DOI:
10.1039/D0RA01559H
(Paper)
RSC Adv., 2020,
10, 13149-13154
Synthesis and characterization of poly(tetraphenylimidazole)s and their application in the detection of fluoride ions†
Received
18th February 2020
, Accepted 24th March 2020
First published on 1st April 2020
Abstract
Here, we describe the synthesis and characterization of a silyl protected tetraphenylimidazole monomer and its homo and co-polymer. The requisite monomer was accessed by Suzuki–Miyaura cross-coupling of 2-(1-(4-bromophenyl)-4,5-diphenyl-1H-imidazol-2-yl)phenol and 4-vinylphenylboronic acid followed by protection of the phenolic group by tert-butyl(chloro)diphenylsilane. The desired polymers were readily synthesized by using free radical polymerization. Both the polymers and monomer were characterized using different analytical techniques including multinuclear NMR, GPC (for polymers), and single crystal X-ray crystallography (for the monomer). By utilizing the greater fluorophilicity of the silyl atom, the polymers were studied as probes for the detection of fluoride ions. The selectivity and sensitivity of the synthesized polymers were investigated in detail.
Introduction
The development of selective and efficient sensors is of prime importance owing to their applications in biological, and analytical processes.1–8 A number of sensors or probes have been reported for the detection of different analytes based on intramolecular charge transfer,9,10 photoinduced electron transfer,11–15 fluorescence resonance energy transfer,16–20 excimer/exciplex formation,21–23 metal–ligand charge transfer,24–27 and twisted intramolecular transfer.28 Another unique process known as excited state intramolecular proton transfer (ESIPT) has given promising materials with applications ranging from organic light emitting diodes to fluorescent probes.29–34 Among the different ESIPT molecules, imidazoles (or) imidazole analogues containing 2-hydroxyphenyl have been studied to a greater extent due to their potential application in electroluminescent, laser dyes and as chemosensor materials.34–38 Using this unique process of 2-hydroxyphenyl imidazoles (or) imidazole analogues, several anion probes have been developed.39–43 The respective imidazole polymers are particularly interesting due to their high thermal stability, the opportunity of using solution processing techniques for device fabrication and so on. Recently, Lin and co-workers44 synthesized thieno-imidazole based polymers and used as probes for the detection of iron, mercury or zinc. Mallavia and co-workers45 have synthesized new fluorescent conjugated polymer microsphere for sensing copper in aqueous solution. Several methodologies have been reported for the incorporation of imidazole moieties into the backbone of the polymers.46–50 However, in comparison with polymers that contain imidazoles in the main chain, polymers with imidazoles especially tetraarylimidazoles as side-chain have received far less attention and the side chain functionalization of imidazoles has not been systematically studied.51–55 Recently, Lu and co-workers56 have reported long fluorescence lifetime europium-complexes of imidazole. In spite of these recent encouraging results, the use of imidazoles in side chain polymers especially for sensing applications are scarcely studied.
Fluoride ion, play an important role in human health where it is primarily used for the treatment of osteoporosis, prevention of dental caries and enamel demineralization.57 Although low doses of fluoride is beneficial, high doses of fluoride ion is dangerous and can cause acute toxicity in humans and animals.58 Hence control of fluoride consumption is a great concern in most of the countries. Over the last few decades, a number of fluoride ion sensors have been reported,59–62 however most of them were based on small molecules. Recently, polymer based sensors/probes gained attention owing to their advantageous features over small-molecule based sensors. For example, Kim and Swager described a novel method for the detection of fluoride ion taking advantage of fluorine affinity towards silicon.63 Jäkle and co-workers64 reported an elegant boron based block co-polymer method for the detection of fluoride ion. With the aim to develop ESIPT based polymers for the detection of fluoride ion we report design and synthesis of silyl protected imidazole based polymers and their sensing ability towards fluoride anion.
