DOI:
10.1039/D0RA02432E
(Paper)
RSC Adv., 2020,
10, 18008-18015
Synthesis and biological evaluation of dehydroabietic acid-pyrimidine hybrids as antitumor agents†
Received
16th March 2020
, Accepted 30th April 2020
First published on 11th May 2020
Abstract
A series of novel dehydroabietic acid derivatives containing pyrimidine moieties were designed and synthesized to explore more efficacious and less toxic antitumor agents according to the principle of combination and hybridization. The cytotoxicity against human liver cancer (HepG2) cells, human breast cancer (MCF-7) cells, human colon cancer (HCT-116) cells, human lung cancer (A549) cells, and human normal liver cells (LO2) was estimated by MTT assay in vitro. Cytotoxic activity screening revealed that most of the compounds showed moderate to high levels of cytotoxicity against these four cancer cell lines and that some displayed more potent inhibitory activities compared with 5-FU. In particular, compound 3b exhibited promising cytotoxicity with IC50 values ranging from 7.00 to 11.93 μM against all the tested cell lines and displayed weak cytotoxicity towards normal cells. Besides, cell cycle analysis indicated that compound 3b mainly arrested MCF-7 cells at the S stage and induced cell apoptosis.
Introduction
As one of the main causes of death globally, cancer has already seriously threatened human health and lives. Despite significant progress in the diagnostic methods and management of cancer, the successful treatment of cancer remains a challenge.1,2 Traditional chemotherapy is still one of the most common methods used for cancer therapy.3 However, the chemotherapeutic drugs usually cause cytotoxicity on normal proliferating tissues and display significant toxic side effects and drug resistance.4 Thus, there is a pressing need for novel and more potent antitumor agents with high selectivity for tumor cells and low toxicity to normal tissue.
Natural products, with a wide structural diversity and pleiotropic effects, are a rich source of new drug candidates and lead molecules.5,6 It is reported that approximately 60% of clinically used antitumor drugs originated from natural products.7 According to the results of investigation in natural products for their potential therapeutic effects, we have been inspired to explore the influences of dehydroabietic acid (DHAA) derivatives for their activities. DHAA is a natural occurring tricyclic diterpenic resin acid, which can be readily isolated from pinus rosin or disproportionate rosin.8 In the early studies, DHAA and its derivatives have been demonstrated to possess a broad spectrum of physiological or pharmacological activities, including antimicrobial,9,10 antifungal,11 antiviral,12 anti-aging,13 anti-inflammatory,14 BK channel-opening15 and herbicidal16 activities. Particularly, it is reported that a great number of DHAA derivatives have various anticancer activities in many human cancers, including cervical carcinoma cells, hepatocarcinoma cells, ovarian cancer cells, and breast cancer cells. Moreover, they were proved to act at various stages of tumor development to inhibit tumor initiation and promotion, as well as to induce tumor cell differentiation and apoptosis.17,18 As a result, dehydroabietic acid is a promising starting material to screen for novel potential antitumor agents.
The pyrimidine moiety, as one of the most important heterocyclic scaffolds, is considered a privileged structure in medicinal chemistry for drug discovery.19,20 Pyrimidine derivatives have been reported to have a broad spectrum of biological activities, such as antiviral,21,22 antibacterial,23 antifungal,24 anti-inflammatory,25,26 COX-2 inhibitors,27 antituberculosis,28 herbicidal29 and insecticidal30 activities. Moreover, previous studies have found that numerous pyrimidine derivatives exhibited promising anticancer activity.31–33
The above mentioned findings indicate that the fusion of a pyrimidine moiety with the skeleton of DHAA may produce novel derivatives with improved anticancer properties. In continuation of our previous work,34–36 we designed and synthesized a series of dehydroabietic acid derivatives bearing pyrimidine as potential anticancer agents according to the strategy of molecular hybridization. The cytotoxicity of the target compounds was evaluated against human normal liver cells (LO2), human liver cancer (HepG2), human breast cancer (MCF-7) cells, human colon cancer (HCT-116) cells and human lung cancer (A549) cells and the possible mechanisms of cell proliferation inhibition were studied.
Results and discussion
Chemistry
The synthetic route of the target dehydroabietic acid-pyrimidine hybrids is shown in Scheme 1. Dehydroabietic acid-2′-bromoethyl ester 2 was obtained by the reaction of dehydroabietic acid (DHAA, 1) and 1,2-dibromoethane refluxed in acetone in the presence of anhydrous potassium carbonate. The intermediate 2 was then reacted with substituted pyrimidine-2-thiol to give target compounds 3a–3o. The structures of all the derivatives were then confirmed by IR, 1H NMR, 13C NMR and high-resolution mass spectroscopy.
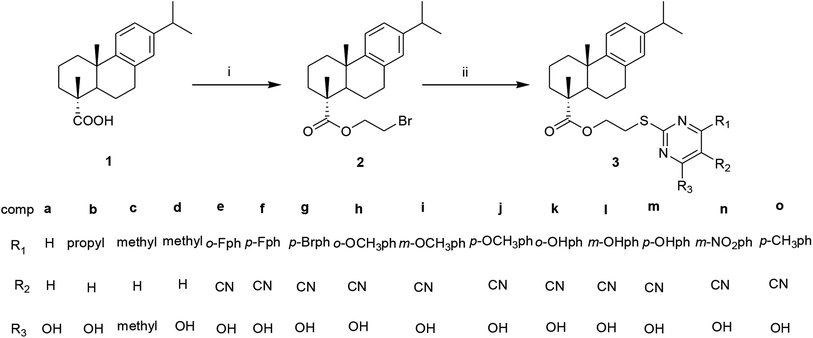 |
| Scheme 1 Synthesis of dehydroabietic acid-pyrimidine hybrids 3a–3o. Reagents and conditions: (i) K2CO3, acetone, 1,2-dibromoethane, 60 °C, 6 h, 78.6%; (ii) K2CO3, DMF, substituted pyrimidine-2-thiol, RT, 8–10 h, 51.0–85.3%. | |
MTT assay
The target compounds (3a–3o) were screened for the in vitro cytotoxicity using MTT assay method on four cancer cell lines liver (HepG2), breast (MCF-7), colon (HCT-116), lung (A549) and normal cell line human liver cells (LO2), using 5-fluorouracil (5-FU) as the positive control. The IC50 (μM) values are depicted in Fig. 1.
