynthesis of secondary metabolites |
ath01110 |
13 |
0.0002 |
Glycine, serine and threonine metabolism |
ath00260 |
3 |
0.0028 |
Peroxisome |
ath04146 |
3 |
0.0042 |
3.6 Discussion
Mustard sprouts contain several bioactive ingredients which have important health benefits, and the melatonin content in germinated sprouts was significantly increased by the heat stress treatment (Fig. 3), suggesting that mustard sprouts can be used as a good material to produce health-supporting functional ingredients. Using mustard sprouts as a source of melatonin for nutritious food would provide the insurance of avoiding multiple undesirable chemical by-products. At present, some commercial formulations exclusively composed of phytomelatonin are known, that the source of phytomelatonin were freeze-dried Montmorency tart cherry skin, rice (Oryza sativa L.), alfalfa (Medicago sativa L.), and aromatic/medicinal plants extracts through concentrations.5 Meanwhile, in the present study, the changes of morphological, physiological, gene expression and proteome in mustard sprouts under heat stress treatment were observed.
Heat stress treatment usually leads to severe damage to cell biofilms, imbalance of intracellular reactive oxygen species metabolism, and large amount of free radicals, which will affect the metabolism of macromolecular substances in plants and further affect the normal growth and development of plants.25 As an indicator of membrane damage and ROS levels, the electrolyte leakage in heat stressed sprouts were significantly enhanced in this study (Fig. 1C and D). It is reported that the plants have evolved a regulatory mechanism as well as an enzyme and antioxidant protection system to remove ROS to eliminate heat-triggered oxidative damage.26 Meanwhile, the raised activity of ROS detoxification enzymes GSH-PX and total antioxidant capacity T-AOC in mustard sprouts was summarized (Fig. 2), which was similar to others brassica of Cruciferae.27 Similarly, the proteome results showed that the abundances of two GSH (A0A178WN35, P46422) and POD (Q1PER6) were increased after heat treatment, implying their significant roles in response to heat stress.
As a plant cell signaling ion, Ca2+ plays a key role in adjusting plant growth and development under biotic and abiotic stress. External stimulating can induce the raise of intracellular Ca2+, which participates in transcriptional adjusting by its bio-regulation on transcription factors. In this study, it is observed that the heat stress treatment can significantly induce Ca2+ influx in mustard sprouts root tip cells (Fig. 2), which is consistent with the increase of Ca2+ in barley root tip cells under NaCl stress.28
In this study, four key genes homologous for melatonin biosynthesis enzyme were identified in mustard by using molecular biological methods. These include two BjTDCs, two BjT5Hs, four BjSNATs, and three BjASMTs. It seems that the different genes of melatonin synthetic are responsible for melatonin synthesis during different stages of mustard sprouts under heat stress conditions. This phenomenon has been observed in rice and cyanobacteria, in which these enzymes represent different sensitivity to different temperatures.29,30 Transcriptional levels of melatonin synthetase were not exactly consistent with the change in melatonin content under heat stress (Fig. 2 and 4). The possible reason may be that the transcriptional levels of genes had space–time nature, and the content of a compound was not always in consistent with the up-regulation of all genes involved in the compound biosynthesis.31
Melatonin in plants is synthesized by four steps of enzymatic reaction of tryptophan.9 In this study, some tryptophan biosynthesis-related DAPs were induced under heat stress conditions, such as tryptophan synthase beta chain (Q9FFW8), cyclase-like protein 2 (Q94JT5), tryptophan synthase alpha chain (Q42529) and anthranilate synthase alpha subunit 1 (F4K0T5). Melatonin, tryptophan and intermediate in rice samples increased significantly after treated with different concentrations of cadmium in comparison to the control.32 In this way, the increase in the levels of four tryptophan biosynthesis-related DAPs (Q9FFW8, Q94JT5, Q42529, F4K0T5) under heat stress treatment in the present study might contribute to the enhancement of melatonin in mustard sprouts.
