DOI:
10.1039/D0RA03350B
(Paper)
RSC Adv., 2020,
10, 32020-32026
Assessment of a developed HPLC-MS/MS approach for determining plasma eupatorin in rats and its application in pharmacokinetics analysis
Received
15th April 2020
, Accepted 23rd August 2020
First published on 28th August 2020
Abstract
Eupatorin, a bioactive compound extracted from Java tea (Orthosiphon stamineus), possesses potent anti-cancer, anti-inflammatory and vasodilation activities. To date, no pharmacokinetics studies on eupatorin have yet been performed. Here, we established and validated a sensitive and selective LC-MS/MS (liquid chromatography-tandem mass spectrometry) approach for determining plasma eupatorin in rats. Chromatographic fractionation was conducted on a Wonda Cract ODS-2 C18 Column (4.6 mm × 150 mm, 5 μm) with a mobile phase containing aqueous 0.1% formic acid and acetonitrile using a flow rate of 0.8 ml min−1. In multiple reaction monitoring mode, precursor-to-product ion transitions for quantification of eupatorin and the internal standard were set at 343.1 → 328.1 and 252.0 → 155.9, respectively. The intra- and inter-day precision and accuracy were found to be below 6.72% and within ±8.26% in rat plasma, respectively. Meanwhile, all values of the matrix effect, recovery and stability were within the accepted ranges. Furthermore, we carried out the pharmacokinetic analysis using the developed method. The pharmacokinetic study revealed that while the Cmax (maximum plasma concentration) of eupatorin and time for reaching the Cmax (Tmax) were 974.886 ± 293.898 μg L−1 and 0.25 h, respectively, the half-life was 0.353 ± 0.026 h. This study will be of great significance to the research on the pharmacology, clinical pharmacy and drug action mechanism of eupatorin.
1. Introduction
Breast cancer is among the most common female malignancies in the world, as well as a leading cause of mortality in women.1,2 In recent years, flavonoids have been shown to possess a wide variety of anti-cancer effects.3 Therefore, the intake of certain flavonoid-containing food could contribute to prevention of the cancer development at an early stage in the patients. As a potential anti-tumor agent against breast cancer, eupatorin (5,3′-di-hydroxy-6,7,4′-tri-methoxy-flavone) exhibited inhibitory effects on the invasion and migration of MDA-MB-231 and MCF-7 cells via suppressed Akt pathway as well as cell cycle blockade.4,5 Eupatorin has been identified as a natural methoxy flavonoid that widely exists in Java tea (Orthosiphon stamineus, OS), the traditional Chinese medicinal herb commonly used as a diuretic as well as for renal system diseases in Southeast Asia and Europe.6–8 Multiple studies have shown that, in addition to anti-breast cancer effect, eupatorin has the effect on inhibiting proliferation and inducing apoptosis in other types of cancer cells.9–11 Moreover, the pharmacological study found that eupatorin possesses strong anti-inflammatory12,13 and vasodilation activities.14 Thus, it is of great significance to study this compound in depth due to its potential application prospect and clinical value.
Pharmacokinetics is the subject of studying the drug changes in vivo, which has important implications for the studies of pharmacology, clinical medicine and elucidation of the mechanism of drug action. Meanwhile, the pharmacokinetic study plays an important role in evaluating the concentration–effect relationship, designing new drug delivery systems and optimizing drug delivery scheme.15 Although the pharmacokinetic analysis of OS has been reported,16 there is no study on the pharmacokinetics of eupatorin. Here, we aimed to undertake a pharmacokinetics analysis of eupatorin in rats and to elucidate its changes and rules under the influence of organism.
High Performance Liquid Chromatography (HPLC)17–19 and ultra-performance liquid chromatography–triple quadrupole mass spectrometry (UPLC-QQQ/MS)20 have been successfully utilized to determine eupatorin. LC-MS/MS approach is known to be widely used in drug analysis due to its high selectivity and sensitivity.21 In the present work, we employed the LC-MS/MS approach for determining plasma eupatorin in rats, while conducting a pharmacokinetics study using this method. The experimental results will be helpful to better understand the pharmacokinetic law of eupatorin in rats, thereby laying a basis for further pharmacological and clinical research.
