DOI:
10.1039/C9RA10406B
(Paper)
RSC Adv., 2020,
10, 26709-26716
Effects of spermine on ileal physical barrier, antioxidant capacity, metabolic profile and large intestinal bacteria in piglets†
Received
11th December 2019
, Accepted 12th July 2020
First published on 17th July 2020
Abstract
Spermine, a polyamine, exerts important roles in alleviating oxidative damage, improving immunity, increasing antioxidant status and digestive enzyme activities, and promoting the development of small intestine. However, information is not available regarding the effects of spermine supplementation on gut barrier function, intestinal microbiota and metabolic profile in piglets. Therefore, this study was designed to explore the effect of spermine administration on these parameters. The experiment was conducted on twenty 12 day-old suckling piglets, which were allocated either to the group fed basal formula milk (control group) or to that fed a basal formula milk that contained spermine (0.4 mmol kg−1 BW per day) for 3 days. Caecal and colonic digesta and ileal tissues were collected at the end of the three-day feeding experiment. The results were as follows: (1) supplementation with spermine increased glutathione S-transferase (GST) capacity by 27.84% and glutathione content by 18.68% in the ileum (P < 0.05). (2) Glutathione peroxidase 1 (GPx1), catalase (CAT), GST, nuclear factor erythroid 2-related factor 2 (Nrf2) and Kelch-like ECH-associated protein1 (Keap1) mRNA levels in ileum were increased in the spermine-supplemented group in contrast to those in the control group (P < 0.05). (3) The spermine-supplemented group increased zonula occludens-1 (ZO-1) (by 42.0%), ZO-2 (by 101.0%), occludin (by 84.0%), claudin 2 (by 98.0%), claudin 3 (by 121.0%), claudin 12 (by 47.0%), claudin 14 (by 68.0%) and claudin 16 (by 73.0%) mRNA levels in ileum relative to the control group (P < 0.05). (4) Supplementation with spermine increased ZO-2 and occludin mRNA levels in ileum by reducing myosin light chain kinase (MLCK) (by 23.0%) mRNA level. (5) Spermine supplementation increased choline, glycerolphosphocholine, creatine and serine levels, and decrease alanine, glutamate, lysine, phenylalanine, threonine, lactate, tyrosine levels in ileum (P < 0.05). (6) The population of Lactobacilli, Bifidobacteria and total bacteria increased, but the number of Escherichia coli decreased in the caecal and colonic digesta after spermine supplementation (P < 0.05). In summary, dietary spermine supplementation promotes ileal health by enhancing antioxidant properties, improving ileal barrier function, modulating metabolic profiles, and maintaining large intestinal microbial homeostasis.
Introduction
Spermine is a single aliphatic biogenic amine compound and can be found in various foods, such as meat, rice and vegetables.1 Spermine has essential functions in multiple cellular physiological processes, such DNA regulation and RNA and protein synthesis, enhances immune functions in spleen and thymus, and partially modulates metabolite changes (e.g. amino acid and lipid metabolism) caused by oxidative stress, facilitates the development of the small intestine and improves growth performance in piglets.2–4 The ileum is the main intestinal segment for protein digestion, small peptide and free amino acids absorption.5 Previous experiments demonstrated that spermine promotes antioxidant defence in the liver, longissimus dorsi, spleen and thymus.4,6,7 However, few studies have investigated the effects of spermine on the antioxidant status of the ileum in pigs. Antioxidant enzymes and non-enzymatic antioxidant substances play roles in scavenging superoxide anion radicals (O2−) and hydroxyl radicals (OH−), and exert a crucial role in maintaining antioxidant status in the body. Increased antioxidant enzyme activity might be a consequence of enhanced gene transcription. However, the effects of spermine on the antioxidant enzyme gene transcription of ileum in piglets remain unclear. A previous study reported that the transcription of endogenous antioxidant enzyme genes in the spleens and thymi of pigs is usually mediated by transcription factor nuclear factor erythroid 2-related factor 2 (Nrf2).4 Nrf2 is the main target of Kelch-like ECH-associated protein1 (Keap1), which serves as a cytosolic repressor of Nrf2.8 However, information regarding the effects of spermine on the signal molecules Nrf2 and Keap1 of ileum in piglets is lacking. In addition to antioxidant status, increased the tight junction proteins (e.g., zonula occludens-1 [ZO-1] and ZO-2) improves intestinal health.9 Moreover, polyamine synthesis is inhibited in differentiated IEC-Cdx2L1 cells (Cdx2-transfected IEC-6 cells, the cell line was derived from small intestinal crypt epithelial cells of rat) by supplementation with α-difluoromethylornithine (a typical inhibitor of polyamine synthesis), which led to decreased tight junction proteins (e.g., ZO-1 and ZO-2). Moreover, this inhibitory effect is prevented by the exogenous polyamine spermidine.10 This finding implies a possible correlation between spermine and tight junction proteins in pigs. Nevertheless, the effects of spermine administration on the tight junction proteins of ileum in pigs have not been investigated. Oxidative stress can decrease intestinal barrier function.11 Microflora can reduce oxidative stress12 and contribute to the maintenance of intestinal health because they exert important roles in the increase of tight junctions,13 the maturation of the immune system and development of normal intestinal morphology.14 Infant formula supplemented with polyamines promotes the proliferation of Bifidobacteria, Lactobacilli and Enterococci in mice.15 Therefore, it could be expected that spermine intake affects intestinal bacteria colonization. A previous study reported that ileal Crohn's disease can be demonstrated to be related with alterations in bacterial carbohydrate metabolism and bacterial–host interactions.16 Intestinal microflora can secrete some metabolites, which can help intestinal development. Moreover, spermine regulates the ileum metabolic profile of rats.17 Pigs are a more effective model for humans than rats, and no information about the response of pig ileum biological systems to spermine supplementation is currently available.
