DOI:
10.1039/D0RA00701C
(Review Article)
RSC Adv., 2020,
10, 12626-12652
Recent advancements in synthetic methodologies of 3-substituted phthalides and their application in the total synthesis of biologically active natural products
Received
22nd January 2020
, Accepted 6th March 2020
First published on 27th March 2020
Abstract
We have provided a critical review that focuses on key developments in the area of 3-substituted phthalides and their role in the development of important biologically active natural products. 3-Substituted phthalides are vital molecules owing to their fascinating biological activity. The scope, isolation, and characterization of various naturally occurring racemic and chiral 3-substituted phthalides have been covered. We have put significant emphasis on recently developed research methodologies for the synthesis of racemic and chiral 3-substituted phthalides. These newer approaches are essential for the development of newer and elegant strategies for the synthesis of phthalide-based or similar molecular architecture with broader substrate scope and higher stereoselectivities. Also, we have discussed the application of 3-substituted phthalides as a precursor for the synthesis of natural products and their analogs.
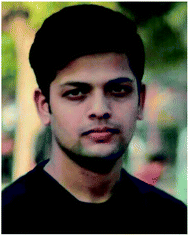 Amardeep Awasthi | Amardeep Awasthi is a Ph.D. research scholar enrolled in the Department of Chemistry, University of Delhi, India. He obtained his M.Sc. from Visvesvaraya National Institute of Technology, Nagpur. Currently, he is pursuing his Ph.D. under the supervision of Prof. Ramesh Chandra at the Department of Chemistry, University of Delhi, India. His research interest focuses on the chemical synthesis of biologically active natural products. His Ph.D. studies are funded by DST-Inspire. |
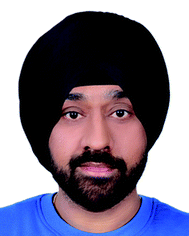 Mandeep Singh | Dr Mandeep Singh is a Dr D. S. Kothari Post-doctoral fellow from 2017 in the Department of Chemistry, University of Delhi, India. He obtained his Ph.D. degree on the topic “Selective Carbon–Carbon Coupling Reactions of Phthalides” from National Chemical Laboratory, Pune, with Dr N. P. Argade in 2013. Then, he moved to Technion-Israel Institute Technology, Haifa, Israel, with Prof. Ehud Keinan to work on host–guest chemistry from 2013–2015. He worked as a research scientist in TEVA API India Pvt. Ltd., Greater Noida, from 2015–2016. His areas of expertise are organic synthesis, supramolecular chemistry, asymmetric synthesis. |
 Garima Rathee | Garima Rathee obtained her M.Sc. from the University of Delhi in 2016 and BSc. from the University of Delhi in 2016. Currently, she is pursuing her Ph.D. under the supervision of Prof. Ramesh Chandra since 2016 at the Department of Chemistry, University of Delhi, India. Her research interests mainly focus on the synthesis of novel environment-friendly materials for water remediation and applications in organic methodology. Her Ph.D. studies are funded by the Department of Chemistry, University of Delhi. |
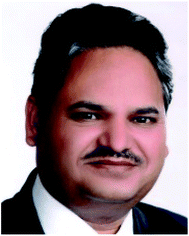 Ramesh Chandra | Prof. Ramesh Chandra (FRSC) is currently Head, Department of Chemistry, University of Delhi and Founder Director, Dr. B. R. Ambedkar Center for Biomedical Research (ACBR) he has been Vice-Chancellor, Bundelkhand University, Jhansi for six years (1999–2005), & President, Indian Chemical Society, Kolkata (2004–2006). He started his research career at University of Delhi, thereafter he went to the New York Hospital-Cornell University Medical Center and The Rockefeller University; Stony brook, New York as Assistant Research Professor. He conducted advanced research at the Harvard University, Massachusetts General Hospital & MIT, Cambridge. He has published 322 scientific Research Papers in International journals of repute and authored several books. His areas of expertise are Organic synthesis, Chemistry–Biology Interface, Catalysis, and Drug Discovery & Development. |
1. Introduction
One of the prime areas of research in organic chemistry has been concerned with the development of small-molecule natural products. A considerable number of modern-day drug developments are inspired by various sets of natural products, such as amino acids, flavonoids, alkaloids, terpenoids, quinonoid, and steroids.1 This area of research has paved the way for an important class of chemistry, known as medicinal chemistry.
An extensive series of biologically important natural products consist of phthalide frameworks.2 Phthalides are a prominent branch of natural products due to their biological importance. The fundamental core structure of phthalide consists of a benzene ring fused with a γ-lactone between carbons 1 and 3 (Fig. 1). All the known phthalide compounds have been recognized as derivatives of 1(3H)-isobenzofuranone. Mainly, phthalides have been found commonly in plant genera and also in fungi, bacteria, and liverworts.
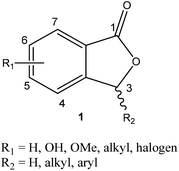 |
| Fig. 1 1(3H)-Isobenzofuranones 1 (phthalides) and their derivatives. | |
More than 180 naturally occurring phthalide derivatives have been identified. Among them, nearly 140 phthalides were isolated from a wide variety of plant species. These isolated phthalides have shown a broad spectrum of important clinical properties, such as anti-platelet accumulation, anti-smooth proliferation, anti-thrombosis, protection against cerebral ischemia, anti-angina, and cardiac function modulation and actions on the central nervous system.3
In ancient times, many phthalide-containing plants were used as herbal medicines. In China, phthalide-containing herbs have been recognized as some of the most commonly used natural medicines in traditional medicinal practice. Rhizoma Chuanxiong (Chinese name Chuanxiong) and Radix Angelicae sinensis (Chinese name Danggui) have been used for the treatment of cerebral- and cardiovascular diseases and female irregular menstruation for more than 2000 years.4
Phthalide containing drugs have gained worldwide popularity because of the wide range of pharmacophore activities of the moiety.5 For example, a significant problem during organ transplantation is a rejection of the transplanted organ. Mycophenolic acid (mycophenolate) 2 is a phthalide-containing immunosuppressant drug given to facilitate organ transplantation. It was the first antibiotic synthesized in a pure crystalline state. The US Food and Drug Administration has also approved this for use in kidney transplantation.6 Similarly, n-butylphthalide (NBP) 3 also became a successful anti-platelet drug for ischemia-cerebral apoplexy7 (Fig. 2). The Chinese government had approved this as an anti-ischemic stroke drug in 2002. Taking these facts into consideration, we can state that phthalide moiety has been used as a valuable framework in synthesizing many pharmaceutical drugs.5
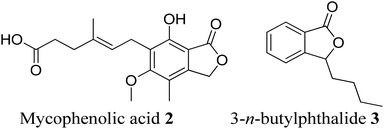 |
| Fig. 2 Structure of mycophenolic acid 2 and 3-n-butylphthalide 3. | |
Some phthalide-containing natural products are also reported in the literature. Phthalide plays a vital role as a building block8 in the synthesis of many natural products. For example, fuscinarin 4 is a potent human CCR5 antagonist, used to block the entry of HIV into host cells.9 However, the bioactivities of (−)-typhaphthalide 5,10 (+)-spiroxaline 6,11 and monascodilone 712 are still not known (Fig. 3).
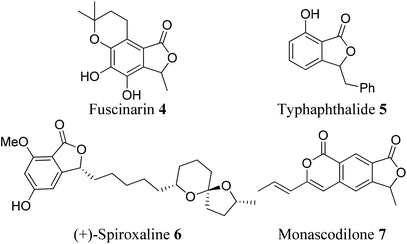 |
| Fig. 3 Naturally occurring 1(3H)-isobenzofuranones 4–7. | |
There are several available reactive sites of phthalides which have been explored, i.e., a nucleophilic attack on C1 carbonyl group, nucleophilic substitution reactions at C3 position carbanion, and reactions on the C4, C5, C6 and C7 positions of the phthalide.13 The 1(3H)-isobenzofuranone was initially synthesized in 1922 by Perkin and coworkers, via thermal decomposition of ethyl 2-(bromomethyl)benzoate.14 Later in 1955, Eliel and coworkers performed the reduction of methyl phthalate to phthalide in good yield using LiAlH4.15 In 1989, Watanabe and coworkers utilized a Diels–Alder reaction between substituted furanones and silyloxydienes to provide substituted phthalides in moderate to excellent yields.16 Recently, directed ortho-metallation, the reaction between homophthalic anhydride and benzil,17 the Heck–Matsuda reaction,18 and many more methods have been introduced to synthesize substituted phthalides.
1.1 Scope for 3-substituted phthalides
As we have already discussed, phthalide moiety is present in many natural products. Phthalides that are substituted at the C-3 positions possess an extensive range of biological and physiological activities.19 This moiety has been an essential intermediate to synthesize versatile natural products.20 This fact has led extensive efforts in the field of 3-substituted phthalides in the past two decades18 (Fig. 4). Different synthetic methodologies of selected natural products using 3-substituted phthalides as intermediates are described in the later part of the review.
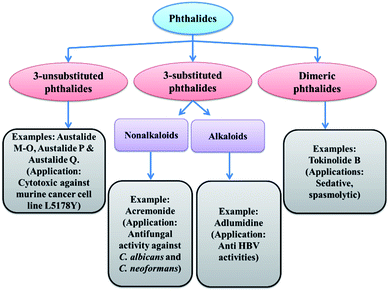 |
| Fig. 4 Classification of phthalides and their examples. | |
Statistically, more than 60% of the drugs currently available on the market are chiral molecules. As a result, asymmetric synthesis of chiral phthalides introducing C-3 chirality has also achieved considerable attention.21 Subsequently, an extensive number of asymmetric synthetic methodologies have been established for a variety of naturally occurring molecules with the potential treatment of different kinds of diseases.
The attention of this review is primarily on the synthesis and reactivity of the active methylene compounds, i.e., 1(3H)-isobenzofuranones. Previously, Mal et al. and Renoux et al. published two excellent reviews on the chemistry of phthalides.5,19 However, as per our knowledge, in previous literature reviews, the chemistry of racemic and chiral 3-substituted 1(3H)-isobenzofuranones and its application in total synthesis of important natural products was not discussed in detail. In this review, the synthetic methodologies for racemic and chiral 3-substituted 1(3H)-isobenzofuranones are discussed with particular emphasis on recent advances. Also, the application of racemic and chiral 3-substituted 1(3H)-isobenzofuranones as precursors for the synthesis of other critical molecular moieties are discussed.
The work presented here can benefit researchers in developing newer efficient strategies. This review paper contributes to ongoing efforts in seeking to develop and expand the utility of 3-substituted phthalides as precursors for much broader objectives.