2. Materials and methods
2.1. Reagents and compounds
The following compounds and reagents were purchased as follows: eupatorin with a purity of more than 98.94% (855-96-9) from Chengdu Desite Co., Ltd (Chengdu, China); HPLC-grade acetonitrile, methanol and formic acid from Fisher Scientific (Waltham, MA, USA); Purified water from Wahaha Group Co., Ltd (Hangzhou, China); CMC–Na (sodium carboxymethyl cellulose) from Guangfu Technology Development Co., Ltd. (Tianjin, China. Sulfamethoxazole was obtained from the National Institutes for Food and Drug Control, Beijing, China).
2.2. Experimental apparatus and parameters
An Agilent Technologies Series 1200 system with an Applied Biosystems/MDS SCIEX 3200 QTRAPTM system were used to conduct the experiments. Chromatographic fractionation was performed on a Wonda Cract ODS-2 C18 column (4.6 mm × 150 mm, 5 μm) using a mobile phase comprising aqueous 0.1% formic acid (solvent A) and acetonitrile (solvent B). The flow rate and injection volume were set at 0.8 ml min−1 and 10 μl, respectively. The following gradient elution was carried out: 0–3 min, eluent B 10–61%; 3–10 min, eluent B 61–85%; and 10–13 min, eluent B 85–95%.
A QTRAP-3200 system including Turbo V sources and turbo-ion spray interface in multiple reaction monitoring mode was utilized to conduct the mass spectrometry. The parameters for the spectrometric measurements were set as follows: curtain gas 35 psi, nebulizer gas 60 psi, heater gas 65 psi, temperature for the turbo gas 650 °C, and ion spray voltage −4500 V. The precursor/product ion pairs of eupatorin and sulfamethoxazole were 343.1→328.1 and 252.0→155.9, respectively. The following software systems were used: Analyst 1.6.2 software (AB SCEIX, USA) for data acquisition; Peakview 2.0 software (AB SCEIX, USA) and MultiQuant 3.0 software (AB SCEIX, USA) for data processing.
2.3. Preparation of standard solutions and quality control sample solutions
0.16 mg ml−1 of eupatorin was prepared with methanol as the stock solution. Then, a serial dilution using methanol was made to prepare standard working solutions at 3.125, 6.25, 12.5, 25, 50, 100, 200, 400, and 800 ng ml−1 as well as quality control (QC) sample solutions, including low 6.25 ng ml−1, medium 50 ng ml−1, and high quality control 600 ng ml−1. Preparation of the internal standard (IS, sulfamethoxazole) working solution (400 ng ml−1) with methanol was performed. All solutions were kept in the refrigerator.
2.4. Sample preparation
Extraction of eupatorin or IS from the blank plasma via protein precipitation was carried out using methanol. 100 μl of IS solution and 300 μl of methanol were spiked to 100 μl of the blank plasma. After being vortexed for 3 min, the mixture was subjected to a 21,380g centrifugation for 10 min at 4 °C. The collected supernatant was evaporated by nitrogen gas and re-dissolved into 100 μl of methano l. The sample was purified by twice centrifugation (21,380g) for 10 min, and a 10 μl of the supernatant was loaded on the LC-MS/MS for analysis.22
2.5. Method validation for pharmacokinetics
The suitability of the developed method used for this investigation was evaluated under the guidelines for bioanalytical method validation issued by US Food and Drug Administration.23
2.5.1. Specificity and selectivity. The blank plasma, blank plasma mixed with eupatorin and IS, as well as plasma samples following oral medication of eupatorin were processed and analyzed to determine specificity and selectivity of the developed method.24
2.5.2. Linearity and sensitivity. 100 μl of IS working solution, 100 μl of above standard solutions and 200 μl of methanol were spiked to 100 μl of the blank plasma. The mixture was vortexed for 3 min, followed by a 21,380g centrifugation for 10 min. After being dried by nitrogen gas, the collected supernatant was re-dissolved into 100 μl of methanol. The sample was purified by twice centrifugation (21,380g) for 10 min, and a 10 μl of the supernatant was loaded on the LC-MS/MS for analysis. The data was processed using MultiQuant 3.0 software to obtain the standard curve of the target compound. In the standard curve, LLOQ (lower limit of quantification) indicates the lowest analyte concentration that can be accurately quantified.25
2.5.3. Precision and accuracy. Six replicates of QC samples at three different concentrations (low, medium and high) were assayed to determine intra- and inter-day precision and accuracy that were indicated by the relative standard deviation (RSD) and relative error (RE), respectively.26 And RSD was expected to be below 15%, while the RE should be within ±15%.27