Intestinal health can be reflected by physical barrier, chemical barrier, immune barrier, microbial barrier, antioxidant capacity, metabolic status, etc. Improving ileal health contributes to improving nutrients digestion and absorption and alleviating inflammatory response. In this study, we have tested the hypothesis that dietary spermine supplementation can promote intestinal health by improving intestinal barrier function, enhancing antioxidant defense, maintaining intestinal microfloral homeostasis, and promoting amino acid metabolism (e.g., protein synthesis) and lipid metabolism (e.g., lipid cholesterol transport and oxidation of fatty acids). Then, we investigated the changes in non-enzymatic antioxidant substances and antioxidant enzymes activities and the expression levels of tight junction proteins, antioxidant enzymes and signal molecules, including Keap1 and Nrf2 genes, and metabolic profile in the ileum, and microbial population in the colon and caecum of piglets that received spermine.
Material and methods
The experimental protocol used in this current work was approved by Sichuan Agricultural University Animal Care and Use Committee (SICAU-2015-056). The approximate composition analysis of the basic formula milk powder in the current study is shown in Table S1.† Nutrient levels of the basic formula milk powder were similar to sow milk, thereby meeting the nutrient requirements of piglets. These piglets were weaned at 9 d of age. After 2 d acclimatization, a total of twenty 12 day-old piglets (Duroc × Landrace × Yorkshire) with initial body weights (BWs) of 3.27–3.33 kg were divided into two groups with 10 replicates per group. Each piglet was fed 7 times a day by bottle feeding. Half of the piglets were fed formula milk containing spermine (0.4 mmol kg−1 BW) for 3 days, and the other half received the same amount of formula milk given to spermine-supplemented piglets with normal saline physiological saline once a day for 3 days in pairs. The spermine doses and spermine-treated time were chosen as described by previous experiments.6,7 The oral spermine dose of 0.4 mmol kg−1 body weight increases precocious intestinal maturation and promotes intestinal growth for 3 days without toxicity.6,7 Which may economically be provided to pigs in large-scale pig production. The health of all pigs was monitored every day. The piglets were provided ad libitum access to clean drinking water during the whole experiment. The controlled room temperature and relative humidity were controlled at approximately 30 °C and 50–60%, respectively.
Before slaughter, the piglets were fasted for 12 h. In the morning (08
:
00 h), all piglets were anaesthetized with an intravenous injection of pentobarbital sodium (15 mg kg−1 body weight) and sacrificed at 15 days of age and ileal tissues (approximately 5 cm) were collected for later enzymatic and RT-PCR analysis. Digesta contents from caecum and colon were used for analysis of microbial populations. All samples were immersed in liquid nitrogen and then stored at −80 °C for later analysis.
Biochemical analysis of ileum
To evaluate the antioxidant status in ileal tissue, antioxidant-related parameters were assessed. The malondialdehyde (MDA) serves as a biomarker of the lipid peroxidation.7 The protein carbonyl (PC) content is used to reflect the degree of proteins oxidative damage.7 MDA content was estimated by the thiobarbituric method (TBA method). PC content was tested from the peak absorbance at 370 nm according to the previous experiment.7 Decreased free radicals are partly attributed to the increased antihydroxyl radical (AHR) and antisuperoxide anion (ASA) activities.18 Glutathione (GSH) is known as a non-enzymatic antioxidant and alleviates oxidative damage. The AHR activity, ASA activity, the GSH content, as well as the total superoxide dismutase (T-SOD) activities and the total antioxidant capacity (T-AOC) were analyzed according to a previous study.18 The catalase (CAT) was evaluated using a colorimetric method. The glutathione peroxidase (GPx) activity was tested on the basis of the oxidation of reduced GSH by GPx. The glutathione S-transferase (GST) activities were spectrophotometrically determined as CAT. Enzymatic antioxidants (i.e., CAT, T-SOD, GPx and GST) participate in eliminating excessive reactive oxygen species. The activities of antioxidant enzymes were measured by using commercial kits (Jiancheng Bioengineering Institute, Nanjing, China). Enzyme activities are expressed as units (U) per milligram or gram of protein.
Total RNA extraction and quantitative RT-PCR determination
The method of the isolation of RNA from the ileal tissue was consistent with our previous study.4 Samples were collected in TRIzol to preserve RNA. The quality of total RNA was tested by using 1.0% agarose gel electrophoresis (Beckman DU-800; CA, USA). Spectrophotometric analysis (A260
:
280 nm ratio) was applied to measurement of the purity of total RNA in the ileal samples. Subsequently, total RNA (about 1 μg) in ileum was used to synthesize cDNA using the PrimeScript™ RT reagent (Takara, Dalian, China). Specific primers of the genes involved in our study were designed with Primer Express Software (version 3.0; Applied Biosystems, Foster City, CA, USA) and synthesized by Takara Biotechnology Company (Takara, Dalian, China) as summarized in Table S2.† The SYBR® Green I PCR Reagent Kit and a real-time PCR instrument (ABI 7900HT, Applied Biosystems, USA) in our laboratory were applied to real-time quantitative PCR analysis. The reaction was implemented in a total volume of 10 μL with 5 μL SYBR® Premix Ex Taq™ II with the ROX reference dye, 1 μL cDNA, 1 μL each of forward and reverse primers, and 2 μL ddH2O. Cycling conditions were as follow: a prerun step at 95 °C for 10 s, 42 cycles of denaturation at 95 °C for 10 s, annealing at 58 °C for 35 s, and extension at 72 °C for 15 s.