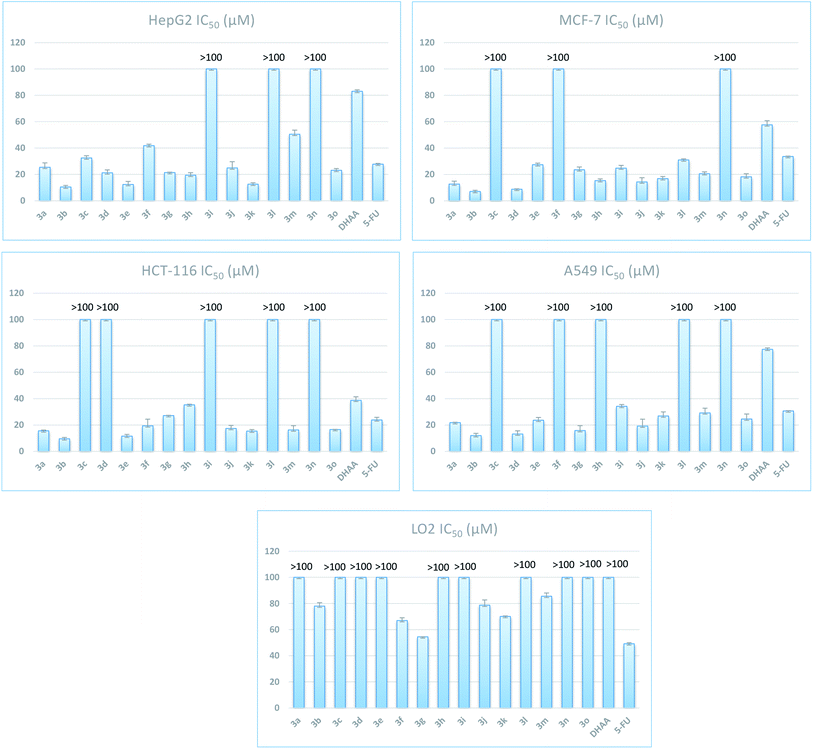 |
| Fig. 1 Effect of compounds 3a–3o against on the cell viability of different cell lines. | |
As shown in Fig. 1, most of target compounds showed certain anticancer activities against the tumor cells (HepG2, MCF-7, HCT-116 and A549). It was obvious that the introduction of pyrimidine on the DHAA skeleton markedly increased antitumor activity compared with the parent compound. Among these dehydroabietic acids pyrimidine derivatives, as the most promising target compounds, compound 3b showed preferable cytotoxic activity against certain cancer cells than the positive control 5-FU, with IC50 values of 10.42 ± 1.20, 7.00 ± 0.96, 9.53 ± 1.03 and 11.93 ± 1.76 μM against the HepG-2, MCF-7, HCT-116 and A549 cancer cells, respectively. All the compounds except compounds 3c, 3f and 3n demonstrated better cytotoxic inhibition than 5-FU on MCF-7 cell line, with IC50 in the range of 7.00–30.92 μM. In addition, compounds 3a, 3b, 3e, 3f, 3j, 3k, 3m and 3o displayed better cytotoxic inhibition than 5-FU on HCT-116 cell line, with IC50 in the range of 9.53–19.42 μM. All the compounds showed lower cytotoxicity on LO2 cells than on that of these four cancer cell lines. When pyrimidine ring is not attached with benzene (3a–3d), the hybrids bearing hydroxyl group exhibited better cytotoxic activity. The presence of fluorine in ortho position of the benzene ring show more active than para. In HepG2, MCF-7, HCT-116 assays, the derivatives attached with benzene bearing an electron-donating group (–OH, –OCH3) in meta position exhibited less cytotoxicity than the other two positions.
The morphological analysis
One important sign of cell apoptotic is the aberrant morphological changes of chromatin. After being treated with compound 3b (0 μM, 5 μM, 10 μM, 15 μM) for 48 h, MCF-7 cells were stained with Hoechst 33258 and observed under fluorescence microscope. The results showed that most of the cells exhibited the weak blue fluorescence in the control cells. In the 5.0 μM, 10.0 μM and 15.0 μM groups, some exhibited bright-blue fluorescence because of chromatin condensation. The number of apoptotic nuclei significantly increased along with the increase of the concentration of 3b to 15.0 μM (Fig. 2).
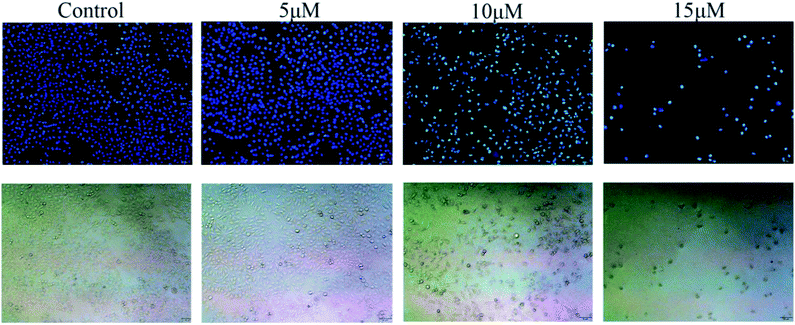 |
| Fig. 2 Morphological change of apoptosis in MCF-7 cells which were treated with compound 3b (0 μM, 5 μM, 10 μM, 15 μM) for 48 h. The treated cells were stained with Hoechst 33258 and observed by a fluorescence microscope. | |
Cell apoptosis assay
To explore the mechanism of cytotoxic activity of compound 3b further, cell apoptosis assay on MCF-7 cells was performed using an Annexin V-FITC&PI Apoptosis Detection Kit. As shown in Fig. 3, the apoptosis rate of MCF-7 cells at different concentrations increased from 2.84% of the control group to 5.87%, 18.30% and 43.30%, respectively. Hence, compound 3b may induce apoptosis of MCF-7 cells in a dose-dependent manner.
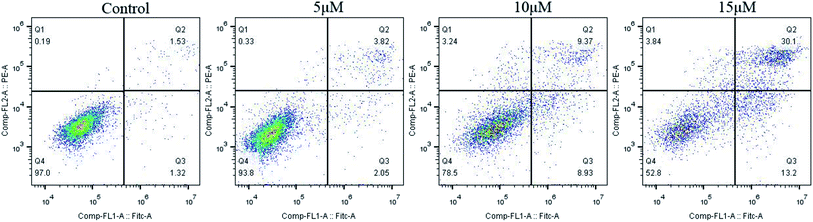 |
| Fig. 3 Effects of compound 3b on cell apoptosis of MCF-7 cells. The cells were treated with different concentrations of compound 3b (0 μM, 5 μM, 10 μM, 15 μM) for 48 h and then measured by flow cytometry. | |
Cell cycle assay
Many anticancer drugs interact with cells leading to cell growth arrest. In order to investigate the effect of our synthesized dehydroabietic acid-pyrimidine hybrids on cell cycle of humor cells, the most promising compound 3b against MCF-7 was chosen to study by flow cytometry according to the results of the MTT assay. Cell cycle distribution was investigated by flow cytometric analysis after staining of the DNA with propidium iodide (PI). MCF-7 cells were treated with different concentrations of compound 3b (0 μM, 5 μM, 10 μM, 15 μM) for 48 h and then measured by flow cytometry. As shown in Fig. 4, the percentages of cells in S phase at different concentrations increased from 30.09% of the control group to 34.03%, 37.71%, and 40.69%. It suggested that target compound 3b mainly arrested cell cycle of MCF-7 in S phase.