1.2 Sources of a different kind of phthalides
Plants, fungi, bacteria, and liverworts have been different sources for phthalides. More than 180 naturally occurring phthalides appear in the literature. Most of these (∼137) are extracted from 202 diverse species of plants; as a result, phthalide-containing plants were long used as herbal medicines.22 Most naturally occurring phthalides are obtained from two plant species, Ligusticum and Angelica, in the Umbelliferae family. Some of the isoquinoline type phthalides such as noscapine 8 and bicuculine 48 are isolated from the poppy family. From the genus Ligusticum, more than 53 naturally occurring phthalides have been isolated from Ligusticum, and 38 biologically phthalides have been isolated from Angelica.20,23
1.3 Extraction, isolation, and characterizations of phthalides
The extraction of naturally occurring biologically active phthalides is one of the critical steps of analysis. It involves techniques such as pre-washing, grinding, and drying of plant materials to obtain a homogenous sample. It should be taken care that potential plant constituents are not degraded during extraction. The selection of solvent also plays a crucial role in the extraction of phthalides, and it largely depends upon the nature of the phthalides. Most phthalides are non-polar, so for the extraction of such molecules, hexane or petroleum ether can be used as an initial extraction solvent. To extract polar phthalides, we use polar solvents, such as ethanol, chloroform, methanol, and ethyl-acetate.21,24 The extraction process for phthalides has remained mostly unchanged over the years; however, some upgraded extraction procedures have been reported.25,26
Phthalides are usually isolated via column chromatography, thin layer chromatography (TLC), and HPLC, with column chromatography being the most common. Silica, alumina, and LH-20 are frequently used adsorbents for column chromatography.27,28 Other techniques that have been used for some specific phthalides include preparative TLC (PTLC), centrifugal circular TLC (CCTLC), medium-pressure liquid chromatography (MPLC), high-speed countercurrent chromatography (HSCCC), droplet-countercurrent chromatography (DCCC), and high-vacuum low-temperature distillation.29
Initially, the characterization of naturally occurring phthalides was carried out through melting points, boiling points, saponification, UV spectroscopy, and hydrolysis techniques. After the development of NMR, IR, GC-MS, and X-ray crystallography, characterizing phthalides has become much easier.
2. Isolated natural products encompassing 3-substituted phthalide framework
Table 1 summarises examples of isolated natural products encompassing 3-substituted phthalide framework
Table 1 Important biologically active natural products encompassing 3-substituted phthalide framework
S. no. |
Natural products |
Isolation sources |
Biological activities |
1 (ref. 30) |
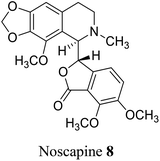 |
Cultivated opium poppy plants |
Anti-tussive, anti-cancer, and potential antineoplastic activities |
2 (ref. 31) |
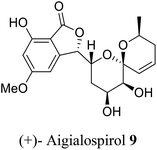 |
Aigialus parvus BCC 5311 |
Not known |
3 (ref. 32) |
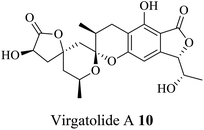 |
Pestalotiopsis virgatula |
Cytotoxic against HeLa (cervical epithelium) cells |
4 (ref. 17) |
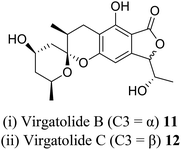 |
Pestalotiopsis virgatula |
Cytotoxic against HeLa (cervical epithelium) cells |
5 (ref. 33) |
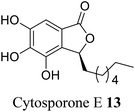 |
Cytosora sp. CR200 |
Anti-microbial |
6 (ref. 34) |
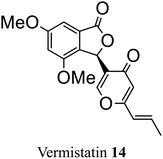 |
Penicillium vermiculatum DANG |
Cytotoxic against tumor cells |
7 (ref. 35) |
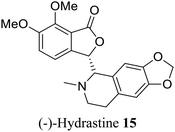 |
Corydails stricta |
Anti-paclitaxel – resistant anti-cancer (ovarian) activity |
8 (ref. 36) |
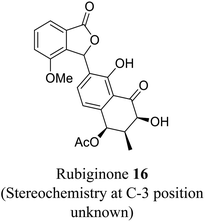 |
Streptomyces sp. |
Anti-bacterial activity, prevents the growth of specific Gram-positive bacteria and cytotoxic against diverse tumor cells |
9 (ref. 37) |
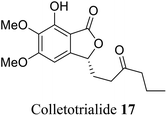 |
Collectotrichum sp. |
Anti-oxidant and chemo preventive properties |
10 (ref. 38) |
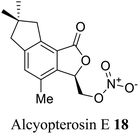 |
Alcyonium paessleri |
Cytotoxicity toward human larynx carcinoma |
11 (ref. 39) |
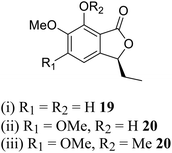 |
Pittosporum illicioides |
In vitro inhibitory activity on neutrophil pro-inflammatory response |
12 (ref. 40) |
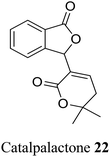 |
Catalpa ouata G. |
Anti-tumor promoting activity |
13 (ref. 41) |
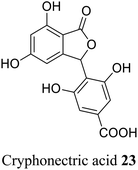 |
Cryphonectria parasitica |
Anti-fungal activity, (inhibits the formation of tomato seedings) |
14 (ref. 42) |
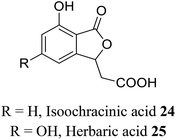 |
Alternaria kikuchiana (isoochracinic acid), Cladosporium herbarum (herbaric acid) |
Anti-bacterial and anti-biotic |
15 (ref. 43) |
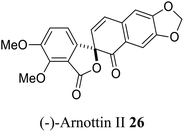 |
Xanthoxylum arnottianum |
Not known |
16 (ref. 44) |
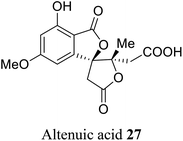 |
Alternaria tenuis |
Not known |
17 (ref. 45) |
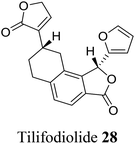 |
Salvia tiliaefolia and Salvia puberula |
Plant growth stimulator |
18 (ref. 46) |
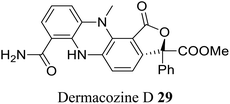 |
Dermacoccus abyssi |
Cytotoxic against different tumor cells lines |
19 (ref. 47) |
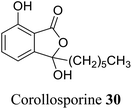 |
Corollospora maritima |
Anti-bacterial activity against Staphylococcus |
20 (ref. 48) |
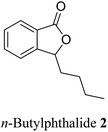 |
Apium graveolens, Angelica sinensis |
Anti-convulsant, anti-stroke and anti-proliferative |
21 (ref. 49) |
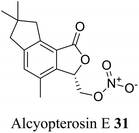 |
Subantarctic soft coral Alcyonium paessleri |
Toxic towards Hep-2 (human larynx carcinoma) cell line |
22 (ref. 50) |
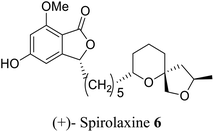 |
Fungus Sporotrichum laxum |
Anti-tumor and active against Helicobacter pylori, also, lower the cholesterol level in the body |
23 (ref. 51) |
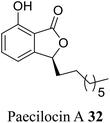 |
Fungus Paecilomyces variotii |
Anti-bacterial activity against pathogenic bacteria including Staphylococcus aureus 3089 and Vibrio parahaemolyticus 7001 |
24 (ref. 52) |
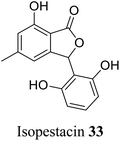 |
Pestalotiopsis microspora |
Anti-fungal activity |
25 (ref. 53) |
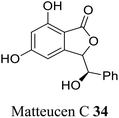 |
Chineses medicinal herb Matteuccia orientalis for the treatment of hemostatics and reliving ostalgia |
Not reported |
26 (ref. 54) |
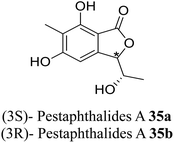 |
Solid culture of an isolate of Pestalotiopsis foedans |
Anti-fungal |
27 (ref. 55) |
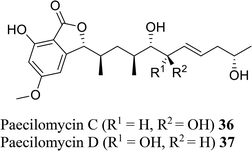 |
Solid culture of Paecilomyces sp. SC0924 |
Anti-fungal |
28 (ref. 56) |
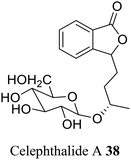 |
Celery seed |
A diuretic for bladder and kidney complaints and adjuvant in arthritic conditions |
29 (ref. 57) |
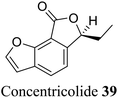 |
Ascomycete Daldinia concentrica |
Anti-HIV-1 |
30 (ref. 58) |
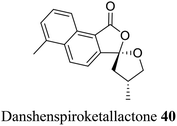 |
Traditional Chinese medicine consisting of Salvia miltiorrhiza |
Immunosuppressants and anti-stroke |
31 (ref. 59) |
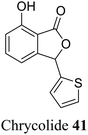 |
Leaves and stem of a popular vegetable Chrysanthemum coronarium |
Anti-feeding activity |
32 (ref. 60) |
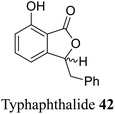 |
Rhizomes of Typha capensis |
Anti-bacterial activity against diarrhea and dysentery |
33 (ref. 61) |
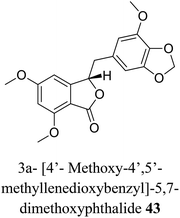 |
Frullania sp. |
Cytotoxic against human promyelocytic leukemia |
34 (ref. 62) |
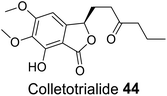 |
Euryops hebecarpus |
Not known |
35 (ref. 63) |
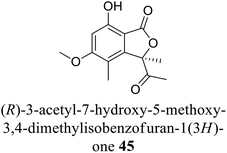 |
Endophytic fungus |
Anti-oxidant activity |
36 (ref. 64) |
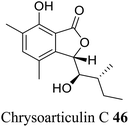 |
Leptosphaeria sp. |
Anti-fungal activity |
37 (ref. 65) |
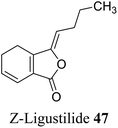 |
Ligusticum porteri |
Anti-proliferative activity (sedative and relaxant) |
38 (ref. 66) |
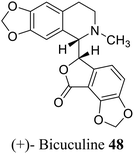 |
Fumaria capreokzta L and Fumaria bella |
Potent GABa receptor antagonist and used to block Ca2+ activated potassium channels |
3. Synthetic routes for 3-substituted 1(3H)-isobenzofuranones
We have classified synthetic routes to access 3-substituted (±)-1(3H)-isobenzofuranones in two major titles. First, we have emphasized recent approaches to generate racemic 3-substituted (±)-1(3H)-isobenzofuranones, and later we have described recent approaches to generate optically pure 3-substituted 1(3H)-isobenzofuranones.
3.1 Recent methodologies for the synthesis of 3-substituted (±)-1(3H)-isobenzofuranones
3.1.1 Metal catalyzed synthesis of 3-substituted (±)-1(3H)-isobenzofuranones. Fan and co-workers67 have reported 3-substituted phthalides 51 via a ruthenium-catalyzed intermolecular cascade reaction of aromatic acids 49 with aromatic aldehydes 50. The synthesis involves the direct insertion of the C–H bond of the aromatic acids into a polar C
O bond of aromatic aldehydes, which is followed by the consecutive intramolecular nucleophilic substitution. The polarity (electrophilicity) of the C
O bond in aromatic aldehydes was increased by having electron-withdrawing groups (NO2, CF3, F, Cl, Br) on the aromatic ring (Scheme 1).
 |
| Scheme 1 Ruthenium catalyzed the synthesis of 3-substituted phthalides. | |
Nguyen and co-workers68 demonstrated a direct route to obtain phthalides 53 via carboxylation of benzoxasiloles 52 with carbon dioxide, using CuI as a catalyst. Several advantages of this methodology are the use of copper salt as a catalyst, economical starting materials and convenient reaction setup (Scheme 2). The main challenge of this methodology was the unexplored reactivity of organosilanes with CO2.