Microbial population determination
Bacterial DNA from the caecal and colonic digesta were isolated using the stool DNA extraction kit (Omega Bio-tek, Doraville, CA, USA) according to the manufacturer's instructions. Primers and probes for total bacteria, Escherichia coli, Lactobacillus and Bifidobacterium used in this experiment are presented in Table S2† and designed according to the latest report,19 and commercially synthesized by Life Technologies Limited. Briefly, the quantification of total bacteria was conducted by using real-time quantitative PCR using SYBR® Premix Ex Taq™ reagents (Takara Biotechnology (Dalian) Co., Ltd., Dalian, China) and CFX-96 Real-Time PCR Detection System (Bio-Rad Laboratories), and the quantification of Escherichia coli, Lactobacillus and Bifidobacterium were carried out by real-time quantitative PCR using PrimeScript™ PCR kit (Perfect Real Time; Takara) and CFX-96 Real-Time PCR Detection System (Bio-Rad Laboratories) as previously described.20,21 Standard curves were manufactured according to the previous study.21 All reactions were specific for the target species. The quantitative PCR data were normalized based on the DNA concentrations. Bacterial copies were transformed (log
10) before statistical analysis.
NMR spectroscopic measurement and data analysis
NMR spectroscopic measurement and NMR data analysis from the ileum were consistent with our previous study.17 Briefly, ileal tissues (about 60 mg) were extracted with 0.6 mL pre-cooled aqueous methanol by a tissue-lyser. The extract was then reconstituted into a 600 μL phosphate buffer (0.1 M NaH2PO4/K2HPO4, pH7.4, 50% v/v D2O). 550 μL solution was transferred into NMR tubes. The proton NMR spectra of ileum samples were acquired on a Bruker Avance II 600 MHz spectrometer. The ileum spectral region of δ0.7 to δ9.5 was integrated into regions with an equal width of 0.002 ppm by using MestreNova 8.1.2 software. Methanol signals were carefully excluded together with region containing H2O signals.
Statistical analyses
The results were expressed as mean value of each treatment. Values of P < 0.05 were used to denote statistical significant differences between treatments. Datas in the study were analyzed by SPSS 22.0 (SPSS Inc., Chicago, IL, USA). Differences between groups were determined with two-sided unpaired Student's t-tests. To evaluate the relationship between variables, correlations analysis was done using Pearson's correlation analysis. Multivariate data analysis was carried out using the software package SIMCA-P+. Principle component analysis (PCA), projection to latent structure-discriminent analysis (PLS-DA) and orthogonal projection to latent structure-discriminent analysis (OPLS-DA) were carried out. In the present study, appropriate correlation coefficients were employed as cutoff values to determine the statistical significance on the discrimination significance (P < 0.05).
Results
Antioxidant indicators in the ileum
Table 1 presents the antioxidant indicators in the ileum. The spermine supplementation increased the GST capacity (by 28%) and the GSH content (by 19%) compared with the control group (P < 0.05). However, MDA and PC contents, and the ASA, AHR, T-SOD, CAT, GPx activities, and T-AOC were not influenced by spermine addition (P > 0.05).
Table 1 Impact of spermine on antioxidant capacity in ileal tissues of pigletsa
Parametersb |
Control |
Spermine |
RMSEc |
P-Value |
Data is expressed as mean value (n = 10). AHR = anti-hydroxyl radical; ASA = anti-superoxide anion; CAT = catalase; GSH = glutathione; MDA = malondialdehyde; T-AOC = total antioxidant capacity; T-SOD = total superoxide dismutase; PC = protein carbonyl; GPx = glutathione peroxidase; GST = glutathione S-transferase. RMSE = root mean square error. |
AHR, U mg−1 protein |
177.91 |
188.33 |
14.89 |
0.495 |
ASA, U g−1 protein |
104.31 |
107.30 |
5.14 |
0.569 |
CAT, U mg−1 protein |
19.95 |
25.49 |
2.64 |
0.053 |
GSH, mg g−1 protein |
6.37 |
7.56 |
0.46 |
0.020 |
MDA, nmol mg−1 protein |
0.27 |
0.21 |
0.02 |
0.054 |
T-AOC, U mg−1 protein |
0.81 |
0.83 |
0.03 |
0.410 |
T-SOD, U mg−1 protein |
24.01 |
24.52 |
2.02 |
0.804 |
PC, nmol mg−1 protein |
4.01 |
3.69 |
0.37 |
0.414 |
GPx, U mg−1 protein |
171.65 |
175.54 |
16.53 |
0.817 |
GST, U mg−1 protein |
113.01 |
144.47 |
10.43 |
0.009 |
mRNA levels of antioxidant-related parameters
The mRNA levels of antioxidant-related genes in the ileum of piglet are presented in Table 2. The result from the current study showed that the GPx1, CAT, GR, GST, Nrf2 and Keap1 mRNA levels were increased in spermine-supplemented group compared with the control group (P < 0.05).
Table 2 Impact of spermine on the expression of antioxidant-related genes in ileal tissue of pigletsa
Parametersb |
Control |
Spermine |
RMSEc |
P-Value |
Data is expressed as mean value (n = 6). SOD1 = superoxide dismutase 1; CAT = catalase; GPx1 = glutathione peroxidase 1; GR = glutathione reductase; Nrf2 = nuclear erythroid 2-related factor 2; Keap1 = Kelch-like ECH-associatedprotein 1. RMSE = root mean square error. |
SOD1 |
1.00 |
1.13 |
0.08 |
0.161 |
GPx1 |
1.00 |
1.36 |
0.06 |
<0.001 |
CAT |
1.00 |
2.11 |
0.08 |
<0.001 |
GR |
1.00 |
1.47 |
0.09 |
<0.001 |
GST |
1.00 |
2.52 |
0.23 |
<0.001 |
Nrf2 |
1.00 |
1.36 |
0.12 |
0.011 |
Keap1 |
1.00 |
1.21 |
0.06 |
0.008 |
The expression of tissue barrier-related genes
The mRNA expression of barrier-related genes in ileum is shown in Table 3. Supplementation with spermine resulted in higher ZO-1, ZO-2, occludin, claudin 2, claudin 3, claudin 12, claudin 14 and claudin 16 mRNA levels compared with the control group (P < 0.05). Moreover, the MLCK and TNF-α gene expression level was decreased by spermine administration relative to the control group (P < 0.05).
