DOI:
10.1039/D0RA01822H
(Paper)
RSC Adv., 2020,
10, 15836-15845
Synthesis and in vitro anti-proliferative evaluation of naphthalimide–chalcone/pyrazoline conjugates as potential SERMs with computational validation†
Received
26th February 2020
, Accepted 14th April 2020
First published on 21st April 2020
Abstract
A series of naphthalimide-chalcone/pyrazoline conjugates was prepared and evaluated for their anti-breast cancer potential against estrogen responsive, i.e. MCF-7 (ER+), and triple-negative, i.e. MDA-MB-231 (ER−), cell lines. The structure-activity-relationship (SAR) was deduced based on the influence of linker length, substituents on the phenyl ring and the generated functionalities, on anti-proliferative activities. Docking simulations further delineate the type of interactions of the designed molecules with the selected targets. This report discloses the scope of triazole tethered naphthalimide-chalcone/pyrazoline conjugates as anti breast cancer agents.
Introduction
Cancer, an uncontrolled growth of cells, is the second leading cause of death worldwide. The most commonly diagnosed cancers are lung (12.6%), breast (11.9%), colorectal (9.8%), and prostate (7.7%).1 Breast cancer is the most frequent cancer among women, affecting 2.1 million women each year. A World Health Organization (WHO) survey estimated that 627
000 women died from breast cancer worldwide in 2018.2 Treatment for breast cancer may include surgery, radiation, chemotherapy, targeted therapy and hormone therapy. The proliferation of mammary cells is stimulated by the binding of hormone estrogen to estrogen receptors (ERs, existing in isomeric forms of ERα and ERβ), the later has developed as target for breast cancer therapy.3 Consequently, selective inhibition of ERα has led to the emergence of drugs known as Selective Estrogen Receptor Modulators (SERMs) and is an important strategy in the treatment of breast cancer.4
Chalcones are a prolific class of flavonoids with significant occurrence in many edible fruits with medicinal properties.5 Chalcones, with an aromatic enone as a central core, showed anti-inflammatory,6 anti-histaminic,7 anti-oxidant,8 anti-protozoal,9 antibacterial,10 antimalarial,11 antifungal,12 anti-HIV13 activities. Moreover, chalcones have shown potent inhibition of several anticancer targets, including thioredoxin reductase, and tubulin polymerization14,15 and by preferentially killing cancer cells over non-cancer cells.16 In this context, to improve the efficacy of chalcones while evading drug-resistance, molecular hybridization involving dual- or multi-target approach has been investigated. For instance, Lee et al.,17 synthesized quinoline–chalcones targeting MDR tumor and metastatic breast cancer cells, whereas Modzelewska et al.,18 reported a series of bis-chalcones with exceptional inhibitory potency in the growth of human breast and colon cancer cells.
Naphthalimides, owing to its flat aromatic structure and DNA-intercalating tendencies, have emerged as target motif in the area of cancer therapy.19 Some mono or bis-naphthalimide derivatives have displayed promising activities against tumor cells, among which, mitonafide, amonafide, elinafide and azonafide proceeded to clinical trials.20,21 However, poor therapeutic index, poor water solubility, and neurological toxicity impeded their further advancement in clinical phase.22 Thus, the ongoing efforts are geared towards modifying the molecular framework of naphthalimide based scaffolds to improve their therapeutic properties. A literary survey has disclosed better results of naphthalimide based scaffolds as anti tumor including anti-breast cancers.23–26
1H-1,2,3-triazole core, on the other hand, presents desirable properties such as hydrogen bonding ability, resistance to oxidation, reduction, and enzymatic hydrolysis in physiological conditions. Besides, they have shown a broad spectrum of medicinal potential including anticancer.27–31 Therefore, on this backdrop and in continuation of our research venture,32–35 the present manuscript describes the hybridization of the chosen pharmacophores i.e. substituted chalcones/pyrazolines and 1,8-naphthalimides using triazole as a linker. Two human breast cancer cell lines i.e. MCF-7 (ER+) and MDA-MB-231 (ER−) were used to investigate the in vitro anti-proliferative potential of the synthesized compounds.
Results and discussion
Chemistry
The first set of precursors were obtained by treating 1,8-naphthalic anhydride 1 with ethanol/propanol amine under reflux to yield 2. Further, compound 2 was treated with mesyl chloride and Et3N in dry CHCl3 to get the mesylated product 3, followed by its treatment with NaN3 in DMF to obtain the precursor 4, (Scheme 1).
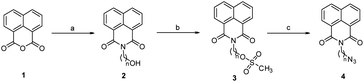 |
| Scheme 1 Reagents and conditions: (a) ethanol amine (n = 2)/propanol amine (n = 3), ethanol, reflux, 1 h (b) mesyl chloride, Et3N, dry CHCl3, RT, 3 h (c) NaN3, DMF, 60 °C, 2 h. | |
For second set of precursors, different aldehydes viz. 6 and 7 were treated with ketone 5, formed by O-propargylation of 4-hydroxy acetophenone, under the basic condition to form the Claisen–Schmidt condensation products 8 and 9. The introduction of pyrazoline rings is done by treating 8/9 with hydrazine hydrate in acetic acid at 130 °C to afford 10 and 11 respectively, (Scheme 2). The rationale for introducing pyrazoline functionality in the designed conjugates is based on its favourable role in enhancing anti-proliferative potential.36,37
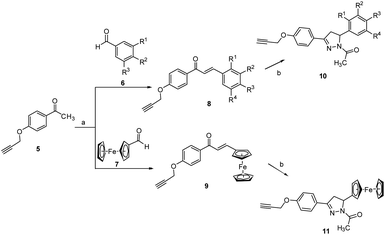 |
| Scheme 2 Reagents and conditions: (a) NaOH (40% w/v), ethanol, RT, 1 h (b) hydrazine hydrate, acetic acid, 130 °C, reflux, 2 h. | |
Subsequently, the target conjugates viz. 12a–h and 13a–h were synthesized by azide–alkyne cycloaddition reaction between 4 and 8/10 using CuSO4 and sodium ascorbate in absolute ethanol, (Scheme 3A). The ferrocene based counterparts viz. 14a–b and 15a–b were synthesized analogously from the cycloaddition reaction between 4 and 9/11, (Scheme 3B). The purification of the reaction mixture via column chromatography using ethyl acetate
:
hexane (10
:
90) mixture as eluent, afforded the desired conjugates in good to excellent yields. The structures of the synthesized molecules were assigned based on the spectral data and analytical evidences. For example, compound 12b, showed molecular ion peak at m/z 559.1975 [M + H]+ in its HRMS (ESI) spectrum. Its 1H NMR spectrum exhibited characteristic singlets at δ 3.83 and 7.76 corresponding to methoxy and triazole ring proton respectively. The signals at δ 188.83, 161.91 and 161.63 corresponding to the carbonyl groups along with requisite signals in 13C NMR spectrum further confirmed the assigned structure.
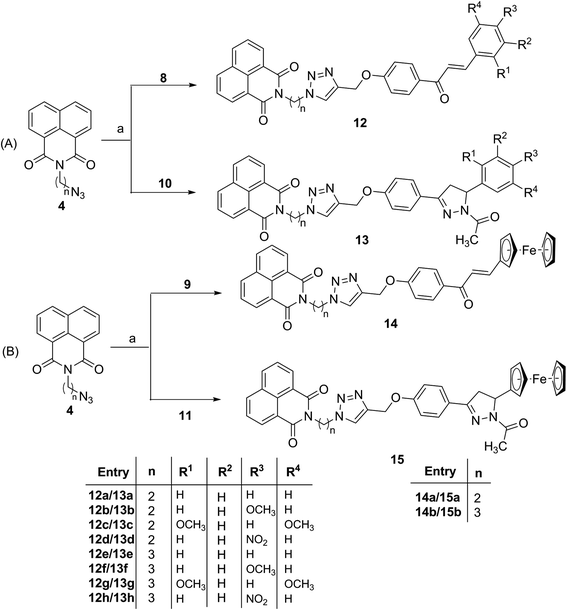 |
| Scheme 3 Reagents and condition: (a) CuSO4, sodium ascorbate, EtOH : H2O (90 : 10), RT, 10 h. | |
In vitro anti-proliferative evaluation and SAR
The synthesized series of triazole tagged naphthalimide-chalcone/pyrazoline conjugates was evaluated for their anti-proliferative activities against estrogen receptor positive and triple-negative human breast cancer cell lines, MCF-7 (ER+) and MDA-MB-231 (ER−) respectively, using MTT assay.38 The percentage growth inhibition of MCF-7 and MDA-MB-231 cells were recorded at varying concentrations of the synthesized conjugates using Plumbagin as the positive control, and the results are depicted in Fig. 1. The IC50 values of the synthesized compounds, which is the concentration required to inhibit the growth of 50% of cells, are included in Table 1.