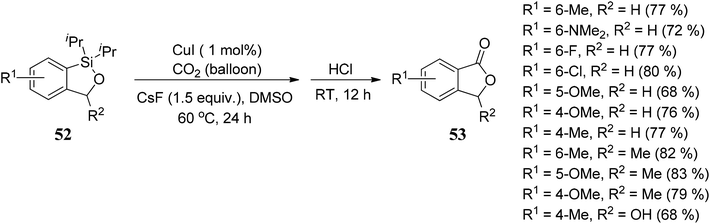 |
| Scheme 2 Synthesis of benzoxasiloles via copper-catalyzed direct carboxylation. | |
Arcadi and co-workers69 have described a palladium-catalyzed hydroarylation and hydrovinylation reaction of γ-propargylic alcohols 54 with aryl iodides 55 to afford crude γ,γ-disubstituted allylic alcohols 56. Allylic alcohols 56 were treated with NaOH followed by acidification afforded 3,3-disubstituted phthalides 59 in good to moderate yields (Scheme 3).
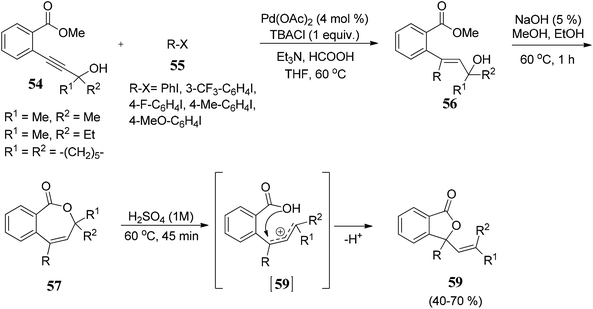 |
| Scheme 3 Synthesis of 3-vinyl phthalides. | |
Matsuda and co-workers70 described an oxidative cyclization of phthalaldehydes 60 and alcohols catalyzed by rhodium(III) catalyst and copper acetate to afford 3-alkoxyphthalides 61 in good to moderate yields (Scheme 4). The reaction is believed to be proceeding via Rh–Cu relay catalytic system.
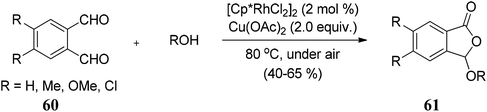 |
| Scheme 4 Synthesis of 3-alkoxy phthalides. | |
The work was further extended to explore the utility of 1,3-dicarbonyl compounds 63 as nucleophiles for the reaction with phthalaldehydes 62 under similar conditions. This led to the synthesis of 3-alkylphthalides 64 in excellent yields (Scheme 5).
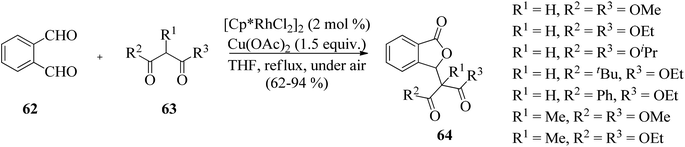 |
| Scheme 5 Synthesis of 3-alkyl phthalides. | |
Gandeepan and co-workers71 demonstrated rhodium(III)-catalyzed regio- and stereoselective synthesis of disubstituted E-phthalides 67 from aryl acids 65 and allenes 66. The reaction proceeded via ortho C–H bond activation followed by an annulation pathway. The scope of the methodology was further investigated on a variety of aryl acids 65 and allenes 66 (Scheme 6).
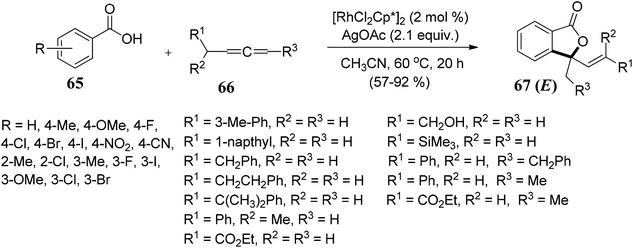 |
| Scheme 6 Scope of arenecarboxylic acids and allenes (synthesis of disubstituted phthalides). | |
3.1.2 Hydroiodination-triggered synthesis of 3-substituted (±)-1(3H)-isobenzofuranones. Hydroiodination-triggered cascade reaction is demonstrated by Kawaguchi and co-workers72 by using I2, PPh3, and H2O in CDCl3 to furnish 3-substituted phthalides 69 in excellent yields. The reaction proceeds via a four-step sequence, i.e., desilylation, hydroiodination, cyclization, and reduction, in one pot (Scheme 7). The present method eliminates the need for a metal catalyst to form phthalides.
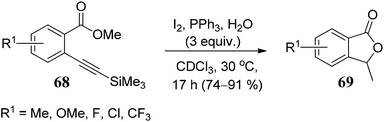 |
| Scheme 7 Hydroiodination-triggered synthesis of 3-methylphthalides. | |
The substrate scope of 2-ethynylbenzoates 70 was also studied by using cyano, chloro, phenyl, and ester groups on the side chain. They were tolerated during the four-step sequence to provide 3-substituted phthalides 71 (Scheme 8).
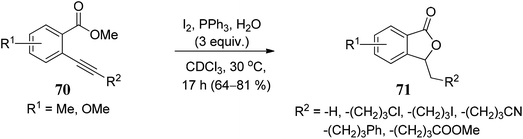 |
| Scheme 8 Synthesis of 3-substituted phthalides triggered via hydroiodination. | |
3.1.3 Synthesis of 3-substituted (±)-1(3H)-isobenzofuranones using β-keto acids as a nucleophile center. Jia and co-workers73 developed a one-pot cascade aldol/cyclization reaction of 72 wherein β-keto acids 73 were directly employed as a nucleophilic center, and glycerol was used as a solvent. Here, β-keto acids functioned as ketone enolate equivalents. An extensive substrate scope for β-keto acids was explored, affording a wide variety of 3-substituted phthalides in good to excellent yields (Scheme 9).
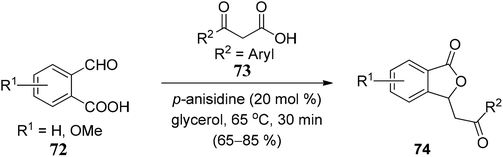 |
| Scheme 9 Synthesis of 3-substituted phthalides using β-keto acids as a nucleophile center. | |
3.1.4 Synthesis of 3-substituted (±)-1(3H)-isobenzofuranones using Schiff base. Perillo and co-workers74 developed cascade reaction of glycine Schiff base 77 with 2-carbomethoxy benzaldehyde 76, which involved aldol condensation followed by cyclization under the acidic conditions to provide α-amino ester 3-substituted phthalides 80 in good yield (Scheme 10). The methodology was further extended to develop an enantioselective version of the reaction to obtain chiral 3-substituted phthalides in high ee's.73
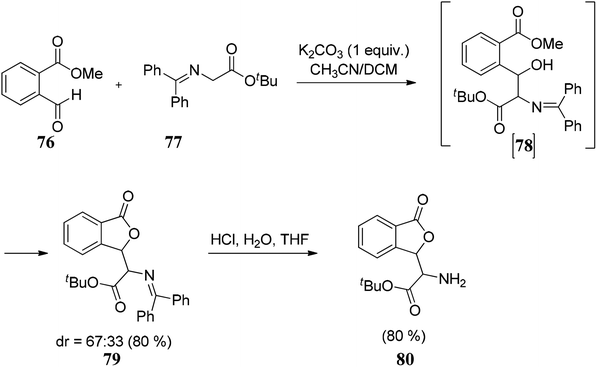 |
| Scheme 10 Synthesis of α-amino ester 3-substituted phthalide. | |
A variety of bifunctional phase-transfer catalysts (PTC) were examined to obtain 3-substituted phthalides in excellent enantioselectivity. Bifunctional PTC 75 (Fig. 5) gave the desired product in moderate ee's (51–71%).73
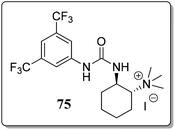 |
| Fig. 5 Bifunctional phase-transfer catalyst. | |
3.1.5 Oxa-Michael addition reaction to generate 3-substituted (±)-1(3H)-isobenzofuranones. Youn and co-workers75 have developed NHC-catalyzed domino oxidation of 2-alkenylbenzaldehydes 81, followed by oxa-Michael addition reaction to afford 3-substituted phthalides 83. The protocol developed has a broad substrate scope and wide functional group tolerance. The success of the domino process could be achieved in two ways; by exploiting atmospheric oxygen as an oxygen atom source and by adding an electron-deficient olefin bearing hetero atom with lone pair of electrons. Also, molecular oxygen in air could play an essential role in transformation, as similar NHC-catalyzed reactions of the same substrates under inert atmosphere produce follow different reaction pathways (Scheme 11).
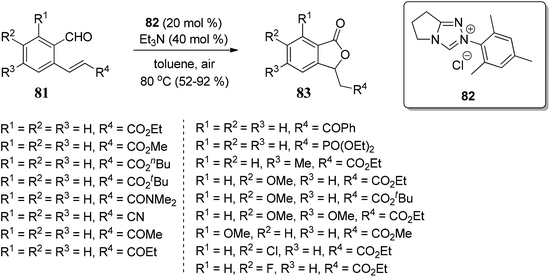 |
| Scheme 11 NHC-catalyzed domino oxidation/oxa-Michael addition of 2-alkenylbenzaldehydes. | |
3.1.6 Friedel–Crafts alkylation reaction to generate 3-substituted (±)-1(3H)-isobenzofuranones. Tang and co-workers76 have developed an efficient methodology to synthesize 3-indolyl-substituted phthalides 86 via Friedel–Crafts alkylation of indoles 85 with 3-hydroxy phthalide 84 using TsOH as the catalyst. The usefulness of the process was studied with variously substituted indoles which reacted efficiently at room temperature to afford phthalides in excellent yields (Scheme 12).
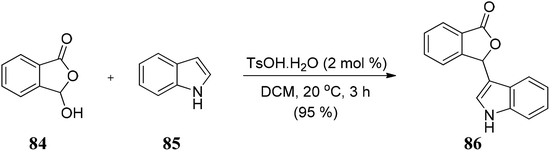 |
| Scheme 12 Synthesis of 3-indolyl-substituted phthalides. | |
3.1.7 NBS mediated free-radical bromination to generate 3-substituted (±)-1(3H) isobenzofuranones. Li and co-workers77 have devised a four-step strategy for the synthesis of 3-substituted phthalide. The condensation reaction of 3-ethoxyphthalide 89 with diethylmalonate carbanion followed by decarboxylation and hydrolysis gave 3-substituted phthalides 92 in 44% overall yield over four steps (Scheme 13). NBS mediated free-radical bromination of phthalide 87 gives 88. The crude 88 was treated with hot ethanol, then cooled to give 89 as white solid.