Results and discussion
The silylated imidazole monomer 3 was synthesized in three steps. The starting material, compound 1 was synthesized in 69% yield, using one-step condensation reaction of benzil, salicylaldehyde, 4-bromo aniline with ammonium acetate by adopting literature procedures (ESI†).65,66 The styryl containing compound 2 (70%) was made via Suzuki–Miyaura cross-coupling between 4-vinylphenylboronic acid and brominated tetraarylimidazole (1). Furthermore, silylated compound 367 was prepared by treating compound 2 with tert-butyl(chloro)diphenylsilane in the presence of a base (Scheme 1). Compound 2 and monomer 3 were characterized by multinuclear NMR and HRMS. Monomer 3 was further confirmed by single crystal X-ray crystallography which crystallizes in a monoclinic system with a space group P21/c. The phenyls attached to the imidazole ring are arranged in a propeller fashion with a torsion angle ranging from 24.37 to 78.11° (Fig. 1). Compound 3 was polymerized using 2,2′-azobis(isobutyronitrile) (AIBN) as the initiator under typical free radical polymerization conditions. We also made random co-polymer in 74% yield using di(ethylene glycol) methyl ether methacrylate as the second monomer to have better solubility in organic solvents (Scheme 2).
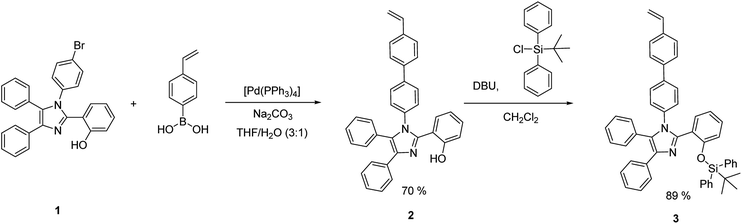 |
| Scheme 1 Synthetic route to the monomer. | |
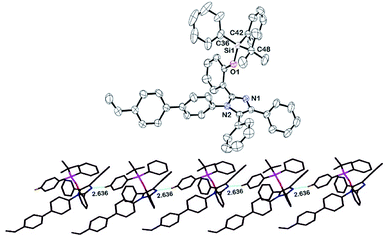 |
| Fig. 1 Molecular structure of the silyl protected monomer (3). (top) ORTEP view with thermal ellipsoids at 30% probability level (hydrogen atoms are omitted for the clarity). Selected bond lengths (Å) and bond angles (°) are as follows: Si1–O1 1.6484(12), Si1–C36 1.866(2), Si1–C42 1.867(2), Si1–C48 1.8807(18), O1–Si1–C36 107.97(9), O1–Si1–C42 108.36(8), O1–Si1–C48 103.53(7), C36–Si1–C42 112.68(9). Angle between the plane constructed by the imidazole ring and the neighbouring phenyl groups are 24.37, 49.03, 74.45 and 78.11. (bottom) Packing diagram with intermolecular C–H⋯π interaction. | |
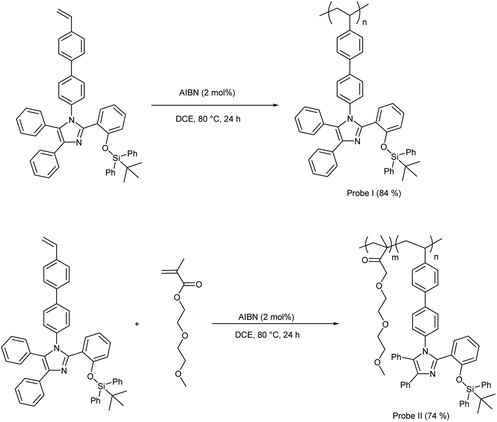 |
| Scheme 2 Synthetic route to the homo- (probe I) and random co-polymer (probe II). | |
The resultant polymers were purified by reprecipitation of dichloromethane solution of polymers in hexanes. Both the polymers were fully characterized by NMR (1H, 13C and 29Si) spectroscopy. 1H NMR spectrum of the polymers has shown characteristic broadening features in their peaks and complete disappearance of the AMX patterned vinyl protons (Fig. 2). Moreover the ratio of the repeat unit of compound 3 to di(ethylene glycol) methyl ether methacrylate in the random co-polymer was found to be 1
:
1, as elucidated using 1H NMR. The molecular weight of the polymers were studied by gel-permeation chromatography (GPC) in tetrahydrofuran using narrow polystyrene standards. The weight-average molecular weights of the homo- and random co-polymer are 16
700 and 25
400 with polydispersity indices (Ð) of 2.51 and 1.95 respectively (ESI†). Based on the GPC and the 1H NMR data, the composition of the random co-polymer was deduced as m
:
n = 14
:
14. The thermal stability of the resultant polymers were analysed using thermogravimetric analysis (Fig. S16†) which reveal that the probes are thermally stable (PDT for probe I = 380 °C and for probe II = 320 °C; ESI†). Furthermore, differential scanning colorimetric studies reveal that probes I & II have high glass transition temperature (Tg) (Tg for probe I is 155 °C and for probe II it is 120 °C; Table S2†).