 |
| Fig. 1 Representative graphs comparing the percentage growth inhibition of MCF-7 and MDA-MB-231 cells at selected concentrations of test compounds. 40 μM Plumbagin was used as a positive control. Data are mean ± standard deviation S.D (n = 3), where *p < 0.05, **p < 0.01 and ***p < 0.001 significant difference to untreated control. | |
Table 1 The IC50 values (in μM) of tested compounds against MCF-7 and MDA-MB-231 cells
Entries |
MCF-7 |
MDA-MB-231 |
12a |
>100 |
>100 |
12b |
>100 |
>100 |
12c |
>100 |
>100 |
12d |
>100 |
>100 |
12e |
>100 |
>100 |
12f |
>100 |
>100 |
12g |
>100 |
>100 |
12h |
>100 |
>100 |
13a |
>100 |
>100 |
13b |
>100 |
>100 |
13c |
>100 |
>100 |
13d |
>100 |
>100 |
13e |
>100 |
>100 |
13f |
98.11 |
>100 |
13g |
62.23 |
>100 |
13h |
>100 |
>100 |
14a |
>100 |
>100 |
14b |
>100 |
>100 |
15a |
>100 |
>100 |
15b |
>100 |
>100 |
4a |
>100 |
>100 |
4b |
>100 |
>100 |
Tamoxifen |
50 |
75 |
Plumbagin |
3.5 |
4.4 |
As evident from Table 1, naphthalimide–chalcone conjugates, 12a–h failed to inhibit the growth of both the cell lines even at the highest tested concentration i.e. 100 μM. Replacement of chalcone core with pyrazoline ring did not result in the improvement of their anti-proliferative activities against MDA-MB-231 cells as evident from conjugates 13a–h. Nevertheless, conjugates 13f and 13g showed moderate cytotoxicity against MCF-7 cell lines. The conjugate 13f bearing a spacer length of n = 3, pyrazoline ring and mono-methoxy substituted phenyl ring, showed IC50 value 98.11 μM whereas 13g with n = 3 and di-methoxy substituted phenyl ring, exhibited an IC50 value 62.23 μM. Further, replacing the aryl core with ferrocene in naphthalimide–chalcones, 14a–b as well as naphthalimide–pyrazolines, 15a–b could not improve activity profiles. Thus, the SAR analysis demonstrated that propyl spacer, pyrazoline ring and electron donating substituents were requisite in order to impart activity in the designed scaffolds. In contrast, ethyl spacer, chalcone core and unsubstituted/nitro substituted/ferrocene tagged conjugates failed to elicit any activity. However, the observed anti-proliferative activities of the active compounds against MCF-7 cells is less as compared to the reference cytotoxic agent, Plumbagin (IC50= 3.5 μM against MCF-7 cells), but comparable to that of standard drug, Tamoxifen (IC50= 50 μM against MCF-7 cells). The graphical representation of generalized SAR for the synthesized compounds is elucidated in Fig. 2.
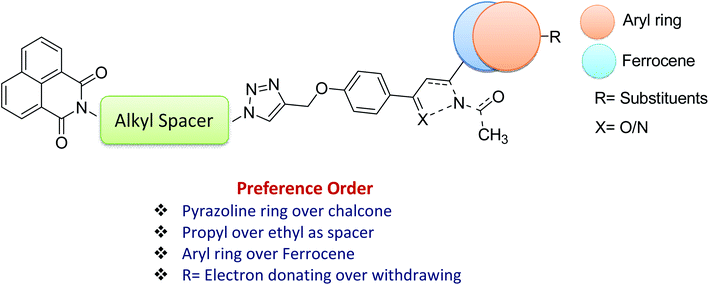 |
| Fig. 2 Generalized SAR of the synthesized compounds. | |
The toxicity of moderately active compounds 13f and 13g was also tested against normal/non-tumor cell line i.e. Human Embryonic Kidney (HEK-293). The compounds were non-cytotoxic with IC50 > 100 μM (Fig. 3 and Table 2).
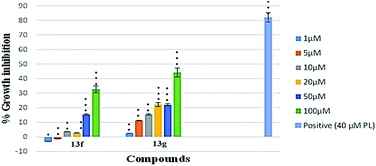 |
| Fig. 3 Representative graph comparing the percentage growth inhibition of HEK-293 cells at selected concentrations of test compounds 13f and 13g. 40 μM Plumbagin was used as a positive control. Data are mean ± standard deviation S.D (n = 3), where *p < 0.05, **p < 0.01 and ***p < 0.001 significant difference to untreated control. | |
Table 2 The IC50 values (in μM) of tested compounds against HEK-293 cells
Entries |
HEK-293 |
13f |
>100 |
13g |
>100 |
Molecular docking and ADMET properties prediction
In order to identify the type of interactions operating between the synthesized compounds (ligands) and the estrogen receptors (protein), the active compounds viz. 13f and 13g were chosen for docking studies with ERα and ERβ. Further an inactive compound 13h was also included for comparison purpose.
The molecular docking analysis of compound 13f (IC50 = 98.11 μM; B.E. = −8.3 kcal mol−1), presented hydrophobic interactions with Leu306, Ala307, Leu310, Ala318, Leu372, and Val368 of ERα receptor. The results also revealed that hydrophobic forces dominate the interactions between 13f and the ERα receptor. Moreover, 1H-1,2,3-triazole ring forms π-alkyl interaction with Val368 while the protonated nitrogen in the triazole ring forms H-bonding interaction with Asp369. The naphthalene ring also forms hydrophobic interaction with residues, Val368 and Leu373 (Fig. 4a). The introduction of a 2,5-dimethoxy substituent in compound 13g (B.E = −8.4 kcal mol−1) enhanced the anti-proliferative potency (IC50 = 62.23 μM) compared to 13f (IC50 = 98.11 μM). Interestingly, this was reflected in the hydrogen bonding and hydrophobic interactions of compound 13g with Leu372, Ala546, and Arg548 in the ligand-binding pocket of ERα. The meta-methoxy unit also featured a conventional H-bonding with the amino unit of Leu372 at a bond distance (b.d) of 2.14 Å and bond angle (b.a) of 138.73°. The ortho-methoxy unit on the other hand interacted with Arg548 and Ala546 via conventional and carbon–hydrogen bonding (b.d = 2.35 Å and 3.35 Å; b.a = 154.37°, respectively). The dimethoxy substituted phenyl ring showed π-alkyl interaction with Leu372. These binding modes helped to lock-in the ligand within the binding site of the ERα receptor for improved binding affinity. The H-bonding interaction of triazole linker with Asp369 is accompanied by π-cation and π-anion interactions with Asp369 and Leu306 while the naphthalene ring is involved in π-alkyl and π-sigma hydrophobic interactions with Ala 318 and Pro365 (Fig. 4b). These results demonstrate that substituents on the phenyl ring can have drastic effects on the potency of these compounds as ERα antagonists.
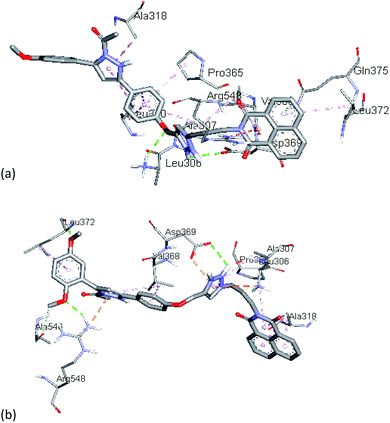 |
| Fig. 4 The docked binding modes of (a) 13f and (b) 13g in the binding site of ERα (PDB:3ERT). | |
The evaluation of potent compounds in the active region of ERβ showed a binding mode for 13f (B.E = −7.8 kcal mol−1) in which the triazole and pyrazoline NH units donates H-bond to the carbonyl oxygen atoms of Val370 and Asp378 respectively (b.d = 2.54 Å and 2.76 Å; b.a = 90.5° and 110° respectively). The naphthalene ring makes electrostatic contacts with Glu291 and H-bonding interaction with Glu372. Whereas the para-methoxy substituent forms hydrophobic interactions with Met379 and Ala382, respectively (Fig. 5a). Further, analysis of the docked complex of 13g (B.E = −8.3 kcal mol−1) showed that the naphthalene unit interacts with Glu291 via electrostatic contact. The dimethoxy substituted phenyl ring formed π-alkyl interaction with Ala382 while the meta-methoxy unit forms a C–H bond with Met379. Strong conventional H-bonding was also observed between the NH group of triazole ring and Val370, (b.d = 2.11, b.a = 135°) (Fig. 5b).
