DOI:
10.1039/D0RA02358B
(Paper)
RSC Adv., 2020,
10, 25475-25479
Silver-catalyzed regioselective deuteration of (hetero)arenes and α-deuteration of 2-alkyl azaarenes†
Received
13th March 2020
, Accepted 28th June 2020
First published on 6th July 2020
Abstract
A simple silver-catalyzed regioselective deuteration of (hetero)arenes and α-deuteration of 2-alkyl azaarenes has been described. This strategy provides an efficient and practical avenue to access various deuterated electron-rich arenes, azaarenes and α-deuterated 2-alkyl azaarenes with good to excellent deuterium incorporation utilizing D2O as the source of deuterium atoms.
Deuterium-labelled organic compounds are of great interest and importance, as they are widely used as internal standards in analytical chemistry,1 tools for elucidation of reaction mechanisms,2 metabolic or pharmacokinetic probes,3 and as biologically active compounds and pharmaceuticals.4 Indeed, the first deuterated drug, deutetrabenazine, which is recognized as a different orphan drug for the treatment of chorea versus tetrabenazine, was approved by the US Food and Drug Administration in April 2017. As a result, the development of efficient and selective routes for the synthesis of deuterium labeled organic compounds is a subject of increasing interest. Among possible approaches to incorporate deuterium atoms into an organic molecule,5 the H/D exchange reaction, which enables the direct deuterium labelling of the desired target molecule without the need to prefunctionalize the starting materials, represents the most straightforward and atom-efficient method. In recent years, extensive studies on H/D exchange reactions of aromatic compounds have been reported, including acid/base promoted pH-dependent H/D exchange reactions of electronically activated aromatic compounds6 (Scheme 1a) and transition metal catalyzed site-selective H/D exchange reactions7 (Scheme 1b). Among these, transition-metal-catalyzed reactions have gained significant momentum because these catalysts can control the site selectivity of H/D exchange reactions. However, the required ligands, the introduction of directing groups and/or the complexity of catalyst synthesis may not be readily accessible or economically viable. Therefore, there remains room to develop a simple, cost efficient and universal deuteration strategy with broad substrate scope, especially in view of the growing demand for deuterium-labelled compounds in synthetic chemistry and pharmaceutical industry.
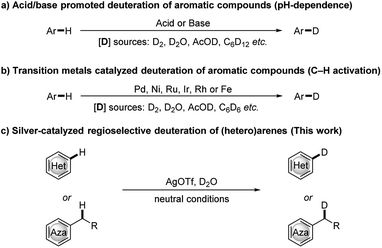 |
| Scheme 1 Selective H/D exchange reactions. | |
In past decades, silver has demonstrated their high efficiency as special mild Lewis acids, becoming catalysts of first choice for many types of catalytic reactions generally performed under mild reaction conditions and through experimentally simple procedures.8 However, despite considerable progress, silver-catalyzed selective H/D exchange reaction has remained much less explored to date.9 Quite recently, Huang reported an efficient Ag2CO3-catalyzed H/D exchange of five-membered heteroarenes using D2O as deuterium source at ambient temperature, but external base such as 1 equiv. of K2CO3 and a phosphine ligand were still needed.9b We here developed a practical and selective silver-catalyzed deuteration of (hetero)arenes and α-deuteration of 2-alkyl azaarenes utilizing D2O as the source of deuterium atom under mild neutral conditions that does not require any other additives (Scheme 1c). This protocol represents a practical and efficient method of silver-catalyzed regioselective H/D exchange reaction under mild neutral conditions, efficiently affording a wide range of deuterated electron-rich arenes, azaarenes and α-deuterated 2-alkyl azaarenes through experimentally simple procedures.