2.5.4. Recovery and matrix effect. ① 100 μl of each QC sample solution at low, medium, and high concentrations, 200 μl of methanol and 100 μl of IS working solution were spiked to 100 μl of the blank plasma. QC samples were prepared according to the above protocol. Six replicates of each concentration were prepared in parallel.② 200 μl of methanol and 100 μl of IS working solution were spiked to 100 μl of the blank plasma. The mixture was processed according to the method above, then 100 μl of each QC sample solution at three different concentrations was added. For each concentration, six replicates were prepared in parallel.
③ 200 μl of methanol and 100 μl of IS working solution were spiked to 100 μl of water. The mixture was processed according to the method above, followed by the addition of 100 μl of each QC sample solution at the three concentration levels. Each concentration was prepared for six replicates in parallel.
The calculation formula of recovery rate was presented as: recovery rate = ①/② × 100%.28
The calculation formula of matrix effect was presented as: matrix effect = ②/③ × 100%.29
2.5.5. Stability. The analyte stability in rat plasma at the three QC concentration levels was evaluated under indicated conditions below (n = 6). For testing the short-term and long-term stabilities, the samples were kept at 25 °C for 24 h and −20 °C for one month before analysis, respectively. To assay the freeze–thaw stability, samples were subjected to three freeze–thaw cycles prior to test. For evaluating the stock solutions stability, samples were kept at 4 °C for one month prior to study. RE was used to define the stability, and the analyte was considered stable if the RE was within ± 15%.30
2.6. Pharmacokinetics analysis
Eighteen male SD rats (200–250 g, 12–14 weeks old) were obtained from the Laboratory Animal Research Center of the Hebei Medical University in China. All experimental procedures involving animals were performed under the guidelines for Care and Use of Laboratory Animals issued by the Hebei Committee, and protocols were approved by Ethics Committee of the Hebei Medical University. Animals were reared at the temperature ranging 22–25 °C and relative humidity of 55–60% under a 12 h light–dark cycle, and were freely fed for at least one week before the experiments. For this study, rats were subjected to a 12 h of fasting with free access to water before the experiment. The rats were administered via gavage with eupatorin in a 0.5% CMC–Na solution (50 mg kg−1).
For plasma preparation, a single blood sample (0.3 ml for each time point) was collected from the canthus of rat eyes at 0, 0.033, 0.083, 0.117, 0.167, 0.25, 0.5, 1, 2, 3, 4, 5 and 7 h after administration, respectively, followed by an immediate centrifugation at 1920 × g for 10 min. After centrifugation, these samples were mixed and stored at −80 °C. The Drug and Statistics 3.0 software program31–33 (DAS 3.0, http://www.drugchina.net) was used to calculate pharmacokinetic parameters of eupatorin in the non-compartmental model.
3. Results and discussion
3.1. Optimization of chromatographic and mass spectrometry conditions
It has been demonstrated that chromatographic conditions, especially the mobile phase composition, are critical for obtaining satisfactory chromatographic performance such as peak symmetry, sensitivity, and short run time, as well as proper ionization.34,35 In this study, we made a comparison between acetonitrile–water and methanol–water mobile phase systems, and found that acetonitrile–water system was superior to methanol–water counterpart. Strikingly, the addition of 0.1% formic acid into water of the mobile phase could not only improve chromatographic peak shape, but also increase the analyte sensitivity. The mass spectrometry conditions were also optimized in the study. The response values of the analyte and IS in negative ion mode were higher than those in positive ion mode. In addition, parameters such as declustering potential (DP) and collision energy (CE) were optimized to achieve the best responses of the analyte and IS. The selected precursor-product ion pairs of eupatorin and IS were 343.1/328.1 and 252.0/155.9, respectively. The chemical structures, MS/MS spectra, DP and CE of eupatorin and IS were presented in Fig. 1.
