DOI:
10.1039/C9RA10847E
(Paper)
RSC Adv., 2020,
10, 6563-6570
Gel properties and flavor characteristics of blended anchovy (Engraulis japonicus) mince and silver carp (Hypophthalmichthys molitrix) surimi
Received
24th December 2019
, Accepted 28th January 2020
First published on 12th February 2020
Abstract
This paper aims to research the gel properties and flavor characteristics of anchovy mince and silver carp surimi blended in different ratios (0/100, 10/90, 20/80, 30/70, 40/60 and 100/0). When the ratios of anchovy mince to silver carp surimi were 10/90 and 20/80, the gel strength of the blended systems increased significantly from 4629.17 g mm to 7568.01 g mm and 6804.22 g mm, respectively. Under these two ratios, the water-holding capacity of the blended systems increased significantly from 56.43% to 71.82% and 69.36%, respectively. In addition, the blended systems formed a highly uniform and dense network structure. At ratios of 0/100, 10/90 and 20/80, the myosin heavy chain was cross-linked, resulting in the formation of macromolecular aggregates. With the increase in anchovy mince content, the brightness and whiteness of the blended systems decreased gradually. When the ratio of anchovy mince to silver carp surimi was 10/90, the sensory evaluation score of the blended system was the highest and the bitterness score the lowest. Under this ratio, the relative contents of esters and aldehydes were the highest, while those of alcohols and ketones were the lowest. In conclusion, the optimum sample formulation contained a 10/90 ratio of anchovy mince to silver carp surimi.
1. Introduction
With the accelerating pace of life, the annual demand for surimi-based products worldwide has increased, especially in China, from 819
100 tons in 2008 to 1
455
460 tons in 2018.1 The main raw material of surimi products is large marine fish resources. However, marine fish resources are increasingly scarce. Low-value fish with large catches and small individuals is mostly used to produce feed. The waste of resources is serious and the added value is low.2 If low-value fish is processed into surimi-based product, the added value is greatly increased.
Anchovy (Engraulis japonicus) is a small marine pelagic fish that feeds on zooplankton. The larger adult fish weighs about 10 g and measure about 10 cm.3 In the past 10 years, the amounts of caught anchovy have increased to more than 1.2 million tons worldwide annually.4 Anchovy is not only rich in resources, but also high in nutritional value. It contains a variety of fatty acids and mineral elements.5 Currently, anchovy is utilized mainly as processed and manufactured fish meal and for the extraction of fish oil.6 However, the potential for anchovy is not fully exploited. Appropriate processing methods could increase its range of applications.
Silver carp (Hypophthalmichthys molitrix) is one of the four most popular freshwater fish species in China. Over the past decade, more than four million tons of silver carp have been caught worldwide, annually.4 Silver carp is exploited because of its abundant yield. Processing silver carp into surimi is a good strategy. However, silver carp is very difficult to gelation, easy to gel and deteriorate.7 The flavor characteristics of silver carp surimi are similar to those of living fish, with a strong fishy, earthy smell.8 Therefore, improving the gel properties and flavor characteristics of silver carp surimi has become the focus of attention.
Many scholars have investigated the gel properties of blended surimi; however, few studies have analyzed its flavor characteristics. Paker et al.9 added the sarcoplasmic protein extracted from silver carp to the Alaska pollock (Theragra chalcogramma) surimi, resulting in significant improvement in brightness and whiteness of the blended surimi gel. Yu et al.7 demonstrated that the breaking force, the breaking distance, and the gel strength of the blended silver carp and the golden threadfin bream (Nemipterus virgatus) surimi were significantly higher than those of single surimi-based product. Amiza et al.10 mixed surimi and silvery catfish in a 40
:
60 ratio to generate a pure surimi gel cost-effectively.
This study investigated the gel properties and flavor characteristics of blended anchovy mince and silver carp surimi. The goal was to optimally utilize the anchovy and improve the gel properties and flavor characteristics of silver carp surimi. It is hoped that this study can understand the change rules of gel properties and flavor characteristics of blended surimi, so as to provide ideas for improving the performance of silver carp surimi-based product.
2. Materials and methods
2.1 Raw materials
Silver carp surimi (AAA grade) was obtained from Honghu Jingli Aquatic Food Co., LTD. (Honghu, China). Frozen anchovy was obtained from Dalian Xinrong Fisheries Market (Dalian, China).
2.2 Anchovy mince
The frozen anchovy was thawed under streaming water. Anchovy was processed (by removing the head, tail, viscera, and skin) to obtain mince. The mince was chopped at low temperatures (<10 °C) uniformly, and stored at −80 °C.
2.3 Amino acid
The amino acid composition and content of anchovy mince and silver carp surimi were analyzed.11
2.4 Gel properties
2.4.1 Blended proportions. The experimental variables included the following ratios of anchovy mince to silver carp surimi: 0/100, 10/90, 20/80, 30/70, 40/60 and 100/0 (w/w).
2.4.2 Gel preparation. To prepare the gels, frozen silver carp surimi was partially thawed at 4 °C for 6 h and cut into small pieces (about 2.5 cm3). The anchovy mince and silver carp surimi were mixed in different ratios as indicated above. First, the mixture was chopped in a vacuum chopper pot (UMC5, Stephan, Germany) for 3 min, and for another 3 min following the addition of 2.5% NaCl. Finally, the moisture content of the paste was adjusted to 78% and chopped for 5 min under vacuum (−0.6 Pa). The temperature of the paste was maintained below 10 °C. The paste was stuffed into commercial 25 mm cellulose casings using a sausage stuffer. After setting, the paste was heated in a water bath (at 40 °C for 30 min and at 90 °C for 20 min). After heating, the gels were cooled promptly in cool water and then stored at 4 °C overnight until further analysis.