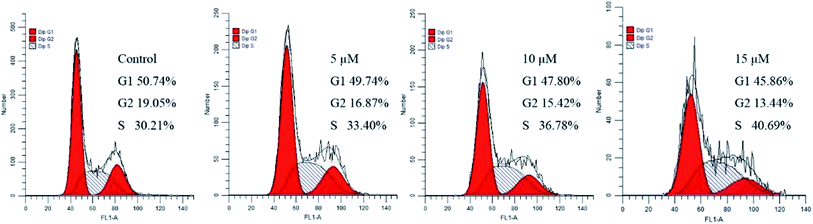 |
| Fig. 4 Effects of compound 3b on cell cycle of MCF-7 cells. MCF-7 cells were treated with compounds 3b (0 μM, 5 μM, 10 μM, 15 μM) for 48 h and then measured by flow cytometry. | |
Experimental
General
All reagents were obtained from commercial suppliers and used without further purification. The reactions were monitored by thin-layer chromatography (TLC) and visualized by UV lamp at a wavelength of 254 nm. The column chromatography was performed on 200–300 silica gel. 1H NMR spectra data were recorded on 600 MHz spectrometer (Bruker Advance spectrometer). 13C NMR spectra data were recorded on 151 MHz spectrometer (Bruker Advance spectrometer). Chemical shifts were recorded in ppm on δ scale using CDCl3 as solvent, tetramethylsilane (TMS) was used as an internal standard and coupling constants (J) in Hz. Mass spectra were determined on an FTMS ESI spectrometer.
Synthesis
General procedure for synthesis of dehydroabietic acid-2′-bromoethyl ester (2). Dehydroabietic acid 1 (21.12 mmol), dibromoethane (40.00 mmol), and anhydrous K2CO3 (2.91 g) were added to 40 mL acetone and the reaction was heated to reflux for 6 h. The solvent was removed under vacuum. The resulting residue was washed with petroleum ether and distilled water successively, and filtered under reduced pressure. Then the residue was dried and purified by silica gel chromatography with a mixture of petroleum ether and ethyl acetate (20
:
1) as eluent to give compound 2.White solid, yield 78.6%, mp 46.2–49.4 °C; 1H NMR (600 MHz, CDCl3) δ 7.22 (d, 1H, J = 8.4 Hz), 7.06 (d, 1H, J = 8.4 Hz), 6.95 (s, 1H), 4.50–4.37 (m, 2H), 3.57–3.54 (t, 2H, J = 6.0 Hz), 3.01–2.97 (m, 1H), 2.94–2.85 (m, 2H), 2.33–2.37 (m, 2H), 1.92–1.71 (m, 5H), 1.47–1.57 (m, 2H), 1.35 (s, 3H), 1.28 (s, 3H), 1.27 (d, 6H, J = 1.8 Hz). 13C NMR (150 MHz, CDCl3) δ 179.56, 148.22, 147.18, 136.13, 128.39, 125.62, 125.40, 65.46, 49.25, 46.08, 39.36, 38.39, 38.09, 34.91, 30.49, 25.46, 23.28, 20.13, 17.98. IR (KBr) ν/cm−1: 2867, 1733, 1496, 1498, 1387, 1243, 1172, 1006, 821, 682.
Procedure for synthesis of target compounds 3a–3o. Compounds 2 (3 mmol), substituted pyrimidine-2-thiol (3 mmol) and dry K2CO3 (3 mmol) were added to dry DMF (20 mL) in a round bottom and the reaction mixture was stirred at room temperature for 8–10 h. When the reaction was completed (monitored by TLC), the reaction mixture was poured into iced water (100 mL), and then acidified with acetic acid. The obtained precipitate was filtered, washed with H2O, dried, and purified via column chromatography on silica gel with petroleum ether/ethyl acetate (v/v = 30
:
1) to afford the compounds 3a–3o.
Hybrid compound 3a. Light yellow solid, yield 56.1%, mp 82.2–84.7 °C; 1H NMR (600 MHz, CDCl3) δ 7.81 (d, J = 6.6 Hz, 1H), 7.28 (s, 1H), 7.18 (d, J = 8.2 Hz, 1H), 7.02 (d, J = 8.1 Hz, 1H), 6.90 (s, 1H), 6.21 (d, J = 6.5 Hz, 1H), 4.34 (dd, J = 31.8, 8.7 Hz, 2H), 3.45 (d, J = 15.9 Hz, 2H), 2.90 (s, 1H), 2.84 (s, 1H), 2.31 (d, J = 11.4 Hz, 1H), 2.24 (d, J = 12.5 Hz, 1H), 2.06 (s, 1H), 1.83 (d, J = 5.4 Hz, 1H), 1.77 (d, J = 9.6 Hz, 2H), 1.66 (s, 1H), 1.43 (dd, J = 13.1, 7.0 Hz, 1H), 1.31 (s, 1H), 1.28 (s, 3H), 1.24 (d, J = 6.9 Hz, 6H), 1.22 (s, 3H). 13C NMR (151 MHz, CDCl3) δ 178.43, 164.93, 161.23, 155.03, 146.93, 145.89, 134.77, 127.05, 124.31, 124.07, 111.22, 62.62, 47.85, 44.82, 38.04, 37.05, 36.76, 33.58, 30.18, 29.44, 25.30, 24.12, 24.10, 21.94, 18.68, 16.60. IR (KBr), ν/cm−1: 3414, 2929, 1724, 1672, 1529, 1460, 1384, 1242, 1172, 1118, 979, 825. HR-MS (m/z) (ESI): calcd for C26H34N2O3S [M + H]+: 457.2525, calcd 457.2505.
Hybrid compound 3b. White solid, yield 70.5%, mp 69.8–71.3 °C; 1H NMR (600 MHz, CDCl3) δ 7.18 (d, J = 8.2 Hz, 1H), 7.02 (d, J = 8.0 Hz, 1H), 6.89 (s, 1H), 4.35 (s, 1H), 4.29 (s, 1H), 3.39 (s, 1H), 2.89 (s, 1H), 2.83 (s, 1H), 2.45 (s, 1H), 2.44 (s, 1H), 2.43 (s, 1H), 2.31 (d, J = 12.0 Hz, 1H), 2.26 (d, J = 12.4 Hz, 1H), 1.78 (s, 1H), 1.77 (s, 1H), 1.68 (s, 1H), 1.67 (s, 1H), 1.66 (s, 1H), 1.65 (s, 1H), 1.50 (d, J = 3.5 Hz, 1H), 1.43 (dd, J = 12.4, 6.5 Hz, 1H), 1.28 (s, 3H), 1.24 (s, 3H), 1.23 (s, 3H), 1.22 (s, 3H), 1.19 (s, 1H), 1.13 (dd, J = 14.2, 7.3 Hz, 1H), 0.96 (s, 1H), 0.95 (s, 2H), 0.94 (s, 1H). 13C NMR (151 MHz, CDCl3) δ 178.45, 169.35, 166.08, 160.15, 146.93, 145.85, 134.76, 127.06, 124.29, 124.06, 107.94, 62.81, 47.83, 44.89, 39.61, 38.05, 37.07, 36.73, 33.58, 30.23, 29.24, 25.32, 24.10, 21.93, 21.11, 18.69, 16.60, 13.82. IR (KBr), ν/cm−1: 3414, 2956, 1728, 1660, 1577, 1539, 1458, 1386, 1230, 1170, 1124, 1037, 821. HR-MS (m/z) (ESI): calcd for C29H42N2O3S [M + H]+: 499.2994, calcd 499.2981.