Table 3 Impact of spermine on the expression of barrier-related genes in ileal tissue of pigletsa
Parametersb |
Control |
Spermine |
RMSEc |
P-Value |
Data is expressed as mean value (n = 6). ZO-1 = zonula occludens 1; ZO-2 = zonula occludens 2; MLCK = myosin light chain kinase. RMSE = root mean square error. |
ZO-1 |
1.00 |
1.42 |
0.08 |
0.001 |
ZO-2 |
1.00 |
2.01 |
0.09 |
<0.001 |
Occludin |
1.00 |
1.84 |
0.12 |
<0.001 |
Claudin 1 |
1.00 |
1.47 |
0.22 |
0.070 |
Claudin 2 |
1.00 |
1.98 |
0.23 |
0.002 |
Claudin 3 |
1.00 |
2.21 |
0.27 |
0.001 |
Claudin 12 |
1.00 |
1.47 |
0.08 |
<0.001 |
Claudin 14 |
1.00 |
1.68 |
0.23 |
0.014 |
Claudin 15 |
1.00 |
1.25 |
0.13 |
0.089 |
Claudin 16 |
1.00 |
1.73 |
0.21 |
0.007 |
MLCK |
1.00 |
0.77 |
0.07 |
0.008 |
TNF-α |
1.00 |
0.78 |
0.08 |
0.020 |
Impact of spermine on caecal and colonic bacteria in piglets
The caecal and colonic microbial population in pigs is shown in Table 4. The number of Lactobacilli, Bifidobacterium and total bacteria in caecal and colonic digesta of pigs fed spermine at a dose of 0.4 mmol kg−1 body weight was higher than that of the pigs fed the control diets (P < 0.05). However, the population of Escherichia coli in caecal and colonic digesta was decreased by supplementation with spermine compared with the control group (P < 0.05).
Table 4 Impact of spermine in caecal and colonic bacteria in digesta of piglets (log
10 (copies per g))a
Parameters |
Control |
Spermine |
RMSEb |
P-Value |
Data is expressed as mean value (n = 6). RMSE = root mean square error. |
Colon |
Total bacteria |
11.1 |
11.17 |
0.03 |
0.029 |
Escherichia coli |
10.19 |
9.31 |
0.06 |
<0.001 |
Lactobacillus |
6.96 |
7.09 |
0.06 |
0.042 |
Bifidobacterium |
6.69 |
7.05 |
0.13 |
0.037 |
![[thin space (1/6-em)]](https://www.rsc.org/images/entities/char_2009.gif) |
Caecum |
Total bacteria |
11.08 |
11.45 |
0.04 |
<0.001 |
Escherichia coli |
10.46 |
9.74 |
0.05 |
<0.001 |
Lactobacillus |
7.17 |
7.77 |
0.04 |
<0.001 |
Bifidobacterium |
6.75 |
7.35 |
0.07 |
<0.001 |
Multivariate data analysis of NMR data
PCA and PLS-DA were carried out on the spectral data (Fig. 1A and B, respectively). Two principal components were calculated, in which 30.4% and 27.5% of the variables were explained by PC1 and PC2, respectively. PCA results showed there was some separation between the control and spermine groups about their metabolic profiles. Furthermore, OPLS-DA strategy analyzed the metabolic changes in piglets ileum from the two groups (Fig. 1C). Compared with control group, spermine increased the betaine, choline, creatine, cytosine, formate, glycerolphosphocholine, isocytosine, scyllo-inositol, serine and taurine levels and decreased the levels of alanine, glutamate, lactate, lysine, myo-inositol, phenylalanine, threonine, tyrosine and uracil in ileum (P < 0.05) (Table 5).
