DOI:
10.1039/D0RA01033B
(Paper)
RSC Adv., 2020,
10, 9730-9735
Stereoselective synthesis of (+)-5-thiosucrose and (+)-5-thioisosucrose†
Received
3rd February 2020
, Accepted 28th February 2020
First published on 6th March 2020
Abstract
(+)-5-Thiosucrose 1, a novel isosteric sulfur analog of sucrose, was synthesized stereoselectively for the first time via indirect β-D-fructofuranosidation involving selective β-D-psicofuranosidation, followed by stereo-inversion of the secondary hydroxy group at the C-3 position on the furanose ring. Glycosidation of protected 5-thio-D-glucose with a D-psicofuranosyl donor provided β-D-psicofuranosyl 5-thio-α-D-glucopyranoside and that with D-fructofuranosyl donor gave α-D-fructofuranosyl 5-thio-α-D-glucopyranoside. Two anomeric stereocenters of the glycosyl donor and acceptor were controlled correctly to provide a single disaccharide among four possible anomeric isomers in the glycosylation. Conversion of the resulting disaccharides afforded (+)-5-thiosucrose 1 and (+)-5-thioisosucrose 2 in excellent yields, respectively. Inhibitory activities of 1 and 2 against α-glucosidase in vitro were also examined.
Introduction
Sucrose (Fig. 1) is one of the common sugars in nature and is an important carbohydrate for energy source in human health. At the same time, it is also a favorite sweetener in our daily life. So far, a variety of synthetic analogs of sucrose have been synthesized as low- or noncalorie alternatives for a healthy dietary purpose. For example, sucralose (Splenda®) is a widely known analog of sucrose in which three hydroxy groups are replaced by chlorine atoms, leading to a hundredfold increase in sweetness compared to that of sucrose.1 Many other derivatives of sucrose with different substituents have also been reported.2 However sucrose analogs in which an atom in the sucrose ring system is changed are far less common. As rare examples, C-sucrose, in which the glycosidic oxygen is replaced by a carbon atom, was synthesized by Kishi et al., and hemicarbasucrose, a carba-analog of sucrose, was reported by Jiménez-Barbero and Sollogoub et al.3 Although sucrose is an actual substrate for α-glucosidase, these modified sucrose analogs are not substrates of α-glycosidase and non-notable activities in inhibition against α-glycosidase were reported.
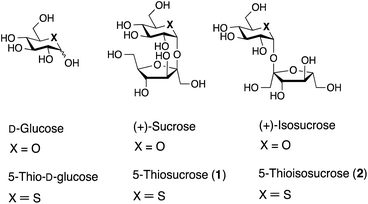 |
| Fig. 1 Structures of glucose, sucrose, isosucrose, and their sulfur analogs. | |
Thiosugars replace a ring oxygen atom with a sulfur atom in carbohydrate, and are extremely rare in nature with the exception of 5-thio-D-mannose4 and salacinols.5 It should be noted that salacinol has potent enzymatic inhibitory activity against α-glucosidase. In fact, it has been already approved and used commercially in the context of dietary drinks or supplement of foods. Hetero monosaccharides, including thiosugars,6 azasugars,7 and carbasugars,8 and their disaccharide analogs9 have been synthesized as sugar mimics and their biological behaviors and functions involving α-glucosidase inhibitory activity were examined.10 However, there has been nothing potent beyond salacinol concerning α-glucosidase inhibition.11,12
α-Glucosidase hydrolyzes sucrose to fructose and glucose, and interestingly, 5-thio-D-glucose inhibits this process.11a,f A pyranose ring oxygen is essentially required for the substrates of α-glucosidase.13 As an isosteric analog of sucrose, 5-thiosucrose 1 and 5-thioisosucrose 2 possesses a 5-thioglucose moiety and would resemble the conformation of sucrose. We thought that 1 would be of interest as a sweetener as well as an inhibitor superior than 5-thio-D-glucose. This research is focused on the stereoselective synthesis of 5-thiosucrose 1 and 5-thioisosucrose 2, and herein we report their synthesis and some biological properties.