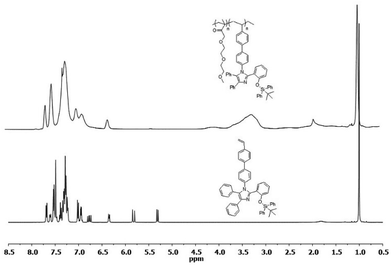 |
| Fig. 2 1H NMR spectrum of compound 3 and random co-polymer (probe II). | |
With well characterized imidazole polymers in hand, we examined their application as fluoride ion probes. Both the polymers displayed one absorption band at 275 nm and an emission band at around 382 nm in THF solutions. To test the sensing ability of the polymers in organic solvents, different equivalents of fluoride ions were added and the response was monitored by absorption as well as emission spectroscopy. On incremental addition of fluoride ions to the solutions of both the probes, the intensity of the structured emission band for the probe I at 381 nm and for the probe II at 382 nm gradually decreases along with the appearance of a new red-shifted emission band for the probe I at 485 nm whilst for the probe II at 488 nm (Fig. 3 and 4).
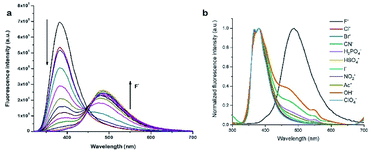 |
| Fig. 3 (a) Emission spectra of probe I (10 μM) upon gradual addition 0.1 eq. (1 μm) of TBAF (0 to 1.3 eq.) in THF when exited at 295 nm. (b) Normalized emission spectra of probe I upon incubation with 10 eq. of F−, Cl−, Br−, CN−, H2PO4−, HSO4−, I−, NO2−, OAc−, OH−, and ClO4− for 15 min. | |
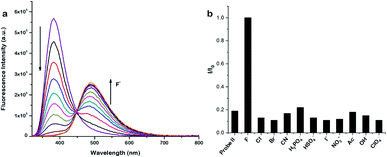 |
| Fig. 4 (a) Emission spectra of probe II (10 μm) upon gradual addition 0.1 eq. (1 μm) of TBAF (0 to 1.2 eq.) in THF when exited at 295 nm. (b) Selectivity of fluoride ion by monitoring the fluorescence intensities of probe II at 488 nm upon incubation with 10 eq. of F−, Cl−, Br−, CN−, H2PO4−, HSO4−, I−, NO2−, OAc−, OH−, and ClO4− for 15 min. | |
As demonstrated by us and others,2,3,5,6,62,63,65,66 probes I & II showed normal Stokes shifted emission (silylated compounds) and the desilylated compounds showed ESIPT emission (Fig. 5). The Stokes shift of the desilylated probes are around 100 nm. The plot of ratios of fluorescence intensities i.e. I485 nm/I381 nm vs. fluoride ion concentration for probe I shows a linear increase between 0–9 μM of fluoride ions with a detection limit of 0.003 μm but for the probe II it is 0–7 μM with a detection limit of 0.177 μm. The ratio of emission intensities varies from 9 to 19 for probe I whereas for probe II it is 11 to 39. To realize the effect of time on sensing the fluoride ion, we monitored the fluorescence intensity for 60 min. These experiments shows that our probes got quenched ≈90% (92% for probe I and 93% for probe II) within five minutes which suggest that our probes are highly sensitive (Fig. S12†).