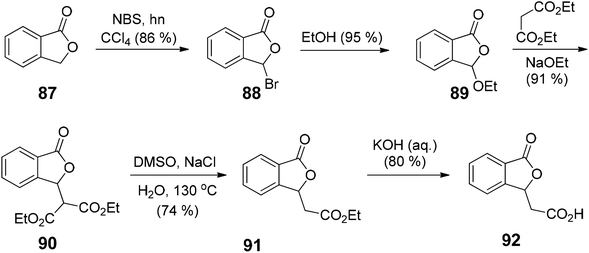 |
| Scheme 13 Synthesis of 3-substituted phthalides. | |
3.1.8 Photochemical catalyzed synthesis of 3-substituted (±)-1(3H)-isobenzofuranones. Tatsugi and co-workers78 demonstrated that the degassed alcoholic solution of indane-1,2,3-trione 93 could be photochemically irradiated to afford 3-alkoxycarbonylphthalides 94 as the major product. During the process, 3-alkoxyphthalide 95 was also obtained in minor quantities (Scheme 14).
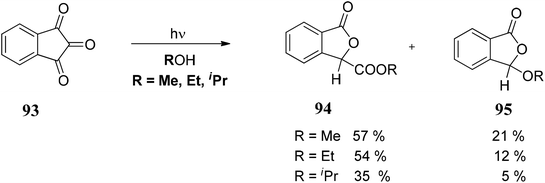 |
| Scheme 14 Photochemical reactions of indane-1,2,3-trione. | |
The initial step of the photochemical process could be the cleavage of a C–O bond to form semidione radical 93a, which under rearrangement forms 93b. Thus, 93b can follow two pathways: (i) it can form the compound 93c, which on reaction with ROH forms 93d followed by protonation to give 3-alkoxycarbonylphthalides 94 or (ii) 93f rearranges to 93g followed by decarbonylation gave phthalides carbene which on quenching with ROH gave 3-alkoxyphthalide 95 (Scheme 15).
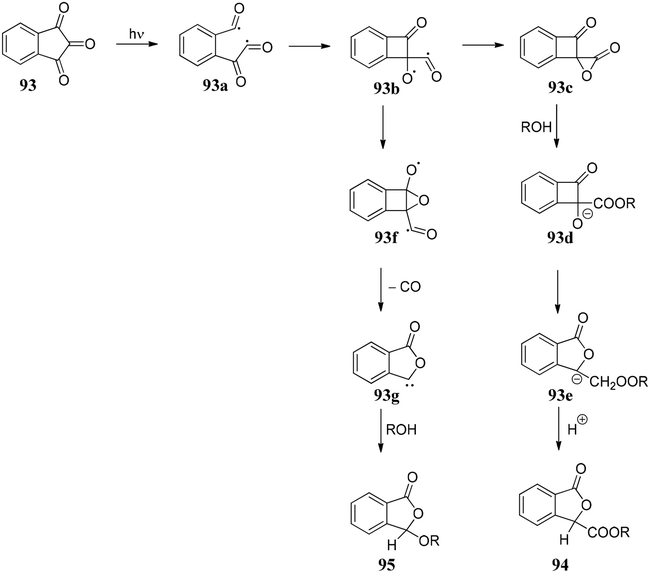 |
| Scheme 15 Proposed mechanistic pathway. | |
3.2 Recent methodologies to synthesize enantiomerically pure 3-substituted 1(3H)-isobenzofuranes
In this section, we have described recent approaches to synthesize enantiomerically pure 3-substituted 1(3H)-isobenzofuranes.
3.2.1 Synthesis of 3-substituted 1(3H)-isobenzofuranones using diverse organozinc reagents. Huang and co-workers79 demonstrated a new protocol for the synthesis of chiral 3-substituted phthalides 99 by carrying out catalytic asymmetric 1,2-addition of methyl 2-formylbenzoates 98, followed by lactonization, using diverse organozinc reagents (Scheme 16). They developed a chiral phosphoramide ligand–Zn(II) complex, which was synthesized from (1R,2R)-diphenylethyelendiamine 100 as catalyst. The efficiency of the process is highlighted by the fact that the enantiopure phthalide 99 was obtained in excellent yields (∼95%) and good enantioselectivities (∼89%).
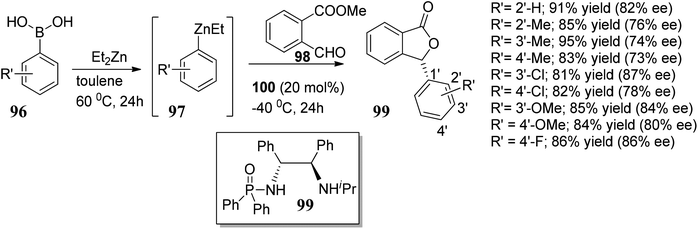 |
| Scheme 16 Asymmetric 1,2-addition/lactonization tandem reaction of methyl 2-formylbenzoate. | |
Carlos and co-workers80 carried out the asymmetric catalytic synthesis of 3-aryl phthalides 102 via sequential asymmetric arylation–lactonization pathway. In the presence of a chiral amino naphthol ligand, the reactive arylating agents, generated by boron–zinc exchange, were reacted with 2-formylbenzoates 101, which was followed by lactonization to yield the corresponding chiral phthalides 102 in excellent yields and enantioselectivities (∼87–90% ee) (Scheme 17). The place of the substituent at the aryl ring was found to have a slight effect on the efficiency of the arylation reactions. The asymmetric addition of an aryl zinc reagent to the 2-formylbenzoate is the enantio determining step.
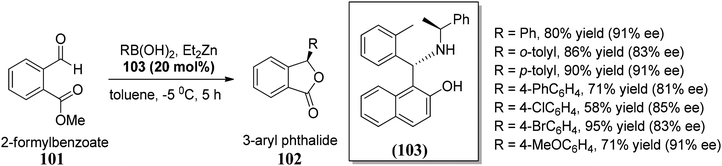 |
| Scheme 17 Asymmetric arylation–lactonization sequence. | |
3.2.2 Synthesis of chiral 3-substituted phthalides via palladium-catalyzed Heck–Matsuda arylation of arenediazonium salt. Kattela and co-workers81 have described an enantioselective synthesis of chiral 3-substituted phthalides via palladium-catalyzed Heck–Matsuda arylation of arenediazonium salt 104 with 2,3-dihydrofurans 105, followed by NaBH4 mediated reduction and lactonization pathway to give chiral phthalides 107 in overall yields and excellent enantioselectivities (up to 98% ee). The strategy was further extended for the synthesis of medicinally important chiral lactones, amines, and olefins (Scheme 18).
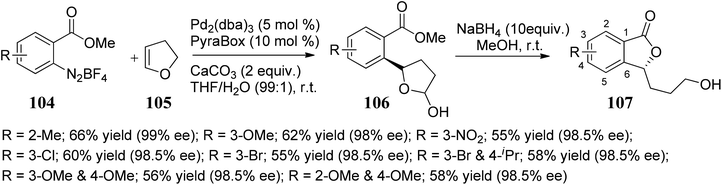 |
| Scheme 18 Heck–Matsuda arylation of dihydrofurans. | |
3.2.3 Novel derived metal complex ligand as a catalyst for the synthesis of chiral 3-substituted phthalides. Ge and co-workers82 have developed the first asymmetric hydrogenation of 3-alkyl/arylidenephthalides 108 to furnish an extensive range of 3-substituted chiral phthalides 109 in admirable enantiomeric excesses (∼98% ee). The hydrogenation process was catalyzed by a novel derived IrI complex of a spiro-[4,4]-1,6-nonadiene-based phosphine–oxazoline ligand (SpinPHOX) 110 as a catalyst. The effectiveness of the protocol further extended for the asymmetric synthesis of enantioselective drugs as well as the bioactive natural products (Scheme 19).
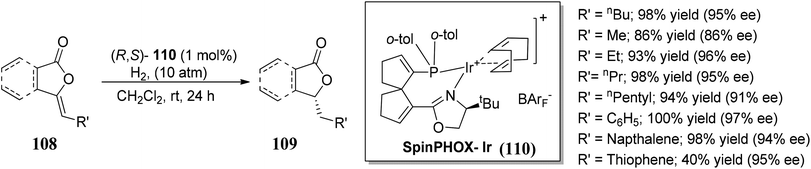 |
| Scheme 19 Asymmetric hydrogenation of the 3-alkyl/arylidenephthalides. | |
Zhang and co-workers83 demonstrated a novel route for the reductive cyclization of 2-acylarylcarboxylate 111 via asymmetric transfer hydrogenation. The reaction was promoted by a new Ru(II)–diamine complex 113, which catalyzes asymmetric transfer hydrogenation and in situ lactonization to provide enantiomerically pure 3-substituted phthalides 112 (Scheme 20).
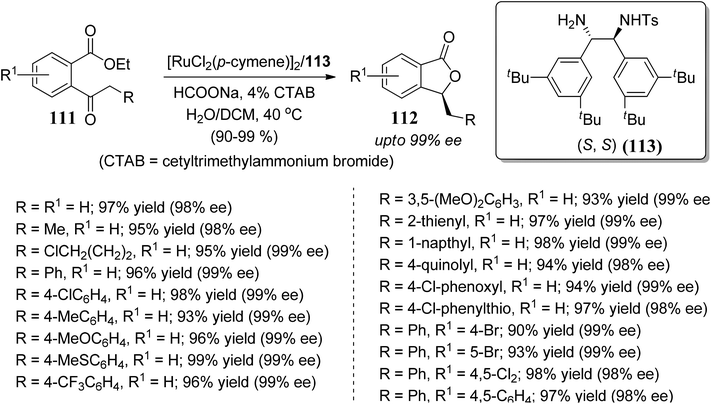 |
| Scheme 20 Asymmetric synthesis of 3-substituted phthalides by ruthenium-catalyzed transfer hydrogenation. | |
The observed excellent enantioselectivity can be explained by a preferable transition state of the Ru–TsDBuPEN complex and ethyl 2-acylarylcarboxylate substrates, which determines the chirality. Hydrogen bonding with the neighboring ester function group of the 2-acylarylcarboxylate substrate might also be accountable for the observed selectivity (Fig. 6).
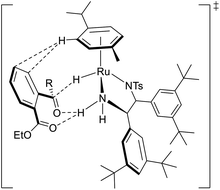 |
| Fig. 6 Proposed transition state. | |
Kumbhar and co-workers84 synthesized bipyridyl ligands. These chiral ligands were applied in the synthesis of chiral phthalides. The reaction sequence involved chromium-catalyzed enantioselective Nozaki–Hiyama–Kishi allylation of substituted benzaldehydes 114, followed by lactonization gave enantiopure phthalides 116 with an optimal ee of 99%. Chiral Cr(II) complex developed using bipyridine alcohol and CrCl3. This utility of the protocol was further extended by accomplishing the synthesis of (S)-cytosporone E in three steps (Scheme 21).