Results and discussion
Although many methods for the stereoselective glycosylation have been reported and utilized,14 early attempts at the synthesis of sucrose15 revealed the difficulties involved with the stereocontrol of its two anomeric centers. For the synthesis of 1 and 2, two anomeric carbon centers connected with β,α- and α,α-O-linkage [1; β-D-Fruf-(2↔1)-α-D-Glcp and 2; α-D-Fruf-(2↔1)-α-D-Glcp] need to be controlled in a glycosylation step. An α-selectivity rather than β-selectivity was reported for glycosidation of D-fructose as an either glycosyl acceptor or donor.14a In fact, glycosidation of D-fructofuranose affords an α-anomer or α-predominant mixtures in most cases.16 On the other hand, 5-thio-D-glucose has been used as a glycosyl donor to form an α-glycosidic bond by the anomeric effect of the sulfur ring.6 However, it has never been used as an acceptor in glycosidation reaction to our best knowledge.
Despite these failures in β-D-fructofuranosidation, the natural occurrence of β-D-furanoside can be found in sucrose and inulin. Therefore, β-directing D-fructofuranosylation has been a challenging task and this has encouraged carbohydrate chemists to develop selective β-D-fructofuranosylation. There is only one elegant example in β-D-fructofuranosylation, reported by Oscarson et al.17 As shown in Scheme 1, they used ethyl thioglycoside 3 as a D-fructofuranosyl donor, in which the C-1 and C-4 hydroxy groups are fixed with a connection of cyclic disiloxyether to block an attack from the α-face of the furanose ring. Stereoselective glycosylation of the acceptor 4 with 3 promoted by dimethyl(methylthio)sulfonium triflate (DMTST) in the presence of 2,6-di-tert-butyl-4-methylpyridine (DTBMP) and 4 Å molecular sieves occurred to give β-furanosyl α-pyranoside 5 with 68% yield, exclusively.17a
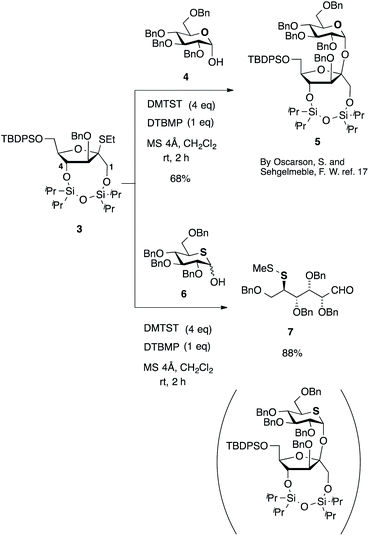 |
| Scheme 1 Reaction of Oscarson's glycosyl donor 3 with acceptors 4 and 6. | |
Accordingly, we initially attempted the Oscarson's method for the synthesis of 1. However, glycosidation of 2,3,4,6-tetra-O-benzyl-5-thio-D-glucopyranose (6)18 with 3 gave no desired disaccharide. Instead, disulfide 7 was isolated in 88% yield. Activation of sulfide by DMTST occurred on the endo-sulfide of the acceptor 6 instead of the exo-sulfide of the donor 3. Therefore, this method could be useful for general oxygen sugars but not for thiosugars.
Since Oscarson's β-D-fructofuranosylation method was found to be incompatible with the synthesis of 1, we turned our attention to indirect synthesis through β-D-psicofuranosylation, which was employed in the stereoselective synthesis of sucrose previously.19 We have reported that glycosidation of D-glucopyranose with D-psicofuranosyl donor20 protecting 3,4-diols with acetonide gave β-D-psicofuranosyl α-D-glucopyranoside, which afforded sucrose after several steps. D-Psicofuranosyl donor was regarded as β-D-fructofuranosyl donor in the disaccharide syntheses.19,21 On the other hand, glycosylation of D-glucopyranose with D-fructofuranosyl donor occurred to give α-D-fructofuranosyl α-D-glucopyranoside predominantly which was used for the synthesis of isosucrose. The course of α-D-fructofuranosylation or β-D-psicofuranosylation could be governed by the stereochemistry of the C-3 hydroxy substituent. Glycosidation of acceptor with D-fructofuranosyl donor is not sufficient for the syntheses of β-D-fructofuranosides, but is suitable for α-D-fructofuranoside. Based on the above results, synthetic plan for 1 and 2 is depicted in Fig. 2.