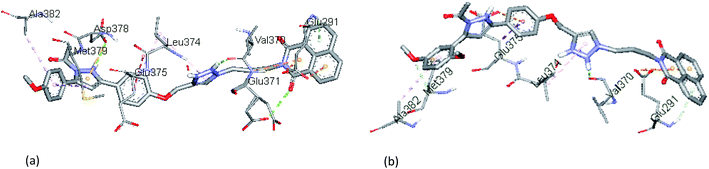 |
| Fig. 5 The docked binding modes of (a) 13f and (b) 13g in the binding site of ERβ (PDB:3LOS). | |
The docked binding modes of 13h in the binding site of ERα and ERβ have been shown in Fig. 6. The compound featured three hydrogen bonds with Gly372, Glu371 and Phe289 (3.00 Å, 2.78 Å and 3.04 Å, respectively), and hydrophobic interactions with Leu374, Cys369, and Glu366 respectively, in the ligand-binding pocket of ERα. Compared to 13f and 13g, compound 13h interacts with amino acid residues in a different active site of ERβ; it showed one hydrogen bond interaction with His377. The binding profile is also accompanied by hydrophobic interactions with Tyr537, Tyr526, Val533, and Lys529, as well as electrostatic interactions with Glu380 and Cys530, respectively. The binding profile in the docked complex of compound 13h with ERα and ERβ partly justifies the low inhibitory activity observed (>100 μM).
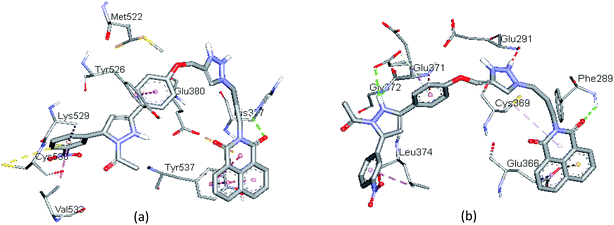 |
| Fig. 6 The docked binding modes of 13h in the binding site of (a) ERα and (b) ERβ. | |
Furthermore, the predicted physicochemical properties and ADMET descriptors (Table S1, see ESI†) suggest good drug-like properties for compounds 13f and 13g. For instance, the compounds present good gastrointestinal absorption which correlates with the predicted water solubility. A closer look at the predicted metabolism interactions towards CYP (Cytochrome P450) isoforms revealed that the compounds are suitable inhibitors of cytochrome P450 and also CYP2D6/CYP3A4 substrate, although 13g seems to be resistant to CYP1A2 inhibitor.
Conclusion
The current work disclosed the synthesis of naphthalimide-chalcone/pyrazoline conjugates tethered by triazole ring and their anti-proliferative effects against two breast cancer cell lines. The anti-proliferative evaluation identified two compounds, 13f and 13g to be explicitly targeting estrogen-responsive breast cancer cell line MCF-7 (ER+) while the whole series was inactive against estrogen non-responsive/triple-negative cell line MDA-MB-231 (ER−). The active compound displayed IC50 value of 62.23 μM which is comparable to the standard anti-breast cancer drug Tamoxifen. In order to confirm their safety profiles, the active compounds were evaluated against HEK-293 cell line and proved to be non-cytotoxic. The docking simulations as well as physiochemical properties were studied to get an insight into the type of interactions and drug likeness of the synthesized compounds. The only limitation is its moderate activity which needs further modification to get enhanced. Thus, the designed molecular framework can be set as therapeutic template to particularly target estrogen responsive breast cancer and thus can contribute in the venture of developing SERMs.
Experimental
General information
Stuart Digital Melting Point apparatus (SMP10) and an open capillary was used to determine melting points and are uncorrected. BRUKER AVANCE II (500 and 125 MHz) and JEOL (400 and 100 MHz) NMR spectrometers were used in order to record 1H and 13C NMR spectra of the synthesized compounds dissolved in CDCl3 (Sigma-Aldrich). Tetramethylsilane (TMS) was kept as reference and chemical shift values are expressed as parts per million (ppm) downfield from TMS. While the coupling constants represented by J values are expressed in hertz (Hz). Splitting patterns are indicated as s: singlet, d: doublet, t: triplet, m: multiplet, dd: double doublet, dt: doublet of a triplet and br: broad peak. Mass spectra were recorded on a Bruker micrOTOF-QII high resolution mass spectrometer.
General procedure for the synthesis of 4
To a well stirred solution of 1,8-naphthalic anhydride, 1 (1 mmol) in absolute ethanol, ethanol/propanol amine (1.5 mmol) was added and the reaction mixture was refluxed for 1 h. On cooling, the reaction mixture was filtered to get solid 2. The intermediate 2 (1 mmol) was treated with mesyl chloride (1.5 mmol) and Et3N (2 mmol) in dry CHCl3 for 3 h at room temperature. The resulting product 3 was further reacted with NaN3 in dry DMF at 60 °C for 2 h to afford 4.
General procedure for the synthesis of 8/9 and 10/11
K2CO3 (2 mmol) was added to a stirred solution of 4-hydroxy acetophenone (1 mmol) in acetone. After the generation of anion, propargyl bromide (1.5 mmol) was added and the reaction mixture was allowed to stir for 5 h to yield 5. The compound 5 (1 mmol) was then dissolved in alkaline ethanol and reacted with different aldehydes 6/7 (1.2 mmol) at room temperature for 1 h to obtain 8/9. Further, hydrazine hydrate (5 mmol) and catalytic amount of acetic acid (0.5 mmol) was added to solution of 8/9 (1 mmol) in ethanol and the reaction mixture was refluxed for 1 h to afford 10/11.
General procedure for the synthesis of 12/13 and 14/15
To the solution of 4 (1 mmol) and 8/10 (1.2 mmol) in ethanol
:
water (90
:
10) catalytic amount of CuSO4 and sodium ascorbate was added. The reaction was monitored on TLC and on its completion, the crude product was extracted using CHCl3
:
H2O. The crude was then purified on column chromatography using ethylacetate
:
hexane (10
:
90) as eluent to afford final set of compounds 12/13. The similar procedure was followed using 4 and 9/11 in order to produce the final hybrids 14/15 in good yields.
Physical and spectral data
2-(2-(4-((4-Cinnamoylphenoxy)methyl)-1H-1,2,3-triazol-1-yl)ethyl)-1H-benzo[de]isoquinoline-1,3(2H)-dione1 (12a). Yield-86%, bright yellow solid, MP = 148–150 °C, 1H NMR (400 MHz, CDCl3): δ 4.68–4.70 (m, 2H, CH2), 4.78–4.80 (m, 2H, CH2), 5.28 (s, 2H, OCH2), 7.10 (d, J = 8.9 Hz, 2H, ArH), 7.30–7.34 (m, 3H, ArH), 7.39 (d, J = 15.5 Hz, 1H, olefinic H), 7.55 (d, J = 8.6 Hz, 2H, ArH), 7.65–7.70 (m, 3H, ArH), 7.75 (s, 1H, triazole-H), 7.95 (d, J = 8.8 Hz, 2H, ArH), 8.15 (dd, J = 0.9, 8.3 Hz, 2H, ArH), 8.40 (dd, J = 0.8, 7.2 Hz, 2H, ArH). 13C NMR (101 MHz, CDCl3) δ 188.69, 163.94, 162.01, 144.02, 134.40, 131.63, 131.56, 131.34, 130.76, 130.33, 129.33, 128.91, 128.35, 128.21, 126.98, 123.40, 121.98, 121.93, 114.71, 114.60, 62.12, 48.02, 39.62. HRMS (ESI) calcd for C32H24N4O4 [M + H]+ 529.1876, found 529.1895.
(E)-2-(2-(4-((4-(3-(4-Methoxyphenyl)acryloyl)phenoxy)methyl)-1H-1,2,3-triazol-1-yl)ethyl)-1H-benzo[de]isoquinoline-1,3(2H)-dione (12b). Yield-85%, bright yellow solid, MP = 154–156 °C, 1H NMR (400 MHz, CDCl3): δ 3.83 (s, 3H, OCH3), 4.64–4.67 (m, 2H, CH2), 4.75–4.78 (m, 2H, CH2), 5.24 (s, 2H, OCH2), 6.91 (d, J = 8.8 Hz, 2H, ArH), 7.00 (d, J = 9.0 Hz, 2H, Ar H), 7.38 (d, J = 15.6 Hz, 1H, olefinic H), 7.57 (d, J = 8.6 Hz, 2H, ArH), 7.67–7.73 (m, 3H, ArH), 7.76 (s, 1H, triazole-H), 7.96 (d, J = 8.9 Hz, 2H, ArH), 8.17 (dd, J = 1.0, 8.4 Hz, 2H, ArH), 8.46 (dd, J = 1.1, 7.2 Hz, 2H, ArH). 13C NMR (101 MHz, CDCl3) δ 188.83, 164.04, 161.91, 161.63, 143.99, 143.79, 134.53, 131.76, 131.68, 131.67, 130.75, 130.21, 128.26, 127.84, 127.08, 123.54, 122.00, 119.57, 114.69, 114.48, 62.13, 55.49, 48.10, 39.70. HRMS (ESI) calcd for C33H26N4O5 [M + H]+ 559.1981, found 559.1975.