The metabolic balance among protein synthesis and degradation play a crucial role in plant's response to environmental stress.33 In translation, ribosomes are large macromolecule ribosomal proteins (r-proteins) responsible for catalyzing protein synthesis in the cell, which plays an important role in regulating cell growth, differentiation and development.34,35 In this work, the abundance of most r-proteins were significantly increased in mustard sprouts under heat stress, such as two 40S r-proteins (Q8GYL5, Q9SIW5), six 60S r-proteins (P38666, Q8LCL3, Q9LUD4, P51419, P41127 and Q42347) and four ribosomal protein family (F4J3P1, A8MS28, A0A178VXA5 and B3H4B6). In some cases, protein folding in vivo is co-translational, that is, it is started before the completion of protein synthesis while the nascent chain is still engaged on the ribosome.36 In mustard sprouts, four major proteins (Q9LNW0, P13853, A0A178UMY5 and P55737) involved in protein folding were up-regulated under heat stress treatment. The results were consistent with the r-protein abundance shown above. These results indicated that the proteins of mustard sprouts were damaged under heat stress and therefore, it is necessary to synthesize more proteins by up-regulating protein folding and r-proteins.
In our proteomic analysis, heat treatment changed the expression levels of most of the proteins associated with energy metabolism. Photosynthesis is the main source of plant biomass, which is directly related to plant productivity and energy utilization. In detail, photosynthesis-related proteins abundance were increased by heat stress treatment, such as photosystem II (PS reaction center protein II CP43, P56778; PSII CP47 reaction center protein, Q8HS55; PSII CP43 reaction center protein; Q8HT11 and photosystem II CP47 reaction center protein, P56777), while photosystem I (PSI reaction center subunit VI-2, Q9SUI6) and PSI P700 chlorophyll an apoprotein (A2, A0A1B1W4U2) were inhibited. The enhancement in relative abundances of some of the photosynthesis-related proteins under heat stress at 55 °C in our study was similar to the findings in grapevine leaves at 43 °C;37 however, Shakeel found that some PSI and PSII-related proteins were inhibited in agave at 55 °C.38
On the basis of the different expression patterns of metabolic pathways and carbohydrate biosynthesis correlated enzymes, it is educed that plants need high energy to cope with heat stress treatment.39,40 In detail, an increase in abundance of ATP synthase F1 complex assembly factor (O22958), ATP-dependent Clp protease (Q9SXJ6) and ATPase ASNA1 homolog protein (Q9LPD0) may imply the increase of energy requirement in mustard sprouts responding heat stress. Increased expression of the ATPases was found in heat-stressed grapevine,37 soybean41 and rice,42 suggesting that the energy pathway in mustard sprouts may be highly affected or disordered by heat stress treatment. Moreover, heat stress treatment up-regulated the abundance of the glutamate–glyoxylate aminotransferase (Q9S7E9) associated with the glyoxylate cycle. Meanwhile, alpha-glucan phosphorylase (Q9LIB2) and alpha-1,4 glucan phosphorylase (W8PUS4) related to sucrose metabolism were increased. Probable fructokinase-7 (Q9FLH8) catalyzes the synthesis of D-fructose 6-phosphate from D-fructose, it is part of the glycolytic/gluconeogenic pathway and plays an important role in maintaining the flux of carbon towards starch formation.43 These results suggest that the mustard sprouts may induce different types of energy- and carbohydrate-related proteins to form a regulatory mechanism to enhance heat tolerance.
4 Conclusions
In this study, we provided a comprehensive overview on the morphological, physiological, gene expression and proteome in mustard sprouts under heat treatment. Heat stress significantly affected sprouts growth and development, induced membrane damage and increased antioxidant capacity. Accumulation of melatonin in mustard sprouts were raised by heat treatment, which was in accordance with the relative abundances of proteins involved in tryptophan biosynthesis and partially coincided with the gene expression of melatonin synthase. Proteins involved in defense/pressure, energy and nucleotide metabolism, protein biosynthesis and protein transport and storage as well as signal transduction and transcription were largely induced while those related to amino acid metabolism-related 8 DAPs were repressed by heat stress. These results provide novel insights into the accumulation of melatonin in mustard sprouts under heat stress, which would certainly contribute to the further understanding of heat-resistant mechanism in the mustard sprouts.