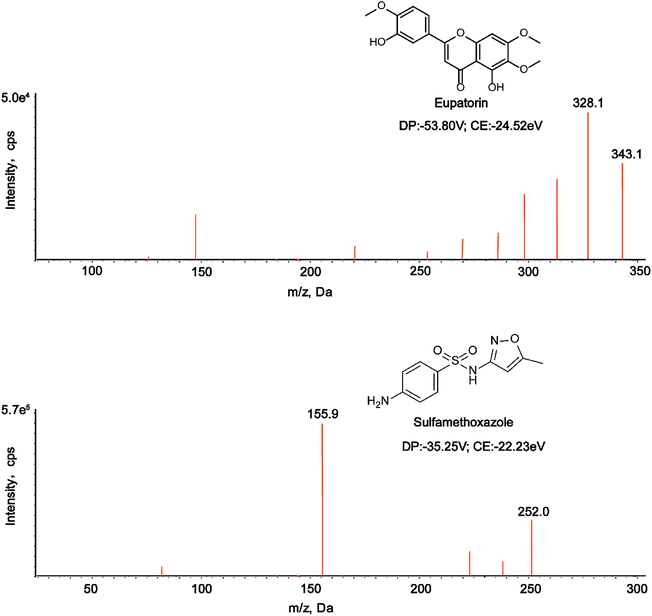 |
| Fig. 1 The chemical structures, MS/MS spectra, DP and CE of eupatorin and sulfamethoxazole in negative mode. | |
3.2. Method validation
3.2.1. Specificity and selectivity. The typical chromatograms of the blank plasma, blank plasma mixed with eupatorin and IS, as well as plasma samples following oral medication of eupatorin were depicted in Fig. 2. The retention times of eupatorin and internal standard were 5.40 min and 4.55 min, respectively, showing no marked endogenous interference on the blank sample during the retention time of eupatorin and internal standard.
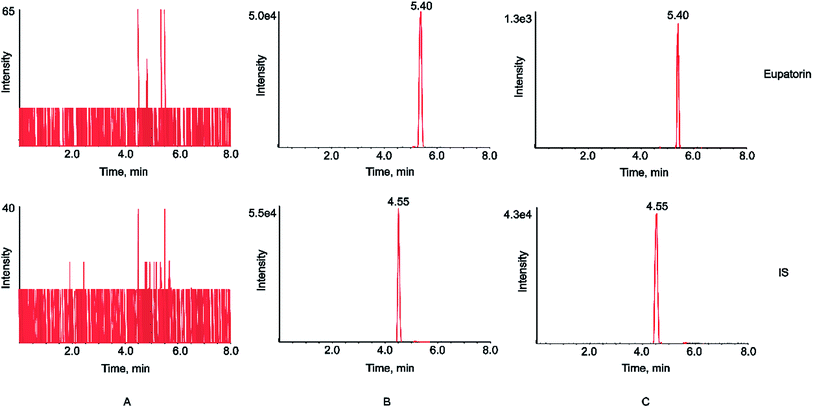 |
| Fig. 2 The MRM chromatograms of rat blank plasma (A), plasma mixed with eupatorin and sulfamethoxazole (B), and plasma samples following oral medication of eupatorin (C). | |
3.2.2. Linearity and sensitivity. Upon data acquisition of a number of standard samples, MultiQuant 3.0 software was used to generate the linear regression curve and calculate the correlation coefficient of the analyte. The regression eupatorin was y = 0.004x − 0.0089 (r = 0.9907), while the linear range and lower limit of quantification were 3.125–1600 ng ml−1 and 3.125 ng ml−1, respectively. These results showed a good linear correlation between the concentration and response value of eupatorin.