(E)-2-(2-(4-((4-(3-(2,5-Dimethoxyphenyl)acryloyl)phenoxy)methyl)-1H-1,2,3-triazol-1-yl)ethyl)-1H-benzo[de]isoquinoline-1,3(2H)-dione (12c). Yield-85%, light brown solid, MP = 146–148 °C, 1H NMR (400 MHz, CDCl3): δ 3.80 (s, 3H, OCH3), 3.86 (s, 3H, OCH3), 4.60 (t, J = 6.8 Hz, 2H, CH2), 4.71 (t, J = 7.0 Hz, 2H, CH2), 5.25 (s, 2H, OCH2), 6.85 (d, J = 8.9 Hz, 1H, ArH), 6.92 (dd, J = 3.1, 9.1 Hz, 1H, ArH), 7.08 (d, J = 8.6 Hz, 2H, ArH), 7.15 (d, J = 3.0 Hz, 1H, Ar H), 7.58 (d, J = 15.6 Hz, 1H, olefinic H), 7.75–7.78 (m, 2H, ArH), 7.89 (s, 1H, triazole-H), 8.01 (d, J = 8.6 Hz, 2H, ArH), 8.05 (d, J = 15.5 Hz, 1H, olefinic H), 8.21 (d, J = 8.1 Hz, 2H, ArH), 8.58 (d, J = 7.4 Hz, 2H, ArH). 13C NMR (101 MHz, CDCl3) δ 189.15, 164.40, 160.95, 153.45, 153.10, 143.65, 140.40, 135.21, 132.10, 131.95, 131.51, 130.90, 128.15, 127.45, 125.10, 123.40, 122.95, 122.35, 117.15, 114.51, 113.85, 112.50, 62.15, 56.20, 55.85, 48.21, 38.40. HRMS (ESI) calcd for C34H28N4O6 [M + H]+ 589.2087, found 589.2072.
(E)-2-(2-(4-((4-(3-(4-Nitrophenyl)acryloyl)phenoxy)methyl)-1H-1,2,3-triazol-1-yl)ethyl)-1H-benzo[de]isoquinoline-1,3(2H)-dione (12d). Yield-85%, yellow solid, MP = 161–163 °C, 1H NMR (400 MHz, CDCl3): δ 4.65–4.68 (m, 2H, CH2), 4.74–4.76 (m, 2H, CH2), 5.25 (s, 2H, OCH2), 6.95 (d, J = 8.8 Hz, 2H, ArH), 7.36 (d, J = 8.7 Hz, 2H, Ar H), 7.40 (d, J = 15.5 Hz, 1H, olefinic H), 7.63 (d, J = 8.8 Hz, 2H, ArH), 7.70–7.74 (m, 3H, ArH), 7.76 (s, 1H, triazole-H), 8.10 (d, J = 8.7 Hz, 2H, ArH), 8.18 (dd, J = 0.8, 8.1 Hz, 2H, ArH), 8.50 (dd, J = 0.7, 7.1 Hz, 2H, ArH). 13C NMR (101 MHz, CDCl3) δ 189.10, 164.10, 161.65, 143.92, 143.70, 141.30, 134.50, 131.91, 131.70, 131.60, 130.66, 130.25, 128.36, 127.95, 125.25, 123.85, 123.28, 122.15, 119.20, 114.40, 62.51, 48.15, 39.61. HRMS (ESI) calcd for C32H23N5O6 [M + H]+ 574.1726, found 574.1737.
2-(3-(4-((4-Cinnamoylphenoxy)methyl)-1H-1,2,3-triazol-1-yl)propyl)-1H-benzo[de]isoquinoline-1,3(2H)-dione (12e). Yield-87%, off-white solid, MP = 135–137 °C, 1H NMR (400 MHz, CDCl3): δ 2.35–2.40 (m, 2H, CH2), 4.60–4.64 (m, 2H, CH2), 4.70–4.74 (m, 2H, CH2), 5.24 (s, 2H, OCH2), 7.12 (d, J = 8.8 Hz, 2H, ArH), 7.31–7.35 (m, 3H, ArH), 7.38 (d, J = 15.6 Hz, 1H, olefinic H), 7.56 (d, J = 8.5 Hz, 2H, ArH), 7.66–7.72 (m, 3H, ArH), 7.76 (s, 1H, triazole-H), 7.96 (d, J = 8.7 Hz, 2H, ArH), 8.16 (dd, J = 1.0, 8.4 Hz, 2H, ArH), 8.42 (dd, J = 1.1, 7.3 Hz, 2H, ArH). 13C NMR (101 MHz, CDCl3) δ 188.65, 163.95, 162.10, 144.10, 134.42, 131.65, 131.57, 131.35, 130.72, 130.30, 129.35, 128.90, 128.40, 128.21, 126.95, 123.41, 121.95, 121.90, 114.71, 114.56, 62.20, 48.15, 38.90, 28.85. HRMS (ESI) calcd for C33H26N4O4 [M + H]+ 543.2032, found 543.2045.
(E)-2-(3-(4-((4-(3-(4-Methoxyphenyl)acryloyl)phenoxy)methyl)-1H-1,2,3-triazol-1-yl)propyl)-1H-benzo[de]isoquinoline-1,3(2H)-dione (12f). Yield-86%, off white solid, MP = 151–153 °C, 1H NMR (400 MHz, CDCl3): δ 2.37–2.43 (m, 2H, CH2), 3.80 (s, 3H, OCH3), 4.61–4.65 (m, 2H, CH2), 4.72–4.75 (m, 2H, CH2), 5.21 (s, 2H, OCH2), 6.89 (d, J = 8.7 Hz, 2H, ArH), 6.95 (d, J = 8.9 Hz, 2H, ArH), 7.35 (d, J = 15.5 Hz, 1H, olefinic H), 7.51 (d, J = 8.5 Hz, 2H, ArH), 7.65–7.72 (m, 3H, ArH), 7.75 (s, 1H, triazole-H), 7.95 (d, J = 8.8 Hz, 2H, ArH), 8.15 (dd, J = 1.0, 8.5 Hz, 2H, ArH), 8.42 (dd, J = 1.1, 7.3 Hz, 2H, ArH). 13C NMR (101 MHz, CDCl3) δ 188.95, 164.10, 161.92, 161.61, 143.98, 143.75, 134.50, 131.76, 131.69, 131.60, 130.85, 130.40, 128.30, 127.91, 127.12, 123.51, 122.10, 119.51, 114.62, 114.45, 62.16, 56.01, 48.10, 39.45, 28.89. HRMS (ESI) calcd for C34H28N4O5 [M + H]+ 573.2138, found 573.2152.
(E)-2-(3-(4-((4-(3-(2,5-Dimethoxyphenyl)acryloyl)phenoxy)methyl)-1H-1,2,3-triazol-1-yl)propyl)-1H-benzo[de]isoquinoline-1,3(2H)-dione (12g). Yield-85%, bright yellow solid, MP = 142–144 °C, 1H NMR (400 MHz, CDCl3): δ 2.37–2.43 (m, 2H, CH2), 3.79 (s, 3H, OCH3), 3.84 (s, 3H, OCH3), 4.25 (t, J = 6.7 Hz, 2H, CH2), 4.48 (t, J = 7.0 Hz, 2H, CH2), 5.24 (s, 2H, OCH2), 6.84 (d, J = 9.0 Hz, 1H, ArH), 6.90 (dd, J = 3.0, 9.0 Hz, 1H, ArH), 7.05 (d, J = 8.7 Hz, 2H, ArH), 7.12 (d, J = 2.9 Hz, 1H, ArH), 7.56 (d, J = 15.8 Hz, 1H, olefinic H), 7.72–7.75 (m, 2H, ArH), 7.87 (s, 1H, triazole-H), 8.00 (d, J = 8.7 Hz, 2H, ArH), 8.02 (d, J = 15.8 Hz, 1H, olefinic H), 8.20 (d, J = 8.2 Hz, 2H, ArH), 8.57 (d, J = 7.3 Hz, 2H, ArH). 13C NMR (101 MHz,) δ 189.39, 164.43, 161.95, 153.52, 153.34, 143.44, 139.53, 134.43, 131.78, 131.67, 131.58, 130.93, 128.20, 127.13, 124.67, 123.33, 122.95, 122.35, 117.11, 114.67, 113.80, 112.49, 62.16, 56.19, 55.92, 48.49, 37.57, 28.99. HRMS (ESI) calcd for C35H30N4O6 [M + H]+ 603.2243, found 603.2255.