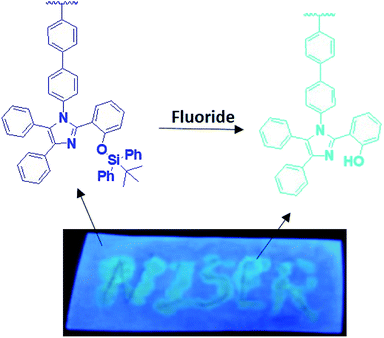 |
| Fig. 5 Schematic representation of sensing mechanism. | |
Selectivity is an important parameter for the success of a chemical probe, to investigate the selectivity of the probes for fluoride ion, we measured the fluorescence spectra of our probes with various other anions such as Cl−, Br−, CN−, I−, NO2−, OAc−, HSO4−, H2PO4−, OH− and ClO4−. As shown in Fig. 3b and 4b, addition of other anions does not results any significant change in the fluorescence behaviour of probe I & II, especially there is no emission band at ≈485 nm. This comparative study indicates that our polymeric probes (both probe I and probe II) displayed excellent selectivity for the detection of fluoride ion over other anions. We further treated our probes with fluoride ion in the presence of different anions to probe the competition of fluoride over other ions which shows that our probes are highly selective even in the presence of different anions (Fig. S13†). Our efforts to evaluate the fluoride ion sensing using 1H NMR titrations did not yield any fruitful results (Fig. S14 and S15†). Control experiments were performed to know the stability of the monomer and the probes I & II in solution. These studies reveal that the monomer and the probes I & II are stable in solution at least for 24 h (Fig. S17†). We also tested the effect of concentration and temperature of our probes along with different ions like Cl−, NO2−, HSO4−. These results reveals that there is no appreciable change in the peak position of the emission (Fig. S18 and S19†), which indicates that the probes are stable at different temperature and concentration.
Experimental
Synthesis of 2-(4,5-diphenyl-1-(4′-vinyl-[1,1′-biphenyl]-4-yl)-1H-imidazol-2-yl)phenol (2)
Under nitrogen, a biphasic solution of imidazole (1.00 g, 2.14 mmol), 4-styrene boronic acid (0.38 g, 2.57 mmol), sodium carbonate (0.68 g, 6.42 mmol) and [Pd(PPh3)4] (3 mol%) in tetrahydrofuran (THF) and water (20
:
8; 56 mL) was heated at reflux for 12 hours. The organic layer was separated and the aqueous layer was extracted twice (20 mL) with ethyl acetate. The combined organic phase was dried with sodium sulfate and concentrated under vacuum. The product was purified by column chromatography to afford off-white compound. Yield: 0.74 g (70%). 1H NMR (400 MHz, CDCl3) δ 5.33 (d, J = 12 Hz, 1H), 5.84 (d, J = 20 Hz, 1H), 6.53 (t, J = 8 Hz, 1H), 6.71 (d, J = 8 Hz, 1H), 6.78 (dd, J = 12 & 20 Hz, 1H), 7.12–7.32 (m, 12H), 7.52 (d, J = 8 Hz, 2H), 7.57–7.64 (m, 6H), 13.39 (s, 1H); 13C NMR (101 MHz, CDCl3): δ 112.88, 114.55, 117.94, 118.16, 126.42, 126.81, 127.05, 127.15, 127.24, 127.80, 128.37, 128.62, 128.91, 130.25, 130.49, 131.35, 136.15, 137.38, 138.55, 141.31, 144.84, 158.32; IR (KBr): ν(cm−1) = 3057 (s), 1602 (m), 1588 (m), 1527 (s), 1485 (m), 1443 (s), 1415 (s), 1384 (m), 1294 (s), 1261 (m), 1184 (s), 1161 (s), 1139 (s), 1074 (s), 1027 (s), 994 (s), 973 (s), 915 (s), 838 (m), 783 (m), 756 (m), 734 (m), 724 (m), 714 (m), 702 (m), 654 (s); HR-MS (ESI†): calcd for C35H26N2O ([M + H]+): 491.2118, found: 491.2133.