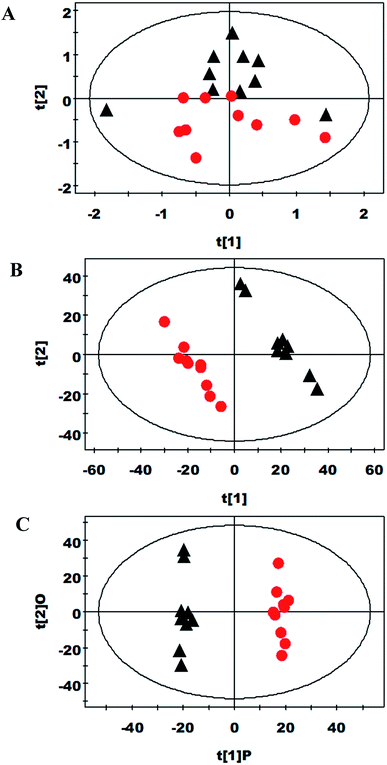 |
| Fig. 1 (A) Principle component analysis (PCA) (R2X = 0.941, Q2 = 0.728), (B) projection to latent structure-discriminent analysis (PLS-DA) (R2X = 0.19, Q2 = 0.648) and (C) orthogonal projection to latent structure-discriminent analysis (OPLS-DA) (R2X = 0.19, Q2 = 0.467) score plots based on the 1H NMR spectra of ileum metabolites derived from the control (black triangles) and spermine groups (red dots). For PLS-DA and OPLS-DA, one ileum sample from control group was excluded because it positioned outside the Hotelling's T2 ellipse on the score plot. | |
Table 5 OPLS-DA coefficients obtained from the NMR data of piglets ileum metabolites derived from the (A) control, (B) spermine groups
Metabolite |
OPLS-DA coefficient (r)a |
P valueb |
B (vs. A) |
B (vs. A) |
Correlation coefficients: positive and negative signs indicate the positive and negative correlation in the concentrations, respectively. The correlation coefficient of |r| higher than 0.602 was used as the cutoff value. Normalized integral of metabolites in the spectrum (normalized to 100). Integrals of the altered metabolites were analyzed statistically employing T test of SPSS 22.0 software (SPSS Inc., Chicago, IL, USA). The level of significance used was P < 0.05. |
Alanine |
−0.793 |
<0.05 |
Betaine |
0.712 |
<0.05 |
Creatine |
0.727 |
<0.05 |
Formate |
0.669 |
<0.05 |
Choline |
0.704 |
<0.05 |
Cytosine |
0.663 |
<0.05 |
Glutamate |
−0.742 |
<0.05 |
Isocytosine |
0.685 |
<0.05 |
Lactate |
−0.748 |
<0.05 |
Glycerolphosphocholine |
0.780 |
<0.05 |
Lysine |
−0.698 |
<0.05 |
Myo-inositol |
−0.688 |
<0.05 |
Phenylalanine |
−0.676 |
<0.05 |
Scyllo-inositol |
0.749 |
<0.05 |
Serine |
0.761 |
<0.05 |
Taurine |
0.756 |
<0.05 |
Threonine |
−0.70 |
<0.05 |
Tyrosine |
−0.758 |
<0.05 |
Uracil |
−0.882 |
<0.05 |
Discussion
The structural integrity of intestinal cells is associated with antioxidant capacity.9 Weaning stress can impair antioxidant defence of the intestine by decreasing antioxidant-related enzyme activities.22 In our previous study, we found that spermine has a potential effect against oxidative stress, promotes intestinal development and improves antioxidant defence.23 This effect was also described in nineteen-day-old male rats and twelve-day-old male piglets by Fang et al.18,23
The antioxidative effect of spermine may be derived from metal chelation or prevention of superoxide generation from tissues.24 In the present study, dietary spermine supplementation did not influence MDA and PC contents and ASA, AHR, T-SOD, CAT, GPx activities and T-AOC in ileum. The result disagree with that of our previous study, which indicated that spermine intake significantly decreases MDA and PC contents but markedly improves ASA, AHR, CAT, T-SOD, T-AOC, GPx activities in the thymi and spleens of piglets.25 The discrepancy between the results of our studies may be attributed to tissue differences. GST, a detoxification enzyme, protects cells by eliminating noxious chemical species. Results from our study showed that spermine intake increases GST activity in the ileum. The results are in accordance with those of Cao et al.,25 who showed that spermine intake enhances GST activities and effectively improves antioxidant defense in the thymus and spleen, because increased GST activity catalyzed the reaction of GSH with toxic substances produced by oxidative metabolism, thereby reducing oxidation damage.
In addition to enzymatic antioxidants, non-enzymatic antioxidants are beneficial in reducing free radicals. Thus, we examined the effect of spermine intake on GSH content in the ileal tissue of piglets. GSH (a non-enzymatic antioxidant) serves as a member of the antioxidant defence system because it detoxifies H2O2 and lipid hydroxides.26 In the present study, the GSH content in the ileum was significantly increased after spermine administration. This result suggested that spermine enhances antioxidant defence capacity at least in part because of the increase in GSH content of ileum. The finding is in line with our previous studies, in which spermine was shown to improve the GSH contents in rat jejunum and liver.6,23
Enzymatic antioxidant activity may be modulated by corresponding mRNA gene expression. Thus, we tested whether spermine administration exerts positive role in the gene expression of antioxidant enzymes in the ileum of piglets. In this study, spermine markedly increased GST mRNA levels in the ileum. This result suggests that the up-regulated expression of GST mRNA in piglets that received spermine may be the reason of increased GST activity in their ileum. Moreover, the GST is a phase II enzyme associated with GSH. One of its main functions is to catalyze the reaction of GSH with various endogenous and exogenous electrophilic compounds to produce a non-toxic or less toxic GSH sulfur conjugates.27 Therefore, enzymatic antioxidant and non-enzymatic antioxidant work together to improve antioxidant defense in body.
Increased in antioxidant enzyme gene expression is related to the up-regulated transcription of the Nrf2 gene.28 Thus, we explored the effects of spermine supplementations on the expression of genes related to signalling molecules in the ileum. Our data showed that spermine supplementation increases the transcript levels of the Nrf2 gene in the ileum, and correlation analysis indicated that the mRNA levels of GST tended to be positively correlated with Nrf2 gene expression in the ileum. These findings suggest that the benefits of spermine supplementation on the gene expression of antioxidant enzymes may be partially ascribed to the enhancement of Nrf2 mRNA expression in the ilea of piglets.