(E)-2-(3-(4-((4-(3-(4-nitrophenyl)acryloyl)phenoxy)methyl)-1H-1,2,3-triazol-1-yl)propyl)-1H-benzo[de]isoquinoline-1,3(2H)-dione (12h). Yield-85%, bright yellow solid, MP = 140–142 °C, 1H NMR (400 MHz, CDCl3): δ 2.36–2.42 (m, 2H, CH2), 4.26 (t, J = 6.6 Hz, 2H, CH2), 4.45 (t, J = 7.0 Hz, 2H, CH2), 5.25 (s, 2H, OCH2), 6.94 (d, J = 8.7 Hz, 2H, ArH), 7.35 (d, J = 8.8 Hz, 2H, ArH), 7.41 (d, J = 15.4 Hz, 1H, olefinic H), 7.64 (d, J = 8.7 Hz, 2H, ArH), 7.71–7.75 (m, 3H, ArH), 7.75 (s, 1H, triazole-H), 8.12 (d, J = 8.8 Hz, 2H, ArH), 8.16 (dd, J = 1.0, 8.0 Hz, 2H, ArH), 8.45 (dd, J = 1.1, 7.2 Hz, 2H, ArH). 13C NMR (101 MHz, CDCl3) δ 189.15, 164.12, 161.64, 143.90, 143.68, 141.28, 134.48, 131.92, 131.75, 131.61, 130.65, 130.21, 128.35, 127.90, 125.20, 123.81, 123.25, 122.10, 119.25, 114.41, 62.50, 48.20, 39.60, 28.90. HRMS (ESI) calcd for C33H25N5O6 [M + H]+ 588.1883, found 588.1896.
2-(2-(4-((4-(1-Acetyl-5-phenyl-4,5-dihydro-1H-pyrazol-3-yl)phenoxy)methyl)-1H-1,2,3-triazol-1-yl)ethyl)-1H-benzo[de]isoquinoline-1,3(2H)-dione (13a). Yield-84%, light brown solid, MP = 82–84 °C, 1H NMR (400 MHz, CDCl3): δ 2.38 (s, 3H, CH3), 2.94 (dd, J = 4.5, 17.4 Hz, 1H, CH2), 3.62 (dd, J = 10.9, 17.3 Hz, 1H, CH2), 4.65–4.68 (m, 2H, CH2), 4.75–4.78 (m, 2H, CH2), 5.21 (s, 2H, OCH2), 5.56 (dd, J = 4.5, 10.5 Hz, 1H, CH), 7.12 (d, J = 8.8 Hz, 2H, ArH), 7.31–7.35 (m, 3H, ArH), 7.54 (d, J = 8.5 Hz, 2H, ArH), 7.65–7.69 (m, 2H, ArH), 7.75 (s, 1H, triazole-H), 7.94 (d, J = 8.8 Hz, 2H, ArH), 8.16 (dd, J = 1.1, 8.4 Hz, 2H, ArH), 8.39 (dd, J = 1.0, 7.3 Hz, 2H, ArH). 13C NMR (101 MHz,) δ 168.75, 164.10, 159.95, 159.10, 153.65, 134.60, 134.25, 131.70, 131.65, 128.31, 128.20, 127.10, 126.95, 124.61, 123.45, 122.05, 115.10, 114.30, 62.10, 55.40, 48.10, 41.75, 39.60, 22.05. HRMS (ESI) calcd for C34H28N6O4 [M + H]+ 585.2250, found 585.2272.
2-(2-(4-((4-(1-Acetyl-5-(4-methoxyphenyl)-4,5-dihydro-1H-pyrazol-3-yl)phenoxy)methyl)-1H-1,2,3-triazol-1-yl)ethyl)-1H-benzo[de]isoquinoline-1,3(2H)-dione (13b). Yield-85%, bright yellow solid, MP = 85–87 °C, 1H NMR (400 MHz, CDCl3): δ 2.37 (s, 3H, CH3), 3.09 (dd, J = 4.4, 17.6 Hz, 1H, CH2), 3.66 (dd, J = 11.7, 17.6 Hz, 1H, CH2), 3.74 (s, 3H, OCH3), 4.64–4.67 (m, 2H, CH2), 4.74–4.77 (m, 2H, CH2), 5.20 (s, 2H, OCH2), 5.51 (dd, J = 4.4, 11.6 Hz, 1H, CH), 6.81 (d, J = 8.7 Hz, 2H, ArH), 6.97 (d, J = 8.9 Hz, 2H, ArH), 7.14 (d, J = 8.6 Hz, 2H, ArH), 7.64 (d, J = 8.9 Hz, 2H, ArH), 7.69–7.73 (m, 2H, ArH), 7.76 (s, 1H, triazole-H), 8.19 (dd, J = 1.0, 8.4 Hz, 2H, ArH), 8.50 (dd, J = 1.1, 7.3 Hz, 2H, ArH). 13C NMR (101 MHz,) δ 168.74, 164.06, 159.99, 159.06, 153.68, 134.52, 134.27, 131.72, 131.68, 128.31, 128.25, 127.08, 126.99, 124.64, 123.45, 122.08, 115.13, 114.30, 62.13, 59.39, 55.35, 48.05, 42.44, 39.72, 22.05. HRMS (ESI) calcd for C35H30N6O5 [M + H]+ 615.2356, found 615.2368.
2-(2-(4-((4-(1-Acetyl-5-(2,5-dimethoxyphenyl)-4,5-dihydro-1H-pyrazol-3-yl)phenoxy)methyl)-1H-1,2,3-triazol-1-yl)ethyl)-1H-benzo[de]isoquinoline-1,3(2H)-dione (13c). Yield-84%, yellow solid, MP = 78–80 °C, 1H NMR (400 MHz, CDCl3): δ 2.38 (s, 3H, CH3), 2.94 (dd, J = 4.4, 17.5 Hz, 1H, CH2), 3.60 (dd, J = 11.6, 17.5 Hz, 1H, CH2), 3.69 (s, 3H, OCH3), 3.80 (s, 3H, OCH3), 4.25 (t, J = 6.7 Hz, 2H, CH2), 4.47 (t, J = 7.0 Hz, 2H, CH2), 5.20 (s, 2H, OCH2), 5.76 (dd, J = 4.5, 11.5 Hz, 1H, CH), 6.54 (d, J = 3.0 Hz, 1H, ArH), 6.71 (dd, J = 2.9, 8.7 Hz, 1H, ArH), 6.76 (d, J = 8.7 Hz, 1H, ArH), 6.98 (d, J = 8.6 Hz, 2H, ArH), 7.64 (d, J = 8.7 Hz, 2H, ArH), 7.74–7.78 (m, 2H, ArH), 7.85 (s, 1H, triazole-H), 8.23 (d, J = 8.1 Hz, 2H, ArH), 8.60 (d, J = 7.3 Hz, 2H, ArH). 13C NMR (101 MHz,) δ 168.76, 164.40, 159.85, 154.50, 153.71, 150.25, 143.60, 134.40, 131.70, 131.56, 130.61, 128.25, 128.20, 127.15, 124.75, 123.20, 122.35, 114.95, 112.45, 112.15, 111.85, 62.10, 56.12, 55.75, 55.40, 48.45, 41.65, 38.01, 22.05. HRMS (ESI) calcd for C36H32N6O6 [M + H]+ 645.2461, found 645.2475.
2-(2-(4-((4-(1-Acetyl-5-(4-nitrophenyl)-4,5-dihydro-1H-pyrazol-3-yl)phenoxy)methyl)-1H-1,2,3-triazol-1-yl)ethyl)-1H-benzo[de]isoquinoline-1,3(2H)-dione (13d). Yield-86%, light brown solid, MP = 94–96 °C, 1H NMR (400 MHz, CDCl3): δ 2.40 (s, 3H, CH3), 3.09 (dd, J = 4.9, 17.0 Hz, 1H, CH2), 3.77 (dd, J = 12.0, 17.6 Hz, 1H, CH2), 4.64–4.67 (m, 2H, CH2), 4.74–4.77 (m, 2H, CH2), 5.21 (s, 2H, OCH2), 5.61 (dd, J = 4.8, 11.9 Hz, 1H, CH), 6.99 (d, J = 8.8 Hz, 2H, ArH), 7.38 (d, J = 8.7 Hz, 2H, ArH), 7.64 (d, J = 8.8 Hz, 2H, ArH), 7.71–7.75 (m, 2H, ArH), 7.77 (s, 1H, triazole-H), 8.16 (d, J = 8.7 Hz, 2H, ArH), 8.21 (dd, J = 0.7, 8.2 Hz, 2H, ArH), 8.51 (dd, J = 0.8, 7.2 Hz, 2H, ArH). 13C NMR (101 MHz,) δ 168.99, 164.10, 160.26, 153.52, 149.03, 147.40, 143.81, 134.62, 131.74, 131.72, 128.37, 128.31, 127.12, 126.77, 124.40, 123.93, 123.51, 122.04, 115.22, 62.09, 59.40, 48.05, 42.21, 39.74, 21.96. HRMS (ESI) calcd for C34H27N7O6 [M + H]+ 630.2101, found 630.2115.