Synthesis of 2-(2-((tert-butyldiphenylsilyl)oxy)phenyl)-4,5-diphenyl-1-(4′-vinyl-[1,1′-biphenyl]-4-yl)-1H-imidazole (3)
To a solution of imidazole (1.00 g, 2.04 mmol) in dichloromethane, 1,8-diazabicyclo[5.4.0]undec-7-ene (DBU) (0.61 mL, 4.08 mmol) was added at −20 °C and stirred for 20 min. at this temperature, then tert-butyl(chloro)diphenyl silane (1.1 mL, 4.08 mmol) was added and the solution was allowed to warm up to room temperature and stirred at the same temperature for 12 h. After water workup, the organic layer was separated and dried over sodium sulphate and concentrated using rotary evaporator. The product was separated by silica gel column chromatography using ethyl acetate and n-hexanes (20
:
80) mixture as the eluent. Yield: 1.32 g, 89%. 1H NMR (400 MHz, CDCl3): δ 1.00 (s, 9H), 5.32 (d, J = 12 Hz, 1H), 5.82 (d, J = 16 Hz, 1H), 6.35 (d, J = 8 Hz, 1H), 6.77 (dd, J = 12 & 16 Hz, 1H), 6.94–6.96 (m, 2H), 7.01 (d, J = 8 Hz, 2H), 7.23–7.34 (m, 14H), 7.39 (t, J = 8 Hz, 2H), 7.46–7.62 (m, 8H), 7.59–7.62 (m, 1H), 7.68 (d, J = 4 Hz, 2H); 13C NMR (101 MHz, CDCl3): δ 19.54, 26.62, 114.22, 117.33, 119.27, 119.50, 120.80, 126.58, 126.70, 126.99, 127.74, 127.78, 128.09, 128.14, 129.56, 129.80, 129.89, 130.24, 130.98, 132.09, 132.30, 132.53, 135.28, 135.39, 136.28, 136.97, 138.42, 145.67, 154.20; 29Si NMR (79 MHz, CDCl3): δ −4.53; IR (KBr): ν(cm−1) = 3448 (s), 3068 (s), 3043 (s), 2956 (s), 2929 (s), 2856 (s), 1496 (m), 1472 (m), 1441 (m), 1429 (m), 1396 (m), 1280 (m), 1255 (m), 1108 (m), 922 (m), 824 (m), 749 (m), 698 (m), 502 (m); HR-MS (ESI): calcd for C51H44N2OSi ([M + H]+): 729.3296, found: 729.3296.
General procedure for the synthesis of homo- and co-polymers
Schlenk tube was charged with the monomers and the free radical initiator 2,2′-azobis(isobutyronitrile) (2 mol%). The system was purged with nitrogen and degassed dichloroethane (DCE) 1.0 mL was added. The reaction mixture was stirred at 80 °C for 24 h. The mixture was then slowly added to hexanes to precipitate the polymers. The resulting solid was redissolved in dichloroethane (1 mL) and reprecipitated from hexanes. The precipitation was repeated three times. The solid was collected and dried under high vacuum to obtain the polymers as a white solid.
Synthesis of homo-polymer (probe I)
The quantity of the silylated monomer (compound 3) (0.729 g, 1.00 mmol). Yield: 0.635 g (84%), 1H NMR (400 MHz, CDCl3): 0.90 (s, tBu-H) ppm, 1.14–2.13 (polymer backbone), 6.16–7.87 (aromatic H). 13C NMR (101 MHz, CDCl3) δ 19.48, 26.49, 40.59, 40.45, 119.21, 122.93, 123.27, 123.87, 125.26, 126.55, 127.77, 128.25, 128.46, 129.24, 129.87, 130.82, 132.27, 132.42, 135.32, 138.42, 145.22, 154.23. 29Si NMR (79 MHz, CDCl3): δ −4.55; IR (KBr): ν(cm−1) = 3422 (s), 3049 (s), 2930 (s), 2857 (s), 1604 (s), 1577 (s), 1525 (m), 1499 (m), 1428 (m), 1391 (s), 1364 (s), 1282 (s), 1252 (s), 1112 (m), 1006 (s), 917 (m), 823 (m), 754 (m), 702 (m), 652 (s), 613 (s), 540 (s), 502 (m). GPC (in tetrahydrofuran against polystyrene standards): Mn = 6,600, Ð = 2.51.
Synthesis of random co-polymer (probe II)
The quantities of the monomers are as follows: silylated monomer (compound 3) (0.729 g, 1.00 mmol) and di(ethylene glycol) methyl ether methacrylate (0.18 mL, 1.00 mmol). Yield: 0.698 g (74%), 1H NMR (400 MHz, CDCl3): 0.98 (s, 9H, tBu-H) ppm, 1.24–2.33 (polymer backbone), 2.88–4.38 (CH, CH2 & CH3), 6.16–8.04 (aromatic H). 13C NMR (101 MHz, CDCl3) δ 19.53, 26.57, 40.61, 41.06, 59.01, 61.93, 68.54. 68.87, 70.32, 71.88, 119.18, 120.71, 123.11, 126.40, 126.51, 127.65, 127.75, 128.12, 128.45, 129.33, 129.43, 130.13, 130.94, 132.21, 132.53, 134.71, 135.36, 135.52, 137.34, 138.11, 138.32, 139.40, 145.58, 154.23. 29Si NMR (79 MHz, CDCl3): δ −4.60; IR (KBr): ν(cm−1) = 3422 (s), 3049 (s), 2930 (m), 2858 (s), 1726 (s), 1604 (s), 1577 (s), 1525 (s), 1499 (m), 1471 (m), 1448 (m), 1428 (s), 1391 (m), 1364 (s), 1282 (m), 1252 (m), 1196 (s), 1111 (m), 1029 (s), 1006 (s), 961 (s), 919 (m), 823 (m), 754 (m), 700 (m), 653 (s), 613 (s), 539 (s), 502 (s). GPC (in tetrahydrofuran against polystyrene standards): Mn = 13
000, Ð = 1.95.