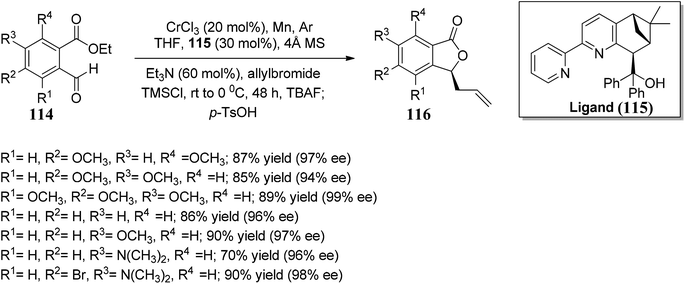 |
| Scheme 21 Enantioselective Nozaki–Hiyama–Kishi allylation. | |
Lu and co-workers85 established an extremely effective and enantioselective approach towards the synthesis of bioactive 3-substituted chiral phthalides 119. The protocol involved ruthenium-catalyzed hydrogenation followed by lactonization of 2-acylarylcarboxylates 117 to furnish 3-substituted chiral phthalides. Different chiral phosphine ligands were employed to obtain good enantioselectivity, the best among them was (S)-SunPhos 118, which helped in the induction of enantioselectivity >99% ee (Scheme 22).
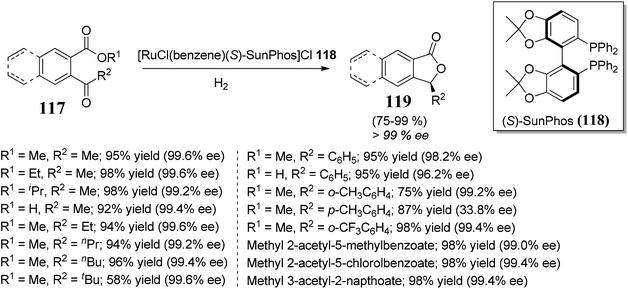 |
| Scheme 22 Asymmetric hydrogenation of 2-acylarylcarboxylate. | |
3.2.4 Chiral bifunctional cinchonine as an organocatalyst for the synthesis of chiral 3-substituted phthalides. Youn and co-workers86 developed an asymmetric domino oxidation/oxa-Michael addition reaction wherein an N-heterocyclic carbene (NHC) 121 and a chiral bifunctional cinchonine organocatalyst 122 work cooperativity to furnish the chiral 3-substituted phthalides 123. The use of a bifunctional cinchonine catalyst helps in achieving excellent enantioselectivity, where it functions both as a base (quinuclidine) and hydrogen bond donor, thus activating nucleophile and electrophile, respectively. Cinchonine works both as a Brønsted base for the generation of NHC as well as a bifunctional catalyst for asymmetric induction (Scheme 23).
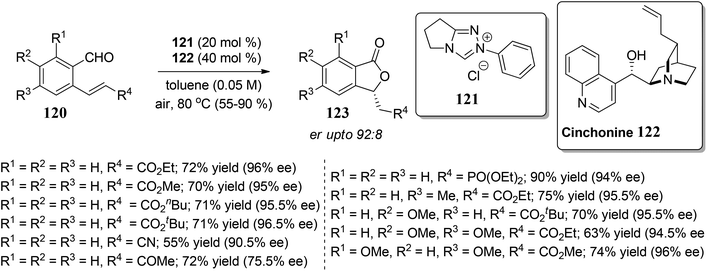 |
| Scheme 23 Asymmetric oxidative cyclization of 2-alkenylbenzaldehydes. | |
3.2.5 Synthesis of chiral 3-substituted phthalides via a nucleophilic addition reaction. Zhang and co-workers87 described a two-step asymmetric route for 3-substituted phthalides 130. The chiral amide 126 was subjected to the treatment with isopropyl magnesium chloride followed by reaction with various aldehydes 128 (Scheme 24). Intramolecular cyclization of the substrate allowed the synthesis of 3-substituted phthalides 130 (∼88% ee).
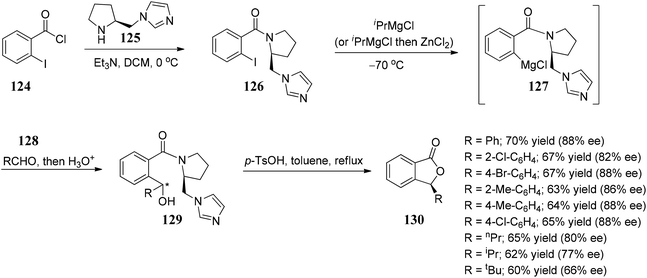 |
| Scheme 24 Direct asymmetric synthesis of 3-substituted phthalides. | |
Davis and co-workers88 developed an enantioselective approach towards the synthesis of 3-substituted phthalides by the addition of phthalide anions 133 to chiral-sulfinimines (N-sulfinyl imines) 132. The present approach was extended for the synthesis of chiral 3-substituted isoquinolones and 3-substituted 4-hydroxy isoquinolines, respectively (Scheme 25).
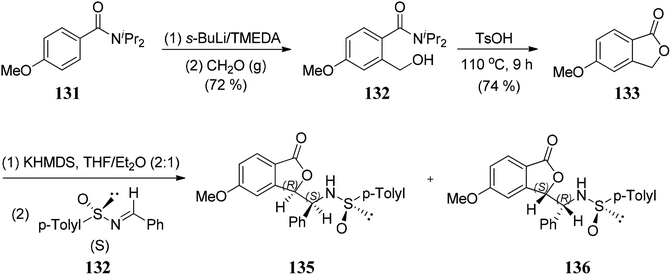 |
| Scheme 25 Reaction of phthalide anion with enantiopure sulfinimines. | |
3.2.6 Tandem aldol-lactonization reactions for the synthesis of chiral 3-substituted phthalides. Ray and co-workers89 carried out chiral Brønsted acid 140 catalyzed, tandem mannich–lactamization, and aldol-lactonization reactions to achieve the enantioselective synthesis of phthalides 143 in good to excellent enantioselectivities (Scheme 26). The developed protocol has broad substrate scope, and a variety of substituted aromatic aldehydes and aromatic amine were used.
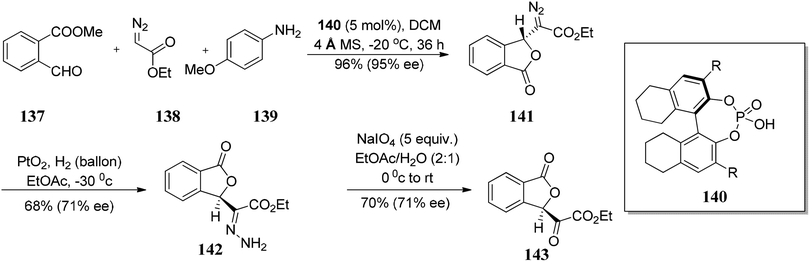 |
| Scheme 26 Tandem aldol-lactonization reactions. | |
4. Application of 3-substituted 1(3H)-isobenzofuranones for the construction of crucial molecular architecture
As discussed in Table 1, we can state that phthalide moiety is present in various biologically active natural products. In this section, we aim to provide some of the examples of 3-substituted 1(3H)-isobenzofuranones for the construction of important molecular architecture.
4.1 Annulation of stabilized phthalide anions with Michael acceptors
Annulation with stabilized phthalide anions along with Michael acceptors is a powerful and convenient tool for obtaining the quinoid natural products. Many natural products consist of a standard quinone unit. The exciting structures and vital biological activities90 of these natural products have provided an influential forum for organic chemists to explore this area of research. The general protocol for this reaction was discovered in the late 1970s simultaneously by Hauser91 and Kraus.92
The phthalide annulation involves the deprotonation of a stabilized phthalides 144 by a strong base and in situ capture of anion by a suitable Michael acceptor 145 followed by Dieckmann-like condensation to afford a bicyclic compound 148 (Scheme 27). Compound 148 undergoes mild oxidation to form biphenol derivatives 149. This methodology presents an elegant way to synthetic naphthol derivatives via phthalide chemistry.
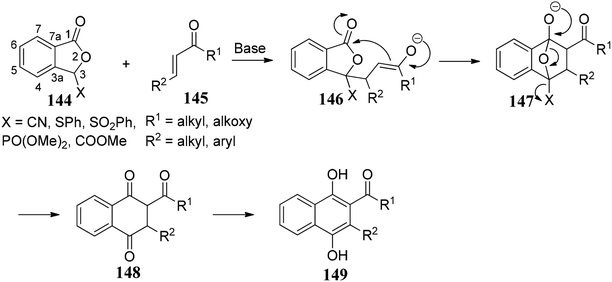 |
| Scheme 27 Annulation of stabilized phthalide anions with Michael acceptors. | |
Chaturvedi and co-workers93 developed a novel route to utilize unsaturated phosphonates for annulation reaction as Hauser acceptors. Good yields of phosphorylated 1,4-dihydroxynaphthalenes 153 are obtained, which on further oxidation results in their corresponding 1,4-naphthoquinones 154. The reaction is successful in providing an efficient, straightforward, and powerful approach for synthesizing disubstituted naphthalene-1,4-diols 153. Naphtha-1,4-diones 154 consist of a various (hetero) aryl groups positioned at 3 and a phosphonate group positioned at 2 (Scheme 28).
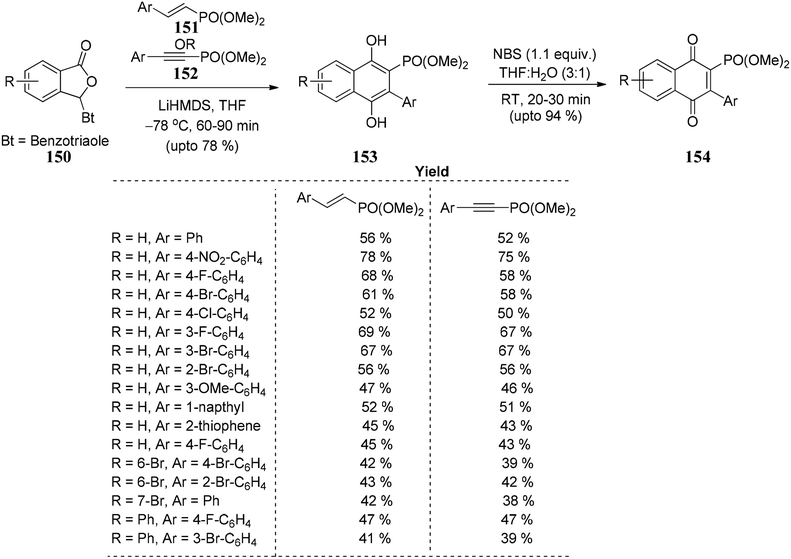 |
| Scheme 28 Hauser–Kraus annulation of 3-substituted phthalides. | |
4.1.1 Metal-free catalytic annulation to develop enantioriched highly functionalized dihydronaphthoquinones. A metal-free catalytic annulation is developed by Zhuang and co-workers,94 which involves Lewis base-mediated asymmetric allylic alkylation and a novel asymmetric intramolecular acyl cyanation of alkenes. This route provides a novel method to obtain enantioriched highly functionalized dihydronaphthoquinones 160 and chiral 3,3-disubstituted phthalides 158 having quaternary carbon centers (Schemes 29 and 30). The reaction involves the use of chiral bifunctional thiourea organocatalyst.