In addition to organ cellular membrane integrity, the structural integrity of ileum is affected by the tight junction proteins at the intercellular level.20 Tight junctions exert a crucial role in maintaining paracellular barrier functions29 and contain ZOs, occludin and claudins. Moreover, the up-regulation of ZO-1 and occludin contributes to the improvement of intestinal barrier function in mice.30 Polyamine can contribute to the synthesis and stability of occludin protein. Thus, polyamine can improve the epithelial barrier function.31 In the present study, supplementation with spermine increased ZO-1, ZO-2, occludin, and claudin 3 mRNA levels, indicating that spermine can help to increase intestinal physical barrier in the ileum of piglets. Moreover, the results are in line with those of Wang et al.,32 who showed that putrescine administration during the suckling period increases protein expression (e.g., occludin and claudin-3) in piglets. Furthermore, decrease in the mRNA levels of ZO-1 and occludin in human Caco-2 cells are possibly due to the up-regulation of MLCK.33 MLCK is a serine/threonine-specific protein kinase that can phosphorylate a specific myosin light chain. Spermine supplementation reduced MLCK gene expression in the present study, and correlation analysis results indicated that MLCK mRNA level is negatively correlated with ZO-2 and occludin mRNA levels in the ileum. This result suggested that spermine up-regulates ZO-2 and occludin mRNA levels by down-regulating MLCK mRNA level in the ileum. Another study showed that TNF-α is involved in the disruption of occludin transcription in human intestinal epithelial cells, thereby inducing the impairment of mucosal barrier.34 In the present study, we found that spermine results in the reduction of TNF-α gene expression level. The finding indicated that spermine improves the intestinal barrier function of the ilea of piglets by changing the expression of tight junction proteins, and this improvement may be partly attributed to the reduction of TNF-α gene expression.
Intestinal microflora play an important role in the development of normal intestinal morphology.21 A previous study reported that infant formulas supplemented with polyamines promote the proliferation of Bifidobacterium and Lactobacillus–Enterococcus bacteria in mice.15 Our results demonstrated that piglets that received spermine (0.4 mmol kg−1 BW) in their diet had increased number of Lactobacilli, Bifidobacteria and total bacteria but decreased Escherichia coli populations in their colon and ceaca. This result may be due to an indirect effect induced by the increased amount of Bifidobacterium and subsequent competitive elimination of E. coli.35 Furthermore, the intestinal microflora have been reported to regulate the expression of intestinal tight junction proteins.36 For instance, dietary Lactobacillus rhamnosus GG supplementation increases the mRNA levels of ZO-1 and occludin in the jejunal mucosae of weaned pigs.36 In the present study, the increased tight junction protein expression levels in the spermine group were consistent with the increased Lactobacillium populations in the colon and caecum. However, the piglets were fasted for 12 h before slaughter. Digesta contents from ileum of most piglets were few and were not enough for analysis of microbial population. Thus, the effect of spermine administration on intestinal microflora in the ileum was not investigated, which needs further study in the future. Furthermore, information about the specific molecular mechanisms that modulate tight junction alterations by the intestinal specific microflora remains lacking, which needs further investigation in the future.
In this study, spermine administration increased the concentration of glycerolphosphocholine (GPC) and choline of ileum. This is consistent with the result of our previous study in rats.17 GPC is synthesized from choline. Accordingly, the increase in ileum concentrations of choline is related to the enhancement in GPC levels in spermine-administrated pigs. GPC also contributes to lipid cholesterol transport and metabolism. Moreover, the ileum concentration of myo-inositol was increased after spermine administration. This carbocyclic polyol provides the structural basis of several secondary messengers (such as inositol phosphates, phosphatidylinositol, and phosphatidylinositol phosphate) in eukaryotic cells.37 Inositol is involved in the oxidation of fatty acids,38 modulation of intracellular calcium concentrations and insulin signal transduction.37 Collectively, spermine supplementation can change lipid metabolism.
Spermine supplementation can regulate amino acid metabolism. Spermine can enhance intestinal maturation; consequently, more amino acids (alanine, glutamate, lysine, phenylalanine, threonine and tyrosine) are converted into proteins, which lead to the decreased levels of amino acids present in the ileum. These results are consistent with those of the previous study: arginine can decrease serum glutamate and alanine in weaning piglets.39 Therefore, spermine supplementation can modulate intestinal amino acid metabolism.
In conclusion, spermine supplementation enhances intestinal health by increasing antioxidant properties, improving intestinal barrier function, modulating amino acid metabolism and lipid metabolism, increasing probiotics, and decreasing harmful bacteria in piglets.
Ethics statement
All procedures in the animal experiment were approved by the Animal Care and Use Committee of Sichuan Agricultural University. The experimental procedure was carried out according to the Guide for the Care and Use of Laboratory Animal of the National Research Council.
Conflicts of interest
The authors declare that they have no competing interests.
Acknowledgements
The authors are grateful to their teammates for ongoing help throughout the experiment. The authors also would like to thank Sichuan Science and Technology Program (No. 2020YJ0398) and Specific Research Supporting Program for Discipline Construction in Sichuan Agricultural University (to G. Liu, No. 03570126).
References
- A. E. Pegg and A. J. Michael, Spermine synthase, Cell. Mol. Life Sci., 2010, 67, 113–121 CrossRef CAS PubMed.
- P. Kang, M. Wang, Y. Hou, Y. Yin, B. Ding, H. Zhu, Y. Liu, Y. Qiu, D. Yi, L. Wang and J. Gong, Effects of oral administration of spermine on the development of small intestine and growth performance of weaned pigs, J. Anim. Vet. Adv., 2012, 11, 2782–2787 CrossRef CAS.
- G. Liu, T. Fang, T. Yan, G. Jia, H. Zhao, Z. Huang, X. Chen, J. Wang and B. Xue, Metabolomic strategy for the detection of metabolic effects of spermine supplementation in weaned rats, J. Agric. Food Chem., 2014, 62, 9035–9042 CrossRef CAS PubMed.
- W. Cao, X. Wu, G. Jia, H. Zhao, X. Chen, C. Wu, J. Tang, J. Wang, J. Cai and G. Liu, New insights into the role of dietary spermine on inflammation, immune function and related-signalling molecules in the thymus and spleen of piglets, Arch. Anim. Nutr., 2017, 71, 175–191 CrossRef CAS PubMed.