2.4.3 Gel strength. Samples were placed at room temperature (25 °C) for 30 min. The gels were cut into 2.0 cm-high cylindrical specimens.12 Gel strength was measured using a TA-XT Plus texture analyzer (Stable Micro Systems, UK) under the following settings: probe type, P/5s; pre-test speed, 1 mm s−1; test speed, 1 mm s−1; post-test speed, 1 mm s−1; compression distance, 15 mm; and trigger force, 10 g.
2.4.4 Whiteness. The L* (lightness), a* (redness/greenness) and b* (yellowness/blueness) of the sample were determined using a CR-400 colorimeter (Minolta, Japan). The formula for calculation of the whiteness was as follows:13
Whiteness = 100 − [(100 − L*)2 + a*2 + b*2]½ |
2.4.5 Water-holding capacity. The sample was cut into 5 mm cylindrical slices, and the quality was M1. The gel slice was wrapped in three-layer filter papers and transferred into a 50 mL centrifuge tube. The sample was centrifuged at 3000 rpm for 15 min at 4 °C (Thermo, US). After centrifugation, the gel sample was re-weighed, and the quality was denoted as M2. The formula for calculating water-holding capacity (WHC) was as follows:14
2.4.6 Microstructure. Microstructure was performed according to Oujifard.15 The sample was cut into cubes (3 mm × 3 mm × 3 mm), and fixed in 2.5% (w/w) glutaraldehyde solution for 24 h. After removing the stationary liquid, it was rinsed three times with phosphate buffer (0.2 mol L−1, pH 7.2) for 15 min each time followed by rinsing with deionized water for 1 h and gradient dehydration with 50%, 70% and 90% ethanol solution for 15 min. Finally, it was rinsed with absolute ethanol for 10 min, and the rinsing was repeated three times. The microstructure of the sample was analyzed via ion sputtering and observed using a scanning electron microscopy (S-4800, Hitachi, Japan) (×1000).
2.4.7 Sodium dodecyl sulphate-polyacrylamide gel electrophoresis. Sodium dodecyl sulphate-polyacrylamide gel electrophoresis (SDS-PAGE) was performed according to Jaczynski.16 The 3.0 g sample was chopped and mixed with 27 mL of 5% (w/v) SDS solution. The sample was homogenized with a high-speed homogenizer for 4 min and heated at 85 °C for 1 h. After cooling, the sample was centrifuged at 11
000 rpm for 15 min at 4 °C, and the supernatant was obtained. The concentration of supernatant was adjusted to 3 mg mL−1, mixed with the buffer (code no. 9172, Takara Bio, China) at a ratio of 1
:
1 (v/v), and boiled for 20 min. A 4% concentrated gum (including 30% Acr/Bis, ultrapure water, 0.5 M Tris–HCl, 10% SDS, 10% APS, TEMED) and 12% separated gum (including 30% Acr/Bis, ultrapure water, 1.5 M Tris–HCl, 10% SDS, 10% APS, TEMED) were used to prepare the gum (Bio-Rad, US). Finally, the electrophoresis map was analyzed by Image software.
2.5 Flavor characteristics
2.5.1 Sensory evaluation. The sensory evaluation of six sample formulations was carried out, and the scores ranged from 1 (dislike extremely) to 5 (like extremely). The smell, color, shape and taste of each sample were evaluated.10
2.5.2 Electronic nose analysis. Electronic nose was performed according to Tian.17 A 5 g sample was cut and transferred into a centrifuge tube. The sample was incubated at room temperature (25 °C) for 30 min. The parameters for measurements were as follows: headspace temperature, 25 °C; internal flow rate and sample flow rate, 300 mL min−1; determination time, 120 s; and cleaning time, 100 s. For electronic nose data analysis, a WinMuster software (PEN3, Airsense, Germany) was used to conduct the principal component analysis (PCA).
2.5.3 Electronic tongue analysis. Electronic tongue was performed according to Liu.18 The sample was cut and added to a food processor. Samples were heated until the central temperature reached 40 °C. A 50 g sample was weighed accurately, and distilled water was added at 40 °C at the ratio of 1
:
5 (m/m). The sample was homogenized with a high-speed homogenizer for 1 min. Finally, the sample was centrifuged at 3000 rpm for 15 min, and a supernatant was obtained. The supernatant was diluted for determination. The samples were measured and analyzed using an electronic tongue (SA 402B, Insent, Japan).
2.5.4 Gas chromatography-mass spectrometry. Gas chromatography-mass spectrometry (GC-MS) was performed according to Ansorena.19 A 4 g sample was cut and weighed accurately, and placed in a headspace bottle followed by the addition of a 4 mL saturated NaCl solution. It was heated for 15 min at 55 °C on a magnetic stirrer. Headspace extraction was performed for 30 min with 50/30 μm activated DVB/CAR/PDMS (7890N-5975C, Agilent, US) followed by removal of the extraction head and analysis using GC-MS for 5 min. Finally, it was analyzed and identified by GC-MS.