Conflicts of interest
There are no conflicts of interest to declare.
Acknowledgements
This work was financially supported by China Postdoctoral Science Foundation funded project (2019M651978), Qing Lan Project of Yangzhou University and Innovation & Entrepreneurship Training Program for College Students of Yangzhou University (X20190924).
References
- M. A. Nawaz, Y. Huang, Z. Bie, W. Ahmed, R. J. Reiter, M. Niu and S. Hameed, Melatonin: current status and future perspectives in plant science, Front. Plant Sci., 2016, 6, 1–13 CrossRef PubMed.
- E. Maronde and J. H. Stehle, The mammalian pineal gland: known facts, unknown facets, Trends Endocrinol. Metab., 2007, 18, 142–149 CrossRef CAS PubMed.
- R. J. Reiter, D. X. Tan, L. C. Manchester, S. D. Paredes and R. M. Sainz, Melatonin and reproduction revisited, Biol. Reprod., 2009, 81, 445–456 CrossRef CAS PubMed.
- L. C. Manchester, A. Coto Montes, J. A. Boga, L. P. H. Andersen, Z. Zhou, A. Galano, J. Vriend, D. X. Tan and R. J. Reiter, Melatonin: an ancient molecule that makes oxygen metabolically tolerable, J. Pineal Res., 2015, 59, 403–419 CrossRef CAS PubMed.
- M. B. Arnao and J. Hernández-Ruiz, Phytomelatonin, natural melatonin from plants as a novel dietary supplement: sources, activities and world market, J. Funct. Foods, 2018, 48, 37–42 CrossRef CAS.
- B. L. Williamson, K. L. Johnson, A. J. Tomlinson, G. J. Gleich and S. Naylor, On-line HPLC-tandem mass spectrometry structural characterization of case-associated contaminants of l-tryptophan implicated with the onset of eosinophilia myalgia syndrome, Toxicol. Lett., 1998, 99, 139–150 CrossRef CAS PubMed.
- B. L. Williamson, A. J. Tomlinson, P. K. Mishra, G. J. Gleich and S. Naylor, Structural characterization of contaminants found in commercial preparations of melatonin: similarities to case-related compounds from tryptophan associated with eosinophilia-myalgia syndrome, Chem. Res. Toxicol., 1998, 11, 234–240 Search PubMed.
- M. B. Arnao and J. Hernández-Ruiz, Functions of melatonin in plants: a review, J. Pineal Res., 2015, 59, 133–150 CrossRef CAS PubMed.
- K. Back, D. Tan and R. J. Reiter, Melatonin biosynthesis in plants: multiple pathways catalyze tryptophan to melatonin in the cytoplasm or chloroplasts, J. Pineal Res., 2016, 61, 426–437 CrossRef CAS PubMed.
- R. J. Reiter and D. X. Tan, Melatonin: an antioxidant in edible plants, Ann. N. Y. Acad. Sci., 2002, 957, 341–344 CrossRef CAS PubMed.
- Y. Byeon and K. Back, Melatonin synthesis in rice seedlings in vivo is enhanced at high temperatures and under dark conditions due to increased serotonin N-acetyltransferase and N-acetylserotonin methyltransferase activities, J. Pineal Res., 2014, 56, 189–195 CrossRef CAS PubMed.
- Y. Aguilera, T. Herrera, V. Benítez, S. M. Arribas, A. L. López De Pablo, R. M. Esteban and M. A. Martín-Cabrejas, Estimation of scavenging capacity of melatonin and other antioxidants: contribution and evaluation in germinated seeds, Food Chem., 2015, 170, 203–211 CrossRef CAS PubMed.
- Y. Byeon, G. Choi, H. Y. Lee and K. Back, Melatonin biosynthesis requires N-acetylserotonin methyltransferase activity of caffeic acid O-methyltransferase in rice, J. Exp. Bot., 2015, 66, 6917–6925 CrossRef CAS PubMed.