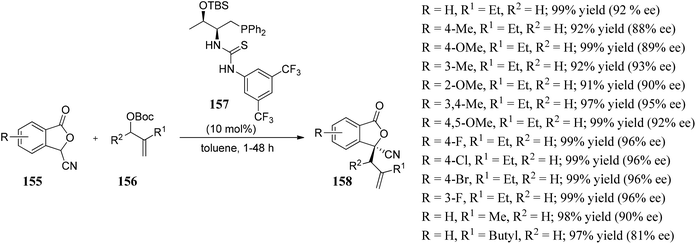 |
| Scheme 29 Lewis base-catalyzed asymmetric allylic alkylation of 3-cyano phthalides. | |
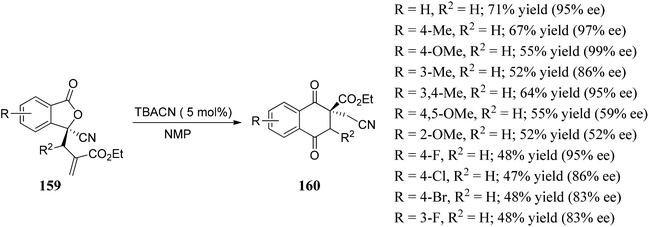 |
| Scheme 30 Annulation reaction of 3-substituted phthalides. | |
4.1.2 Hauser–Kraus annulation to give naphthoquinol–carbohydrate hybrids. Chakraborty and co-workers95 described a regioselective approach to naphthoquinone/naphthoquinol–carbohydrate hybrids using 3-cyano phthalides 161 as one of the essential precursors. In this approach, anionic annulation of 3-cyano phthalides 161 takes place with an acrylate appended sugar moiety 162 (Scheme 31).
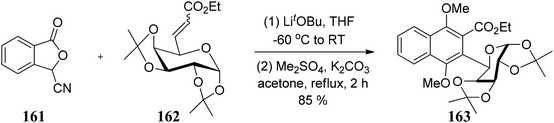 |
| Scheme 31 Hauser–Kraus annulation to give naphthoquinol–carbohydrate hybrids. | |
4.1.3 Total synthesis of uncialamycin via Hauser–Kraus annulation. Nicolaou and co-workers96 described the total synthesis of uncialamycin, and a Hauser–Kraus annulation was employed as one of the critical reactions for the synthesis of uncialamycin core (Scheme 32).
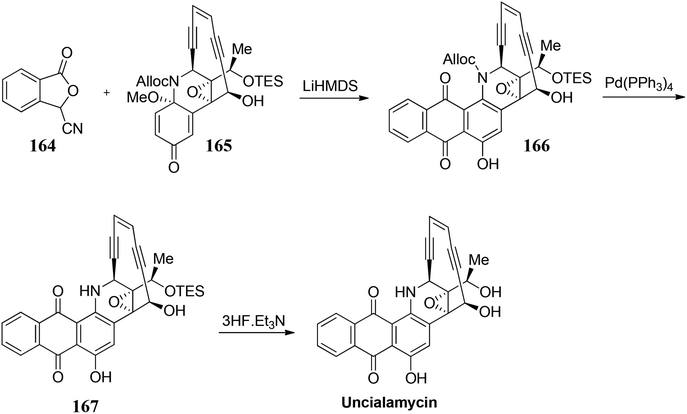 |
| Scheme 32 Total synthesis of uncialamycin. | |
4.1.4 Total synthesis of the griseusin B scaffold (bioactive natural product) via Hauser–Kraus annulation. Naysmith and co-workers97 developed a convergent route for the synthesis of the griseusin B scaffold (bioactive natural product). The main steps of the synthetic journey include the highly effective one-pot Hauser–Kraus annulation followed by methylation and double deprotection–spirocyclization sequence that directly results in the target tetracyclic ring system (Scheme 33).
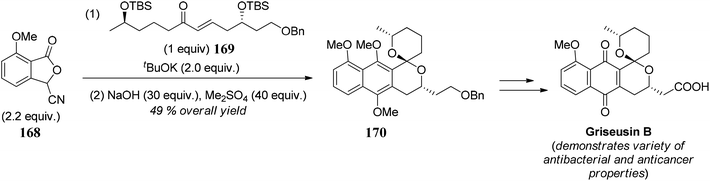 |
| Scheme 33 Total synthesis of the griseusin B scaffold. | |
4.2 Total synthesis of (−)-α-noscapine
(−)-α-Noscapine (narcotine), which was initially isolated from Papaver somniferum L.,98 is a non-addictive anti-tussive agent with little to no significant toxicity.99 (−)-α-noscapine also displays other probable scientific utilities100 for the treatment of life-threating diseases. Naturally occurring noscapine consists of two adjacent chiral centers: one at C-5′ position of tetrahydroisoquinoline ring and another at the C-3 position of phthalide framework.
Xu and co-workers101 commenced with the synthesis of meconine-3-carboxylic acid 173, which could be synthesized from pure 2,3-dimethoxybenzoic acid 171 and glyoxylic acid 172 in the presence of a conc. H2SO4. While the amine functionality 174 could be easily prepared from gallic acid over a nine-step sequence. The amide bond (C5′–C3 bond formation) was formed from the acyl chloride derivative of 173 and free amine 174 to give compound 175 in 89% yield (Scheme 34). The next step of the sequence was Bischler–Napieralski reaction in the presence of POCl3. The cyclization took place efficiently to give imine, which was further reduced to afford tetrahydroisoquinoline 176. After extensive optimization of NaBH4/NaBH3CN mediated reduction, it was concluded that low reaction temperature was critical for the high diastereoselectivity and moderately high yields. Subsequently, Eschweiler–Clarke reaction was used to obtain an N-methylated compound, 177 in 75% yield. RANEY® Ni was used for hydrogenation of 177 to produce target compound 8. Further recrystallization of the crude sample gave pure (±)-α-noscapine 8.
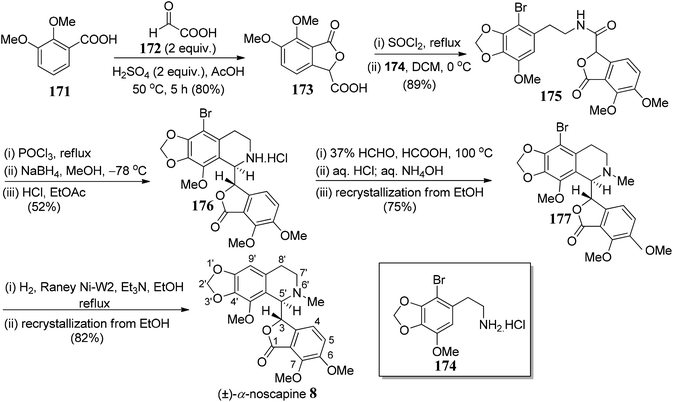 |
| Scheme 34 Total synthesis of (±)-α-noscapine. | |
4.3 Total synthesis of olaparib
Olaparib is an FDA approved targeted therapy for the treatment of cancer. It is a PARP inhibitor, inhibiting poly ADP ribose polymerase (PARP), an enzyme that plays a role in DNA repair. It targets cancer cells in people with hereditary BRCA1 or BRCA2 mutations, which include some ovarian, breast, and prostate cancers.102
Lou and co-workers103 demonstrated an effective protocol for the synthesis of olaparib. The synthesis initiated by 3-phosphono-phthalide 178, which on further reaction with aromatic aldehyde 179 gave 180, which further on reaction with hydrazine hydrate underwent ring expansion to give 181. 181 on amide coupling with 182 gave the desired drug, olaparib (Scheme 35).
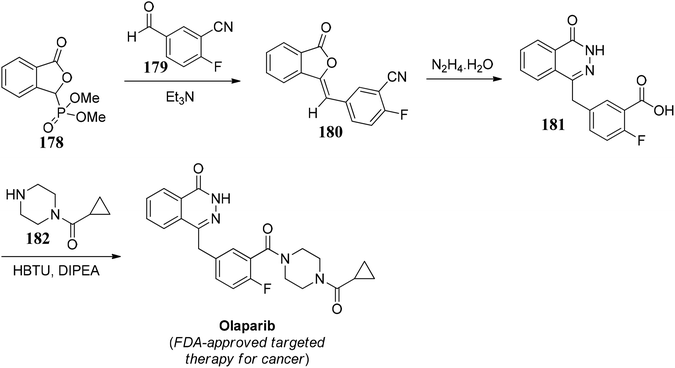 |
| Scheme 35 Synthesis of FDA approved the anti-cancer drug olaparib. | |
4.4 Synthesis of cytogenin (a bioactive natural product)
Gadakh104 and co-workers developed a route for the synthesis of 3-carbethoxy-isocoumarins 184. The reagent system used in this methodology is DEAD/PPh3/TBHP through 1,2-shift intramolecular ring expansion, or we can say that the simple elimination is dependent on the various functional groups present on 3-substituted phthalides (Scheme 36).
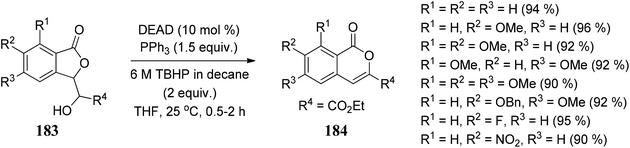 |
| Scheme 36 Intramolecular ring expansion of 3-substituted phthalides to isocoumarins. | |
The methodology is also used to synthesize cytogenin (a bioactive natural product) (Scheme 37).
 |
| Scheme 37 Synthesis of cytogenin'. | |
4.5 InBr3-catalyzed Friedel–Crafts reaction on 3-indolyl-substituted phthalides to develop unsymmetrical bis(indolyl)methanes (BIMs)
Lin and co-workers105 developed a convenient, efficient, and novel synthetic route for synthesizing the unsymmetrical bis(indolyl)methanes (BIMs) 189 via InBr3-catalyzed Friedel–Crafts reaction by reacting indoles 188 with 3-indolyl-substituted phthalides 187 in water to obtain 189 in excellent yields (Scheme 38). These BIMs compounds present have excellent anti-Alzheimer's disease activity.
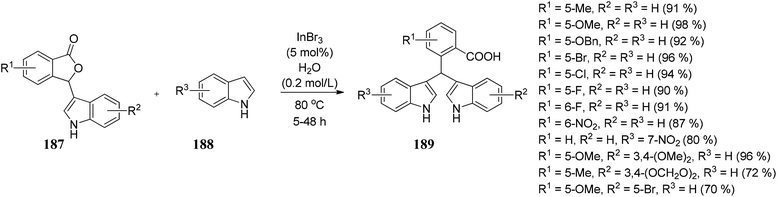 |
| Scheme 38 InBr3 catalyzed Friedel–Crafts reaction between indole and 3-indolyl-substituted phthalides. | |
5. Conclusions
In the past decade, there has been considerable attention in the area of phthalides (more specifically 3-substituted phthalides) due to the development of various phthalides-based drugs. This has led to the development of elegant research methodologies with diverse applications in academic and industrial laboratories on micro- and macroscale operations. In light of the continued research in the area of 3-substituted phthalides, we have made an effort to present a critical review on the chemistry of 3-substituted phthalides. The chemistry of phthalides has been reviewed, but an independent and detailed review on the chemistry of 3-substituted phthalides is unavailable.