- A. Libao-Mercado, Y. Yin, J. van Eys and C. F. M. de Lange, True ileal amino acid digesbility and endogenous ileal amino acid losses in growing pigs fed wheat shorts- or casein-based diets, J. Anim. Sci., 2006, 84, 1351–1361 CrossRef CAS PubMed.
- G. Liu, W. Cao, G. Jia, H. Zhao, X. Chen, J. Wang and C. Wu, Effect of spermine on liver and spleen antioxidant status in weaned rats, Anim. Feed Sci. Technol., 2016, 25, 335–342 CrossRef.
- T. Fang, J. Zheng, W. Cao, G. Jia, H. Zhao, X. Chen, J. Cai, J. Wang and G. Liu, Effects of spermine on the antioxidant status and gene expression of antioxidant-related signaling molecules in the liver and longissimus dorsi of piglets, Animal, 2018, 12, 1208–1216 CrossRef CAS PubMed.
- S. A. Reisman, R. L. Yeager, M. Yamamoto and C. D. Klaassen, Increased Nrf2 activation in livers from Keap1-knockdown mice increases expression of cytoprotective genes that detoxify electrophiles more than those that detoxify reactive oxygen species, Toxicol. Sci., 2009, 108, 35–47 CrossRef CAS PubMed.
- H. Wen, L. Feng, W. Jiang, Y. Liu, J. Jiang, S. Li, L. Tang, Y. Zhang, S. Kuang and X. Zhou, Dietary tryptophan modulates intestinal immune response, barrier function, antioxidant status and gene expression of TOR and Nrf2 in young grass carp (Ctenopharyngodon idella), Fish Shellfish Immunol., 2014, 40, 275–287 CrossRef CAS PubMed.
- X. Guo, J. N. Rao, L. Liu, T. Zou, K. M. Keledjian, D. Boneva, B. S. Marasa and J. Y. Wang, Polyamines are necessary for synthesis and stability of occludin protein in intestinal epithelial cells, Am. J. Physiol.: Gastrointest. Liver Physiol., 2005, 288, G1159–G1169 CrossRef CAS PubMed.
- S. Cao, H. Wu, C. Wang, Q. Zhang, L. Jiao, F. Lin and C. H. Hu, Diquat-induced oxidative stress increases intestinal permeability, impairs mitochondrial function, and triggers mitophagy in piglets, J. Anim. Sci., 2018, 96, 1795–1805 CrossRef PubMed.
- C. B. Forsyth, A. Farhadi, S. M. Jakate, Y. Tang, M. Shaikh and A. Keshavarzian, Lactobacillus GG treatment ameliorates alcohol-induced intestinal oxidative stress, gut leakiness, and liver injury in a rat model of alcoholic steatohepatitis, Alcohol, 2009, 43, 163–172 CrossRef CAS PubMed.
- Y. Wu, H. Xu, X. Cao, R. Liu, L. Tang, Z. Zeng and W. Li, Bacillus amyloliquefaciens ameliorates H2O2-induced oxidative damage by regulating transporters, tight junctions, and apoptosis gene expression in cell line IPEC-1, Probiotics Antimicrob. Proteins, 2020, 12, 649–656 CrossRef CAS PubMed.
- I. I. Ivanov and D. R. Littman, Modulation of immune homeostasis by commensal bacteria, Curr. Opin. Microbiol., 2011, 14, 106–114 CrossRef CAS PubMed.
- C. Gómez-Gallego, M. C. Collado, T. Ilo, U. M. Jaakkola, M. J. Bernal, M. J. Periago, S. Salminen, G. Ros and R. Frias, Infant formula supplemented with polyamines alters the intestinal microbiota in neonatal BALB/cOlaHsd mice, J. Nutr. Biochem., 2012, 23, 1508–1513 CrossRef PubMed.
- A. R. Erickson, B. L. Cantarel, R. Lamendella, Y. Darzi, E. F. Mongodin, C. Pan, M. Shah, J. Halfvarson, C. Tysk, B. Henrissat, J. Raes, N. C. Verberkmoes, C. M. Fraser, R. L. Hettich and J. K. Jansson, Integrated metagenomics/metaproteomics reveals human host-microbiota signatures of Crohn's disease, PLoS One, 2012, 7, e49138 CrossRef CAS PubMed.
- G. Liu, T. Yan, T. Fang, G. Jia, X. Chen, H. Zhao, J. Wang and C. Wu, Nutrimetabolomic analysis provides new insights into spermine-induced ileum-system alterations for suckling rats, RSC Adv., 2015, 5, 48769–48778 RSC.
- T. Fang, G. Liu, W. Cao, X. Wu, G. Jia, H. Zhao, X. Chen, C. Wu and J. Wang, Spermine: new insights into the intestinal development and serum antioxidant status of suckling piglets, RSC Adv., 2016b, 6, 31323–31335 Search PubMed.
- X. Wu, D. Chen, B. Yu, Y. Luo, P. Zheng, X. Mao, J. Yu and J. He, Effect of different dietary non-starch fiber fractions on growth performance, nutrient digestibility, and intestinal development in weaned pigs, Nutrition, 2018, 51–52, 20–28 CrossRef CAS PubMed.
- H. Chen, D. Chen, J. Michiels and S. De Smet, Dietary fiber affects intestinal mucosal barrier function by regulating intestinal bacteria in weaning piglets, Commun. Agric. Appl. Biol. Sci., 2013, 78, 71–78 CAS.
- J. Wan, F. Jiang, Q. Xu, D. Chen, B. Yu, Z. Huang, X. Mao, J. Yu and J. He, New insights into the role of chitosan oligosaccharide in enhancing growth performance, antioxidant capacity, immunity and intestinal development of weaned pigs, RSC Adv., 2017, 7, 9669–9679 RSC.