Hybrid compound 3c. Yellow solid, yield 81.8%, mp 103.1–104.3 °C; 1H NMR (600 MHz, CDCl3) δ 7.18 (d, J = 8.2 Hz, 1H), 7.02 (d, J = 9.5 Hz, 1H), 6.90 (s, 1H), 6.69 (s, 1H), 4.40 (s, 1H), 4.34 (s, 1H), 3.42 (s, 2H), 2.89 (d, J = 6.3 Hz, 1H), 2.85 (s, 1H), 2.39 (s, 6H), 2.31 (d, J = 12.2 Hz, 1H), 2.26 (s, 1H), 1.84 (dd, J = 11.8, 6.6 Hz, 1H), 1.80 (d, J = 11.0 Hz, 2H), 1.71 (s, 1H), 1.66 (d, J = 10.4 Hz, 1H), 1.47 (s, 2H), 1.29 (s, 3H), 1.24 (d, J = 6.9 Hz, 6H), 1.22 (s, 3H). 13C NMR (151 MHz, CDCl3) δ 178.54, 170.30, 167.23, 147.00, 145.80, 134.89, 127.08, 124.30, 124.04, 115.96, 63.39, 47.84, 44.86, 38.07, 37.08, 36.70, 33.59, 30.28, 29.57, 25.36, 24.11, 23.96, 21.93, 18.74, 16.63. IR (KBr), ν/cm−1: 3429, 2929, 2378, 1724, 1645, 1579, 1537, 1440, 1340, 1265, 1172, 1124, 1033, 887. HR-MS (m/z) (ESI): calcd for C28H38N2O2S [M + H]+: 467.2732, calcd 467.2711.
Hybrid compound 3d. Pink solid, yield 54.8%, mp 119.4–121.1 °C; 1H NMR (600 MHz, CDCl3) δ 7.18 (d, J = 8.2 Hz, 1H), 7.02 (d, J = 8.2 Hz, 1H), 6.89 (s, 1H), 6.07 (s, 1H), 4.37 (s, 1H), 4.33 (s, 1H), 3.46 (d, J = 6.4 Hz, 1H), 2.90 (d, J = 7.6 Hz, 1H), 2.83 (d, J = 6.9 Hz, 1H), 2.32 (d, J = 12.3 Hz, 1H), 2.27 (d, J = 5.8 Hz, 1H), 2.25 (s, 3H), 1.85 (d, J = 4.8 Hz, 1H), 1.78 (d, J = 8.9 Hz, 2H), 1.75 (d, J = 5.6 Hz, 1H), 1.72 (d, J = 3.0 Hz, 1H), 1.67 (s, 1H), 1.50 (t, J = 5.1 Hz, 1H), 1.44 (dd, J = 13.1, 7.1 Hz, 1H), 1.29 (s, 3H), 1.24 (d, J = 6.9 Hz, 6H), 1.23 (s, 3H). 13C NMR (151 MHz, CDCl3) δ 178.42, 166.05, 165.44, 159.66, 146.94, 145.85, 134.79, 127.06, 124.30, 124.06, 108.59, 62.71, 47.85, 44.87, 38.04, 37.07, 36.73, 33.58, 30.22, 29.36, 25.33, 24.26, 24.12, 24.09, 21.94, 18.69, 16.60. IR (KBr), ν/cm−1: 3441, 2922, 2362, 1724, 1649, 1544, 1462, 1246, 1172, 1128, 954, 848. HR-MS (m/z) (ESI): calcd for C27H38N2O3S [M + H]+: 471.2681, calcd 471.2681.
Hybrid compound 3e. Yellow solid, yield 59.7%, mp 137.5–138.8 °C; 1H NMR (600 MHz, CDCl3) δ 7.61 (d, J = 8.4 Hz, 2H), 7.36 (d, J = 8.4 Hz, 2H), 7.15 (d, J = 8.3 Hz, 1H), 7.00 (d, J = 8.2 Hz, 1H), 6.85 (s, 1H), 4.26 (s, 1H), 4.19 (s, 1H), 3.83 (t, J = 4.7 Hz, 1H), 3.20 (s, 2H), 2.97 (s, 1H), 2.90 (s, 1H), 2.81 (s, 3H), 2.27 (d, J = 12.9 Hz, 1H), 2.20 (s, 1H), 1.56 (s, 1H), 1.31 (s, 1H), 1.25 (s, 1H), 1.24 (s, 1H), 1.22 (s, 3H), 1.21 (d, J = 1.4 Hz, 6H), 1.17 (s, 3H). 13C NMR (151 MHz, CDCl3) δ 178.64, 170.77, 166.21, 162.68, 146.81, 145.90, 134.56, 134.50, 131.63, 130.15, 127.07, 126.07, 124.28, 124.10, 119.26, 90.19, 66.37, 61.61, 63.10, 47.76, 44.89, 38.03, 37.01, 36.74, 33.56, 30.22, 29.28, 25.26, 24.11, 21.93, 18.65, 16.59. IR (KBr), ν/cm−1: 3442, 2929, 2212, 1724, 1678, 1600, 1544, 1469, 1305, 1238, 1161, 1118, 1004, 844, 802. HR-MS (m/z) (ESI): calcd for C33H36FN3O3S [M + H]+: 574.2540, calcd 574.2534.
Hybrid compound 3f. Yellow solid, yield 72.8%, mp 83.5–84.9 °C; 1H NMR (600 MHz, CDCl3) δ 7.76 (s, 1H), 7.75 (s, 1H), 7.14 (d, J = 8.2 Hz, 1H), 6.99 (d, J = 8.0 Hz, 1H), 6.87 (t, J = 8.5 Hz, 2H), 6.83 (s, 1H), 4.20 (d, J = 40.8 Hz, 3H), 3.87 (s, 7H), 3.16 (s, 2H), 2.79 (s, 3H), 2.17 (d, J = 12.6 Hz, 1H), 1.21 (d, J = 6.9 Hz, 6H), 1.19 (s, 3H), 1.15 (s, 3H). 13C NMR (151 MHz, CDCl3) δ 178.74, 171.34, 166.41, 165.10, 163.56, 146.81, 145.91, 134.56, 131.97, 130.99, 130.93, 127.04, 124.27, 124.10, 119.29, 115.46, 115.32, 89.76, 63.17, 47.77, 44.90, 38.04, 37.00, 36.69, 33.56, 30.19, 29.08, 25.24, 24.08, 24.07, 21.89, 18.63, 16.52. IR (KBr), ν/cm−1: 3414, 2954, 2218, 1722, 1616, 1548, 1469, 1305, 1246, 1172, 1105, 1004, 802, 761. HR-MS (m/z) (ESI): calcd for C33H36FN3O3S [M + H]+: 574.2540, calcd 574.2534.