2.6 Statistical analysis
The experimental data were analyzed and processed using the SPSS 19.0 software (average differences were evaluated by a Tukey's test using a 95% confidence interval), and plotted with Origin 9.0 software. Each group of samples was measured three times in parallel.
3. Results and discussion
3.1 Amino acid
Table 1 shows the amino acid composition and content of anchovy mince and silver carp surimi. The main amino acids of anchovy mince were Glu, Asp, Lys, Leu and Arg. The composition and content of main amino acids of silver carp surimi were consistent with those of anchovy mince.20 The main umami amino acids were Glu and Asp21 which accounted for 15.01% and 14.95% of anchovy mince and silver carp surimi, respectively. The main bitterness amino acids were Val, Met, Iso, Leu, Phe, Lys, His and Arg,22 which constituted 25.29% and 21.71% of anchovy mince and silver carp surimi, respectively. This index provides a basis for the gel properties and flavor characteristics.
Table 1 Amino acid composition and content (dry matter, g/100 g) of anchovy mince and silver carp surimia
AA composition |
Anchovy mince |
Silver carp surimi |
AA denotes amino acid. |
Aspartic (Asp) |
5.45 ± 0.12 |
5.14 ± 0.19 |
Glutamic (Glu) |
9.56 ± 0.21 |
9.81 ± 0.34 |
Serine (Ser) |
2.15 ± 0.14 |
2.12 ± 0.07 |
Glycine (Gly) |
1.82 ± 0.07 |
2.04 ± 0.12 |
Alanine (Ala) |
3.47 ± 0.09 |
2.82 ± 0.11 |
Tyrosine (Tyr) |
1.92 ± 0.05 |
1.68 ± 0.05 |
Proline (Pro) |
1.73 ± 0.03 |
1.74 ± 0.09 |
Threonine (Thr) |
2.60 ± 0.05 |
2.36 ± 0.07 |
Valine (Val) |
3.90 ± 0.12 |
2.49 ± 0.08 |
Methionine (Met) |
1.93 ± 0.14 |
1.58 ± 0.05 |
Isoleucine (Iso) |
2.46 ± 0.09 |
2.33 ± 0.08 |
Leucine (Leu) |
5.24 ± 0.19 |
4.25 ± 0.15 |
Phenylalanine (Phe) |
2.11 ± 0.11 |
1.86 ± 0.07 |
Lysine (Lys) |
3.46 ± 0.21 |
4.94 ± 0.17 |
Histidine (His) |
2.82 ± 0.10 |
1.03 ± 0.04 |
Arginine (Arg) |
3.37 ± 0.15 |
3.23 ± 0.13 |
3.2 Gel properties
3.2.1 Gel strength. The gel strength is a product of breaking force and breaking distance. The breaking force reflects the firmness of surimi gel, and the breaking distance reflects the binding between surimi proteins.23 Gel strength is a major indicator of the hardness and elastic properties of surimi. The changes in breaking force, breaking distance and gel strength of blended anchovy mince and silver carp surimi are shown in Fig. 1.
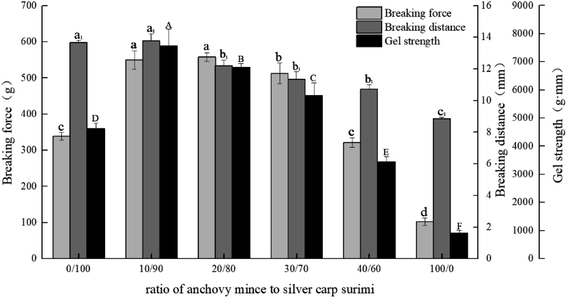 |
| Fig. 1 Changes in breaking force, breaking distance, and gel strength of blended anchovy mince and silver carp surimi. a–d Means ± SD represents the significance between breaking forces or WHCs (p < 0.05); a1–c1 means ± SD represents the significance between breaking distances (p < 0.05); A−F means ± SD represents the significance between gel strengths (p < 0.05). | |
According to Fig. 1, the breaking force, breaking distance, and gel strength of silver carp surimi gel were higher than those of anchovy mince. With increase in anchovy mince content, the breaking force and gel strength of the blended systems increased initially and then decreased eventually. When the ratios of anchovy mince to silver carp surimi were 10/90, 20/80 and 30/70, the gel strength of the blended systems increased significantly compared with that of the silver carp surimi gel (p < 0.05), and increased from 4629.17 g mm to 7568.01 g mm, 6804.22 g mm and 5805.38 g mm, respectively. When the ratio of anchovy mince to silver carp surimi was 40/60, the gel strength of the blended system was lower than that of pure silver carp surimi.
The protein was separated from kilka and silver carp using the pH-shift method, the gel strength of the blended protein was enhanced at a ratio of 1
:
1 (w/w). This is due to the exposure of active sulfhydryl and hydrophobic groups caused by the unfolding of some proteins.24 When the croaker-mackerel surimi blend was mixed at a ratio of 1
:
2 (w/w), it yielded a similar gel strength of blended surimi and mackerel surimi.25 The results of this experiment were similar to those reported in the literature. The gel strength of the anchovy mince was not favorable; however, it partly replaced the silver carp surimi, resulting in higher gel strength and reduce the cost of surimi product.