Initially, we began our studies by examining the model deuteration of 1,2,3,4-tetrahydroquinoline (1a) with D2O using various commercially available silver salts as the catalyst. To our delight, 94% deuterium incorporation was achieved in the ortho- and para-positions by using 10 mol% AgOTf in CDCl3 at 90 °C (Table 1, entry 1). Other silver salts such as Ag2CO3, Ag3PO4, AgBF4, AgOAc and Ag2O were also tested as catalysts, but gave low deuterium incorporation (entries 2–6). AgOTf showed higher activity probably because of the slow release of TfOH in the reaction.9a Control experiment suggests that AgOTf is necessary in this reaction (Table 1, entry 7). We then tested various solvents for this deuteration, and found that THF and 1,4-dioxane can also give good results, but DCE, CH3CN afforded a slightly low deuterium incorporation (entries 8–11). The reduction of the D2O amount from 20 to 5 equiv. led to a decrease in deuterium incorporation from 94% to 62% (Table 1, entry 12). When the reaction temperature was decreased to 60 °C, the efficiency of deuterium incorporation dramatically decreased, only giving 58% deuterium incorporation in the ortho- and para-positions (entry 13). In addition, under standard reaction conditions, other Lewis acids such as Cu(OTf)2, Zn(OTf)2, ZnCl2, FeCl3, and La(OTf)3 could also catalyze the H/D exchange, but giving a low deuterium incorporation, especially in the para-position.10,11
Table 1 Optimization of conditions for the silver-catalyzed deuteration of 1aa
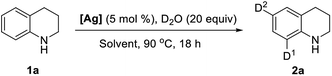
|
Entry |
[Ag] |
Solvent |
Deuterium incorporation (D1, D2)b |
Reaction conditions: 1a (0.5 mmol), [Ag] (0.025 mmol), D2O (10 mmol), solvent (1.0 mL), 90 °C for 18 h. Deuterium incorporation was determined by 1H NMR. D2O (2.5 mmol). At 60 °C. |
1 |
AgOTf |
CDCl3 |
94%, 94% |
2 |
Ag2CO3 |
CDCl3 |
11%, 8% |
3 |
Ag3PO4 |
CDCl3 |
21%, 23% |
4 |
AgBF4 |
CDCl3 |
91%, 58% |
5 |
AgOAc |
CDCl3 |
21%, 10% |
6 |
Ag2O |
CDCl3 |
13%, 10% |
7 |
— |
CDCl3 |
<5%, <5% |
8 |
AgOTf |
DCE |
74%, 81% |
9 |
AgOTf |
THF |
94%, 93% |
10 |
AgOTf |
1,2-Dioxane |
90%, 90% |
11 |
AgOTf |
CH3CN |
77%, 91% |
12c |
AgOTf |
CDCl3 |
62%, 62% |
13d |
AgOTf |
CDCl3 |
58%, 58% |
With the optimized catalytic system in hand, we evaluated the scope and limitations of this selective H/D exchange reaction. We firstly examined the deuteration of various electronic-rich arenes, and the results are listed in Table 2. We were pleased to find out that highly selective H/D exchanges with good to excellent degrees of deuteration were achieved. A variety of substituted 1,2,3,4-tetrahydroquinolines were smoothly converted to the deuterated isotopologues with high levels of deuterium incorporation (2a–2f). The bromide and dimethylphenylsilyl substituents could be well tolerated in this catalytic system. The deuteration of indoline derivatives as well as N-methyl aniline derivatives could be achieved with good to excellent deuterium incorporation in the ortho- and para-positions (2g–2l). The 9,10-dihydroacridine 1m was also deuterated to afford 2m in 88% yield with excellent deuterium incorporation. Moreover, other electron-rich aromatic compounds such as 1,3,5-trimethoxybenzene (1n) and multi-substituted anisole (1o) were also suitable substrates for the selective deuteration affording 2n and 2o with good deuterium incorporation. The conversion of N,N-disubstituted aniline derivatives such as N,N-dimethylaniline under the standard conditions only led to quite poor D-incorporation (<20% D incorporation).