3.2.3. Precision and accuracy. Results of intra- and inter-day precision and accuracy of eupatorin were summarized in Table 1. As shown in the table, the intra- and inter-day RSDs were ≤6.72% and 5.97%, respectively, while the corresponding REs were within ±6.76% and ±8.26%, respectively. This study validated a precise, reliable and reproducible method for determining plasma eupatorin in rats.
Table 1 Intra- and inter-day precision and accuracy of plasma eupatorin in rats
Conc. (ng ml−1) |
Intra-day (n = 6) |
Inter-day (n = 6) |
Measured conc. (ng ml−1) |
Precision, RSD (%) |
Accuracy, RE (%) |
Measured conc. (ng ml−1) |
Precision, RSD (%) |
Accuracy, RE (%) |
6.25 |
6.16 ± 0.41 |
6.72 |
−1.43 |
6.48 ± 0.37 |
5.68 |
3.72 |
50 |
53.38 ± 2.55 |
4.77 |
6.76 |
54.13 ± 3.23 |
5.97 |
8.26 |
600 |
574.02 ± 15.80 |
2.75 |
−4.33 |
613.91 ± 22.01 |
3.59 |
2.32 |
3.2.4. Recovery and matrix effect. As summarized in Table 2, the mean recoveries of eupatorin at three different concentration levels were determined to be 81.17–90.01%, while the corresponding matrix effects ranged from 81.66% to 88.97%. All RSD values were less than 7.04%.
Table 2 The mean recoveries and matrix effects of plasma eupatorin in rats (n = 6)
Analyte |
Spiked concentration (ng mL−1) |
Extraction recoveries |
Matrix effects |
Mean (%) |
RSD (%) |
Mean (%) |
RSD (%) |
Eupatorin |
6.25 |
83.56 |
7.04 |
81.66 |
1.60 |
50 |
81.17 |
6.16 |
88.97 |
3.01 |
600 |
90.01 |
2.24 |
85.80 |
6.57 |
3.2.5. Stability. Table 3 summarized the stability data of eupatorin at low, medium, and high concentrations under four distinct conditions. The data showed that eupatorin was stable during the 24 h storage at 25 °C (RE: −1.70–5.39), 30 day storage at −20 °C (RE: −0.37–4.20), or after three freeze–thaw cycles (RE: 0.51–6.54). In addition, good stability was observed in the stock solution of eupatorin kept at 4 °C for one month (RE: 1.98–8.60).
Table 3 The stability of plasma eupatorin in rats (n = 6)
Storage condition |
Eupatorin |
Nominal conc. (ng ml−1) |
Found conc. (ng ml−1) |
RE (%) |
Short-term |
6.25 |
6.14 ± 0.385 |
−1.70 |
50 |
52.70 ± 2.149 |
5.39 |
600 |
609.05 ± 29.597 |
1.51 |
Long-term |
6.25 |
6.27 ± 0.400 |
0.27 |
50 |
52.10 ± 1.948 |
4.20 |
600 |
597.79 ± 30.462 |
−0.37 |
Freeze–thaw cycles |
6.25 |
6.66 ± 0.134 |
6.54 |
50 |
51.53 ± 0.970 |
3.07 |
600 |
603.05 ± 30.867 |
0.51 |
Stock solution |
6.25 |
6.37 ± 0.421 |
1.98 |
50 |
54.30 ± 2.661 |
8.60 |
600 |
621.57 ± 19.355 |
3.59 |
3.3. Pharmacokinetic study
Here, the developed LC-MS/MS approach was successfully used in the pharmacokinetic analysis of plasma eupatorin in rats. The concentration–time curve of eupatorin in rat plasma was depicted in Fig. 3. Table 4 listed various pharmacokinetic parameters such as the Cmax (maximum plasma concentration), Tmax (time for reaching the maximum concentration), T1/2 (the half-life), the area after administration under the concentration–time curve (AUC0−t, AUC0−∞), CLz/F (the clearance), and Vz/F (volume of distribution). All these parameters were subjected to the analysis using the non-compartment model and indicated by mean ± SD.