We have reviewed the isolation and biological activities of various 3-substituted phthalides. We have presented pivotal research methodologies for the synthesis of racemic and chiral 3-substituted phthalides. These newer approaches are essential for the development of newer and elegant strategies for the synthesis of phthalide-based or similar molecular architecture with broader substrate scope and higher stereoselectivities. Also, we have reviewed the application of 3-substituted phthalides as a precursor for the synthesis of natural products and their analogs. Through this review, we have provided enough contextual information on the chemistry of 3-substituted phthalides, which can inspire organic chemists to develop methodologies for the synthesis of biologically and medicinally important molecules.
Conflicts of interest
The authors declare no conflict of interest.
Acknowledgements
This work was supported by DST, and CSIR, New Delhi. A. A and G. R gratefully acknowledge DST-INSPIRE and Department of Chemistry, University of Delhi, Delhi, for their respective fellowships. M. S thanks UGC for the award of DSKPDF.
References
- G. M. Cragg, D. J. Newman and K. M. Sander, J. Nat. Prod., 1997, 60, 52 CrossRef CAS PubMed.
- T. K. Devo and A. I. Scott, Handbook of Naturally Occurring Compounds, Elsevier, 1975 Search PubMed.
-
(a) S. F. Brady, M. M. Wagenaar, M. Sing, J. E. Janso and J. Clardy, J. Org. Lett., 2000, 2, 404 Search PubMed;
(b) G. Lin, S. S. K. Chan, H. S. Chung and S. L. Li, Stud. Nat. Prod. Chem., 2005, 32, 611 CAS.
- P. M. Hon, C. M. Lee, T. F. Choang, K. Y. Chui and H. N. C. Wong, Phytochemistry, 1990, 29, 1189 CrossRef CAS.
- R. Karmakar, P. Pahari and D. Mal, Chem. Rev., 2014, 114(12), 6213 CrossRef CAS PubMed.
- Risk Evaluation and Mitigation Strategy (REMS) Under Review for CellCept and Myfortic, U.S. Food and Drug Administration, retrieved 9th March 2019 Search PubMed.
- X. Diao, P. Deng, C. Xie, X. Li, D. Zhang, Y. Zhang and X. Chen, Drug Metab. Dispos., 2013, 41, 430 CrossRef CAS PubMed.
-
(a) V. Snieckus, Chem. Rev., 1990, 90, 879 CrossRef CAS;
(b) D. Mal and P. Pahari, Chem. Rev., 2007, 107, 1892 CrossRef CAS PubMed;
(c) C. D. Donner, Tetrahedron, 2013, 69, 3747 CrossRef CAS;
(d) E. Hernández, J. M. Vélez and C. P. Vlaar, Tetrahedron Lett., 2007, 48, 8972 CrossRef PubMed;
(e) K. Rathwell and M. A. Brimble, Synthesis, 2007, 5, 643 Search PubMed;
(f) A. S. Mitchell and R. A. Russell, Tetrahedron, 1995, 51, 5207 CrossRef CAS.
- K. Yoganathan, C. Rossant, S. Ng, Y. Huang, M. S. Butler and A. D. Buss, J. Nat. Prod., 2003, 66, 1116 CrossRef CAS PubMed.
- F. O. Shode, A. S. Mahomed and C. B. Rogers, Phytochemistry, 2002, 61, 955 CrossRef CAS PubMed.
- A. Arnone, G. Assante, G. Nasini and O. Vajna de Pava, Phytochemistry, 1990, 29, 613 CrossRef CAS.
- D. Wild, G. Toth and H. U. Humpf, J. Agric. Food Chem., 2002, 50, 3999 CrossRef CAS PubMed.
-
(a) M. Singh and N. P. Argade, Org. Chem., 2010, 75, 3121 CrossRef CAS PubMed;
(b) D. Mal and P. Pahari, Chem. Rev., 2007, 107, 1892 CrossRef CAS PubMed;
(c) S. Natelson and A. Pearl, J. Am. Chem. Soc., 1936, 58, 2448 CrossRef CAS.
- W. Davies and W. H. Perkin, J. Chem. Soc., Trans., 1922, 121, 2202 RSC.
- E. L. Eliel, A. W. Burgstahler, D. E. Rivard and L. Haefele, J. Am. Chem. Soc., 1955, 77, 5092 CrossRef CAS.
- M. Watanabe, M. Tsukazaki, Y. Hamada, M. Iwao and S. Furukawa, Chem. Pharm. Bull., 1989, 37, 2948 CrossRef CAS.
- V. Nair, R. R. Paul and E. Suresh, Synthesis, 2010, 21, 3741 CrossRef.
- E. T. da Penha, J. A. Forni, A. F. P. Biajoli and C. R. D. Correia, Tetrahedron Lett., 2011, 52, 6342 CrossRef CAS.
- C. Len and B. Renoux, Targets Heterocycl. Syst., 2005, 9, 311 CAS.
-
(a) J. Taunton, J. L. Wood and S. L. Schreiber, J. Am. Chem. Soc., 1993, 115, 10378 CrossRef CAS;
(b) G. Sartori, F. Bigi, X. Tao, C. Porta, R. Maggi, G. Predieri, M. Lanfranchi and M. A. Pellinghelli, J. Org. Chem., 1995, 60, 6588 CrossRef CAS;
(c) M. L. Patil, H. B. Borate, D. E. Ponde, B. M. Bhawal and V. H. Deshpande, Tetrahedron Lett., 1999, 40, 4437 CrossRef CAS;
(d) M. L. Patil, H. B. Borate, D. E. Ponde and V. H. Deshpande, Tetrahedron, 2002, 58, 6615 CrossRef CAS;
(e) K. Knepper, R. E. Ziegert and S. T. Brase, Tetrahedron, 2004, 60, 8591 CrossRef CAS;
(f) V. Gonnot, S. Tisserand, M. Nicolas, R. Baati and C. Mioskowski, Tetrahedron Lett., 2007, 48, 7117 CrossRef CAS;
(g) Z. Fei and F. E. McDonald, Org. Lett., 2007, 9, 3547 CrossRef CAS PubMed.
- For selected examples, see:
(a) A. V. Karnik and S. S. Kamath, Synthesis, 2008, 12, 1832 CrossRef;
(b) K. Tanaka, T. Osaka, K. Noguchi and M. Hirano, Org. Lett., 2007, 9, 1307 CrossRef CAS PubMed;
(c) R. Pedrosa, S. Sayaleroy and M. Vicente, Tetrahedron, 2006, 62, 10400 CrossRef CAS;
(d) M. Kosaka, S. Sekiguchi, J. Naito, M. Uemura, S. Kuwahara, M. Watanabe, N. Harada and K. Hiroi, Chirality, 2005, 17, 218 CrossRef CAS PubMed;
(e) B. Witulski and A. Zimmermann, Synlett, 2002, 11, 1855 CrossRef;
(f) M. Takahashi, N. Sekine, T. Fujita, S. Watanabe, K. Yamaguchi and M. Sakamoto, J. Am. Chem. Soc., 1998, 120, 12770 CrossRef CAS.
- G. Lin, S. S. K. Chan, H. S. Chung and S. L. Li, Stud. Nat. Prod. Chem., 2005, 32, 611 CAS.
- J. J. Beck and S. C. Chou, J. Nat. Prod., 2007, 70, 891 CrossRef CAS PubMed.
- S. Sasidharan, Y. Chen, D. Saravanan, K. M. Sundram and L. Y. Latha, Afr. J. Tradit., Complementary Altern. Med., 2011, 8, 1 CrossRef CAS.
- S. C. Lao, S. P. Li, K. K. W. Kan, P. Li, J. B. Wan, Y. T. Wang, T. T. X. Dong and K. W. K. Tsim, Anal. Chim. Acta, 2004, 526, 131 CrossRef CAS.
- P. Li, S. P. Li, S. C. Lao, C. M. Fu, K. K. W. Kan and Y. T. Wang, J. Pharm. Biomed. Anal., 2006, 40, 1073 CrossRef CAS PubMed.
- H. Mitsuhashi, U. Nagai, T. Muramatsu and H. Tashiro, Chem. Pharm. Bull., 1960, 8, 243 CrossRef CAS.
- Y. H. Li, S. L. Peng, Y. Zhou, K. B. Yu and L. S. Ding, Planta Med., 2006, 72, 652 CrossRef CAS PubMed.
- Y. Wei, W. Huang and Y. Gu, J. Chromatogr., 2013, 1284, 53 CrossRef CAS PubMed.
- R. Tomar, A. Sahni, I. Chandra, V. Tomar and R. Chandra, Mini-Rev. Org. Chem., 2018, 15, 345 CrossRef CAS.
- R. Figueroa, R. P. Hsung and C. C. Guevarra, Org. Lett., 2007, 9, 4857 CrossRef CAS PubMed.
- J. Li, L. Li, Y. Si, X. Jiang, L. Guo and Y. Che, Org. Lett., 2011, 13, 2670 CrossRef CAS PubMed.
- T. Ohzeki and K. Mori, Biosci., Biotechnol., Biochem., 2003, 67, 2584 CrossRef CAS PubMed.
- J. Fuska, D. Uhrin, B. Proksa, Z. Voticky and J. Ruppeldt, J. Antibiot., 1986, 39, 1605–1608 CrossRef CAS PubMed.
- J.-H. Huang and G. A. R. Johnston, Br. J. Pharmacol., 1990, 99, 727 CrossRef CAS PubMed.
- C. Puder, A. Zeeck and W. Beil, J. Antibiot., 2000, 53, 329 CrossRef CAS PubMed.
- K. Tianpanich, S. Prachya, S. Wiyakrutta, C. Mahidol, S. Ruchirawar and P. Kittakoop, J. Nat. Prod., 2011, 74, 79 CrossRef CAS PubMed.
- H. Inouye, T. Okuda, Y. Hirata, N. Nagakura and M. Yoshizaki, Chem. Pharm. Bull., 1967, 15, 786 CrossRef PubMed.
- A. Arnone, G. Assante, G. Nasini, S. Strada and A. Vercesi, J. Nat. Prod., 2002, 65, 48–50 CrossRef CAS PubMed.
-
(a) R. Jadulco, G. Brauers, R. A. Edrada, R. Ebel, V. Wray, S. Sudarsono and P. Proksch, J. Nat. Prod., 2002, 65, 730 CrossRef CAS PubMed;
(b) U. Höller, J. B. Gloer and D. T. Wicklow, J. Nat. Prod., 2002, 65, 876 CrossRef PubMed.
- H. Ishii, T. Ishikawa and J. Haginiwa, Yakugaku Zasshi, 1977, 97, 890 CrossRef CAS PubMed.
-
(a) S. N. Yeola and R. S. Mali, ChemInform, 1987, 18, 639 CrossRef;
(b) P. J. Choi, J. Sperry and M. A. Brimble, J. Org. Chem., 2010, 75, 7388 CrossRef CAS PubMed.
- F. Konno, T. Ishikawa, M. Kawahata and K. Yamaguchi, J. Org. Chem., 2009, 74, 9818 CrossRef PubMed.
- D. J. Williams, Tetrahedron Lett., 1973, 14, 693 CrossRef.
-
(a) L. Rodriguez-Hahn, R. O'Reilly, B. Esquivel, E. Maldonado, A. Ortega, J. Cárdenas and R. A. Toscano, J. Org. Chem., 1990, 55, 3522 CrossRef CAS;
(b) G. Xu, L. Peng, X. Niu, Q. Zhao, R. Li and H. Sun, Helv. Chim. Acta, 2004, 87, 949 CrossRef CAS.