Table 2 Silver-catalyzed selective deuteration of various electron-rich arenesa

|
Reaction conditions: 1 (0.5 mmol), AgOTf (0.025 mmol), D2O (10 mmol), CDCl3 (1.0 mL), 90 °C for 18 h. Isolated yield are given. 1,4-dioxane (1.0 mL) was used. |
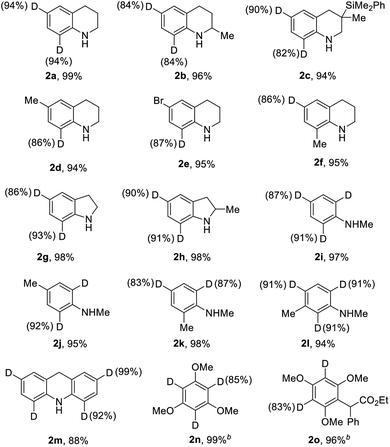 |
Subsequently, this catalytic system was applied to the deuteration of indole and azole derivatives. The deuteration of these compounds is of great interest and importance, as they are frequently observed in many pharmaceuticals and biologically active molecules.12 As shown in Table 3, a range of indoles with either electron-rich or electron-poor substituents were smoothly deuterated at C3 positions to afford deuterium-labelled products in excellent yields with good to excellent deuterium incorporation (4a–4m). Notably, functional groups such as chloride, ester, and aldehyde were well tolerated with this catalytic system. The 2,5-dimethyl pyrrole (3n) was also suitable substrate to afford 4n with 93% deuterium incorporation in the C3 and C4 positions. Remarkably, electron-deficient aza-arenes such as imidazoles and benzimidazole were also readily deuterated at C2 positions with high levels of deuterium incorporation. 1-Methyl benzimidazole (3q) could be also employed in this catalytic system, affording 4q with 97% deuterium incorporation. Also, 1,2,4-triazole could be smoothly deuterated at C3 and C5 positions with excellent deuterium incorporation. Unfortunately, the conversion of benzooxazole and benzothiazole under the standard conditions could not lead to D-incorporation.
Table 3 Silver-catalyzed selective deuteration of various azaarenesa

|
Reaction conditions: 3 (0.5 mmol), AgOTf (0.025 mmol), D2O (10 mmol), CDCl3 (1.0 mL), 90 °C for 18 h. Isolated yield are given. |
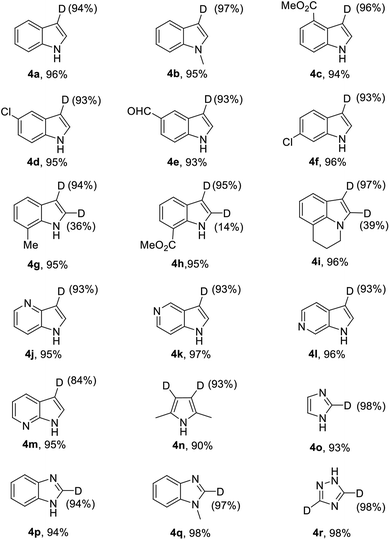 |
Furthermore, by using this silver catalytic system, we also successfully achieved the regioselective deuteration at the α-positions of 2-alkyl azaarenes. As shown in Table 4, the deuteration of 1,2,3,4-tetrahydroacridine gave the deuterated product 6a efficiently with 95% deuterium incorporation under the current reaction conditions. When the 2-methylquinoxaline was employed, both methyl group and C3 position were readily deuterated with high deuterium incorporation. In addition, the α-positions of alkyl-substituted quinolines 5c, 5d and 2-benzylpyridine 5e could be deuterated, giving the corresponding products in good yields, albeit with 24–40% deuterium incorporation. Also, α-deuteration of oxindole 5f gave deuterated product 6f in 96% yield and 91% deuterium incorporation.
Table 4 Silver-catalyzed α-deuteration of 2-alkyl azaarenesa
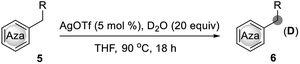
|
Reaction conditions: 5 (0.5 mmol), AgOTf (0.025 mmol), D2O (10 mmol), THF (1.0 mL), 90 °C for 18 h. Isolated yield are given. |
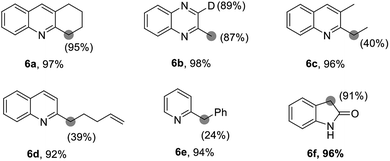 |
Conclusions
In summary, we have developed an efficient silver-catalyzed regioselective deuteration of (hetero)arenes and α-deuteration of 2-alkyl azaarenes using the simple D2O as the deuterating reagent. The reaction proceeds under neutral mild conditions in high yields with a good to excellent degree of deuterium incorporation. Electron-rich arenes and heteroarenes, such as 1,2,3,4-tetrahydroquinolines, indoline derivatives, N-methyl anilines, 1,3,5-trimethoxybenzene and indoles, were all deuterated with high deuterium incorporation. The protocol was also applied to the deuteration of electron-deficient azoles. Moreover, the α-position deuteration of 2-alkyl azaarenes was also achieved under mild neutral conditions.
Conflicts of interest
There are no conflicts to declare.