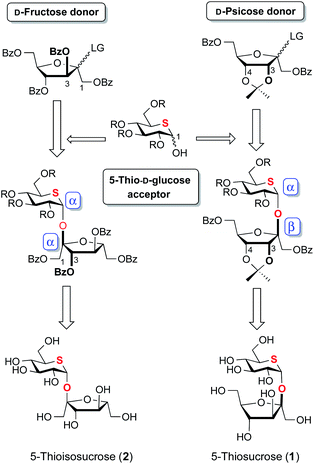 |
| Fig. 2 Synthetic plan for 5-thiosucrose 1 and 5-thioisosucrose 2. | |
D-Fructofuranosyl donor with 5-thioglucose acceptor would give α-D-fructofuranosyl 5-thio-α-D-glucopyranoside, of which anomeric centers would matched with the stereochemistry of 2. Glycosylation of 5-thioglucose acceptor with D-psicofuranosyl donor would give β-D-psicofuranosyl 5-thio-α-D-glucopyranoside of which anomeric centers would matched with the stereochemistry of 1. This disaccharide will lead to 1 after a stereo-inversion at the C-3 hydroxy group. In both cases, α-glycoside on the anomeric center of 5-thioglucopyranose would be formed by the strong anomeric effect of the thiane ring.
Synthesis of 5-thioisosucrose (2)
Several D-fructofuranosyl donors are available. Fructofuranosyl halide,16a fructofuranosyl phosphite,16c 2-O-acetylfructofuranose,16e 2-thiofructofuranoside,16d and fructofuranosyl N-phenyltrifluoroacetimidate16f have been reported. Nevertheless, most of these glycosidations entail synthetic difficulties since D-fructose possesses a sterically congested anomeric hydroxy group due to the structure of ketohexofuranose. In addition, the choice of leaving groups is restricted in this case because a sensitive cyclic sulfide unit exists in the glycosyl acceptor for the synthesis of 2.
As shown in Scheme 2, we examined three different D-fructofuranosyl donors, thus imidate donor22 8b, phosphite donor 8c,16c and benzyl phthalate donor23 8d. These donors were prepared from 1,3,4,6-tetra-O-benzoyl-D-fructofuranose (8a).16b Trimethylsilyl trifluoromethanesulfonate (TMSOTf) promoted glycosylation of 2,3,4,6-tetra-O-acetyl-5-thio-D-glucose 9 (ref. 18) with each glycosyl donor 8b, 8c, or 8d24 at −40 °C in dichloromethane in the presence of 4 Å molecular sieves resulted in the formation of 2α,1α-disaccharide 10 [α-D-Fruf-(2↔1)-α-D-Glcp] as a single isomer with 73%, 80%, and 70% yields, respectively. It is noteworthy that a single isomer (2α,1α) was formed exclusively among the four possible anomeric isomers (2β,1α, 2α,1α, 2α,1β, and 2β,1β) in this glycosidation, while D-glucopyranosyl acceptor reported in the synthesis of isosucrose produced two anomeric isomers (2α,1α, 2α,1β) in moderate selectivities (47
:
53,16a 4
:
1,16c and 84
:
16 (ref. 16e)). Although the three fructofuranosyl donors used in this study showed similar reactivity and selectivity, phthalate donor 8d is regarded as the most convenient donor because it is readily prepared and stable under storage, in comparison with other donors 8b and 8c.
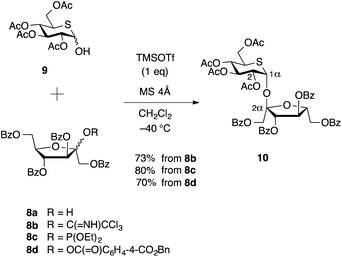 |
| Scheme 2 α-D-Fructofuranosylation of 5-thio-D-glucopyranose 9. | |
Stereochemistry at the anomeric positions in 10 was determined by the coupling constant in the 1H NMR spectrum and the chemical shifts in the 13C{1H} NMR spectrum. The J1,2 value of the pyranose ring proton is 3.1 Hz, which is in the typical value of α-glucopyranoside, and it is identical to that of 1,2-cis-α-D-glucopyranoside. The 13C{1H} NMR chemical shift of the anomeric position of the furanose ring exhibits at 109.0 ppm, which is identified to that of α-D-fructofuranoside.17a,25 These data supported the structure of the disaccharide 10.
Finally, treatment of 10 with NaOMe in MeOH furnished the synthesis of 2 in 90% yield (Scheme 3). Compound 2 was synthesized in two steps from D-fructose donor with strict stereocontrol of two anomeric centers. The corresponding octaacetate 11 was prepared in 89% yield in order to compare the analytical data with the related disaccharides shown in Table 1.