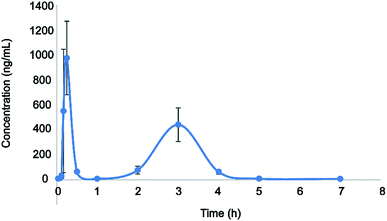 |
| Fig. 3 The concentration–time curve of plasma eupatorin in rats after single oral medication (50 mg kg−1). The data were represented as mean ± SD, n = 6. | |
Table 4 The pharmacokinetic parameters of plasma eupatorin in rats following oral medication (n = 6)
Pharmacokinetic parameters |
Eupatorin |
Cmax (μg L−1) |
974.886 ± 293.898 |
Tmax (h) |
0.25 |
T1/2 (h) |
0.353 ± 0.026 |
AUC0−t (μg l−1 h−1) |
831.224 ± 246.677 |
AUC0−∞ (μg l−1 h−1) |
831.313 ± 246.661 |
CLz/F (L kg−1 h−1) |
25.718 ± 7.266 |
Vz/F (L kg−1) |
13.265 ± 4.457 |
The pharmacokinetic study revealed that the Cmax and Tmax were 974.886 ± 293.898 μg L−1 and 0.25 h, respectively, indicating that eupatorin was rapidly absorbed by the gastrointestinal system. Meanwhile, the T1/2 was 0.353 ± 0.026 h, showing that the analyte has a short residence time in the body and can be administered multiple times to improve clinical efficacy. In addition, we found that the mean plasma concentration displayed double peaks after oral medication of eupatorin; the first and second peaks occurred at around 0.25 h and 3 h, respectively. Consistent with the previous studies,29,36,37 the above phenomenon may be related to the heterogeneity of gastrointestinal absorption. It has been suggested that multiple absorption sites are located on distinct parts of the gastrointestinal system. Given the difference in the permeability of the lumen endocardium at different sites, the absorption time and rate vary among the different parts after oral administration. The absorbed drug superimposed in the blood, and the double peak phenomenon of the drug–time curve appeared.38,39 In addition, it could be caused by the irregular gastric emptying.40–42 It has been shown that the irregular gastric emptying could be a main reason for the double peaks in plasma concentration profiles. However, the underlying mechanism of this observation in the pharmacokinetics analysis remains to be further investigated. Overall, this study can lay a theoretical foundation for the preclinical and clinical application of eupatorin.
4. Conclusions
This study established and assessed a rapid and sensitive LC-MS/MS analytical approach for determining plasma eupatorin in rats, while successfully conducting a pharmacokinetic analysis using the developed method. We showed that following oral medication, eupatorin was quickly absorbed, reaching the maximum blood concentration and displaying the secondary absorption. Moreover, eupatorin had a short residence time in the body, as indicated by its short half-life, and can be administered multiple times to improve the clinical efficacy. Thus, these findings could lay a foundation for the clinical application of eupatorin and provide technical support for consuming eupatorin-containing food and drugs.
Conflicts of interest
The authors have no conflicts of interest to disclose.
References
- W. G. Ihraiz, M. Ahram and S. K. Bardaweel, Acta Pharm., 2020, 70, 179–190 CAS.
- A. Tellez, C. Rodriguez-Padilla, D. M. Juarez-Garcia, L. Jaime-Bernal, T. Sanchez-Jauregui, D. Almaraz-Castruita and H. Vielma-Ramirez, Am. J. Clin. Hypn., 2020, 62, 298–310 CrossRef PubMed.
- D. M. Kopustinskiene, V. Jakstas, A. Savickas and J. Bernatoniene, Nutrients, 2020, 12, 457 CrossRef.
- N. A. Razak, N. Abu, W. Y. Ho, N. R. Zamberi, S. W. Tan, N. B. Alitheen, K. Long and S. K. Yeap, Sci. Rep., 2019, 9, 1514 CrossRef PubMed.
- V. Androutsopoulos, R. R. Arroo, J. F. Hall, S. Surichan and G. A. Potter, Breast Cancer Res., 2008, 10, R39 CrossRef PubMed.