Hybrid compound 3g. Yellow solid, yield 78.9%, mp 81.2–83.9 °C; 1H NMR (600 MHz, CDCl3) δ 7.56 (s, 1H), 7.28 (s, 1H), 7.18 (s, 1H), 7.16 (d, J = 8.2 Hz, 1H), 7.02 (s, 1H), 6.91 (s, 1H), 6.86 (s, 1H), 4.26 (s, 1H), 4.21 (s, 1H), 3.83 (s, 1H), 3.33 (s, 1H), 2.90 (s, 1H), 2.86 (s, 1H), 2.84 (d, J = 6.9 Hz, 1H), 2.82 (s, 1H), 2.32 (s, 1H), 2.28 (d, J = 10.6 Hz, 1H), 1.80 (s, 1H), 1.74 (s, 2H), 1.61 (s, 1H), 1.31 (s, 1H), 1.25 (s, 3H), 1.23 (d, J = 3.9 Hz, 6H), 1.22 (s, 3H). 13C NMR (151 MHz, CDCl3) δ 179.28, 173.51, 173.44, 164.94, 158.76, 146.87, 145.81, 134.69, 131.00, 127.04, 125.09, 124.26, 124.02, 119.19, 116.01, 92.33, 63.58, 47.78, 44.84, 38.03, 36.99, 36.52, 33.57, 30.15, 28.74, 25.23, 24.10, 21.79, 18.62, 16.41. IR (KBr), ν/cm−1: 3412, 2594, 2216, 1724, 1616, 1537, 1463, 1244, 1172, 1124, 1035, 823. HR-MS (m/z) (ESI): calcd for C33H36BrN3O3S [M + H]+: 636.1719, calcd 636.1715.
Hybrid compound 3h. Yellow solid, yield 77.4%, mp 144.2–145.1 °C; 1H NMR (600 MHz, CDCl3) δ 8.04 (s, 1H), 7.46 (s, 1H), 7.17 (d, J = 8.2 Hz, 1H), 7.04 (d, J = 8.4 Hz, 1H), 7.02 (s, 1H), 7.00 (s, 1H), 6.88 (s, 1H), 4.35 (d, J = 23.2 Hz, 2H), 3.89 (s, 3H), 3.43 (s, 2H), 2.98 (s, 3H), 2.91 (s, 3H), 2.31 (s, 1H), 2.22 (d, J = 10.8 Hz, 1H), 1.75 (d, J = 9.7 Hz, 2H), 1.63 (s, 1H), 1.48 (s, 1H), 1.26 (s, 3H), 1.23 (s, 6H), 1.20 (s, 3H). 13C NMR (151 MHz, CDCl3) δ 178.48, 167.77, 165.98, 162.90, 157.12, 146.89, 145.86, 134.72, 132.62, 130.51, 127.07, 125.18, 124.26, 124.05, 120.75, 115.47, 111.66, 97.10, 62.53, 55.46, 47.84, 44.88, 38.03, 37.05, 36.75, 36.70, 33.57, 31.66, 25.28, 24.10, 21.92, 18.64, 16.59. IR (KBr), ν/cm−1: 3419, 2929, 2222, 1724, 1674, 1533, 1465, 1381, 1247, 1172, 1105, 1045, 756. HR-MS (m/z) (ESI): calcd for C34H39N3O4S [M + H]+: 586.2740, calcd 586.2735.
Hybrid compound 3i. Yellow solid, yield 65.5%, mp 101.8–102.7 °C; 1H NMR (600 MHz, CDCl3) δ 8.02 (s, 1H), 7.30 (d, J = 7.7 Hz, 1H), 7.24 (s, 1H), 7.14 (s, 1H), 7.10 (s, 1H), 6.97 (s, 1H), 6.82 (s, 1H), 4.18 (s, 2H), 3.63 (s, 3H), 3.27 (s, 3H), 3.13 (s, 2H), 2.79 (s, 3H), 2.25 (s, 1H), 2.14 (s, 1H), 1.68 (s, 2H), 1.54 (s, 1H), 1.30 (s, 1H), 1.20 (d, J = 6.9 Hz, 6H), 1.17 (s, 3H), 1.14 (s, 3H). 13C NMR (151 MHz, CDCl3) δ 178.81, 173.21, 172.35, 167.42, 162.74, 159.26, 146.86, 145.81, 137.68, 134.67, 129.22, 127.04, 124.23, 124.01, 120.99, 116.86, 113.42, 89.59, 63.58, 55.24, 47.71, 44.87, 38.04, 36.99, 36.61, 33.56, 31.56, 30.16, 25.23, 24.10, 21.85, 18.64, 16.51. IR (KBr), ν/cm−1: 3464, 2954, 2204, 1722, 1645, 1550, 1465, 1301, 1242, 1174, 1126, 1008, 785. HR-MS (m/z) (ESI): calcd for C34H39N3O4S [M + H]+: 586.2740, calcd 586.2734.
Hybrid compound 3j. Yellow solid, yield 52.9%, mp 133.8–134.5 °C; 1H NMR (600 MHz, CDCl3) δ 7.72 (d, J = 8.7 Hz, 2H), 7.12 (d, J = 8.3 Hz, 1H), 6.97 (d, J = 8.2 Hz, 1H), 6.82 (s, 1H), 6.67 (d, J = 8.5 Hz, 2H), 4.22 (s, 1H), 4.15 (s, 1H), 3.66 (s, 3H), 3.10 (s, 1H), 3.06 (s, 1H), 2.94 (s, 1H), 2.87 (s, 1H), 2.79 (d, J = 6.9 Hz, 1H), 2.77 (s, 1H), 2.23 (d, J = 12.1 Hz, 1H), 2.15 (d, J = 13.7 Hz, 1H), 1.66 (s, 1H), 1.65 (s, 1H), 1.59 (s, 1H), 1.52 (d, J = 7.9 Hz, 1H), 1.41 (s, 1H), 1.25 (s, 1H), 1.21 (s, 3H), 1.19 (s, 3H), 1.15 (s, 3H), 1.12 (s, 3H). 13C NMR (151 MHz, CDCl3) δ 178.94, 174.75, 166.92, 162.77, 161.51, 146.86, 145.78, 134.69, 130.30, 128.79, 127.05, 124.23, 123.97, 113.51, 88.02, 63.66, 55.20, 47.71, 44.89, 38.06, 36.99, 36.61, 33.55, 31.56, 30.20, 28.81, 25.23, 24.08, 21.83, 18.64, 16.45. IR (KBr), ν/cm−1: 3414, 2931, 2204, 1722, 1612, 1539, 1471, 1313, 1253, 1178, 1122, 804. HR-MS (m/z) (ESI): calcd for C34H39N3O4S [M + H]+: 586.2740, calcd 586.2731.