- H. Singh, B. P. Singh and H. Prasad, Weed management in Brassica species, Indian J. Agron., 2001, 46, 533–537 Search PubMed.
- B. Francesca, B. Elisa, F. Gaia, R. Luigi and R. Rita, Dietary melatonin supplementation could be a promising preventing/therapeutic approach for a variety of liver diseases, Nutrients, 2018, 10, 1135–1159 CrossRef PubMed.
- M. L. Dionisio-Sese and S. Tobita, Antioxidant responses of rice seedlings to salinity stress, Plant Sci., 1998, 135, 1–9 CrossRef CAS.
- S. Moore and W. H. Stein, A Modified Ninhydrin Reagent for the Photometric Determination of Amino Acids and Related Compounds, J. Biol. Chem., 1954, 211, 907–913 CAS.
- M. M. Bradford, A rapid and sensitive method for the quantitation of microgram quantities of protein utilizing the principle of protein-dye binding, Anal. Biochem., 1976, 72, 248–254 CrossRef CAS PubMed.
- M. B. Arnao and J. Hernández-Ruiz, Growth conditions determine different melatonin levels in Lupinus
albus L., J. Pineal Res., 2013, 55, 149–155 CrossRef CAS PubMed.
- Q. Lei, L. Wang, D. Tan, Y. Zhao, X. Zheng, H. Chen, Q. Li, B. Zuo and J. Kong, Identification of genes for melatonin synthetic enzymes in 'Red Fuji' apple (Malus domestica Borkh.cv.Red) and their expression and melatonin production during fruit development, J. Pineal Res., 2013, 55, 443–451 CAS.
- K. J. Livak and T. D. Schmittgen, Analysis of relative gene expression data using real-time quantitative PCR, Method, 2002, 25, 402–408 CrossRef PubMed.
- Y. Yin, F. Qi, L. Gao, S. Rao, Z. Yang and W. Fang, iTRAQ-based quantitative proteomic analysis of dark-germinated soybeans in response to salt stress, RSC Adv., 2018, 8, 17905–17913 RSC.
- X. Jin, R. Yang, L. Guo, X. Wang, X. Yan and Z. Gu, iTRAQ analysis of low-phytate mung bean sprouts treated with sodium citrate, sodium acetate and sodium tartrate, Food Chem., 2017, 218, 285–293 CrossRef CAS PubMed.
- Y. Yin, R. Yang, Y. Han and Z. Gu, Comparative proteomic and physiological analyses reveal the protective effect of exogenous calcium on the germinating soybean response to salt stress, J. Proteomics, 2015, 113, 110–126 CrossRef CAS PubMed.
- W. Wang, B. Vinocur and A. Altman, Plant responses to drought, salinity and extreme temperatures: towards genetic engineering for stress tolerance, Planta, 2003, 218, 1–14 CrossRef CAS PubMed.
- L. Guo, R. Yang, Y. Zhou and Z. Gu, Heat and hypoxia stresses enhance the accumulation of aliphatic glucosinolates and sulforaphane in broccoli sprouts, Eur. Food Res. Technol., 2016, 242, 107–116 CrossRef CAS.
- L. Guo, Z. Gu, X. Jin and R. Yang, iTRAQ-based proteomic and physiological analyses of broccoli sprouts in response to the stresses of heat, hypoxia and heat plus hypoxia, Plant Soil, 2017, 414, 355–377 CrossRef CAS.
- Y. Ma, P. Wang, T. Zhou, Z. Chen, Z. Gu and R. Yang, Role of Ca2+ in phenolic compound metabolism of barley (Hordeum vulgare L.) sprouts under NaCl stress, J. Sci. Food Agric., 2019, 99, 5176–5186 CrossRef CAS PubMed.
- S. Park, Y. Byeon and K. Back, Functional analyses of three ASMT gene family members in rice plants, J. Pineal Res., 2013, 55, 409–415 CAS.