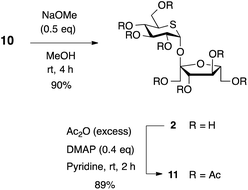 |
| Scheme 3 Synthesis of 5-thioisosucrose 2 and its acetate 11. | |
Table 1 Comparison of octaacetyl disaccharides 18, 11, 19, and 20
Compound |
Specific rotationa |
Chemical shifts of furanose protonsb (δ) |
Coupling constants of furanose protonsb (Hz) |
[α]D |
H-1a |
H-1b |
H-3 |
H-4 |
H-5 |
H-6a |
H-6b |
J3,4 |
J4,5 |
J5,6a |
J5,6b |
CHCl3 was used as a solvent. Benzene-d6 was used as a solvent. These values were obtained from the literature.30 |
18 |
+67.6 |
4.38 |
4.28 |
5.71 |
5.62 |
4.22 |
4.52 |
4.52 |
5.9 |
5.6 |
5.5 |
5.5 |
11 |
+147.8 |
4.52 |
4.29 |
5.69 |
4.87 |
4.55 |
4.54 |
4.30 |
0.5 |
3.3 |
4.1 |
7.7 |
19c |
+60.0 |
4.37 |
4.29 |
5.71 |
5.55 |
4.20 |
4.41 |
4.41 |
5.4 |
5.7 |
5.4 |
5.4 |
20c |
+83.5 |
4.79 |
3.98 |
5.69 |
4.84 |
4.45 |
4.39 |
3.96 |
1.0 |
3.0 |
4.0 |
2.0 |
Synthesis of 5-thiosucrose (1)
According to the synthetic plan, we started the synthesis of 1 through β-D-psicofuranosidation. The initial step of this synthesis involved β-selective glycosidation of 6 with the O-protected D-psicofuranosyl donor 12,18,19,24 which was prepared from D-psicose in 49% overall yield in five steps.20b Reaction of 6 with 12 in the presence of TMSOTf in dichloromethane at −40 to −20 °C afforded the desired glycoside 13 in 76% yield as a single stereoisomer (Scheme 4). The configuration of the anomeric center on the pyranoside ring in 13 was identified as that of α-D-glucopyranoside by a J1,2 value of 2.9 Hz. The anomeric center in D-psicofuranoside was identical to β-glycoside. In general, the C2-carbon of psicofuranoside appears between 107–109 ppm for β anomers and between 103–105 ppm for α anomers,19,20 and the 13C{1H} NMR chemical shift of the anomeric carbon existed at 109.9 ppm in 13.
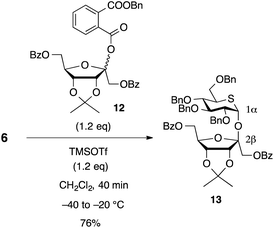 |
| Scheme 4 Psicofuranosylation with 5-thio-α-D-glucopyranose 6. | |
In this reaction, protected 5-thioglucose 6 performed an α-directing acceptor as same as 9 in the synthesis of 5-thioisosucrose. The glycosidation occurred on the β-face of the furanose donor 12 because of the steric influence of the acetonide group existing on the α-side of the ring. Considering that D-psicofuranosylation of the corresponding α-D-glucose gave a mixture in the ratio of 2
:
1 (α-glucopyranoside vs. β-glucopyranoside),19 it should be noted that the predominant selectivity for 5-thio-D-glucopyranose 6 vs. D-glucopyranose is quite interesting in psicofuranosylation of 5-thiopyranose and pyranose donors.26
Conversion of β-D-psicofuranoside 13 to β-D-fructofuranoside 17 was carried out in four steps (Scheme 5):
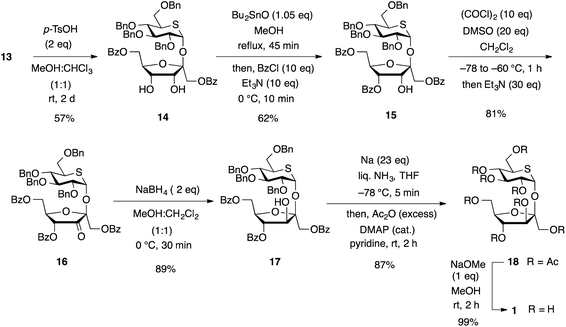 |
| Scheme 5 Synthesis of 1. | |
Deprotection of acetonide group in 13 with p-toluenesulfonic acid in MeOH gave diol 14 in 57% yield. Benzoate 15 was obtained from 14 via a stannylene intermediate. Treatment of 14 with Bu2SnO in MeOH at reflux temperature followed by benzoylation on the C-4 hydroxy group with benzoyl chloride gave 15 selectively.27 The Swern conditions will be a choice for oxidation of the secondary hydroxy group in the presence of cyclic sulfide. The secondary alcohol of 15 was oxidized smoothly to give ketone 16 in 81% yield. Then, reduction of the ketone 16 with NaBH4 occurred from the bottom of the furanose ring selectively to convert to β-D-fructofuranoside 17 in 89% yield. The direction of hydride attack in the reduction of 3-ketone is controlled by the adjacent 2-β-glycosidic bond to give 2,3-syn-product.28 Removal of both O-benzoyl and O-benzyl groups in disaccharide 17 under the Birch conditions and successive acetylation gave octaacetate 18 in 87% yield. After deprotection of all acetyl groups under Zemplén's conditions,29 the synthesis of 1 was accomplished in 99% yield.