Hybrid compound 3k. Yellow solid, yield 82.6%, mp 92.6–93.8 °C; 1H NMR (600 MHz, CDCl3) δ 8.06 (s, 1H), 7.94 (s, 1H), 7.77 (s, 1H), 7.34 (s, 1H), 7.18 (s, 1H), 7.01 (s, 1H), 6.88 (s, 1H), 4.55 (s, 1H), 4.43 (s, 1H), 3.49 (s, 1H), 3.43 (s, 1H), 2.99 (s, 2H), 2.92 (s, 2H), 2.88 (s, 1H), 2.87 (d, J = 4.4 Hz, 1H), 2.83 (s, 1H), 2.32 (d, J = 12.3 Hz, 1H), 2.24 (d, J = 12.6 Hz, 1H), 1.87 (dd, J = 15.3, 5.8 Hz, 1H), 1.79 (s, 2H), 1.68 (s, 1H), 1.31 (s, 3H), 1.23 (s, 3H), 1.22 (s, 6H). 13C NMR (151 MHz, CDCl3) δ 179.48, 167.26, 163.16, 157.05, 146.72, 145.98, 135.46, 134.52, 130.14, 127.07, 124.27, 124.17, 120.80, 120.18, 116.79, 93.31, 62.45, 48.06, 45.01, 37.99, 37.06, 36.85, 36.69, 33.57, 31.79, 30.11, 29.35, 25.27, 24.09, 21.94, 18.62, 16.64. IR (KBr), ν/cm−1: 3441, 2954, 1761, 1606, 1539, 1328, 1224, 1166, 1033, 817, 767. HR-MS (m/z) (ESI): calcd for C33H37N3O4S [M + H]+: 572.2583, calcd 572.2574.
Hybrid compound 3l. Yellow solid, yield 51.0%, mp 88.4–89.3 °C; 1H NMR (600 MHz, CDCl3) δ 8.38 (s, 1H), 8.06 (s, 1H), 7.65 (s, 1H), 7.33 (s, 1H), 7.16 (s, 1H), 7.02 (s, 1H), 6.88 (s, 1H), 4.46 (d, J = 26.5 Hz, 3H), 3.57 (s, 3H), 2.98 (s, 2H), 2.28 (dd, J = 21.1, 12.5 Hz, 3H), 1.78 (s, 3H), 1.69 (d, J = 16.3 Hz, 3H), 1.29 (s, 3H), 1.23 (s, 3H), 1.22 (s, 3H), 1.21 (s, 3H). 13C NMR (151 MHz, CDCl3) δ 178.40, 162.95, 160.10, 154.46, 146.85, 145.88, 134.93, 134.65, 127.06, 126.21, 125.19, 124.25, 124.11, 117.36 62.49, 61.46, 47.88, 44.93, 38.02, 37.05, 36.79, 36.72, 33.54, 31.67, 30.24, 30.08, 25.31, 24.09, 24.07, 21.99, 18.68, 16.62. IR (KBr), ν/cm−1: 3441, 2929, 2368, 1724, 1606, 1539, 1463, 1323, 1269, 1126, 1008, 819, 765. HR-MS (m/z) (ESI): calcd for C33H37N3O4S [M + H]+: 572.2583, calcd 572.2555.
Hybrid compound 3m. Red solid, yield 67.8%, mp 71.8–72.7 °C; 1H NMR (600 MHz, CDCl3) δ 8.16 (s, 1H), 7.98 (d, J = 8.9 Hz, 2H), 7.16 (d, J = 8.2 Hz, 2H), 7.00 (s, 1H), 6.86 (s, 1H), 4.39 (d, J = 7.1 Hz, 3H), 2.97 (s, 1H), 2.90 (s, 2H), 2.28 (dd, J = 29.3, 13.1 Hz, 3H), 1.41 (t, J = 7.1 Hz, 4H), 1.31 (s, 1H), 1.29 (s, 3H), 1.24 (s, 3H), 1.23 (s, 6H), 1.21 (s, 3H). 13C NMR (151 MHz, CDCl3) δ 178.55, 163.18, 162.77, 154.39, 146.91, 145.91, 134.69, 133.73, 127.02, 124.86, 124.31, 124.09, 116.25, 115.38, 99.84, 66.39, 66.31, 47.88, 44.85, 38.03, 37.04, 36.65, 33.57, 30.17, 25.26, 24.10, 21.89, 18.67, 16.61. IR (KBr), ν/cm−1: 3446, 2931, 2220, 1724, 1645, 1591, 1510, 1458, 1263, 1174, 831. HR-MS (m/z) (ESI): calcd for C33H37N3O4S [M + H]+: 572.2583, calcd 572.2575.
Hybrid compound 3n. Yellow solid, yield 85.3%, mp 90.7–91.5 °C; 1H NMR (600 MHz, CDCl3) δ 8.71 (s, 1H), 7.52 (s, 1H), 7.18 (s, 1H), 7.15 (d, J = 8.3 Hz, 1H), 7.03 (d, J = 8.1 Hz, 1H), 7.01 (s, 1H), 6.84 (s, 1H), 4.34 (s, 1H), 4.28 (s, 1H), 3.83 (s, 1H), 3.38 (s, 1H), 2.91 (s, 1H), 2.83 (s, 1H), 2.82 (s, 1H), 2.81 (s, 1H), 2.28 (d, J = 12.5 Hz, 1H), 2.19 (d, J = 13.1 Hz, 1H), 1.72 (s, 1H), 1.70 (s, 1H), 1.60 (d, J = 8.6 Hz, 1H), 1.31 (s, 1H), 1.25 (s, 2H), 1.24 (s, 6H), 1.22 (s, 3H), 1.21 (s, 3H). 13C NMR (151 MHz, CDCl3) δ 178.58, 165.23, 148.16, 146.93, 146.80, 145.91, 136.85, 134.72, 134.54 129.83, 127.07, 127.03, 125.98, 124.30, 124.12, 123.80, 66.39, 62.54, 61.63, 47.84, 44.88, 38.08, 38.00, 37.01, 33.58, 33.55, 29.73, 25.24, 24.10, 21.9, 18.69, 16.55. IR (KBr), ν/cm−1: 3412, 2954, 2218, 1724, 1616, 1533, 1465, 1348, 1244, 1172, 1120, 823. HR-MS (m/z) (ESI): calcd for C33H36N4O5S [M + H]+: 601.2485, calcd 601.2471.
Hybrid compound 3o. Yellow solid, yield 78.6%, mp 106.3–108.7 °C; 1H NMR (600 MHz, CDCl3) δ 7.74 (s, 2H), 7.14 (s, 1H), 7.07 (s, 2H), 7.00 (s, 1H), 6.84 (s, 1H), 4.60 (s, 1H), 4.26 (d, J = 36.2 Hz, 2H), 3.25 (s, 2H), 2.96 (s, 1H), 2.89 (s, 1H), 2.80 (s, 3H), 2.43 (s, 1H), 2.31 (s, 3H), 2.18 (s, 1H), 1.70 (s, 2H), 1.21 (s, 3H), 1.20 (s, 6H), 1.16 (s, 3H). 13C NMR (151 MHz, CDCl3) δ 178.75, 167.78, 159.28, 146.88, 145.81, 143.17, 141.57, 134.70, 133.12 (C-9), 129.51 (C-34, C-36), 128.80 (C-11), 127.05 (C-33, C-37), 124.25, 124.02, 115.42, 63.18, 49.39, 47.77, 44.90, 38.04, 37.02, 36.67, 33.56, 31.63, 29.22, 25.27, 24.10, 24.08, 21.60, 18.65, 16.52. IR (KBr), ν/cm−1: 3441, 2927, 2208, 1722, 1653, 1544, 1462, 1371, 1296, 1244, 1178, 1002, 800, 727. HR-MS (m/z) (ESI): calcd for C33H36N4O5S [M + H]+: 572.2583, calcd 572.2582.
