DOI:
10.1039/D0RA03400B
(Paper)
RSC Adv., 2020,
10, 19003-19007
Cascade annulation reaction (CAR): highly diastereoselective synthesis of pyranopyrazole scaffolds†
Received
16th April 2020
, Accepted 11th May 2020
First published on 19th May 2020
Abstract
An unprecedented domino protocol for the novel synthesis of highly diverse and functionalized tetrahydro pyranopyrazole scaffolds using chalcone epoxide has been reported for the first time. This synthetic protocol generates three consecutive stereogenic centres in a highly diastereoselective manner with the formation of vicinal diol and a quaternary carbon centre. A wide range of substrates were utilized for the scope of this methodology and provided very good yields of pyranopyrazoles. The pyranopyrazoles were also transformed into densely functionalized tetrasubstituted olefins.
Introduction
Pyrazole and its derivatives are a versatile class of organic molecules, widely distributed in pharmaceuticals and agrochemicals, exhibiting a wide range of biological activities.1 In leading drugs, such as Viagra2a and Celebrex,2b they are present as the integral part, and therefore the synthesis of pyrazole motifs is considered important in the pharmaceutical industry.2c The design of cyclic ethers3 and its substructures is an important synthetic target material in organic chemistry since they form the structural nuclei of numerous natural products.4 Moreover, several types of bioactive molecules having wide medical and agrochemical applications contain a pyranopyrazole ring. The fused pyrazole5 molecular entities, display numerous biological activities such as anti-HIV,6 antimicrobial,7 antibacterial and antifungal,8 anticancer,9 and anti-inflammatory activity.10 Some of the representative examples of bioactive tetrahydropyranopyrazoles are shown in Fig. 1.
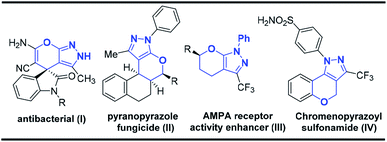 |
| Fig. 1 Some representative examples of bioactive pyranopyrazole scaffolds. | |
On the other hand, epoxides have attracted considerable attention as they play a very important role in organic synthetic chemistry,11 as well as in the pharmaceutical industry.12 Particularly, the relative ease of preparation of chalcone epoxides makes them a promising starting material for the preparation of various heterocyclic compounds.13 Due to the importance of pyranopyrazole framework in pharmacological and biological studies, particularly in the designing of new drug candidates, several groups have focused their efforts in the synthesis of pyranopyrazole scaffolds. For example, pyranopyrazoles have been synthesized via one-pot, Michael/Wittig/oxa-Michael reaction sequence,14 solvent free multicomponent cascade reaction,15 NHC catalyzed annulation reaction,16 and through the reaction of isatylidine β, γ-unsaturated α-ketoester and enynones with pyrazolones17 (Scheme 1). Even though various methods are present in the literature to synthesize pyranopyrazoles, a new and practical alternative protocol for the synthesis of pyranopyrazoles is remarkably essential. To the best of our knowledge, the construction of the pyranopyrazole derivatives using chalcone epoxide and pyrazolone has not been reported so far in the literature. Hence, we design the first methodology to synthesize pyranopyrazoles via a cascade annulation reaction (CAR) using chalcone epoxide 1 and pyrazolone 2.
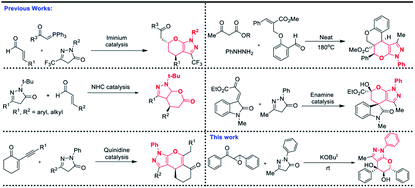 |
| Scheme 1 Reported methods and present approach for the synthesis of pyranopyrazole scaffolds. | |
In continuation of our ongoing research program in the field of heterocyclic chemistry and domino reactions,18 we herein report an unprecedented method for the construction of highly functionalized pyranopyrazole scaffolds using chalcone epoxides and pyrazolone in a highly diastereoselective manner via CAR.
Results and discussions
To execute our idea, we initially investigated the reaction of chalcone epoxide 1a (1 eq.) and pyrazolone 2a (1 eq.) with 1 equivalent of base (K2CO3) in CH3CN as a solvent at room temperature which provided the desired product tetrahydropyranopyrazole 3a as a white solid in 35% yield as shown in Table 1 and entry 1. Next, different types of bases such as L-Proline, KOBut, piperidine, DABCO, NaH, DBU, Cs2CO3, NaOH, and KHCO3 were screened in presence of solvents like MeOH, EtOH, acetone, tBuOH, DCM and iPrOH. The yield of the final product got increased from 35 to 62% (Table 1, entries 2–16). Interestingly, the yield of the final product was improved to 78%, when KOBut is used as a base and EtOH as a solvent (Table 1 and entry 17). The yield of the reaction did not improve significantly when the base loading is increased from 1 to 1.2 equivalents. The combination of KOBut (1 eq.) in ethanol is found to be the optimal condition for the cascade annulation reaction (CAR) as given in Table 1.