Stereochemistry
The specific rotations, and chemical shifts and coupling constant of 1H NMR for octaacetates of 5-thiosucrose and 5-thioisosucrose (18 and 11) are summarized with the corresponding data reported for sucrose and isosucrose (19 and 20)30 in Table 1. Their structures are shown in Fig. 3. Specific rotation values of 5-thioisosucrose and isosucrose (11 and 20) are relatively larger than those of 5-thiosucrose and sucrose (18 and 19). Chemical shifts of each α,α-anomers (11 and 20) and those of β,α-anomers (18 and 19) are comparable in 1H NMR. The chemical shifts of the H-4 protons in furanose ring are characteristic. Their difference (ca. 0.7 ppm) can be observed between 19 and 20, and thioanalogs 18 and 11. Coupling constants of J3,4 and J4,5 in sucrose 19 and thiosucorse 18 are larger than those of isosucrose 20 and thioisosucorse 11. Thus, all these results supported the structures of 5-thiodisaccharides 11 and 18.
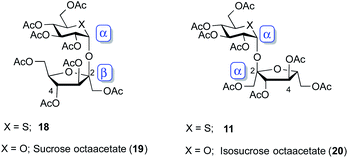 |
| Fig. 3 Octaacetate of 5-thiosucrose 18, 5-thioisososucrose 11, sucrose 19, and isosucrose 20. | |
Biological study
Inhibitory activities against α-glycosidase for compounds 1, 2, and 5-thio-D-glucose were examined in vitro using rat intestinal α-glucosidase. In literature, 5-thio-D-glucose is reported to be a weak to moderate inhibitor for α-glucosidase.11f,12 In the present study, 5-thio-D-glucose showed 48% inhibition at 8 mM. It was regrettable that neither 1 nor 2 exhibited any inhibition at 8 mM, while commonly used α-glucosidase inhibitor such as acarbose and voglibose work at nM levels. Although 5-thio-D-glucose has a sweet taste, 1 was found to be a little bitter rather than sweet, in rough-and-ready taste analyses.
Conclusions
The first stereoselective syntheses of 1 and 2 were achieved by stereoselective glycosidation. The key steps involved D-fructofuranosylation and D-psicofuranosylation of protected 5-thio-D-glucose acceptors 9 and 6 to afford α-D-fructofuranosyl 5-thio-α-D-glucopyranoside 10 and β-D-psicofuranosyl 5-thio-α-D-glucopyranoside 13 with high stereoselectivity in excellent yields, respectively. The configurations of their two-anomeric centers were strictly controlled in a single glycosidation step, in which the strong anomeric effect of 5-thio-D-glucopyranose was observed. We have demonstrated that 5-thio-D-glucopyranose works as an α-directing glycosyl acceptor for the first time. Although neither 1 nor 2 exhibit α-glucosidase inhibitory activity or sweetness, current results will aid in the design of new α-glucosidase inhibitors and the synthesis of other disaccharide of thiosugar derivatives.
Conflicts of interest
There are no conflicts to declare.
Acknowledgements
We thank professor Hisashi Matsuda and professor emeritus Masayuki Yoshikawa of Kyoto Pharmaceutical University to guide our experiments in α-glucosidase assay.
Notes and references
-
(a) M. R. Jenner, Sucralose: unveiling its properties and applications, in Progress in Sweeteners, ed. T. H. Grenby, Elsevier Applied Science, London, U.K., 1989, pp. 121–141 Search PubMed;
(b) V. L. Grotz, S. Molinary, R. C. Peterson, M. E. Quinlan and R. Reo, Sucralose, in Alternative Sweetners, ed. L. O'Brien-Nabors, CRC Press, Boca Raton, FL, 4th edn, 2006, pp. 181–196 Search PubMed.