3.2.2. Whiteness. Whiteness is the key index reflecting the color of surimi. The whiteness of the gel is mainly affected by the protein composition, the protein profile and the optical characteristics of the particles.26 The changes in whiteness of blended anchovy mince and silver carp surimi are shown in Table 2. With increased in anchovy mince content, the L* and whiteness of the blended systems decreased significantly compared with that of the silver carp surimi gel (p < 0.05), from 77.79 to 60.10, and from 75.71 to 57.91, respectively. When the anchovy content increased gradually, a* and b* of the blended systems increased significantly (p < 0.05), from −5.04 to −1.05, and from 15.7 to 13.28, respectively. Mince has a lower brightness than surimi, because of higher myoglobin content.27
Table 2 Changes in whiteness of blended anchovy mince and silver carp surimia
A/S |
L* |
a* |
b* |
Whiteness |
A denotes anchovy mince, and S denotes silver carp surimi. A/S indicates the ratio of anchovy mince to silver carp surimi. a–f Means ± SD that have no superscript in common within a column are significantly different from each other (p < 0.05). |
0/100 |
77.79 ± 0.48a |
−5.04 ± 0.05f |
8.44 ± 0.22e |
75.71 ± 0.36a |
10/90 |
73.94 ± 0.30b |
−3.99 ± 0.10e |
10.70 ± 0.14d |
71.55 ± 0.30b |
20/80 |
71.36 ± 0.67c |
−3.15 ± 0.10d |
12.08 ± 0.22c |
68.76 ± 0.62c |
30/70 |
69.51 ± 0.35d |
−2.69 ± 0.02c |
12.81 ± 0.35b |
66.81 ± 0.33d |
40/60 |
68.19 ± 0.87e |
−1.97 ± 0.11b |
13.53 ± 0.26a |
65.37 ± 0.73e |
100/0 |
60.10 ± 2.63f |
−1.05 ± 0.53a |
13.28 ± 0.81a |
57.91 ± 2.27f |
The current study suggests that the whiteness of blended (silver carp and white croaker) surimi was negatively correlated with gel strength probably due to the absorption of visible light by the compact myofibrillar matrix.28 The experimental results were similar to those of this experiment. However, a few studies indicated that the whiteness of blended surimi gel was positively correlated with gel strength. The muscle protein of silver carp was added to Alaska pollock surimi, and the brightness and whiteness of the blended sample were significantly improved compared with the myofibrillar protein in silver carp,9 which was contrary to our findings.
3.2.3 WHC. The WHC is important physical indices of surimi gel quality, and together reflect the water retention capacity of surimi gel. The high WHC indicate that the gel network strongly combined with water, and decreased the water loss rate.29 The changes in WHC of blended anchovy mince and silver carp surimi are shown in Fig. 2.
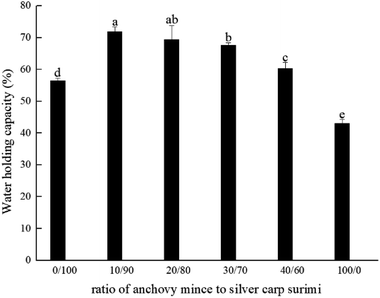 |
| Fig. 2 Changes in water-holding capacity of blended anchovy mince and silver carp surimi. a–d Means ± SD represents the significance between WHCs (p < 0.05). | |
As shown in Fig. 2, the anchovy mince had the lowest WHC. With increased anchovy mince content, the WHC of the blended systems improved significantly (p < 0.05). When the ratios of anchovy mince to silver carp surimi were 10/90 and 20/80, the WHC of the blended systems increased significantly compared with that of the silver carp surimi gel (p < 0.05), from 56.43% to 71.82% and 69.36%, respectively. With the increase in anchovy mince content, the crosslinking network structure of the blended systems was further enhanced. The compact microstructure may hinder the outflow of water and increase the WHC.30 The WHC of silver carp surimi was improved by blending with Nemipterus virgatus surimi.7 The WHC of mackerel surimi was improved by blending with croaker surimi.25 The results suggest that the silver carp surimi can be partially replaced by anchovy mince, thereby enhancing the WHC of the blended systems, which was consistent with previous results of gel strength.
3.2.4 Microstructure. The microstructure of surimi gel comprises mostly gel network structure. The density and pore size of the surimi gel are related to gel strength, WHC, and cooking loss rate. The changes in microstructure of blended anchovy mince and silver carp surimi are shown in Fig. 3.
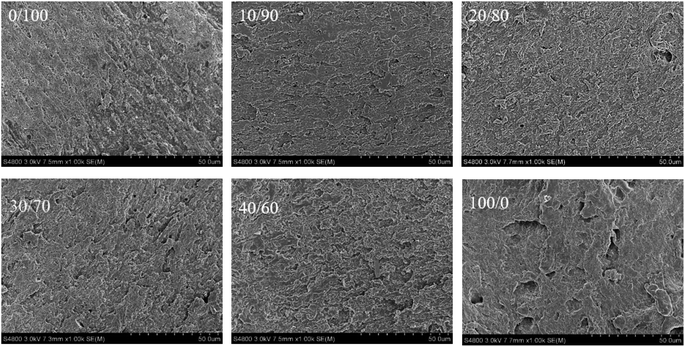 |
| Fig. 3 Changes in microstructure of blended anchovy mince and silver carp surimi (×1000). A denotes anchovy mince, and S denotes silver carp surimi. A/S indicates the ratio of anchovy mince to silver carp surimi. | |
As shown in Fig. 3, the microstructure of anchovy mince, silver carp surimi and blended systems showed obvious differences. The microstructure of silver carp surimi gel was uneven, rough and lacked an ordered network structure. In comparison, the microstructure of the blended systems was relatively smooth, homogeneous, and contained small pores. With the increase in anchovy mince content, the blended systems gradually formed a denser network structure. Especially, at ratios of 10/90 and 20/80, the blended systems formed highly uniform and orderly three-dimensional network structure. The dense gel network structure of surimi gel facilitated tight binding of additional free water, increasing the WHC and gel strength.