Cells culture
Cells. Human liver cancer (HepG2), human breast cancer (MCF-7) cells, human colon cancer (HCT-116) cells, human lung cancer (A549) cells and human normal liver cells (LO2) were provided by the Institute of Biochemistry and Cell Biology, China Academy of Sciences. All cell lines were seeded into 70 mm Cell and Tissue Culture Dishes in DMEM (Dulbecco's Modified Eagle Medium) medium with 10% FBS (fetal bovine serum), 100 mg mL−1 of streptomycin and 100 units per mL of penicillin, and then incubated at 37 °C with a humidified atmosphere of 5% CO2.
In vitro cytotoxicity
The in vitro cytotoxic potency of the compounds 3a–3o was evaluated by 3-(4,5-dimethylthiazol-2-yl)-2,5-diphenyltetrazolium bromide (MTT) assay with 5-FU as the positive control. After counting the number of cells, the cells were seeded in 96-well plates (180 μL per well at a density of 5 × 104 cells per mL) with DMEM (Dulbecco's Modified Eagle Medium) medium and then incubated for 24 h at 37 °C in 5% CO2. The cells were treated with various concentrations of the synthesized compounds and positive control 5-FU, and then the plates were incubated for 48 h at 37 °C with 5% CO2. Next, 20 μL solution of MTT (5 mg mL−1) was added to each well. After incubation for 4 h at 37 °C, the supernatants were carefully removed, and formazan precipitates were dissolved in 150 μL of dimethyl sulfoxide (DMSO). Plates were then shaken (300 rpm) for 10 min. Finally, the absorbance was measured at 490 nm by an enzyme labeling instrument (ELx800). The final IC50 values were calculated by the Bliss method (n = 5). All the tests were repeated in at least three independent experiments.
Hoechst 33258 staining assay
MCF-7 cells were seeded on a sterile cover slip in six-well plates and treated with compound 3b (0 μM, 5 μM, 10 μM, 15 μM) for 48 h. The culture medium containing compounds was removed, and the cells were fixed in 4% paraformaldehyde for 10 min. Then the cells were washed with PBS and stained with Hoechst 33258 at 37 °C for 5 min in darkness. After being washed with PBS, the cells were observed with the OLYMPUS 1X73 fluorescence microscope using 350 nm for excitation and 460 nm for emission.
Cell apoptosis assay
At the density of 2 × 105 cells per mL of the DMEM medium with 10% FBS on 6-well plates to the final volume of 2 mL. The plates were incubated for overnight and then treated with different concentrations compound 3b for 48 h. After 48 h, the cells were collected and washed twice with PBS and then resuspend cells in 100 μL 1× binding buffer. The cells were subjected to 5 μL of FITC Annexin V and 5 μL propidium iodide (PI) staining using annexin-V FITC apoptosis kit (BD, Pharmingen) and incubate for 30 min at RT (25 °C) in the dark. Then, 100 μL 1× binding buffer were added. The apoptosis ratio was quantified by a flow cytometer (Becton-Dickinson Accuri C6) and data were analysed using the FlowJo software.
Cell cycle assay
The MCF-7 cells line were treated with different concentrations of compound 3b. After 48 h of incubation, cells were washed twice with ice-cold PBS, fixed and permeabilized with ice-cold 70% ethanol at 4 °C overnight. The cells were treated with 100 μg mL−1 RNase A at 37 °C for 30 min, after washed with ice-cold PBS, and finally stained with 1 mg mL−1 propidium iodide (PI) (BD, Pharmingen) in the dark at 4 °C for 30 min. The samples were analysed by a flow cytometer (Becton-Dickinson Accuri C6) and data were analysed using the ModFitLT software.
Conclusions
In summary, a series of novel dehydroabietic acid derivatives bearing pyrimidine moiety were designed and synthesized via two steps, and their cytotoxic activity against four cancer cells (HepG2, MCF-7, HCT-116, and A549) were evaluated by using MTT assay. The results showed that most compounds inhibited tested human cancer cell lines were better than parent compound and the commercial anticancer drug 5-FU. It's remarkable that the most effective compound 3b inhibited HepG2, MCF-7, HCT-116 and A549 cell lines with IC50 values of 10.42 μM, 7.00 μM, 9.53 μM, 11.93 μM, respectively, which were more than 2 times higher than 5-FU. We also found that compound 3b could induce cell apoptosis of MCF-7 cells, which may attribute to cell cycle arrest in S phase. Taken together, these results emphasize the potential of this series of derivatives as new antitumor drugs, further studies focusing on the derivatives are still in progress.
Conflicts of interest
There are no conflicts to declare.
Acknowledgements
This work was supported by the Guangxi Natural Science Foundation of China (2018GXNSFAA138165, 2018GXNSFAA281200), the State Key Laboratory for Chemistry and Molecular Engineering of Medicinal Resources (Guangxi Normal University) (CMEMR2019-B02), the Open Fund of Guangxi Key Laboratory of Chemistry and Engineering of forest Products (GXFC18-02), the Open Fund of Guangxi Key Laboratory of Agricultural Resources Chemistry and Biotechnology, the Cultivating Project of a Thousand of Young and Middle-aged Key Teachers in Guangxi Colleges and Universities, the Youth Promotion Project Fund of Guangxi (2019KY0551), and the Project to Improve the Basic Research Ability of Middle and Young Teachers in Guilin Medical University (2018glmcy015), the China University Students Innovative Project (201910601033).
References
- S. Dadashpour and S. Emami, Eur. J. Med. Chem., 2018, 150, 9–29 CrossRef CAS PubMed.
- W. H. Qu, Q. J. Yang, G. C. Wang, Z. H. Wang, P. Huang, W. Huang, R. Zhang and D. Y. Yan, RSC Adv., 2020, 10, 8958–8966 RSC.
- C. Ma, Y. Wang, F. Dong, Z. Wang, Y. Zhao, Y. Shan and S. Wang, Chem. Biol. Drug Des., 2019, 94, 1457–1466 CAS.
- N. Kerru, P. Singh, N. Koorbanally, R. Raj and V. Kumar, Eur. J. Med. Chem., 2017, 142, 179–212 CrossRef CAS PubMed.
- B. B. Mishra and V. K. Tiwari, Eur. J. Med. Chem., 2011, 46, 4769–4807 CrossRef CAS PubMed.
- S. Mondal, S. Bandyopadhyay, M. K. Ghosh, S. Mukhopadhyay, S. Roy and C. Mandal, Anti-Cancer Agents Med. Chem., 2012, 12, 49–75 CrossRef CAS PubMed.
- D. J. Newman, G. M. Cragg and K. M. Snader, J. Nat. Prod., 2003, 66, 1022–1037 CrossRef CAS PubMed.