Acknowledgements
We gratefully acknowledge National Natural Science Foundation of China (NSFC) (21702028), Department of Science and Technology of Jilin Province (20180520206JH), the Collaborative Fund of Luzhou Government and Southwest Medical University (2019LZXNYDJ16) and the Research Fund of Southwest Medical University (2017-ZRQN-037) for financial support.
Notes and references
-
(a) J. Atzrodt and V. J. Derdau, Pd- and Pt-catalyzed H/D exchange methods and their application for internal MS standard preparation from a Sanofi-Aventis perspective, J. Label. Compd. Radiopharm., 2010, 53, 674–685 CrossRef CAS
;
(b) A. Nakanishi, Y. Fukushima, N. Miyazawa, K. Yoshikawa, T. Maeda and Y. J. Kurobayashi, Quantitation of Rotundone in Grapefruit (Citrus paradisi) Peel and Juice by Stable Isotope Dilution Assay, J. Agric. Food Chem., 2017, 65, 5026–5033 CrossRef CAS PubMed
;
(c) E. Stokvis, H. Rosing and J. H. Beijnen, Stable isotopically labeled internal standards in quantitative bioanalysis using liquid chromatography/mass spectrometry: necessity or not?, Rapid Commun. Mass Spectrom., 2005, 19, 401–407 CrossRef CAS PubMed
;
(d) C.-Y. Kao and R. W. Giese, Measurement of N7-(2‘-Hydroxyethyl)guanine in Human DNA by Gas Chromatography Electron Capture Mass Spectrometry, Chem. Res. Toxicol., 2005, 18, 70–75 Search PubMed
. -
(a) E. M. Simmons and J. F. Hartwig, On the Interpretation of Deuterium Kinetic Isotope Effects in C−H Bond Functionalizations by Transition-Metal Complexes, Angew. Chem., Int. Ed., 2012, 51, 3066–3072 CrossRef CAS PubMed
;
(b) T. Giagou and M. P. Meyer, Kinetic Isotope Effects in Asymmetric Reactions, Chem.–Eur. J., 2010, 16, 10616–10628 CrossRef CAS PubMed
. -
(a) E. M. Isin, C. S. Elmore, G. N. Nilsson, R. A. Thompson and L. Weidolf, Use of Radiolabeled Compounds in Drug Metabolism and Pharmacokinetic Studies, Chem. Res. Toxicol., 2012, 25, 532–542 Search PubMed
;
(b) P. Uhl, G. Fricker, U. Haberkorn and W. Mier, Radionuclides in drug development, Drug Discovery Today, 2015, 20, 198–208 CrossRef CAS PubMed
;
(c) A. E. Mutlib, Application of Stable Isotope-Labeled Compounds in Metabolism and in Metabolism-Mediated Toxicity Studies, Chem. Res. Toxicol., 2008, 21, 1672–1689 Search PubMed
;
(d) S. Nag, L. Lehmann, G. Kettschau, M. Toth, T. Heinrich, A. Thiele, A. Varrone and C. Halldin, Development of a novel fluorine-18 labeled deuterated fluororasagiline ([18F]fluororasagiline-D2) radioligand for PET studies of monoamino oxidase B (MAO-B), Bioorg. Med. Chem., 2013, 21, 6634–6641 CrossRef CAS PubMed
;
(e) K. Y. Wong, Y. Xu and L. Xu, Review of computer simulations of isotope effects on biochemical reactions: from the Bigeleisen equation to Feynman's path integral, Biochim. Biophys. Acta, Proteins Proteomics, 2015, 1854, 1782–1794 CrossRef CAS PubMed
. -
(a) A. Katsnelson, Heavy drugs draw heavy interest from pharma backers, Nat. Med., 2013, 19, 656 CrossRef CAS PubMed
;
(b) Y. Zhang, M. D. Tortorella, Y. Wang, J. Liu, Z. Tu, X. Liu, Y. Bai, D. Wen, X. Lu, Y. Lu and J. Talley, Synthesis of Deuterated Benzopyran Derivatives as Selective COX-2 Inhibitors with Improved Pharmacokinetic Properties, ACS Med. Chem. Lett., 2014, 5, 1162–1166 CrossRef CAS PubMed