3.2.5 SDS-PAGE. The protein patterns of surimi gel mainly include myosin heavy chain (MHC), actin (AC) and tropomyosin (TM). The MHC is the main contributor of the myofibrillar protein to the gel capacity. The higher the content of MHC, the higher is the gel capacity of surimi. The changes in SDS-PAGE pattern of blended anchovy mince and silver carp surimi are shown in Fig. 4.
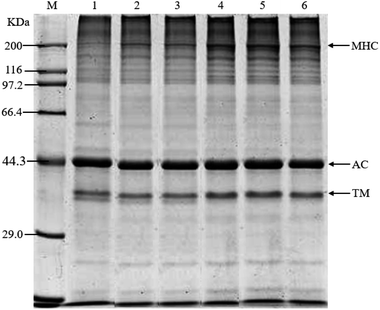 |
| Fig. 4 Changes in SDS-PAGE pattern of blended anchovy mince and silver carp surimi. A denotes anchovy mince, and S denotes silver carp surimi. A/S indicates the ratio of anchovy mince to silver carp surimi. M-mark; 1-0/100; 2-10/90; 3-20/80; 4-30/70; 5-40/60; 6-100/0. | |
As shown in Fig. 4, with the increase in anchovy mince content, the MHC of the blended systems gradually increased in depth. When the ratios of anchovy mince to silver carp surimi were 10/90 and 20/80, the MHC strips of the blended systems were the thinnest and the lightest. The MHC strip of the anchovy mince was the deepest. That was because the MHC of the gel sample with adequate gel strength undergoes crosslinking via salt bridges, forming larger aggregates, which do not enter the separation gel, thereby explaining the relatively shallow MHC strip.31 These findings were consistent with previous results.
3.3 Flavor characteristics
3.3.1 Sensory evaluation. Six groups of samples were assessed and scored by 20 persons. Among the 20 students, there are 10 men and 10 women, and they come from different regions and have rich professional knowledge. The results are displayed in Table 3, which show higher scores when the ratios of anchovy mince to silver carp surimi were 10/90 and 20/80. As shown in Table 3, when the content in anchovy mince increased gradually, the smell value of the blended systems increased significantly (p < 0.05), which may be due to the fact that anchovy was a sea fish, with a better flavor than that of freshwater fish. Color is an important quality parameter of surimi-based product quality, and assessors depend on their vision to assess the color of the sample. The deeper the color of the blended systems, the lower is the acceptability of the assessors. With the increase in anchovy mince content, the shape value of the blended systems first increased and then decreased. The shape value of the blended systems was consistent with the previous gel strength. The taste value of the blended systems was consistent with smell and shape changes. The result of sensory evaluation showed that the partial substitution of anchovy mince for silver carp surimi effectively improved the flavor of surimi-based product.
Table 3 Changes in sensory evaluation of blended anchovy mince and silver carp surimia
A/S |
Smell |
Color |
Shape |
Taste |
Score |
A denotes anchovy mince, and S denotes silver carp surimi. A/S indicates the ratio of anchovy mince to silver carp surimi. a–d Means ± SD that have no superscript in common within a column are significantly different from each other (p < 0.05). |
0/100 |
3.00 ± 1.00b |
5.00 ± 0.00a |
4.33 ± 0.58ab |
2.67 ± 0.58b |
13.67 ± 0.58c |
10/90 |
4.33 ± 0.58a |
4.33 ± 0.58a |
5.00 ± 0.00a |
4.33 ± 0.57a |
17.33 ± 0.57a |
20/80 |
4.33 ± 0.57a |
3.33 ± 0.57b |
4.33 ± 0.57ab |
4.33 ± 0.57a |
15.67 ± 0.57b |
30/70 |
4.50 ± 0.00a |
2.67 ± 0.58bc |
3.67 ± 0.58bc |
3.67 ± 0.58ab |
13.50 ± 1.00c |
40/60 |
4.55 ± 1.00a |
2.33 ± 0.57c |
3.00 ± 1.00c |
3.33 ± 0.58ab |
13.22 ± 0.58c |
100/0 |
4.67 ± 0.58a |
1.33 ± 0.58d |
1.33 ± 0.57d |
3.33 ± 0.57ab |
9.33 ± 0.58d |
3.3.2 Electronic nose. The results of the electronic nose tracking analysis of the blended systems were analyzed by PCA using the software of the electronic nose. The results are shown in Fig. 5. The PCA diagram of the blended systems showed that the contribution rate of the first principal component (PC1) reached 91.28%, and the contribution rate of the second principal component (PC2) reached 7.54%. The total contribution rate was 98.82%, which was greater than 95%, indicating very minor interference.32 When the ratios of anchovy mince to silver carp surimi were 0/100 and 100/0, the contribution rate of the first principal component in both cases showed the biggest difference. With the increase in anchovy mince content, the PC1 of the blended systems increased gradually. When the ratios of anchovy mince to silver carp surimi were 10/90, 20/80 and 30/70, the distribution of the first principal component axis was relatively close, indicating that the smell of the three blended systems was relatively similar. There was no overlap between the different data groups, indicating that the flavor components of the gel samples could be distinguished by PCA.