-
(a) L. Hough and K. S. Mufti, Carbohydr. Res., 1973, 29, 291 CrossRef CAS;
(b) I. D. Jenkins and S. Thang, Aust. J. Chem., 1984, 37, 1925 CrossRef CAS;
(c) C. Simiand, E. Samain, O. R. Martin and H. Driguez, Carbohydr. Res., 1995, 267, 1 CrossRef CAS PubMed;
(d) F. W. Lichtenthaler and S. Mondel, Carbohydr. Res., 1997, 303, 293 CrossRef CAS.
-
(a) U. C. Dyer and Y. Kishi, J. Org. Chem., 1988, 53, 3383 CrossRef CAS;
(b) D. J. O'Leary and Y. Kishi, J. Org. Chem., 1993, 58, 304 CrossRef;
(c) B. López-Méndez, C. Jia, Y. Zhang, L.-H. Zhang, P. Sinaÿ, J. Jiménez-Barbero and M. Sollogoub, Chem.–Asian J., 2008, 3, 51 CrossRef PubMed.
- R. J. Capon and J. K. MacLeod, J. Chem. Soc., Chem. Commun., 1987, 1200 RSC.
-
(a) M. Yoshikawa, T. Murakami, H. Shimada, H. Matsuda, J. Yamahara, G. Tanabe and O. Muraoka, Tetrahedron Lett., 1997, 38, 8367 CrossRef CAS;
(b) M. Yoshikawa, T. Murakami, K. Yashiro and H. Matsuda, Chem. Pharm. Bull., 1998, 46, 1339 CrossRef CAS PubMed;
(c) M. Yoshikawa, T. Morikawa, H. Matsuda, G. Tanabe and O. Muraoka, Bioorg. Med. Chem., 2002, 10, 1547 CrossRef CAS PubMed;
(d) O. Muraoka, K. Yoshikai, H. Takahashi, T. Minematsu, G. Lu, G. Tanabe, T. Wang, H. Matsuda and M. Yoshikawa, Bioorg. Med. Chem., 2006, 14, 500 CrossRef CAS PubMed.
-
(a) J. G. Fernandez-Bolaños, N. A. L. Al-Masoudi and I. Maya, Adv. Carbohydr. Chem. Biochem., 2001, 57, 21 CrossRef;
(b) M. Sakono, A. Seko, Y. Takeda, M. Hachisu, A. Koizumi, K. Fujikawa, H. Seto and Y. Ito, RSC Adv., 2016, 6, 76879 RSC; 5-thioglucose;
(c) M. S. Feather and R. L. Whistler, Tetrahedron Lett., 1962, 15, 667 CrossRef;
(d) N. A. Hughes, J. Chem. Soc., Chem. Commun., 1979, 319 RSC;
(e) H. Yuasa, J. Tamura and H. Hashimoto, J. Chem. Soc., Perkin Trans. 1, 1990, 2763 RSC;
(f) H. Driguez and B. Henrissat, Tetrahedron Lett., 1981, 22, 5061 CrossRef CAS;
(g) J. Uenishi and H. Ohmiya, Tetrahedron, 2003, 59, 7011 CrossRef CAS; 5-thiogalactose;
(h) J. E. N. Shin and A. S. Perlin, Carbohydr. Res., 1979, 76, 165 CrossRef CAS; 5-thiomannose;
(i) H. Yuasa, Y. Izukawa and H. Hashimoto, J. Carbohydr. Chem., 1989, 8, 753 CrossRef CAS.
-
(a) H. J. M. Gijsen, L. Qiao, W. Fitz and C.-H. Wong, Chem. Rev., 1996, 96, 443 CrossRef CAS PubMed;
(b) V. H. Lillelund, H. H. Jensen, X. Liang and M. Bols, Chem. Rev., 2002, 102, 515 CrossRef CAS PubMed; 5-azaglucose (nojirimycin);
(c) S. Inoue, T. Tsuruoka, T. Iyo and T. Niida, Tetrahedron, 1968, 24, 2125 CrossRef.