- R. Pariyani, I. S. Ismail, A. Azam, A. Khatib, F. Abas, K. Shaari and H. Hamza, J. Pharm. Biomed. Anal., 2017, 135, 20–30 CrossRef CAS PubMed.
- M. A. Hossain and S. M. Mizanur Rahman, Arabian J. Chem., 2015, 8, 218–221 CrossRef CAS.
- N. D. Yuliana, A. Khatib, A. M. Link-Struensee, A. P. Ijzerman, F. Rungkat-Zakaria, Y. H. Choi and R. Verpoorte, Planta Med., 2009, 75, 132–136 CrossRef CAS PubMed.
- K. Lee, D. Hyun Lee, Y. J. Jung, S. Y. Shin and Y. H. Lee, Appl. Biol. Chem., 2016, 59, 193–199 CrossRef CAS.
- S. Estevez, M. T. Marrero, J. Quintana and F. Estevez, PLoS One, 2014, 9, e112536 CrossRef PubMed.
- I. Doleckova, L. Rarova, J. Gruz, M. Vondrusova, M. Strnad and V. Krystof, Fitoterapia, 2012, 83, 1000–1007 CrossRef CAS PubMed.
- M. Laavola, R. Nieminen, M. F. Yam, A. Sadikun, M. Z. Asmawi, R. Basir, J. Welling, H. Vapaatalo, R. Korhonen and E. Moilanen, Planta Med., 2012, 78, 779–786 CrossRef CAS PubMed.
- M. F. Yam, V. Lim, I. M. Salman, O. Z. Ameer, L. F. Ang, N. Rosidah, M. F. Abdulkarim, G. Z. Abdullah, R. Basir, A. Sadikun and M. Z. Asmawi, Molecules, 2010, 15, 4452–4466 CrossRef CAS PubMed.
- M. F. Yam, C. S. Tan, M. Ahmad and R. Shibao, Eur. J. Pharmacol., 2016, 789, 27–36 CrossRef CAS PubMed.
- I. H. Baek, Xenobiotica, 2019, 49, 734–739 CrossRef CAS PubMed.
- Z. Guo, B. Li, J. Gu, P. Zhu, F. Su, R. Bai, X. Liang and Y. Xie, Molecules, 2019, 24, 3057 CrossRef CAS PubMed.
- N. H. Saidan, A. F. Aisha, M. S. Hamil, A. M. Majid and Z. Ismail, Pharmacogn. Res., 2015, 7, 23–31 CrossRef PubMed.
- M. F. Yam, E. A. Mohamed, L. F. Ang, L. Pei, Y. Darwis, R. Mahmud, M. Z. Asmawi, R. Basir and M. Ahmad, J. Acupunct. Meridian Stud., 2012, 5, 176–182 CrossRef PubMed.
- G. Akowuah, I. Zhari, I. Norhayati, A. Sadikun and S. Khamsah, Food Chem., 2004, 87, 559–566 CrossRef CAS.
- Z. Guo, X. Liang and Y. Xie, J. Pharm. Biomed. Anal., 2019, 164, 135–147 CrossRef CAS PubMed.
- C. Liang, J. Yin, Y. Ma, X. Zhang and L. Zhang, J. Pharm. Biomed. Anal., 2020, 177, 112835 CrossRef CAS PubMed.
- L. Li, R. Feng, X. Feng, Y. Chen, X. Liu, W. Sun and L. Zhang, RSC Adv., 2020, 10, 10552–10558 RSC.
- U.S. Department of Health and Human Services, Food and Drug Administration, Center for Drug Evaluation and Research (CDER), Guidance for Industry, Bioanalytical Method Validation, 2018 Search PubMed.
- F. Zhang, L. Sun, J. Zhai, T. Xia, W. Jiang, M. Li, S. Gao, X. Tao, W. Chen and Y. Chai, BioMed Res. Int., 2019, 2019, 1854323 Search PubMed.
- W. Zhao, L. Pang, D. Xu and N. Zhang, Molecules, 2015, 20, 9084–9098 CrossRef CAS PubMed.