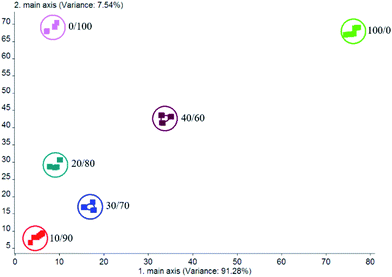 |
| Fig. 5 Principal component analysis of blended anchovy mince and silver carp surimi. A denotes anchovy mince, and S denotes silver carp surimi. A/S indicates the ratio of anchovy mince to silver carp surimi. | |
3.3.3 Electronic tongue. An electronic tongue, also known as taste sensor or taste fingerprint analyzer, is a modern instrument for qualitative and quantitative analysis, and includes data processing methods for pattern recognition.33 Fig. 6 is a radar image of the electronic tongue sample. Bitterness is caused by the bitter substance in surimi-based product. Based on the radar image of bitterness, when the ratios of anchovy mince to silver carp surimi were 10/90 and 20/80, the bitterness value of the blended systems were relatively low. It probably because when anchovy mince content was lower, the content of bitterness amino acid (including Val, Met, Iso, Leu, Phe, Lys, His and Arg) was lower, and therefore, the bitterness value of the blended systems was low. Based on the radar image of umami, with the increase of anchovy mince content, the umami value of the blended systems increased gradually. Umami is mainly induced by amino acids in surimi-based product. The increase in anchovy mince content gradually elevated the total content of Glu and Asp. The data of electronic tongue were consistent with the results of sensory evaluation.
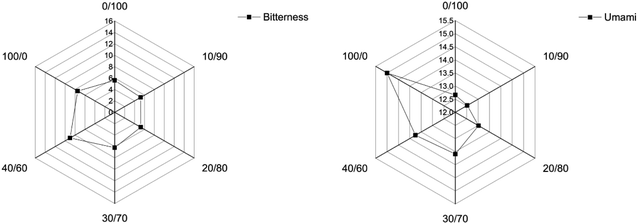 |
| Fig. 6 Bitterness and umami radar maps of blended anchovy mince and silver carp surimi. A denotes anchovy mince, and S denotes silver carp surimi. A/S indicates the ratio of anchovy mince to silver carp surimi. | |
3.3.4 GC-MS. The results of GC-MS are presented in Table 4. Esters account for a specific proportion of surimi products. Esters usually have sweet and typical fruit flavors, and result from esterification of acids and alcohols.34 With the increased content in anchovy mince, the relative esters content in the blended systems was higher, indicating that the aroma of the blended systems was enhanced gradually. 2-Ethylhexyl cyanoacetate does not exist in silver carp surimi, indicating that this substance exists only in anchovy mince. Alcohols are derived from lipid oxidation, amino acid reduction and carbohydrate metabolism, and contribute to an earthy flavor.35 Alcohols have high threshold. 1-Octene-3-ol is the oxidation product of linoleic acid, which has mushroom flavor and earthy smell, so it has an important contribution to the peculiar smell of fish. When the content in anchovy mince was lower, the alcohols content of the blended systems was lower, which indicated a lighter earthy smell of the blended systems.
Table 4 Gas chromatography-mass spectrometry results of blended anchovy mince and silver carp surimi
Classification |
Compound name |
RT/min |
RI |
Relative amount/% |
0/100 |
10/90 |
20/80 |
30/70 |
40/60 |
100/0 |
Esters |
Ethyl acetate |
3.0196 |
<800 |
1.5939 |
3.0295 |
3.3413 |
3.9189 |
3.7856 |
4.9045 |
2-Ethylhexyl cyanoacetate |
17.1074 |
822.6574 |
— |
0.0922 |
0.1097 |
0.3584 |
0.8527 |
0.9245 |
Butyl isobutyrate |
31.6624 |
811.6050 |
0.1495 |
— |
— |
— |
— |
— |
Alcohols |
1-Penten-3-ol |
3.5714 |
<800 |
— |
— |
— |
— |
— |
3.6919 |
3-Hexanol |
3.8137 |
<800 |
— |
— |
— |
— |
— |
10.1584 |
2-Methyl-2-butanol |
3.9175 |
<800 |
— |
— |
— |
— |
— |
2.3483 |
3-Methyl-1-butanol |
5.0654 |
<800 |
— |
— |
— |
— |
0.4984 |
2.2716 |
1-Butanol |
5.4403 |
<800 |
— |
— |
— |
— |
7.0249 |
6.8470 |
1-Hexanol |
9.7953 |
816.2212 |
0.8122 |
0.7805 |
0.709 |
0.6984 |
0.6851 |
0.4201 |
1-Octen-3-ol |
14.5471 |
858.2727 |
2.6345 |
1.3636 |
1.3733 |
1.9081 |
1.919 |
4.5621 |
2-Ethylhexanol |
16.7690 |
815.9163 |
2.2822 |
— |
— |
— |
— |
— |
Aldehyde |
Valeraldehyde |
2.4639 |
<800 |
— |
— |
— |
— |
— |
2.0288 |
Isovaleraldehyde |
3.0754 |
<800 |
— |
— |
— |
— |