2-(3-(4-((4-(1-Acetyl-5-phenyl-4,5-dihydro-1H-pyrazol-3-yl)phenoxy)methyl)-1H-1,2,3-triazol-1-yl)propyl)-1H-benzo[de]isoquinoline-1,3(2H)-dione (13e). Yield-87%, light brown solid, MP = 78–80 °C, 1H NMR (400 MHz, CDCl3): δ 2.35–2.40 (m, 5H, CH3 + CH2), 2.95 (dd, J = 4.8, 17.9 Hz, 1H, CH2), 3.61 (dd, J = 11.1, 17.8 Hz, 1H, CH2), 4.65–4.68 (m, 2H, CH2), 4.74–4.77 (m, 2H, CH2), 5.25 (s, 2H, OCH2), 5.56 (dd, J = 4.7, 11.5 Hz, 1H, CH), 7.12 (d, J = 8.7 Hz, 2H, ArH), 7.32–7.36 (m, 3H, ArH), 7.55 (d, J = 8.5 Hz, 2H, ArH), 7.65–7.68 (m, 2H, ArH), 7.76 (s, 1H, triazole-H), 7.95 (d, J = 8.7 Hz, 2H, ArH), 8.15 (dd, J = 0.9, 8.5 Hz, 2H, ArH), 8.40 (dd, J = 0.8, 7.5 Hz, 2H, ArH). 13C NMR (101 MHz,) δ 168.72, 164.20, 159.90, 159.20, 153.60, 134.62, 134.26, 131.75, 131.66, 128.30, 128.21, 127.12, 126.91, 124.60, 123.41, 122.06, 115.12, 114.35, 62.12, 55.41, 48.15, 41.70, 39.42, 29.15, 22.09. HRMS (ESI) calcd for C35H30N6O4 [M + H]+ 599.2407, found 599.2419.
2-(3-(4-((4-(1-Acetyl-5-(4-methoxyphenyl)-4,5-dihydro-1H-pyrazol-3-yl)phenoxy)methyl)-1H-1,2,3-triazol-1-yl)propyl)-1H-benzo[de]isoquinoline-1,3(2H)-dione (13f). Yield-85%, light brown solid, MP = 82–84 °C, 1H NMR (400 MHz, CDCl3): δ 2.36–2.40 (m, 5H, CH3 + CH2), 3.10 (dd, J = 4.4, 17.5 Hz, 1H, CH2), 3.65 (dd, J = 11.6, 17.5 Hz, 1H, CH2), 3.75 (s, 3H, OCH3), 4.63–4.66 (m, 2H, CH2), 4.75–4.78 (m, 2H, CH2), 5.21 (s, 2H, OCH2), 5.50 (dd, J = 4.5, 11.3 Hz, 1H, CH), 6.82 (d, J = 8.6 Hz, 2H, ArH), 6.96 (d, J = 8.8 Hz, 2H, ArH), 7.15 (d, J = 8.5 Hz, 2H, ArH), 7.65 (d, J = 8.9 Hz, 2H, ArH), 7.70–7.75 (m, 2H, ArH), 7.77 (s, 1H, triazole-H), 8.20 (dd, J = 1.1, 8.5 Hz, 2H, ArH), 8.48 (dd, J = 1.2, 7.4 Hz, 2H, ArH). 13C NMR (101 MHz,) δ 168.70, 164.10, 159.90, 159.10, 153.65, 134.53, 134.25, 131.75, 131.65, 128.35, 128.20, 127.10, 126.99, 124.65, 123.45, 122.10, 115.20, 114.30, 62.15, 59.40, 55.30, 48.12, 42.40, 39.70, 29.10, 22.04. HRMS (ESI) calcd for C36H32N6O5 [M + H]+ 629.2512, found 629.2525.
2-(3-(4-((4-(1-Acetyl-5-(2,5-dimethoxyphenyl)-4,5-dihydro-1H-pyrazol-3-yl)phenoxy)methyl)-1H-1,2,3-triazol-1-yl)propyl)-1H-benzo[de]isoquinoline-1,3(2H)-dione (13g). Yield-88%, light brown solid, MP = 76–78 °C, 1H NMR (400 MHz, CDCl3): δ 2.38–2.42 (m, 5H, CH2 + CH3), 2.95 (dd, J = 4.5, 17.6 Hz, 1H, CH2), 3.61–3.66 (m, 1H, CH2), 3.68 (s, 3H, OCH3), 3.79 (s, 3H, OCH3), 4.26 (t, J = 6.6 Hz, 2H, CH2), 4.48 (t, J = 7.0 Hz, 2H, CH2), 5.19 (s, 2H, OCH2), 5.77 (dd, J = 4.4, 11.6 Hz, 1H, CH), 6.55 (d, J = 2.9 Hz, 1H, ArH), 6.70 (dd, J = 2.9, 8.8 Hz, 1H, ArH), 6.78 (d, J = 8.8 Hz, 1H, ArH), 6.97 (d, J = 8.7 Hz, 2H, ArH), 7.63 (d, J = 8.7 Hz, 2H, ArH), 7.73–7.77 (m, 2H, ArH), 7.84 (s, 1H, triazole-H), 8.22 (d, J = 8.1 Hz, 2H, ArH), 8.58 (d, J = 7.3 Hz, 2H, ArH).13C NMR (101 MHz,) δ 168.75, 164.44, 159.88, 154.55, 153.72, 150.23, 143.62, 134.45, 131.68, 131.59, 130.62, 128.27, 128.23, 127.14, 124.79, 123.21, 122.36, 114.98, 112.41, 112.14, 111.87, 62.09, 56.11, 55.71, 55.42, 48.48, 41.70, 37.58, 29.02, 22.04. HRMS (ESI) calcd for C37H34N6O6 [M + H]+ 659.2618, found 659.2627.
2-(3-(4-((4-(1-Acetyl-5-(4-nitrophenyl)-4,5-dihydro-1H-pyrazol-3-yl)phenoxy)methyl)-1H-1,2,3-triazol-1-yl)propyl)-1H-benzo[de]isoquinoline-1,3(2H)-dione (13h). Yield-85%, bright yellow solid, MP = 86–88 °C, 1H NMR (400 MHz, CDCl3): δ 2.35–2.40 (m, 5H, CH2 + CH3), 3.10 (dd, J = 5.2, 16.9 Hz, 1H, CH2), 3.76 (dd, J = 11.9, 16.8 Hz, 1H, CH2), 4.63–4.66 (m, 2H, CH2), 4.75–4.78 (m, 2H, CH2), 5.23 (s, 2H, OCH2), 5.60 (dd, J = 5.1, 11.8 Hz, 1H, CH), 6.95 (d, J = 8.7 Hz, 2H, ArH), 7.35 (d, J = 8.4 Hz, 2H, ArH), 7.64 (d, J = 8.8 Hz, 2H, ArH), 7.70–7.76 (m, 2H, ArH), 7.76 (s, 1H, triazole-H), 8.15 (d, J = 8.3 Hz, 2H, ArH), 8.20 (dd, J = 0.8, 8.1 Hz, 2H, ArH), 8.52 (dd, J = 0.9, 7.3 Hz, 2H, ArH). 13C NMR (101 MHz,) δ 168.95, 164.20, 160.20, 153.50, 149.10, 147.38, 143.80, 134.62, 131.75, 131.70, 128.40, 128.30, 127.20, 126.71, 124.45, 123.90, 123.50, 122.10, 115.20, 62.10, 59.40, 48.10, 42.25, 38.01, 29.10, 21.95. HRMS (ESI) calcd for C35H29N7O6 [M + H]+ 644.2257, found 644.2265.