Conclusions
In summary, ESIPT masked tetraaryl substituted imidazole polymers (home and copolymer) have been successfully synthesized using classic free radical polymerization method. Upon addition of fluoride ion both the polymers exhibited large Stokes shift which is an ESIPT emission. Most importantly both the probes showed high selectivity for fluoride ion over other ions. We expect our results will have significant impact in developing luminescent polymers for biological imaging and organic electronics. Further studies to tune the solubility of the polymers in aqueous medium are ongoing in our group.
Conflicts of interest
There are no conflicts to declare.
Acknowledgements
The authors thank Science & Engineering Research Board (SERB) (EMR/2017/000620), New Delhi and Department of Atomic Energy (DAE) for financial support. RVRNC thank CSIR for research fellowship.
Notes and references
- E. Galbraith and T. D. James, Chem. Soc. Rev., 2010, 39, 3831 RSC.
- P. Chen, W. Bai and Y. Bao, J. Mater. Chem. C, 2019, 7, 11731 RSC.
- L. Gai, J. Mack, H. Lu, T. Nyokong, Z. Li, N. Kobayashi and Z. Shen, Coord. Chem. Rev., 2015, 285, 24 CrossRef.
- H. Kobayashi, M. Ogawa, R. Alford, P. L. Choyke and Y. Urano, Chem. Rev., 2010, 110, 2620 CrossRef CAS PubMed.
- M. H. Lee, J. S. Kim and J. L. Sessler, Chem. Soc. Rev., 2015, 44, 4185 RSC.
- S. Dhiman, M. Ahmad, N. Singla, G. Kumar, P. Singh, V. Luxami, N. Kaur and S. Kumar, Coord. Chem. Rev., 2020, 405, 213138 CrossRef CAS.
- D. Wang and B. Z. Tang, Acc. Chem. Res., 2019, 52, 2559 CrossRef CAS PubMed.
- R. Shunmugam, G. J. Gabriel, C. E. Smith, K. A. Aamer and G. N. Tew, Chem.–Eur. J., 2008, 14, 3904 CrossRef CAS PubMed.
- G. Zhou, M. Baumgarten and K. Müllen, J. Am. Chem. Soc., 2008, 130, 12477 CrossRef CAS.
- S.-Y. Li, Z.-B. Sun and C.-H. Zhao, Inorg. Chem., 2017, 56, 8705 CrossRef CAS.
- W. Sun, M. Li, J. Fan and X. Peng, Acc. Chem. Res., 2019, 52, 2818 CrossRef CAS.
- S. Madhu and M. Ravikanth, Inorg. Chem., 2014, 53, 1646 CrossRef CAS.
- K. Mawai, S. Nathani, P. Roy, U. P. Singh and K. Ghosh, Dalton Trans., 2018, 47, 6421 RSC.
- X.-H. Xu, C. Liu, Y. Mei and Q.-H. Song, J. Mater. Chem. B, 2019, 7, 6861 RSC.
- X. Pang, J. Ge, X. Yu, Y. Li, F. Shen, Y. Wang and J. Ren, New J. Chem., 2019, 43, 10554 RSC.
- W. Xu, S. Liu, H. Sun, X. Zhao, Q. Zhao, S. Sun, S. Cheng, T. Ma, L. Zhou and W. Huang, J. Mater. Chem., 2011, 21, 7572 RSC.
- B. Qiu, Y. Zeng, R. Hu, L. Chen, J. Chen, T. Yu, G. Yang and Y. Li, ACS Omega, 2018, 3, 18153 CrossRef CAS.
- M. J. Chang, K. Kim, K. S. Park, J. S. Kang, C. S. Lim, H. M. Kim, C. Kang and M. H. Lee, Chem. Commun., 2018, 54, 13531 RSC.