- Y. Byeon, K. Lee, Y. Park, S. Park and K. Back, Molecular cloning and functional analysis of serotonin N-acetyltransferase from the cyanobacterium Synechocystis sp. PCC 6803, J. Pineal Res., 2013, 55, 371–376 CAS.
- X. Li, M. R. Uddin, W. T. Park, Y. B. Kim, J. M. Seo, S. Kim, I. Nou, J. Lee, H. Kim and S. U. Park, Accumulation of anthocyanin and related genes expression during the development of cabbage seedlings, Process Biochem., 2014, 49, 1084–1091 CrossRef CAS.
- T. Ye, X. Yin, L. Yu, S. J. Zheng, W. J. Cai, Y. Wu and Y. Q. Feng, Metabolic analysis of the melatonin biosynthesis pathway using chemical labeling coupled with liquid chromatography-mass spectrometry, J. Pineal Res., 2019, 66, 1–12 CrossRef PubMed.
- I. V. Hinkson and J. E. Elias, The dynamic state of protein turnover: it's about time, Trends Cell Biol., 2011, 21, 293–303 CrossRef CAS PubMed.
- I. F. Chang, M. Szick, S. Bailey and S. Julia, Proteomic characterization of evolutionarily conserved and variable proteins of arabidopsis cytosolic ribosomes, Plant Physiol., 2005, 137, 848–862 CrossRef CAS PubMed.
- P. Giavalisco, D. Wilson, T. Kreitler, H. Lehrach, J. Klose, J. Gobom and P. Fucini, High heterogeneity within the ribosomal proteins of the Arabidopsis thaliana 80S ribosome, Plant Mol. Biol., 2005, 57, 577–591 CrossRef CAS PubMed.
- C. M. Dobson, Protein folding and misfolding, Nature, 2003, 426, 884–890 CrossRef CAS PubMed.
- G. T. Liu, L. Ma, W. Duan, B. Wang, J. Li, H. Xu, X. Yan, B. Yan, S. Li and L. Wang, Differential proteomic analysis of grapevine leaves by iTRAQ reveals responses to heat stress and subsequent recovery, BMC Plant Biol., 2014, 14, 1–17 CrossRef PubMed.
- S. N. Shakeel, S. Aman and N. U. Haq, Proteomic and transcriptomic analyses of agave americanain response to heat stress, Plant Mol. Biol. Rep., 2013, 31, 840–851 CrossRef CAS.
- F. Han, H. Chen, X. J. Li, M. F. Yang and S. H. Shen, A comparative proteomic analysis of rice seedlings under various high-temperature stresses, Biochim. Biophys. Acta, Proteins Proteomics, 2009, 1794, 1625–1634 CrossRef CAS PubMed.
- R. Mittler, Abiotic stress, the field environment and stress combination, Trends Plant Sci., 2006, 11, 1–19 CrossRef PubMed.
- V. L. Oswaldo, B. Josef, G. Nicolas, N. Cuong, I. A. Mariel, Z. Ning, J. Trupti, X. Dong, H. Kim and W. Karl, Soybean roots grown under heat stress show global changes in their transcriptional and proteomic profiles, Front. Plant Sci., 2016, 7, 1–12 Search PubMed.
- D. G. Lee, N. Ahsan, S. H. Lee, K. Y. Kang, J. D. Bahk, I. J. Lee and B. H. Lee, A proteomic approach in analyzing heat-responsive proteins in rice leaves, Proteomics, 2010, 7, 3369–3383 CrossRef PubMed.
- A. Erika, Structural Analysis of Arabidopsis thaliana Chromosome 5. VIII. Sequence features of the regions of 1
081
958 bp covered by seventeen physically assigned P1 and TAC clones, DNA Res., 1998, 5, 379–391 CrossRef PubMed.
Footnote |
† Electronic supplementary information (ESI) available. See DOI: 10.1039/c9ra10089j |
|
This journal is © The Royal Society of Chemistry 2020 |
Click here to see how this site uses Cookies. View our privacy policy here.