;
(c) Y. Zhu, J. Zhou and B. Jiao, Deuterated Clopidogrel Analogues as a New Generation of Antiplatelet Agents, ACS Med. Chem. Lett., 2013, 4, 349–352 CrossRef CAS PubMed
;
(d) A. Mullard, Deuterated drugs draw heavier backing, Nat. Rev. Drug Discovery, 2016, 15, 219–221 CrossRef CAS PubMed
;
(e) T. G. Gant, Using Deuterium in Drug Discovery: Leaving the Label in the Drug, J. Med. Chem., 2014, 57, 3595–3611 CrossRef CAS PubMed
. -
(a) T. Junk and W. J. Catallo, Hydrogen isotope exchange reactions involving C–H (D, T) bonds, Chem. Soc. Rev., 1997, 26, 401–406 RSC
;
(b) Y. Sawama, Y. Monguchi and H. Sajiki, Efficient H–D Exchange Reactions Using Heterogeneous Platinum-Group Metal on Carbon–H2–D2O System, Synlett, 2012, 23, 959–972 CrossRef CAS
;
(c) J. Atzrodt, V. Derdau, W. J. Kerr and M. Reid, C−H Functionalisation for Hydrogen Isotope Exchange, Angew. Chem., Int. Ed., 2018, 57, 3022–3047 CrossRef CAS PubMed
;
(d) J. Atzrodt, V. Derdau, T. Fey and J. Zimmermann, The Renaissance of H/D Exchange, Angew. Chem., Int. Ed., 2007, 46, 7744–7765 CrossRef CAS PubMed
. -
(a) A. Martins and M. Lautens, A Simple, Cost-Effective Method for the Regioselective Deuteration of Anilines, Org. Lett., 2008, 10, 4351–4353 CrossRef CAS PubMed
;
(b) S. Rasku, K. Whl, J. Koskimies and T. Hase, Synthesis of isoflavonoid deuterium labeled polyphenolic phytoestrogens, Tetrahedron, 1999, 55, 3445–3454 CrossRef CAS
;
(c) J. C. Seibles, D. M. Bollinger and M. Orchin, Synthesis of Perdeuteriobenzo[a]pyrene, Angew. Chem., Int. Ed. Engl., 1977, 16, 656–657 CrossRef
;
(d) J. L. Garnett, M. A. Long, R. F. W. Vining and T. Mole, New simple method for rapid, selective aromatic deuteration using organoaluminum dihalide catalysts, J. Am. Chem. Soc., 1972, 94, 5913–5914 CrossRef CAS
;
(e) M. Pohjoispää, R. Mera-Adasme, D. Sundholm, S. Heikkinen, T. Hase and K. J. Wähälä, Capricious Selectivity in Electrophilic Deuteration of Methylenedioxy Substituted Aromatic Compounds, Org. Chem., 2014, 79, 10636–10640 CrossRef PubMed
. -
(a) W. J. Kerr, D. M. Lindsay, M. Reid, J. Atzrodt, V. Derdau, P. Rojahn and R. Weck, Iridium-catalysed ortho-H/D and -H/T exchange under basic conditions: C–H activation of unprotected tetrazoles, Chem. Commun., 2016, 52, 6669–6672 RSC
;
(b) W. J. Kerr, M. Reid and T. Tuttle, Iridium-Catalyzed C–H Activation and Deuteration of Primary Sulfonamides: An Experimental and Computational Study, ACS Catal., 2015, 5, 402–410 CrossRef CAS
;
(c) B. McAuley, M. J. Hickey, L. P. Kingston, J. R. Jones, W. J. S. Lockley, A. N. Mather, E. Spink, S. P. Thompson and D. J. J. Wilkinson, Convenient and efficient deuteration of functionalized aromatics with deuterium oxide: catalysis by cycloocta-1,5-dienyliridium(I) 1,3-dionates, J. Label. Compd. Radiopharm., 2003, 46, 1191–1204 CrossRef CAS
;
(d) D. Hesk, P. R. Das and B. J. Evans, Deuteration of acetanilides and other substituted aromatics using [Ir(COD)(Cy3P)(Py)]PF6 as catalyst, J. Label. Compd. Radiopharm., 1995, 36, 497–502 CrossRef CAS
;
(e) J. R. Heys, A. Y. L. Shu, S. G. Senderoff and N. M. J. Phillips, Deuterium exchange labelling of substituted aromatics using [IrH2(Me2CO)2(PPh3)2]BF4, J. Label. Compd. Radiopharm., 1993, 33, 431–438 CrossRef CAS