- C. Zhao, X. Zhang, K. Li, S. Zhu, Z. Guo, L. Zhang, F. Wang, Q. Fei, S. Luo, P. Shi, H. Tian and W.-H. Zhu, J. Am. Chem. Soc., 2015, 137, 8490 CrossRef CAS.
- K. Xu, L. He, X. Yang, Y. Yang and W. Lin, Analyst, 2018, 143, 3555 RSC.
- Q. Song, A. Bamesberger, L. Yang, H. Houtwed and H. Cao, Analyst, 2014, 139, 3588 RSC.
- L. Gai, H. Chen, B. Zou, H. Lu, G. Lai, Z. Li and Z. Shen, Chem. Commun., 2012, 48, 10721 RSC.
- S. K. Kim, J. H. Bok, R. A. Bartsch, J. Y. Lee and J. S. Kim, Org. Lett., 2005, 7, 4839 CrossRef CAS.
- Y. Kurishita, T. Kohira, A. Ojida and I. Hamachi, J. Am. Chem. Soc., 2010, 132, 13290 CrossRef CAS PubMed.
- Y. Sun, N. Ross, S.-B. Zhao, K. Huszarik, W.-L. Jia, R.-Y. Wang, D. Macartney and S. Wang, J. Am. Chem. Soc., 2007, 129, 7510 CrossRef PubMed.
- S.-B. Ko, J.-S. Lu, Y. Kang and S. Wang, Organometallics, 2013, 32, 599 CrossRef.
- R. S. Vadavi, H. Kim, K. M. Lee, T. Kim, J. Lee, Y. S. Lee and M. H. Lee, Organometallics, 2012, 31, 31 CrossRef.
- W. Che, G. Li, J. Zhang, Y. Geng, Z. Xie, D. Zhu and Z. Su, J. Photochem. Photobiol., A, 2018, 358, 274 CrossRef.
- J. E. Kwon and S. Y. Park, Adv. Mater., 2011, 23, 3615 CrossRef.
- P. Chou, D. McMorrow, T. J. Aartsma and M. Kasha, J. Phys. Chem., 1984, 88, 4596 CrossRef CAS.
- N. Suzuki, A. Fukazawa, K. Nagura, S. Saito, H. Kitoh-Nishioka, D. Yokogawa, S. Irle and S. Yamaguchi, Angew. Chem., Int. Ed., 2014, 53, 8231 CrossRef CAS.
- J. Zhao, S. Ji, Y. Chen, H. Guo and P. Yang, Phys. Chem. Chem. Phys., 2012, 14, 8803 RSC.
- N. Suzuki, K. Suda, D. Yokogawa, H. Kitoh-Nishioka, S. Irle, A. Ando, L. M. G. Abegão, K. Kamada, A. Fukazawa and S. Yamaguchi, Chem. Sci., 2018, 9, 2666 RSC.
- A. C. Sedgwick, L. Wu, H.-H. Han, S. D. Bull, X.-P. He, T. D. James, J. L. Sessler, B. Z. Tang, H. Tian and J. Yoon, Chem. Soc. Rev., 2018, 47, 8842 RSC.
- J. E. Kwon, S. Park and S. Y. Park, J. Am. Chem. Soc., 2013, 135, 11239 CrossRef CAS.
- C. H. Kim, J. Park, J. Seo, S. Y. Park and T. Joo, J. Phys. Chem. A, 2010, 114, 5618 CrossRef CAS.
- S.-J. Lim, J. Seo and S. Y. Park, J. Am. Chem. Soc., 2006, 128, 14542 CrossRef CAS.
- S. Kim, D. W. Chang, S. Y. Park, H. Kawai and T. Nagamura, Macromolecules, 2002, 35, 2748 CrossRef CAS.
- Q. Chu, D. A. Medvetz and Y. Pang, Chem. Mater., 2007, 19, 6421 CrossRef CAS.
- N. Singh, N. Kaur, R. C. Mulrooney and J. F. Callan, Tetrahedron Lett., 2008, 49, 6690 CrossRef CAS.
- T.-I. Kim, H. J. Kang, G. Han, S. J. Chung and Y. Kim, Chem. Commun., 2009, 5895 RSC.
- Y. Xu and Y. Pang, Chem. Commun., 2010, 46, 4070 RSC.