— |
2.5165 |
Hexanal |
6.8016 |
822.4479 |
1.7622 |
10.1350 |
10.9103 |
11.4223 |
2.9795 |
0.9933 |
Heptaldehyde |
10.6720 |
842.7879 |
0.7868 |
0.4965 |
— |
— |
— |
— |
Ketone |
2-Butanone |
2.5216 |
<800 |
— |
— |
— |
— |
— |
0.8643 |
3-Pentanone |
4.0847 |
<800 |
2.8715 |
2.4062 |
— |
— |
— |
— |
2,3-Octanedione |
14.8308 |
866.5924 |
2.5063 |
— |
— |
— |
— |
— |
Alkanes |
2-Chloro-2-methylpropane |
4.1367 |
<800 |
— |
— |
— |
— |
— |
1.1227 |
Dodecane |
24.8906 |
894.9051 |
0.4555 |
— |
— |
— |
— |
— |
3,5-Dimethyloctane |
14.8078 |
865.9179 |
1.6133 |
— |
— |
— |
— |
— |
N-Pentadecane |
32.9699 |
839.0735 |
0.2790 |
— |
— |
— |
2.0098 |
1.3176 |
N-Heptadecane |
41.4011 |
871.0415 |
0.431 |
0.9515 |
1.2400 |
1.6808 |
1.3298 |
3.0015 |
Dipentene |
16.4498 |
809.5578 |
0.2314 |
0.5194 |
0.4289 |
0.3968 |
0.1529 |
0.1490 |
2-Phenyl-1-propene |
19.3762 |
867.8526 |
— |
— |
— |
— |
— |
0.5118 |
1-Hexadecene |
30.0993 |
863.6439 |
0.3259 |
0.2684 |
— |
— |
— |
— |
Toluene |
5.76905 |
<800 |
2.4429 |
0.3519 |
— |
— |
— |
— |
Ethylbenzene |
9.1780 |
897.4132 |
0.4851 |
— |
— |
— |
— |
— |
o-Xylene |
9.4780 |
806.6061 |
0.2290 |
— |
— |
— |
— |
— |
m-Xylene |
9.6279 |
811.1485 |
0.7970 |
— |
— |
— |
— |
— |
Other |
N-Methylhexylamine |
1.841 |
<800 |
— |
— |
— |
— |
— |
2.3583 |
Trimethylamine hydrochloride |
2.1947 |
<800 |
6.3245 |
5.8512 |
5.5011 |
5.0939 |
3.1917 |
2.2724 |
Isopropylamine |
2.4754 |
<800 |
17.9439 |
— |
— |
— |
— |
— |
2-Butene oxide |
3.1330 |
<800 |
— |
1.3125 |
— |
— |
— |
— |
2,3-Dihydrofuran |
6.0633 |
<800 |
0.4076 |
— |
— |
— |
— |
— |
Ethylene oxide |
2.0082 |
<800 |
2.1519 |
2.5865 |
4.5621 |
6.5307 |
7.5811 |
8.2541 |
Aldehydes are mostly derived from the oxidation of unsaturated fatty acids. The aldehydes content is usually lower than that of the other compounds, and account for a fatty flavor.32 Hexanal is the product of linoleic acid oxidation, with the smell of raw oil, green grass and apple. When the ratios of anchovy mince to silver carp surimi were 10/90, 20/80 and 30/70, the content of the blended systems aldehydes were higher, which was consistent with the previous results. Ketones have higher threshold than aldehydes, and are produced by oxidation or thermal degradation of polyunsaturated fatty acids, amino acid degradation or microbial oxidation.36 Ketones contribute minimally to fish odor, but they do enhance the fishy odor. When the content of anchovy mince was reduced, the ketone content of the blended systems was higher, resulting in a stronger fishy smell.
4. Conclusion
The ratio of anchovy mince to silver carp surimi affected the gel strength, whiteness, WHC, cooking loss rate, microstructure, and SDS-PAGE, as well as smell, taste, and volatile substances. The optimum sample formulation contained anchovy mince and silver carp surimi in a 10/90 ratio, which resulted in the highest gel strength, the highest WHC, and the most desirable sensory attributes. Because anchovy is marine fish, its may contain various endogenous enzymes, which will enhance the gel properties of the blended system, and the specific mechanism needs further experimental study.
Conflicts of interest
The authors have declared no conflicts of interest.
Acknowledgements
This study was supported by the grant from National Key R&D Program of China (2018YFD0901004), National Natural Science Foundation of China (31571868, 31972107, 31771999, 31701631, 31701629), and Natural Science Foundation of Liaoning Province (20180550889) and Liaoning Revitalization Talents Program (XLYC1907040, 1807133).
References
- L. Xu and F. Wu, China Fishery Ministry, China Agriculture Press, Beijing, China, 2019.
- L. Abbey, M. Glover-Amengor, M. O. Atikpo, A. Atter and J. Toppe, Food Sci. Nutr., 2017, 5, 374–379 CrossRef PubMed.
- I. Aydin and N. Gokoglu, Eur. J. Lipid Sci. Technol., 2014, 116, 996–1001 CrossRef.