- W. M. Abdel Mageed, B. F. Milne, M. Wagner, M. Schumacher, P. Sandor, W. Pathom, M. Goodfellow, A. T. Bull, K. Horikoshi, R. Ebel, M. Diederich, H.-P. Fiedler and M. Jaspars, Org. Biomol. Chem., 2010, 8, 2352 RSC.
- K. Liberra, R. Jansen and U. Lindequist, Pharmazie, 1998, 53, 578 CAS.
- H. Mitsuhashi, T. Muramatsu, U. Nagai, T. Nakano and K. Ueno, Chem. Pharm. Bull., 1963, 11, 1317 CrossRef CAS PubMed.
- J. A. Palermo, M. V. R. Brasco, C. Pagnuolo and A. M. Seldes, J. Org. Chem., 2000, 65, 4482 CrossRef CAS PubMed.
- A. Arnone, G. Assante, G. Nasini and O. Vajna de Pava, Phytochemistry, 1990, 29, 613 CrossRef CAS.
- J. Liu, F. Li, E. L. Kim, J. L. Li, J. Hong, K. S. Bae, H. Y. Chung, H. S. Kim and J. H. Jung, J. Nat. Prod., 2011, 74, 1823 Search PubMed.
- G. Strobel, E. Ford, J. Worapong, J. K. Harper, A. M. Arif, D. M. Grant, P. C. W. Fung and R. M. W. Chau, Phytochemistry, 2002, 60, 179 CrossRef CAS PubMed.
- L. Xu, Z. He, J. Xue, X. Chen and X. Wei, J. Nat. Prod., 2010, 73, 885 CrossRef CAS PubMed.
- J. Kitajima, T. Ishikawa and M. Satoh, Phytochemistry, 2003, 64, 1003 CrossRef CAS PubMed.
- X. D. Qin, Z. J. Dong, J. K. Liu, L. M. Yang, R. R. Wang, Y. T. Zheng, Y. S. Wu and Q. T. Zheng, Helv. Chim. Acta, 2006, 89, 127 CrossRef CAS.
- D. Y. Kong, X. J. Liu, M. K. Teng and Z. H. Rao, Acta Pharmacol. Sin., 1985, 20, 747 CAS.
- X. D. Qin, Z. J. Dong, J. K. Liu, L. M. Yang, R. R. Wang, T. T. Zheng, Y. Lu, Y. S. Wu and Q. T. Zheng, Helv. Chim. Acta, 2006, 89, 127 CrossRef CAS.
- F. Asari, T. Kusumi, G. Z. Zheng, Y. Z. Cen and H. Kakisawa, Chem. Lett., 1990, 19, 1885 CrossRef.
- M. Tada and K. Chiba, Agric. Biol. Chem., 1984, 48, 1367 CAS.
- F. O. Shde, A. S. Mahomed and C. Rogers, Phytochemistry, 2002, 61, 955 CrossRef.
- M. Chinworrungsee, P. Kittakoop, M. Isaka, R. Chanphen, M. Tanticharoen and Y. Thebtaranonth, J. Chem. Soc., Perkin Trans. 1, 2002, 22, 2473 RSC.
- K. Tianpanich, S. Prachya, S. Wiyakrutta, C. Mahidol, S. Ruchirawat and P. Kittakoop, J. Nat. Prod., 2011, 74, 79 CrossRef CAS PubMed.
- W. C. Tayone, M. Honma, S. Kanamaru, S. K. Tanaka, T. Nehira and M. Hashimoto, J. Nat. Prod., 2011, 74, 425 CrossRef CAS PubMed.
- J. Jeon, E. Julianti, H. Oha, W. Park, D. C. Oh, K. B. Oh and J. Shin, Tetrahedron Lett., 2013, 54, 3111 CrossRef CAS.
- A. Leon, R. A. Toscano, J. Tortoriello and G. Delgado, Nat. Prod. Res., 2011, 25, 1234 CrossRef CAS PubMed.
- P. Forgacs, J. Provost, A. Touche and A. Jehanno, J. Nat. Prod., 1986, 49, 178 CrossRef CAS.
- J. Fan, P.-M. Wang, J.-N. Wang, X. Zhao, Z.-W. Liu, J.-F. Wei and X.-Y. Shi, Sci. China: Chem., 2018, 61, 153 CrossRef CAS.
- T. V. Nguyen, W. J. Yoo and S. Kobayashi, Green Chem., 2017, 19, 2501 RSC.
- A. Arcadi, G. Fabrizi, A. Goggiamani and F. Marinelli, J. Org. Chem., 2015, 80, 6986 CrossRef CAS PubMed.
- T. Matsuda, K. Suzuki, S. Abe, H. Kirikae and N. Okada, Tetrahedron, 2015, 71, 9264 CrossRef CAS.
- P. Gandeepan, P. Rajamalli and C. H. Cheng, Chem.–Eur. J., 2015, 21, 9198 CrossRef CAS PubMed.
- S.-i. Kawaguchi, K. Nakamura, K. Yamaguchi, Y. Sato, Y. Gonda, M. Nishioka, M. Sonoda, A. Nomoto and A. Ogawa, Eur. J. Org. Chem., 2017, 5343 CrossRef CAS.
- L. Jia and F. Han, J. Org. Chem., 2017, 13, 1425 CAS.
- M. Perillo, A. Di Mola, R. Filosa, L. Palombi and A. Massa, RSC Adv., 2014, 4, 4239 RSC.
- S. W. Youn, H. S. Song and J. H. Park, Org. Biomol. Chem., 2014, 12, 2388 RSC.
- H. Tang, X. Zhang, A. Song and Z. Zhang, Mod. Res. Catal., 2012, 1, 11 CrossRef CAS.
- G. Li, D. Yin and X. T. Liang, Synth. Commun., 2004, 34, 1183 CrossRef CAS.
- J. Tatsugi, T. Hara and Y. Izawa, Chem. Lett., 1997, 26, 177 CrossRef.
- H. Huang, Y. Wang, H. Zong and L. Song, Appl. Organomet. Chem., 2019, 33, e4643 CrossRef.
- A. M. Carlos, R. Stieler and D. S. Lüdtke, Org. Biomol. Chem., 2019, 17, 283 RSC.
- S. Kattela, E. C. de Lucca Jr and C. R. D. Correia, Chem.–Eur. J., 2018, 24, 17691 CrossRef CAS PubMed.
- Y. Ge, Z. Han, Z. Wang, C. G. Feng, Q. Zhao, G. Q. Lin and K. Ding, Angew. Chem., Int. Ed., 2018, 130, 13324 CrossRef.
- B. Zhang, M. H. Xu and G. Q. Lin, Org. Lett., 2009, 11, 4712 CrossRef CAS PubMed.
- S. V. Kumbhar and C. Chen, RSC Adv., 2018, 8, 41355 RSC.
- B. Lu, M. Zhao, G. Ding, X. Xie, L. Jiang, V. Ratovelomanana-Vidal and Z. G. Zhang, ChemCatChem, 2017, 9, 3989 CrossRef CAS.
- S. W. Youn, H. S. Song and J. H. Park, Org. Lett., 2014, 16, 1028 CrossRef CAS PubMed.
- Z. B. Zhang, Y. Q. Lu and X. F. Duan, Synthesis, 2011, 3435 CAS.
- F. A. Davis and Y. W. Andemichael, J. Org. Chem., 1999, 64, 8627 CrossRef CAS.
- S. K. Ray, M. M. Sadhu, R. G. Biswas, R. A. Unhale and V. K. Singh, Org. Lett., 2019, 21, 417 CrossRef CAS PubMed.
- H. W. Moore, Science, 1977, 197, 527 CrossRef CAS PubMed.
- F. M. Hauser and R. P. Rhee, J. Org. Chem., 1978, 43, 178 CrossRef CAS.
- G. A. Kraus and H. Sugimoto, Tetrahedron Lett., 1978, 43, 2263 CrossRef.
- A. K. Chaturvedi and N. Rastogi, J. Org. Chem., 2016, 81, 3303 CrossRef CAS PubMed.
- Z. Zhuang, Z. P. Hu and W. W. Liao, Org. Lett., 2014, 16, 3380 CrossRef CAS PubMed.
- S. Chakraborty, G. Das, S. Ghosh and D. Mal, Org. Biomol. Chem., 2016, 14, 10636 RSC.
- K. C. Nicolaou, Y. Wang, M. Lu, D. Mandal, M. R. Pattanayak, R. Yu and L. Pasunoori, J. Am. Chem. Soc., 2016, 138, 8235 CrossRef CAS PubMed.
- B. J. Naysmith and M. A. Brimble, Org. Lett., 2013, 15, 2006 CrossRef CAS PubMed.
- V. Tomar, S. Kukreti, S. Prakash, J. Madan and R. Chandra, Curr. Top. Med. Chem., 2017, 17, 174 CrossRef CAS PubMed.
-
(a) D. W. Empey, L. A. Laitinen, G. A. Young, C. E. Bye and D. T. D. Hughes, Eur. J. Clin. Pharmacol., 1979, 16, 393 CrossRef CAS PubMed;
(b) B. Dahlström, T. Mellstrand, C. G. Löfdahl and M. Johansson, Eur. J. Clin. Pharmacol., 1982, 22, 535 CrossRef PubMed.
-
(a) J. Zhou, D. Panda, J. W. Landen, L. Wilson and H. C. Joshi, J. Biol. Chem., 2002, 277, 39777 CrossRef CAS PubMed;
(b) Y. Ke, K. Ye, H. E. Grossniklaus, D. R. Archer, H. C. Joshi and J. A. Kapp, Cancer Immunol. Immunother., 2000, 49, 217 CrossRef CAS PubMed;
(c) J. W. Landen, R. Lang, S. J. McMahon, N. M. Rusan, A. M. Yvon, A. W. Adams and D. R. Archer, Cancer Res., 2002, 62, 4109 CAS;
(d) J. W. Landen, V. Hau, M. Wang, T. Davis, B. Ciliax, B. H. Wainer and D. R. Archer, Cancer Res., 2004, 10, 5187 CAS.
- J. Ni, H. Xiao, L. Weng, X. Wei and Y. Xu, Tetrahedron, 2011, 67, 5162 CrossRef CAS.
- P. C. Fong, D. S. Boss, T. A. Yap, A. Tutt, P. Wu, M. Mergui-Roelvink and A. Ashworth, N. Engl. J. Med., 2009, 361, 123 CrossRef CAS PubMed.
- X.-y. Lou, X. Yang, Y.-l. Ding, J.-j. Wang, Q.-y. Yan, X.-g. Huang, Y.-h. Guo, X.-j. Wang and W.-s. Xiang, Chem. Res. Chin. Univ., 2013, 29, 231 CrossRef CAS.
- S. K. Gadakh and A. Sudalai, RSC Adv., 2014, 4, 57658 RSC.
- H. Lin, Y. Zang, X. Sun and G. Lin, J. Chem., 2012, 30, 2309 CAS.
|
This journal is © The Royal Society of Chemistry 2020 |
Click here to see how this site uses Cookies. View our privacy policy here.