(E)-2-(2-(4-((4-(3-Ferrocenyl-acryloyl)phenoxy)methyl)-1H-1,2,3-triazol-1-yl)ethyl)-1H-benzo[de]isoquinoline-1,3(2H)-dione (14a). Yield-85%, red solid, MP = 220–222 °C, 1H NMR (400 MHz, CDCl3): δ 4.18 (s, 5H, FeH), 4.47 (s, 2H, FeH), 4.60 (s, 2H, FeH), 4.69 (t, J = 6.5 Hz, 2H, CH2), 4.80 (t, J = 7.0 Hz, 2H, CH2), 5.26 (s, 2H, OCH2), 7.03 (d, J = 8.5 Hz, 2H, ArH), 7.13 (d, J = 15.1 Hz, 1H, olefinic H), 7.72–7.76 (m, 3H, ArH + olefinic H), 7.78 (s, 1H, triazole-H), 7.97 (d, J = 8.5 Hz, 2H, ArH), 8.22 (dd, J = 0.9, 8.1 Hz, 2H, ArH), 8.50 (dd, J = 0.8, 7.3 Hz, 2H, ArH). 13C NMR (101 MHz, CDCl3) δ 188.20, 164.45, 161.72, 146.15, 143.41, 134.45, 131.89, 131.65, 131.50, 130.65, 128.20, 127.25, 123.30, 122.30, 118.80, 114.61, 79.54, 71.30, 69.92, 69.10, 62.31, 48.45, 39.12. HRMS (ESI) calcd for C36H28FeN4O4 [M + H]+ 637.1538, found 637.1549.
(E)-2-(2-(4-((4-(3-Ferrocenyl-acryloyl)phenoxy)methyl)-1H-1,2,3-triazol-1-yl)propyl)-1H-benzo[de]isoquinoline-1,3(2H)-dione (14b). Yield-85%, brick red solid, MP = 210–212 °C, 1H NMR (400 MHz, CDCl3): δ 2.37–2.44 (m, 2H, CH2), 4.15 (s, 5H, FeH), 4.26 (t, J = 6.7 Hz, 2H, CH2), 4.45 (t, J = 1.8 Hz, 2H, FeH), 4.49 (t, J = 7.1 Hz, 2H, CH2), 4.56 (t, J = 1.8 Hz, 2H, FeH), 5.24 (s, 2H, OCH2), 7.05 (d, J = 8.8 Hz, 2H, ArH), 7.11 (d, J = 15.3 Hz, 1H, olefinic H), 7.69–7.77 (m, 3H, ArH + olefinic H), 7.87 (s, 1H, triazole-H), 7.98 (d, J = 8.8 Hz, 2H, ArH), 8.21 (dd, J = 0.7, 8.2 Hz, 2H, ArH), 8.58 (dd, J = 0.8, 7.2 Hz, 2H, ArH). 13C NMR (101 MHz, CDCl3) δ 188.18, 164.44, 161.78, 146.01, 143.46, 134.44, 131.87, 131.67, 131.59, 130.69, 128.21, 127.14, 123.29, 122.36, 118.85, 114.64, 79.43, 71.32, 69.85, 69.02, 62.16, 48.49, 37.57, 29.01. HRMS (ESI) calcd for C37H30FeN4O4 [M + H]+ 651.1694, found 651.1685.
2-(2-(4-((4-(1-Acetyl-5-ferrocenyl-4,5-dihydro-1H-pyrazol-3-yl)phenoxy)methyl)-1H-1,2,3-triazol-1-yl)ethyl)-1H-benzo[de]isoquinoline-1,3(2H)-dione (15a). Yield-85%, light brown solid, MP = 198–200 °C, 1H NMR (400 MHz, CDCl3): δ 2.26 (s, 3H, CH3), 3.45 (dd, J = 4.8, 17.3 Hz, 1H, CH2), 3.56 (dd, J = 11.6, 17.6 Hz, 1H, CH2), 3.94–3.96 (m, 2H, CH2), 4.07–4.12 (m, 7H, FeH), 4.20–4.24 (m, 2H, CH2), 4.47 (s, 2H, FeH), 5.25 (s, 2H, OCH2), 5.45 (dd, J = 4.6, 11.5 Hz, 1H, CH), 7.05 (d, J = 8.1 Hz, 2H, ArH), 7.73–7.78 (m, 4H, ArH), 7.86 (s, 1H, triazole-H), 8.21 (d, J = 7.7 Hz, 2H, ArH), 8.56 (d, J = 6.8 Hz, 2H, ArH). 13C NMR (101 MHz,) δ 168.82, 164.42, 159.95, 153.81, 143.60, 134.40, 131.75, 131.60, 128.30, 128.20, 127.14, 124.70, 123.26, 122.35, 115.16, 87.42, 70.30, 68.69, 68.27, 62.10, 55.30, 48.45, 39.65, 37.56, 22.20. HRMS (ESI) calcd for C38H32FeN6O4 [M + H]+ 693.1912, found 693.1925.
2-(2-(4-((4-(1-Acetyl-5-ferrocenyl-4,5-dihydro-1H-pyrazol-3-yl)phenoxy)methyl)-1H-1,2,3-triazol-1-yl)propyl)-1H-benzo[de]isoquinoline-1,3(2H)-dione (15b). Yield-85%, light brown solid, MP = 190–192 °C, 1H NMR (400 MHz, CDCl3): δ 2.27 (s, 3H, CH3), 2.39–2.42 (m, 2H, CH2), 3.47 (d, J = 15.5 Hz, 1H, CH2), 3.59 (dd, J = 11.6, 16.3 Hz, 1H, CH2), 3.97 (s, 1H, CH2), 4.07–4.11 (m, 7H, FeH), 4.26 (s, 2H, CH2), 4.46–4.49 (m, 3H, FeH + CH2), 5.23 (s, 2H, OCH2), 5.43 (d, J = 8.5 Hz, 1H, CH), 7.05 (d, J = 8.0 Hz, 2H, ArH), 7.72–7.77 (m, 4H, ArH), 7.87 (s, 1H, triazole-H), 8.22 (d, J = 7.8 Hz, 2H, ArH), 8.58 (d, J = 6.8 Hz, 2H, ArH). 13C NMR (101 MHz,) δ 168.81, 164.46, 159.99, 153.83, 143.61, 134.47, 131.69, 131.60, 128.25, 128.23, 127.16, 124.72, 123.28, 122.36, 115.17, 87.45, 70.35, 68.66, 68.27, 62.13, 55.29, 48.50, 39.68, 37.58, 29.03, 22.11. HRMS (ESI) calcd for C39H34FeN6O4 [M + H]+ 707.1991, found 707.1985.
Materials and methods
Cell culturing
MCF7 cells were cultured in DMEM media with 10% FBS, and 1% penicillin–streptomycin and MDA-MB-231 cells were cultured in DMEM supplemented with 5% FBS and 1% penicillin–streptomycin, both incubated at 37 °C and 5% carbon dioxide.
MTT assay
Cells were seeded in 96 well plates at a density of 5000 cells per well in triplicate in media. After 24 hours, the test compounds diluted in complete Dulbecco's media Eagle's medium (DMEM) were added to each well. Cells were treated with a range of different concentrations of the compounds (1, 5, 10, 20, 50, 100 μM) for 24 hours at 37 °C and 5% carbon dioxide. Subsequently, sterile 5 μl of 5 mg mL−1 MTT (Sigma-Aldrich) dissolved in PBS was added to each well and incubated with cells for 2 hours. Solubilization solution (10% SDS, 10 mM HCl) of equal volume to the wells was then added to each well, which was incubated with cells for 16 hours at 37 °C. The optical density of each well was read at 570 nm using a microtiter plate reader (Thermo Fisher Scientific Multiskan GO Microplate Reader, SkanIt™ software).
Statistical analysis
The statistical analysis was performed using Excel®, and IC50 values were estimated using GraphpadPrism5 software (Hearne Scientific Software). The experiments were performed in duplicate, and the statistical significance was calculated using the student's t-test. A p-value of less than 0.05 was used to estimate the significance of the observations. A Z-factor was calculated for each 96-well plate and assays having Z-factor above >0.5 were included in the statistical analysis.39
Molecular docking
Molecular docking was carried out using Auto dock Vina40 and the crystal structures of human estrogen receptor alpha (ERα, PDB code 3ERT, 1.9 Å resolution) and estrogen receptor beta (ERβ, PDB code 3OLS, 2.2 Å resolution). The compatibility between the residue and the moderate active ligands was further checked using Protein–ligand Interaction Profiler software. SwissADME41 and pkCSM42 web-based applications were used to predict the ADMET properties of active compounds.
Abbreviations
ER | Estrogen receptor |
SERMs | Selective estrogen receptor modulators |
SAR | Structure activity relationship |
Conflicts of interest
The authors declare no conflict of interest.
Acknowledgements
Department of Science and Technology (DST), New Delhi, India, under INSPIRE programme (Shalini IF160180) has been gratefully acknowledged for fellowship. PS thanks the National Research Foundation South Africa for a Competitive Grant for unrated Researchers (Grant No. 121276), and the Centre for High-Performance Computing (CHPC), Cape Town, for the supercomputing facilities.