-
(a) T. Suami and S. Ogawa, Adv. Carbohydr. Chem. Biochem., 1990, 48, 21 CrossRef CAS PubMed;
(b) O. Arjona, A. M. Gómez, L. J. Cristóbal and J. Plumet, Chem. Rev., 2007, 107, 1919 CrossRef CAS PubMed; voglibose;
(c) S. Horii, H. Fukase, T. Matsuo, Y. Kameda, N. Asano and K. Matsui, J. Med. Chem., 1986, 29, 1038 CrossRef CAS PubMed.
-
(a) S. Mehta and B. M. Pinto, Tetrahedron Lett., 1992, 33, 7675 CrossRef CAS;
(b) H. Yuasa, O. Hindsgaul and M. M. Palcic, J. Am. Chem. Soc., 1992, 114, 5891 CrossRef CAS;
(c) S. Mehta, K. L. Jordan, T. Weimar, U. C. Kreis, R. J. Batchelor, F. W. B. Winstein and B. M. Pinto, Tetrahedron: Asymmetry, 1994, 5, 2367 CrossRef CAS;
(d) H. Hashimoto, M. Kawanishi and H. Yuasa, Chem.–Eur. J., 1996, 2, 556 CrossRef CAS PubMed;
(e) B. D. Johnston and B. M. Pinto, J. Org. Chem., 1998, 63, 5797 CrossRef CAS PubMed;
(f) Y. Morii, H. Matsuda, K. Ohara, M. Hashimoto, K. Miyairi and T. Okuno, Bioorg. Med. Chem., 2005, 13, 5113 CrossRef CAS PubMed.
- C.-H. Wong, Y. Ichikawa, T. Krach, C. G.-L. Narvor, D. P. Dumas and G. C. Look, J. Am. Chem. Soc., 1991, 113, 8137 CrossRef CAS.
-
(a) D. J. Hoffman and R. L. Whistler, Biochemistry, 1968, 7, 4479 CrossRef CAS PubMed;
(b) R. L. Whistler and W. C. Lake, Biochem. J., 1972, 130, 919 CAS;
(c) B. Hellman, Å. Lernmark, J. Sehlin, I.-B. Täljedal and R. L. Whistler, Biochem. Pharmacol., 1973, 22, 29 CrossRef CAS PubMed;
(d) J. R. Zysk, A. A. Bushway, R. L. Whistler and W. W. Carlton, J. Reprod. Fertil., 1975, 45, 69 CrossRef CAS PubMed;
(e) J. H. Kim, S. H. Kim, E. W. Hahn and C. W. Song, Science, 1978, 200, 206 CrossRef CAS PubMed;
(f) Y. L. Merrer, M. Fuzier, I. Dosbaa, M.-J. Foglietti and J.-C. Depezay, Tetrahedron, 1997, 53, 16731 CrossRef.
- T. Kajimoto, K. K.-C. Liu, R. L. Pederson, Z. Zhong, Y. Ichikawa, J. A. Porco Jr and C.-H. Wong, J. Am. Chem. Soc., 1991, 113, 6187 CrossRef CAS.
-
(a) S. Chiba, Biosci., Biotechnol., Biochem., 1997, 61, 1233 CrossRef CAS PubMed;
(b) E. B. de Melo, A. S. Gomes and I. Carvalho, Tetrahedron, 2006, 62, 10277 CrossRef.
-
(a) P. Fügedi, Glycosylation methods, in The Organic Chemistry of Sugars, ed. D. E. Levy and P. Fügedi, CRC Press, Boca Raton, FL, 2006 Search PubMed;
(b) Handbook of Chemical Glycosylation, ed. A. V. Demchenko, WILEY-VCH, Weinheim, Germany, 2008 Search PubMed.
-
(a) R. U. Lemieux and G. Huber, J. Am. Chem. Soc., 1953, 75, 4118 CrossRef CAS;
(b) R. U. Lemieux and G. Huber, J. Am. Chem. Soc., 1956, 78, 4117 CrossRef CAS;
(c) H. Tsuchida and M. Komoto, Agric. Biol. Chem., 1965, 29, 239 CAS;
(d) R. K. Ness and H. G. Fletcher Jr, Carbohydr. Res., 1971, 17, 465 CrossRef CAS;
(e) D. E. Iley and B. Fraser-Reid, J. Am. Chem. Soc., 1975, 97, 2563 CrossRef CAS;
(f) B. Fraser-Reid and D. E. Iley, Can. J. Chem., 1979, 57, 645 CrossRef CAS.