;
(f) M. J. Hickey, J. R. Jones, L. P. Kingston, W. J. S. Lockley, A. N. Mather, B. M. McAuley and D. J. Wilkinson, Iridium-catalysed labelling of anilines, benzylamines and nitrogen heterocycles using deuterium gas and cycloocta-1,5-dienyliridium(I) 1,1,1,5,5,5-hexafluoropentane-2,4-dionate, Tetrahedron Lett., 2003, 44, 3959–3961 CrossRef CAS
;
(g) D. Giunta, M. Hölscher, C. W. Lehmann, R. Mynott, C. Wirtz and W. Leitner, Room Temperature Activation of Aromatic C-H Bonds by Non-Classical Ruthenium Hydride Complexes Containing Carbene Ligands, Adv. Synth. Catal., 2003, 345, 1139–1145 CrossRef CAS
;
(h) B. Groll, M. Schnurch and M. D. Mihovilovic, Selective Ru(0)-Catalyzed Deuteration of Electron-Rich and Electron-Poor Nitrogen-Containing Heterocycles, J. Org. Chem., 2012, 77, 4432–4437 CrossRef PubMed
;
(i) C. P. Lenges, P. S. White and M. Brookhart, Hydrogen/Deuterium Exchange Reactions and Transfer Hydrogenations Catalyzed by [C5Me5Rh(olefin)2] Complexes: Conversion of Alkoxysilanes to Silyl Enolates, J. Am. Chem. Soc., 1999, 121, 4385–4396 CrossRef CAS
;
(j) M. Zhan, H. Jiang, X. Pang, T. Zhang, R. Xu, L. Zhao, Y. Liu, Y. Gong and Y. Chen, A convenient method for the Ru(0)-catalyzed regioselective deuteration of N-alkyl-substituted anilines, Tetrahedron Lett., 2014, 55, 5070–5073 CrossRef CAS
;
(k) S. Ma, G. Villa, P. S. Thuy-Boun, A. Homs and J. Q. Yu, Palladium-Catalyzed ortho-Selective C−H Deuteration of Arenes: Evidence for Superior Reactivity of Weakly Coordinated Palladacycles, Angew. Chem., Int. Ed., 2014, 53, 734–737 CrossRef CAS PubMed
;
(l) A. M. Krause-Heuer, N. R. Yepuri, T. A. Darwish and P. J. Holden, Mild Conditions for Deuteration of Primary and Secondary Arylamines for the Synthesis of Deuterated Optoelectronic Organic Molecules, Molecules, 2014, 19, 18604–18617 CrossRef PubMed
;
(m) H. Sajiki, N. Ito, H. Esaki, T. Maesawa, T. Maegawa and K. Hirota, Aromatic ring favorable and efficient H–D exchange reaction catalyzed by Pt/C, Tetrahedron Lett., 2005, 46, 6995–6998 CrossRef CAS
;
(n) V. Derdau, J. Atzrodt, J. Zimmermann, C. Kroll and F. Brückner, Hydrogen-Deuterium Exchange Reactions of Aromatic Compounds and Heterocycles by NaBD4-Activated Rhodium, Platinum and Palladium Catalysts, Chem.–Eur. J., 2009, 15, 10397–10404 CrossRef CAS PubMed
;
(o) D. Zhao, H. Luo, B. Chen, W. Chen, G. Zhang and Y. Yu, Palladium-Catalyzed H/D Exchange Reaction with 8-Aminoquinoline as the Directing Group: Access to ortho-Selective Deuterated Aromatic Acids and β-Selective Deuterated Aliphatic Acids, J. Org. Chem., 2018, 83, 7860–7866 CrossRef CAS PubMed
;
(p) Y. Sawama, K. Park, T. Yamada and H. Sajiki, New Gateways to the Platinum Group Metal-Catalyzed Direct Deuterium-Labeling Method Utilizing Hydrogen as a Catalyst Activator, Chem. Pharm. Bull., 2018, 66, 21–28 CrossRef CAS PubMed
;
(q) J. Krüger, B. Manmontri and G. Fels, Iridium-Catalyzed H/D Exchange, Eur. J. Org. Chem., 2005, 1402–1408 CrossRef
;
(r) T. Yamada, K. Park, N. Yasukawa, K. Morita, Y. Monguchi, Y. Sawama and H. Sajiki, Mild and Direct Multiple Deuterium-Labeling of Saturated Fatty Acids, Adv. Synth. Catal., 2016, 358, 3277–3282 CrossRef CAS