- X. C. Huang, R. Z. Huang, Z. X. Liao, Y. M. Pan, S. H. Gou and H. S. Wang, Eur. J. Med. Chem., 2016, 108, 381–391 CrossRef CAS PubMed.
- M. Berger, A. Roller and N. Maulide, Eur. J. Med. Chem., 2017, 126, 937–943 CrossRef CAS PubMed.
- W. M. Zhang, T. Yang, X. Y. Pan, X. L. Liu, H. X. Lin, Z. B. Gao, C. G. Yang and Y. M. Cui, Eur. J. Med. Chem., 2017, 127, 917–927 CrossRef CAS PubMed.
- N. Y. Chen, W. G. Duan, G. S. Lin, L. Z. Liu, R. Zhang and D. P. Li, Mol. Diversity, 2016, 20, 897–905 CrossRef CAS PubMed.
- B. Zapata, M. Rojas, L. Betancur-Galvis, A. C. Mesa-Arango, D. Pérez-Guaita and M. A. González, MedChemComm, 2013, 4, 1239–1246 RSC.
- J. Kim, Y. G. Kang, J. Y. Lee, D. H. Choi, Y. U. Cho, J. M. Shin, J. S. Park, J. H. Lee, W. G. Kim, D. B. Seo, T. R. Lee, Y. Miyamoto and K. T. No, Mol. Cell. Endocrinol., 2015, 412, 216–225 CrossRef CAS PubMed.
- M. S. Kang, S. Hirai, T. Goto, K. Kuroyanagi, J. Y. Lee, T. Uemura, Y. Ezaki, N. Takahashi and T. Kawada, Biochem. Biophys. Res. Commun., 2008, 369, 333–338 CrossRef CAS PubMed.
- T. Tashima, Y. Toriumi, Y. Mochizuki, T. Nonomura, S. Nagaoka, K. Furukawa and H. Tsuru, Bioorg. Med. Chem., 2006, 14, 8014–8031 CrossRef CAS PubMed.
- W. G. Duan, X. R. Li, Q. J. Mo, J. X. Huang, B. Cen, X. T. Xu and F. H. Lei, Holzforschung, 2011, 65, 191–197 CAS.
- W. Gu, T. T. Miao, D. W. Hua, X. Y. Jin, X. B. Tao, C. B. Huang and S. F. Wang, Bioorg. Med. Chem. Lett., 2017, 27, 1296–1300 CrossRef CAS PubMed.
- H. Xu, L. Liu, X. Fan, G. Zhang, Y. Li and B. Jiang, Bioorg. Med. Chem.
Lett., 2017, 27, 505–510 CrossRef CAS PubMed.
- P. C. Diao, Q. Li, M. J. Hu, Y. F. Ma, W. W. You, K. H. Hong and P. L. Zhao, Eur. J. Med. Chem., 2017, 134, 110–118 CrossRef CAS PubMed.
- T. Gregorić, M. Sedić, P. Grbčić, A. Tomljenović Paravić, S. Kraljević Pavelić, M. Cetina, R. Vianello and S. Raić-Malić, Eur. J. Med. Chem., 2017, 125, 1247–1267 CrossRef PubMed.
- K. Jin, H. Yin, E. De Clercq, C. Pannecouque, G. Meng and F. Chen, Eur. J. Med. Chem., 2018, 145, 726–734 CrossRef CAS PubMed.
- T. G. Kraljević, M. Klika, M. Kralj, I. Martin-Kleiner, S. Jurmanović, A. Milić, J. Padovan and S. Raić-Malić, Bioorg. Med. Chem. Lett., 2012, 22, 308–312 CrossRef PubMed.
- M. M. Sekhar, U. Nagarjuna, V. Padmavathi, A. Padmaja, N. V. Reddy and T. Vijaya, Eur. J. Med. Chem., 2018, 145, 1–10 CrossRef PubMed.
- M. Saleeb, C. Sundin, Ö. Aglar, A. F. Pinto, M. Ebrahimi, Å. Forsberg, H. Schüler and M. Elofsson, Eur. J. Med. Chem., 2018, 143, 568–576 CrossRef CAS PubMed.
- R. M. Borik, N. M. Fawzy, S. M. Abu-Bakr and M. S. Aly, Molecules, 2018, 23, 1398 CrossRef PubMed.
- K. Ohmoto, M. Okuma, T. Yamamoto, H. Kijima, T. Sekioka, K. Kitagawa, S. Yamamoto, K. Tanaka, K. Kawabata, A. Sakata, H. Imawaka, H. Nakai and M. Toda, J. Med. Chem., 2000, 43, 4927–4929 CrossRef CAS PubMed.
- M. A. Abdelgawad, R. B. Bakr and A. A. Azouz, Bioorg. Chem., 2018, 77, 339–348 CrossRef CAS PubMed.
- P. X. Liu, Y. Yang, Y. X. Tang, T. Yang, Z. T. Sang, Z. Y. Liu, T. Y. Zhang and Y. F. Luo, Eur. J. Med. Chem., 2019, 163, 169–182 CrossRef CAS PubMed.
- K. J. Li, R. Y. Qu, Y. C. Liu, J. F. Yang, P. Devendar, Q. Chen, C. W. Niu, Z. Xi and G. F. Yang, J. Agric. Food Chem., 2018, 66, 3773–3782 CrossRef CAS PubMed.
- X. H. Liu, Q. Wang, Z. H. Sun, D. E. Wedge, J. J. Becnel, A. S. Estep, C. X. Tan and J. Q. Weng, Pest Manage. Sci., 2017, 73, 953–959 CrossRef CAS PubMed.
- D. Yao, Y. Zhou, L. Zhu, L. Ouyang, J. Zhang, Y. Jiang, Y. Zhao, D. Sun, S. Yang, Y. Yu and J. Wang, Eur. J. Med. Chem., 2017, 140, 155–171 CrossRef CAS PubMed.
- I. H. Eissa, A. M. El-Naggar and M. A. El-Hashash, Bioorg. Chem., 2016, 67, 43–56 CrossRef CAS PubMed.
- A. Thiriveedhi, R. V. Nadh, N. Srinivasu, Y. Bobde, B. Ghosh and K. V. G. C. Sekhar, Toxicol. In Vitro, 2019, 60, 87–96 CrossRef CAS PubMed.
- X. Wang, F. H. Pang, L. Huang, X. P. Yang, X. L. Ma, C. N. Jiang, F. Y. Li and F. H. Lei, Int. J. Mol. Sci., 2018, 19(10), 3116 CrossRef PubMed.
- F. Y. Li, X. Wang, W. G. Duan and G. S. Lin, Molecules, 2017, 22, 1087 CrossRef PubMed.
- F. Y. Li, L. Huang, Q. Li, X. Wang, X. L. Ma, C. N. Jiang, W. G. Duan and F. H. Lei, Molecules, 2019, 24, 4191 CrossRef CAS PubMed.
Footnotes |
† Electronic supplementary information (ESI) available. See DOI: 10.1039/d0ra02432e |
‡ Lin Huang and Rong Huang have equally contributed as a first author. |
|
This journal is © The Royal Society of Chemistry 2020 |
Click here to see how this site uses Cookies. View our privacy policy here.