-
(a) A. Klemer, K. Gaupp and E. Buhe, Tetrahedron Lett., 1969, 52, 4585 CrossRef;
(b) A. Bouali, G. Descotes, D. F. Ewing, A. Grouiller, J. Lefkidou, A.-D. Lespinasse and G. Mackenzie, J. Carbohydr. Chem., 1992, 11, 159 CrossRef CAS;
(c) T. Müller, R. Schneider and R. R. Schmidt, Tetrahedron Lett., 1994, 35, 4763 CrossRef;
(d) Y.-L. Li and Y.-L. Wu, Tetrahedron Lett., 1996, 37, 7413 CrossRef CAS;
(e) T. Yamanoi, N. Misawa and M. Watanabe, Tetrahedron Lett., 2007, 48, 6458 CrossRef CAS;
(f) G. Lian, Q. Gao and F. Lin, Carbohydr. Res., 2008, 343, 2992 CrossRef CAS PubMed.
-
(a) S. Oscarson and F. W. Sehgelmeble, J. Am. Chem. Soc., 2000, 122, 8869 CrossRef CAS;
(b) S. Oscarson and F. W. Sehgelmeble, J. Org. Chem., 2002, 67, 8457 CrossRef CAS PubMed;
(c) S. Oscarson and F. W. Sehgelmeble, Tetrahedron: Asymmetry, 2005, 16, 121 CrossRef CAS.
- An α-anomer exists predominantly (α
:
β = >10
:
1) in 6 and 9. - J. Uenishi and A. Ueda, Tetrahedron: Asymmetry, 2008, 19, 2210 CrossRef CAS.
- Other examples of β-D-psicofuranosylations;
(a) J. Uenishi and A. Ueda, Heterocycles, 2009, 77, 1297 CrossRef CAS;
(b) A. Ueda, T. Yamashita and J. Uenishi, Carbohydr. Res., 2010, 345, 1722 CrossRef CAS PubMed;
(c) A. Ueda, Y. Nishimura, Y. Makura, M. Tanaka and J. Uenishi, Heterocycles, 2018, 97, 729 CrossRef CAS.
- A. Ueda, T. Yamashita and J. Uenishi, Heterocycles, 2010, 81, 1711 CrossRef CAS.
- R. R. Schmidt and J. Michel, Angew. Chem., Int. Ed. Engl., 1980, 19, 731 CrossRef.
- K. S. Kim, Y. J. Lee, H. Y. Kim, S. S. Kang and S. Y. Kwon, Org. Biomol. Chem., 2004, 2, 2408 RSC.
- A mixture of α- and β-anomers; for 8d (α
:
β = 1
:
1.2) and for 12 (α
:
β = 1
:
15). - S. J. Angyal and G. S. Bethell, Aust. J. Chem., 1976, 29, 1249 CrossRef CAS.
- The similar results were observed in the case of D-glucopyranosylation of D-fructofuranose as well as D-tagatofuranose. The glycosylation of 4 with D-fructofuranose 8c gave a mixture of pyranosides, α-D-Fruf-(2↔1)-α-D-Glcp and α-D-Fruf-(2↔1)-β-D-Glcp, in a 5
:
4 ratio. Y. Makura, A. Ueda, T. Matsuzaki, T. Minamino and M. Tanaka, Tetrahedron, 2019, 75, 3758 CrossRef CAS. - D. Wagner, J. P. H. Verheyden and J. G. Moffatt, J. Org. Chem., 1974, 39, 24 CrossRef CAS.
-
(a) A. Földesi, T. V. Maltseva, Z. Dinya and J. Chattopadhyaya, Tetrahedron, 1998, 54, 14487 CrossRef;
(b) A. Földesi, A. Trifonova, Z. Dinya and J. Chattopadhyaya, J. Org. Chem., 2001, 66, 6560 CrossRef PubMed.
- G. Zemplén and E. Pacsu, Ber. Dtsch. Chem. Ges., 1929, 62, 1613 CrossRef.
- G. R. Newkome, J. D. Sauer, V. K. Majestic, N. S. Bhacca, H. D. Braymer and J. D. Wander, Carbohydr. Res., 1976, 48, 1 CrossRef CAS.
Footnote |
† Electronic supplementary information (ESI) available: Experimental details, copies of 1H, 13C, and gCOSY NMR spectra. See DOI: 10.1039/d0ra01033b |
|
This journal is © The Royal Society of Chemistry 2020 |
Click here to see how this site uses Cookies. View our privacy policy here.