- L. H. Zhu, K. L. Zhao, X. L. Chen and J. X. Xu, Impact of weaning and an antioxidant blend on intestinal barrier function and antioxidant status in pigs, J. Anim. Sci., 2012, 90, 2581–2589 CrossRef CAS PubMed.
- T. Fang, G. Jia, H. Zhao, X. Chen, J. Tang, J. Wang and G. Liu, Effects of spermine supplementation on the morphology, digestive enzyme activities, and antioxidant capacity of intestine in weaning rats, Anim. Nutr., 2016, 2, 370–375 CrossRef PubMed.
- E. LØvaas, Hypothesis: spermine may be an important epidermal antioxidant, Med. Hypotheses, 1995, 45, 59–67 CrossRef.
- W. Cao, X. Xu, G. Jia, H. Zhao, X. Chen, C. Wu, J. Tang, J. Wang, J. Cai and G. Liu, Roles of spermine in modulating the antioxidant status and Nrf2 signalling molecules expression in the thymus and spleen of suckling piglets-new insight, J. Anim. Physiol. Anim. Nutr., 2018, 102, e183–e192 CrossRef CAS PubMed.
- C. C. Yang, J. Y. Fang, T. L. Hong, T. C. Wang, Y. E. Zhou and T. C. Lin, Potential antioxidant properties and hepatoprotective effects of an aqueous extract formula derived from three Chinese medicinal herbs against CCl4-induced liver injury in rats, Int. Immunopharmacol., 2013, 15, 106–113 CrossRef CAS PubMed.
- M. Y. Hung, T. Y. Fu, P. H. Shih, C. P. Lee and G. C. Yen, Du-Zhong (Eucommia ulmoides Oliv.) leaves inhibits CCl4-induced hepatic damage in rats, Food Chem. Toxicol., 2006, 44, 1424–1431 CrossRef CAS PubMed.
- D. D. Zhang, Mechanistic studies of the Nrf2–Keap1 signaling pathway, Drug Metab. Rev., 2006, 38, 769–789 CrossRef CAS PubMed.
- J. R. Turner, Intestinal mucosal barrier function in health and disease, Nat. Rev. Immunol., 2009, 9, 799–809 CrossRef CAS PubMed.
- X. Sun, H. Yang, K. Nose, S. Nose, E. Q. Haxhija, H. Koga, Y. Feng and D. H. Teitelbaum, Decline in intestinal mucosal IL-10 expression and decreased intestinal barrier function in a mouse model of total parenteral nutrition, Am. J. Physiol.: Gastrointest. Liver Physiol., 2008, 294, G139–G147 CrossRef CAS PubMed.
- X. Guo, J. N. Rao, L. Liu, T. Zou, K. M. Keledjian, D. Boneva, B. S. Marasa and J. Y. Wang, Polyamines are necessary for synthesis and stability of occludin protein in intestinal epithelial cells, Am. J. Physiol.: Gastrointest. Liver Physiol., 2005, 288, G1159–G1169 CrossRef CAS PubMed.
- J. Wang, G. R. Li, B. E. Tan, X. Xiong, X. F. Kong, D. F. Xiao, L. W. Xu, M. M. Wu, B. Huang, S. W. Kim and Y. L. Yin, Oral administration of putrescine and proline during the suckling period improves epithelial restitution after early weaning in piglets, J. Anim. Sci., 2015, 93, 1679–1688 CrossRef CAS PubMed.
- L. Nahidi, S. T. Leach, D. A. Lemberg and A. S. Day, Osteoprotegerin exerts its pro-inflammatory effects through nuclear factor-κB activation, Dig. Dis. Sci., 2013, 58, 3144–3155 CrossRef CAS PubMed.
- J. Mankertz, S. Tavalali, H. Schmitz, A. Mankertz, E. O. Riecken, M. Fromm and J. D. Schulzke, Expression from the human occludin promoter is affected by tumor necrosis factor alpha and interferon gamma, J. Cell Sci., 2000, 113, 2085–2090 CAS.
- C. M. Yang, P. R. Ferket, Q. H. Hong, J. Zhou, G. T. Cao, L. Zhou and A. G. Chen, Effect of chito-oligosaccharide on growth performance, intestinal barrier function, intestinal morphology and cecal microflora in weaned pigs, J. Anim. Sci., 2012, 90, 2671–2676 CrossRef CAS PubMed.
- X. Mao, C. Gu, H. Hu, J. Tang, D. Chen, B. Yu, J. He, J. Yu, J. Luo and G. Tian, Dietary lactobacillus rhamnosus gg supplementation improves the mucosal barrier function in the intestine of weaned piglets challenged by porcine rotavirus, PLoS One, 2016, 11, e0146312 CrossRef PubMed.
- G. Di Paolo and P. De Camilli, Phosphoinositides in cell regulation and membrane dynamics, Nature, 2006, 443, 651–657 CrossRef CAS PubMed.
- R. A. Siddiqui, K. A. Havey and G. P. Zaloga, Modulation of enzymatic activities by n − 3 polyunsaturated fatty acids to support cardiovascular health, J. Nutr. Biochem., 2008, 19, 417–437 CrossRef CAS PubMed.
- Q. He, H. Tang, P. Ren, X. Kong, G. Wu, Y. Yin and Y. Wang, Dietary supplementation with L-arginine partially conteracts serum metabonome induced by weaning stress in piglets, J. Proteome Res., 2011, 10, 5214–5221 CrossRef CAS PubMed.
Footnote |
† Electronic supplementary information (ESI) available. See DOI: 10.1039/c9ra10406b |
|
This journal is © The Royal Society of Chemistry 2020 |
Click here to see how this site uses Cookies. View our privacy policy here.