- Z. Xu, L. Xu, J. Zhou, Y. Xu, W. Zhu and X. Qian, Chem. Commun., 2012, 48, 10871 RSC.
- R. Satapathy, Y.-H. Wu and H.-C. Lin, Org. Lett., 2012, 14, 2564 CrossRef CAS PubMed.
- A. Salinas-Castillo and R. Mallavia, Chem. Commun., 2010, 46, 1263 RSC.
- M. Toba, T. Nakashima and T. Kawai, Macromolecules, 2009, 42, 8068 CrossRef CAS.
- Y. Bao, H. Wang, Q. Li, B. Liu, Q. Li, W. Bai, B. Jin and R. Bai, Macromolecules, 2012, 45, 3394 CrossRef CAS.
- T. Wang, N. Zhang, R. Bai and Y. Bao, J. Mater. Chem. C, 2018, 6, 266 CAS.
- B. S. Kumar, B. Sana, G. Unnikrishnan, T. Jana and K. S. S. Kumar, Polym. Chem., 2020, 11, 1043 RSC.
- M. Neophytou, H. A. Ioannidou, T. A. Ioannou, C. L. Chochos, S. P. Economopoulos, P. A. Koutentis, G. Itskos and S. A. Choulis, Polym. Chem., 2012, 3, 2236 RSC.
- C. G. Overberger and N. Vorchheimer, J. Am. Chem. Soc., 1963, 85, 951 CrossRef CAS.
- Q. Zeng, P. Cai, Z. Li, J. Qin and B. Z. Tang, Chem. Commun., 2008, 1094 RSC.
- S. Dong, D. Ou, J. Qin and Z. Li, J. Polym. Sci., Part A: Polym. Chem., 2011, 49, 3314 CrossRef CAS.
- X. Chen, S. Kang, M. J. Kim, J. Kim, Y. S. Kim, H. Kim, B. Chi, S.-J. Kim, J. Y. Lee and J. Yoon, Angew. Chem., Int. Ed., 2010, 49, 1422 CrossRef CAS.
- H. Pu, Y. Qin, D. Wan and Z. Yang, Macromolecules, 2009, 42, 3000 CrossRef CAS.
- C. Yang, J. Xu, J. Ma, D. Zhu, Y. Zhang, L. Liang and M. Lu, Polym. Chem., 2012, 3, 2640 RSC.
- K. L. Kirk, Biochemistry of the Elemental Halogens and Inorganic Halides, Plenum Press, New York, 1991 Search PubMed.
- M. Kleerekoper, Endocrinol. Metabol. Clin, 1998, 27, 441 CrossRef CAS.
- Y. Zhou, F. F. Zhang and J. Yoon, Chem. Rev., 2014, 114, 5511 CrossRef CAS.
- C. R. Wade, A. E. Broomsgrove, S. Aldridge and F. P. Gabbai, Chem. Rev., 2010, 110, 3958 CrossRef CAS.
- S. Yamaguchi, S. Akiyama and K. Tamao, J. Am. Chem. Soc., 2001, 123, 11372 CrossRef CAS.
- K. Dhanunjayarao, V. Mukundam and K. Venkatasubbaiah, J. Mater. Chem. C, 2014, 2, 8599 RSC . (references cited therein).
- T. H. Kim and T. M. Swager, Angew. Chem., Int. Ed., 2003, 42, 4803 CrossRef CAS.
- F. Cheng, E. M. Bonder and F. Jäkle, J. Am. Chem. Soc., 2013, 135, 17286 CrossRef CAS.
- S. Park, O.-H. Kwon, S. Kim, S. Park, M.-G. Choi, M. Cha, S. Y. Park and D.-J. Jang, J. Am. Chem. Soc., 2005, 127, 10070 CrossRef CAS.
- K. Dhanunjayarao, V. Mukundam and K. Venkatasubbaiah, Sens. Actuators, B, 2016, 232, 175 CrossRef CAS.
- The purity of the monomer was further confirmed using HPLC. The HPLC data reveal that the monomer is extremely pure (Fig. S20).†.
Footnotes |
† Electronic supplementary information (ESI) available. CCDC 1975843. For ESI and crystallographic data in CIF or other electronic format see DOI: 10.1039/d0ra01559h |
‡ BPRA & RVRNC contributed equally. |
|
This journal is © The Royal Society of Chemistry 2020 |
Click here to see how this site uses Cookies. View our privacy policy here.