- L. Garibaldi and S. Funge-Smith, FAO Fishery and Aquaculture Statistics, Fishery Year Book, Rome, Italy, 2018 Search PubMed.
- J. A. Park, S. Y. Joo, M. S. Cho and J. E. Oh, Fish. Sci., 2018, 84, 1091–1098 CrossRef.
- W. Tang, H. Zhang, L. Wang and H. Qian, Int. J. Food Sci. Technol., 2014, 49, 969–975 CrossRef.
- Y. Yu, S. Yi, Y. Xu, J. Shao, J. Li, Y. Li and G. Ji, Food Sci., 2016, 37, 17–22 Search PubMed.
- X. Fu, S. Xu and Z. Wang, Food Res. Int., 2009, 42, 90 CrossRef.
- I. Paker and K. E. Matak, LWT–Food Sci. Technol., 2015, 63, 985–991 CrossRef.
- M. A. Amiza and S. C. Ng, J. Aquat. Food Prod. Technol., 2015, 24, 213–226 CrossRef.
- AOAC, Official Methods of Analysis of the Association of Official Analytical Chemists, Washington, DC, 17th edn, 2002 Search PubMed.
- M. Chaijan, W. Panpipat and S. Benjakul, Food Chem., 2010, 121, 85–92 CrossRef.
- J. W. Park, J. Food Sci., 1994, 59, 525–527 CrossRef.
- I. Sanchez-Gonzalez, P. Carmona, P. Moreno, J. Borderías, I. Sanchez-Alonso, A. Rodríguez-Casado and M. Careche, Food Chem., 2008, 106, 56–64 CrossRef.
- A. Oujifard, S. Benjakul, M. Ahmad and J. Seyfabadi, LWT–Food Sci. Technol., 2012, 47, 261–266 CrossRef.
- J. Jaczynski and J. W. Park, J. Food Sci., 2004, 69, 53–57 CrossRef.
- H. Tian, F. Li, L. Qin, H. Yu and X. Ma, J. Food Sci., 2014, 79, 2346–2353 CrossRef PubMed.
- D. Liu, S. Li, N. Wang, Y. Deng, L. Sha, S. Gai, H. Liu and X. Xu, J. Food Sci., 2017, 82, 1076–1082 CrossRef PubMed.
- D. Ansorena, O. Gimeno, I. Astiasaran and J. Bello, Food Res. Int., 2001, 34, 67–75 CrossRef.
- J. Toppe, S. Albrektsen, B. Hope and A. Aksnes, Comp. Biochem. Physiol., Part B: Biochem. Mol. Biol., 2007, 146, 395–401 CrossRef PubMed.
- Z. Yu, H. Jiang, R. Guo, B. Yang, G. You, M. Zhao and X. Liu, Food Res. Int., 2018, 108, 144–150 CrossRef PubMed.
- V. Stoeger, K. I. Liszt, B. Lieder, M. Wendelin, M. Zopun, J. Hans and V. Somoza, J. Agric. Food Chem., 2018, 66, 6762–6771 CrossRef PubMed.
- P. Tanyamon and B. Soottawat, RSC Adv., 2017, 7, 52423–52434 RSC.
- M. Abdollahi, M. Rezaei, A. Jafarpour and I. Undeland, Food Chem., 2017, 229, 695–709 CrossRef PubMed.
- W. Panpipat, M. Chaijan and S. Benjakul, Food Chem., 2010, 122, 1122–1128 CrossRef.
- H. Ding, X. Li, R. Li, S. Yi, Y. Xu, H. Mi and J. Li, J. Texture Stud., 2019, 50, 332–340 CrossRef.
- A. Elyasi, E. Zakipour Rahim Abadi, M. A. Sahari and P. Zare, Int. Food Res. J., 2010, 17, 915–920 Search PubMed.
- L. Liu, Y. Luo, Y. Song, H. Shen and H. Hong, J. Aquat. Food Prod. Technol., 2013, 22, 36–46 CrossRef.
- S. Chanarat and S. Benjakul, J. Sci. Food Agric., 2013, 83, 929–937 Search PubMed.
- R. Gu, X. Xiao, J. Sun, L. Shi and H. Yang, Int. J. Food Prop., 2018, 21, 1743–1754 CrossRef.
- J. Pan, H. Jia, M. Shang, C. Xu, H. Lian, H. Li and X. Dong, J. Texture Stud., 2018, 49, 578–585 CrossRef.
- H. Zhang, Y. Zhu, S. Chen, C. Xu, Y. Yu, X. Wang and W. Shi, Food Sci. Nutr., 2018, 6, 2079–2091 CrossRef.
- H. Jiang, M. Zhang, B. Bhandari and B. Adhikari, Food Rev. Int., 2018, 3, 1–24 Search PubMed.
- T. Tian, H. Yang, F. Yang, B. Li, J. Sun, D. Wu and J. Lu, LWT–Food Sci. Technol., 2018, 89, 542–550 CrossRef.
- R. Jin, R. Meng, H. Zhang, X. Yang and Z. Wu, Int. J. Food Sci. Technol., 2018, 53, 2045–2053 CrossRef.
- D. Klein, S. Maurer, U. Herbert, J. Kreyenschmidt and P. Kaul, Food Anal. Methods, 2018, 11, 88–98 CrossRef.
|
This journal is © The Royal Society of Chemistry 2020 |