. -
(a) M. Naodovic and H. Yamamoto, Asymmetric Silver-Catalyzed Reactions, Chem. Rev., 2008, 108, 3132–3148 CrossRef CAS PubMed
;
(b) Y. Yamamoto, Silver-Catalyzed Csp−H and Csp−Si Bond Transformations and Related Processes, Chem. Rev., 2008, 108, 3199–3222 CrossRef CAS PubMed
;
(c) Q.-Z. Zheng and N. Jiao, Ag-catalyzed C–H/C–C bond functionalization, Chem. Soc. Rev., 2016, 45, 4590–4627 RSC
;
(d) G. Fang and X. Bi, Silver-catalysed reactions of alkynes: recent advances, Chem. Soc. Rev., 2015, 44, 8124–8173 RSC
;
(e) K. Sekine and T. Yamada, Silver-catalyzed carboxylation, Chem. Soc. Rev., 2016, 45, 4524–4532 RSC
;
(f) M. P. Muñoz, Silver and platinum-catalysed addition of O–H and N–H bonds to allenes, Chem. Soc. Rev., 2014, 43, 3164–3183 RSC
. -
(a) An example on the catalytic H/D exchange of arenes in acidic solvents, in which silver salt was examined, see: D. Munz, M. Webster-Gardiner, R. Fu, T. Strassner, W. A. Goddard III and T. B. Gunnoe, Proton or Metal? The H/D Exchange of Arenes in Acidic Solvents, ACS Catal., 2015, 5, 769–775 CrossRef CAS
;
(b) An example on the catalytic H/D exchange of hydrogen isotope exchange of five-membered heteroarenes by Ag2CO3/JohnPhos, see: E.-C. Li, G.-Q. Hu, Y.-X. Zhu, H.-H. Zhang, K. Shen, X.-C. Hang, C. Zhang and W. Huang, Ag2CO3-Catalyzed H/D Exchange of Five-Membered Heteroarenes at Ambient Temperature, Org. Lett., 2019, 21, 6745–6749 CrossRef CAS PubMed
. - Other Lewis acids were also tested under the standard condition, however, gave a lower deuterium incorporation, especially in the para-position, Cu(OTf)2 (75% D ortho, 75% D para), Zn(OTf)2 (60% D ortho, 20% D para), ZnCl2 (24% D ortho, 14% D para), FeCl3 (75% D ortho, 40% D para), and La(OTf)3 (<10% D ortho, <10% D para).
- Recently, Werner and coworkers reported B(C6F5)3-catalyzed regioselective deuteration of electron-rich aromatic and heteroaromatic compounds, see: W. Li, M.-M. Wang, Y. Hu and T. Werner, B(C6F5)3-Catalyzed Regioselective Deuteration of Electron-Rich Aromatic and Heteroaromatic Compounds, Org. Lett., 2017, 19, 5768–5771 CrossRef CAS PubMed
. -
(a) T. Eicher and S. Hauptmann, The Chemistry of Heterocycles, Wiley-VCH, Weinheim, 2003 CrossRef
;
(b) M. Ishikura, T. Abe, T. Choshi and S. Hibino, Simple indole alkaloids and those with a non-rearranged monoterpenoid unit, Nat. Prod. Rep., 2013, 30, 694–752 RSC
;
(c) V. Sharma, P. Kumar and D. Pathak, Biological Importance of the Indole Nucleus in Recent Years: A Comprehensive Review, J. Heterocycl. Chem., 2010, 47, 491–502 CAS
;
(d) A. J. Kochanowska-Karamyan and M. T. Hamann, Marine Indole Alkaloids: Potential New Drug Leads for the Control of Depression and Anxiety, Chem. Rev., 2010, 110, 4489–4497 CrossRef CAS PubMed
;
(e) J. S. Carey, D. Laffan, C. Thomson and M. T. Williams, Analysis of the reactions used for the preparation of drug candidate molecules, Org. Biomol. Chem., 2006, 4, 2337–2347 RSC
.
Footnote |
† Electronic supplementary information (ESI) available: Experimental details, and characterization data. See DOI: 10.1039/d0ra02358b |
|
This journal is © The Royal Society of Chemistry 2020 |
Click here to see how this site uses Cookies. View our privacy policy here.