- L. Zhao, W. Li, Y. Li, H. Xu, L. Lv, X. Wang, Y. Chai and G. Zhang, J. Chromatogr. Sci., 2015, 53, 1185–1192 CAS.
- B. Yang, L. Xie, S. Peng, K. Sun, J. Jin, Y. Zhen, K. Qin and B. Cai, J. Sep. Sci., 2019, 42, 2341–2350 CrossRef CAS PubMed.
- E. Ezzeldin, M. Iqbal, R. Al-Salahi and T. El-Nahhas, J. Pharm. Biomed. Anal., 2019, 166, 244–251 CrossRef CAS PubMed.
- X. Zhang, J. Guan, H. Zhu and T. Niu, J. Chromatogr. B: Anal. Technol. Biomed. Life Sci., 2014, 971, 126–132 CrossRef CAS PubMed.
- C. Zhao, S. Han, S. Yang and W. Xin, Biomed. Chromatogr., 2019, 33, e4667 CAS.
- B.-H. Bao, A. Kang, Y. Zhao, Q. Shen, J.-S. Li, L.-Q. Di and J.-X. Li, J. Chromatogr. B, 2017, 1052, 60–65 CrossRef CAS PubMed.
- M. Shi, M. Xu and L. Yin, J. Pharm. Pharmacol., 2020, 72, 1061–1071 CrossRef CAS PubMed.
- L. Wang, X. Ren, Y. He, G. Cui, Z. Wei, J. Jia, J. Cao, Y. Liu, B. Cong, Y. Niu and K. Yun, Eur. J. Drug Metab. Pharmacokinet., 2020, 45, 477–485 CrossRef CAS PubMed.
- M. Ding, W. Ma, X. Wang, S. Chen, S. Zou, J. Wei, Y. Yang, J. Li, X. Yang, H. Wang, Y. Li, Q. Wang, H. Mao, X. M. Gao and Y. X. Chang, J. Ethnopharmacol., 2019, 242, 112055 CrossRef CAS PubMed.
- Z. Wang, Q. Wu, Y. Meng, Y. Sun, Q. Wang, C. Yang, Q. Wang, B. Yang and H. Kuang, J. Chromatogr. B: Anal. Technol. Biomed. Life Sci., 2015, 985, 164–171 CrossRef CAS PubMed.
- R. Shi, S. Qiao, D. Yu, X. Shi, M. Liu, X. Jiang, Q. Wang and L. Zhang, J. Chromatogr. B: Anal. Technol. Biomed. Life Sci., 2011, 879, 1625–1632 CrossRef CAS PubMed.
- Z. Zhu, L. Zhao, X. Liu, J. Chen, H. Zhang, G. Zhang and Y. Chai, J. Chromatogr. B: Anal. Technol. Biomed. Life Sci., 2010, 878, 2184–2190 CrossRef CAS PubMed.
- S. K. Thapa, M. Upadhyay, T. H. Kim, S. Shin, S. J. Park and B. S. Shin, Molecules, 2019, 24, 2037 CrossRef CAS PubMed.
- M. Zhang, X. Ren, S. Yue, Q. Zhao, C. Shao and C. Wang, Molecules, 2019, 24, 3117 CrossRef CAS PubMed.
- Y. Metsugi, Y. Miyaji, K.-i. Ogawara, K. Higaki and T. Kimura, Pharm. Res., 2007, 25, 886–895 CrossRef PubMed.
- H. Ding, Y. Liu, Z. Zeng, H. Si, K. Liu, Y. Liu, F. Yang, Y. Li and D. Zeng, Res. Vet. Sci., 2012, 93, 374–377 CrossRef CAS PubMed.
- B. Ji, Y. Zhao, P. Yu, B. Yang, C. Zhou and Z. Yu, Talanta, 2018, 190, 450–459 CrossRef CAS PubMed.
Footnote |
† These authors contributed equally to this work. |
|
This journal is © The Royal Society of Chemistry 2020 |
Click here to see how this site uses Cookies. View our privacy policy here.