Notes and references
- Cancer Facts and Figures 2019, American Cancer Society, https://www.cancer.org/research/cancer-facts-statistics/all-cancer-facts-figures/cancer-facts-figures-2019.html.
- https://www.who.int/cancer/prevention/diagnosis-screening/breast-cancer/en/.
- W. F. Anderson, N. Chatterjee, W. B. Ershler and O. W. Brawley, Breast Cancer Res. Treat., 2002, 76, 27 CrossRef CAS PubMed.
- B. H. Mitlak and F. J. Cohen, Drugs, 1999, 57, 653 CrossRef CAS PubMed.
- M. N. Gomes, E. N. Muratov, M. Pereira, J. C. Peixoto, L. P. Rosseto, P. V. L. Cravo, C. H. Andrade and B. J. Neves, Molecules, 2017, 22, 1210 CrossRef PubMed.
- D. Israf, T. Khaizurin, A. Syahida, N. Lajis and S. Khozirah, Mol. Immunol., 2007, 44, 673 CrossRef CAS PubMed.
- T. Yamamoto, M. Yoshimura, F. Yamaguchi, T. Kouchi, R. Tsuji, M. Saito, A. Obata and M. Kikuchi, Biosci., Biotechnol., Biochem., 2004, 68, 1706 CrossRef CAS PubMed.
- N. Aoki, M. Muko, E. Ohta and S. Ohta, J. Nat. Prod., 2008, 71, 1308 CrossRef CAS PubMed.
- M. Chen, S. B. Christensen, J. Blom, E. Lemmich, L. Nadelmann, K. Fich, T. G. Theander, A. Kharazmi and A. Licochalcone, Antimicrob. Agents Chemother., 1993, 37, 2550 CrossRef CAS PubMed.
- P. M. Sivakumar, S. Ganesan, P. Veluchamy and M. Doble, Chem. Biol. Drug Des., 2010, 76, 407 CrossRef CAS PubMed.
- H. Suwito, J. Mustofa, P. Pudjiastuti, M. Z. Fanani, Y. Kimata-Ariga, R. Katahira, T. Kawakami, T. Fujiwara, T. Hase, H. M. Sirat and N. N. T. Puspaningsih, Molecules, 2014, 19, 21473 CrossRef PubMed.
- P. Boeck, P. C. Leal, R. A. Yunes, V. Cechinel, S. Lopez, M. Sortino, A. Escalante, R. L. E. Furlan and S. Zacchino, Arch. Pharm., 2005, 338, 87 CrossRef CAS PubMed.
- S. Cheenpracha, C. Karalai, C. Ponglimanont, S. Subhadhirasakul and S. Tewtrakul, Bioorg. Med. Chem., 2006, 14, 1710 CrossRef CAS PubMed.
- S. Burmaoglu, O. Algul, D. Aktas, A. Gobek and G. Gulbol, Bioorg. Med. Chem. Lett., 2016, 26, 3172 CrossRef CAS PubMed.
- S. Ducki, Adv. Anticancer Agents Med. Chem., 2009, 9, 336 CrossRef CAS PubMed.
- V. R. Solomon and H. Lee, Biomed. Pharmacother., 2012, 66, 213 CrossRef CAS PubMed.
- I. K. Lindamulage, H.-Y. Vu, C. Karthikeyan, J. Knockleby, Y.-F. Lee, P. Trivedi and H. Lee, Sci. Rep., 2017, 7, 10298 CrossRef PubMed.
- A. Modzelewska, C. Pettit, G. Achanta, N. E. Davidson, P. Huang and S. R. Khan, Bioorg. Med. Chem., 2006, 14, 3491 CrossRef CAS PubMed.
- H.-H. Gong, D. Addla, J.-S. Lv and C.-H. Zhou, Curr. Top. Med. Chem., 2016, 16, 3303 CrossRef CAS PubMed.
- T. Brider, B. Redko, F. Grynszpan and G. Gellerman, Tetrahedron Lett., 2014, 55, 6675 CrossRef CAS.
- R. Seliga, M. Pilatova, M. Sarissky, V. Viglasky, M. Walko and J. Mojzis, Mol. Biol. Rep., 2013, 40, 4129 CrossRef CAS PubMed.
- E. Diaz-Rubio, M. Martin, J. M. López-Vega, A. Casado and A. Benavides, Invest. New Drugs, 1994, 12, 277 CrossRef CAS PubMed.
- J. Noro, J. Maciel, D. Duarte, A. C. D. Olival, C. Baptista, A. C. D. Silva, M. J. Alves and P. Kong Thoo Lin, Org. Chem.: Curr. Res., 2015, 4, 144 Search PubMed.
- M. F. Braña, M. Cacho, M. A. Garcia, B. de Pascual-Teresa, A. Ramos, M. T. Dominguez, J. M. Pozuelo, C. Abradelo, M. F. Rey-Stolle, M. Yuste and M. Banez-Coronel, J. Med. Chem., 2004, 47, 1391 CrossRef PubMed.
- X. Liang, K. Xu, Y. Xu, J. Liu and X. Qian, Toxicol. Appl. Pharmacol., 2011, 256, 52 CrossRef CAS PubMed.
- X. Liang, Y. Xu, K. Xu, J. Liu and X. Qian, Mol. Cancer Res., 2010, 8, 1619 CrossRef CAS PubMed.
- S. Nagarajan, P. Shanmugavelan, M. Sathishkumar, R. Selvi, A. Ponnuswamy, H. Harikrishnan and V. Shanmugaiah, Chin. Chem. Lett., 2014, 25, 419 CrossRef CAS.
- M. W. Pertino, C. Theoduloz, E. Butassi, S. Zacchino and G. Schmeda-Hirschmann, Molecules, 2015, 20, 8666 CrossRef CAS PubMed.
- L. Zhao, L. Mao, G. Hong, X. Yang and T. Liu, Bioorg. Med. Chem. Lett., 2015, 25, 2540 CrossRef CAS PubMed.
- N. R. Penthala, L. Madhukuri, S. Thakkar, N. R. Madadi, G. Lamture, R. L. Eoff and P. A. Crooks, MedChemComm, 2015, 6, 1535 RSC.
- N. Pokhodylo, O. Shyyka and V. Matiychuk, Sci. Pharm., 2013, 81, 663 CrossRef CAS PubMed.
- S. Kumar, S. T. Saha, L. Gu, G. Palma, S. Perumal, A. S. Pillay, P. Singh, A. Anand, M. Kaur and V. Kumar, ACS Omega, 2018, 3, 12106 CrossRef CAS PubMed.
- B. Sharma, A. Singh, L. Gu, S. T. Saha, A. S. Pillay, N. Cele, P. Singh, M. Kaur and V. Kumar, RSC Adv., 2019, 9, 9809 RSC.
- S. Kumar, G. Palma, S. Perumal, M. Kaur, A. S. Pillay, R. Raj, P. Singh and V. Kumar, RSC Adv., 2019, 9, 42409 RSC.
- A. Rani, G. I. Singh, R. Kaur, M. Kaur, G. Palma, S. Perumal, O. Ebenezer, P. Awolade, P. Singh and V. Kumar, J. Organomet. Chem., 2020, 907, 121072 CrossRef.
- M. Karabacak, M. D. Altıntop, H. İ. Çiftçi, R. Koga, M. Otsuka, M. Fujita and A. Özdemir, Molecules, 2015, 20, 19066 CrossRef CAS PubMed.
- H. Wang, J. Zheng, W. Xu, C. Chen, D. Wei, W. Ni and Y. Pan, Molecules, 2017, 22, 1635 CrossRef PubMed.
- S. Sagar, L. Esau, B. Moosa, N.
M. Khashab, V. B. Bajic and M. Kaur, Adv. Anticancer Agents Med. Chem., 2014, 14, 170 CrossRef CAS PubMed.
- J. H. Zhang, T. D. Y. Chung and K. R. Oldenburg, J. Biomol. Screen, 1999, 4, 67 CrossRef PubMed.
- O. Trott and A. J. Olson, J. Comput. Chem., 2009, 31, 455 Search PubMed.
- http://www.swissadme.ch/.
- http://biosig.unimelb.edu.au/pkcsm/prediction.
Footnote |
† Electronic supplementary information (ESI) available: Scanned copies of 1H and 13C spectra of few representative compounds i.e. 12b, 12g, 13b, 13d, 13g, 14b, 15b and Table S1. See DOI: 10.1039/d0ra01822h |
|
This journal is © The Royal Society of Chemistry 2020 |
Click here to see how this site uses Cookies. View our privacy policy here.