Table 1 Optimization conditions for the synthesis of tetrahydropyranopyrazole 3a under various conditionsa,b
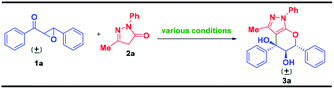
|
Entry |
Base |
Equiv. |
Solvent |
Time |
Yieldb (%) |
All reactions were carried out with 1 mmol scale of chalcone epoxide. Isolated yield of the pure product obtained after column chromatography purification. |
1 |
K2CO3 |
1.0 |
CH3CN |
12 h |
35 |
2 |
L-Proline |
0.2 |
MeOH |
10 h |
21 |
3 |
KOBut |
1.0 |
CH3CN |
10 h |
45 |
4 |
Piperdine |
1.0 |
CH3CN |
10 h |
46 |
5 |
DABCO |
1.0 |
CH3CN |
10 h |
21 |
6 |
NaH |
1.0 |
CH3CN |
12 h |
53 |
7 |
DBU |
0.5 |
CH3CN |
10 h |
50 |
8 |
Cs2CO3 |
0.5 |
CH3CN |
10 h |
46 |
9 |
NaOH |
0.5 |
MeOH |
10 h |
37 |
10 |
KHCO3 |
1.0 |
EtOH |
10 h |
55 |
11 |
NaH |
1.0 |
MeOH |
12 h |
60 |
12 |
KOBut |
1.0 |
Acetone |
12 h |
40 |
13 |
KOBut |
1.0 |
tBuOH |
12 h |
35 |
14 |
KOBut |
1.0 |
DCM |
12 h |
38 |
15 |
KOBut |
1.0 |
MeOH |
12 h |
58 |
16 |
KOBut |
1.0 |
iPrOH |
12 h |
62 |
17 |
KOBut |
1.0 |
EtOH |
12 h |
78 |
18 |
KOBut |
1.2 |
EtOH |
12 h |
78 |
To the best of our knowledge, this is the first report for the synthesis of pyranopyrazoles utilizing chalcone epoxide and pyrazolone under ambient temperature. The highlight of this reaction is that it is highly diastereoselective in nature, affording predominantly only one pair of diastereomer. Prompted by this result, we have examined different types of chalcone epoxides with pyrazolone under the optimal condition which successfully provided the wide variety of tetrahydropyrano pyrazoles 3 in very good yields (60–81%) and the results are summarized in Table 2.
Table 2 Substrate scope of tetrahydropyranopyrazole derivativesa,b
All the reactions were carried out using 1 mmol of chalcone epoxide and 1 mmol of pyrazolone with 1 equiv. of KOBut in the presence of ethanol solvent (5 ml). Isolated yields of the pure products. |
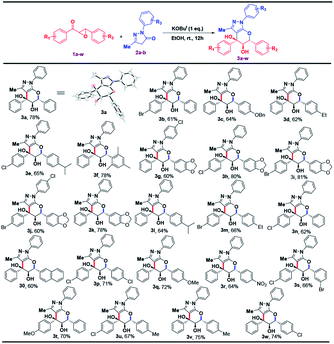 |
To further investigate the enantioselectivity of this new annulative domino protocol, optically pure epoxide 1aa was chosen as the reactant.19 The reaction of this chiral epoxide 1aa with pyrazolone 2a provided the corresponding diol 3aa in 75% yield with enantiomeric ratio 78
:
22 (refer ESI†). The enantioselective nature of the reaction is confirmed by the HPLC analysis of the final product using chiral column where only two peaks are obtained in the chromatogram thereby unambiguously confirming the presence of enantiomer as shown in Scheme 2 (3aa).
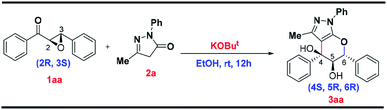 |
| Scheme 2 Synthesis of optically active pyranopyrazole scaffold from chiral chalcone epoxide. | |
The stereochemical (relative stereochemistry) outcome of the trans conformation (5th and 6th position) of the compounds 3a–w was confirmed by the coupling constant of proton value at 5th and 6th positions in the 1H-NMR spectrum. In compound 3a, the 1H NMR spectrum has peaks at δ 4.12 ppm (d, 1H, J = 9.9 Hz, H6) and 5.28 ppm (d, 1H, J = 9.9 Hz, H5), in which the coupling constants (J = 9.9 Hz) clearly indicated an anti-geometry.
Stereochemistry of the nucleophilic addition of the enolate to the ketone can be explained by the transition state models given in Fig. 2. The attack of the enolate on the ketone takes place from Si face leading to the formation of C–C bond in TS-I. The alternative Re face attack of the enolate to the ketone is unfavored due to steric hindrance between the oxirane ring and methyl group in the TS-II. Similarly, the above mentioned concept is applicable to the transition states TS-III and TS-IV respectively for the other isomer.
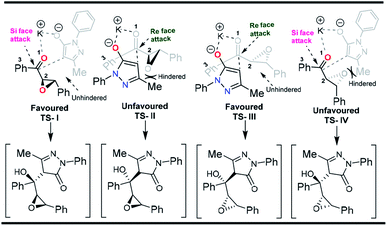 |
| Fig. 2 Possible transition state models for the racemic chalcone epoxide with C-nucleophile. | |
Further, the syn diol conformation of the product arises due to the favored chair conformation of the six membered pyran ring where the hydrogen bonding20 takes place between the axial H and OH. The other conformation for the pyran ring in the chair form is disfavored due to 1,3-diaxial interactions between the phenyl group and hydrogen atom and therefore the trans diol is not formed (Fig. 3). Furthermore, we have confirmed the syn conformation of the vicinal diol groups in structure 3a by NOESY NMR spectrum. Finally, the relative stereochemistry of the compound 3a was confirmed by single crystal XRD analysis.
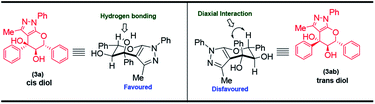 |
| Fig. 3 Favoured (3a) and disfavoured (3ab) conformations. | |
To demonstrate the synthetic transformation of the product 3a, couple of reactions were performed as shown in Scheme 3. A very interesting chemoselective etherification has been carried out where selectively the tertiary alcohol has been converted into corresponding ethers which might be proceeding via SN1 reaction mechanism as shown in Scheme 3. The reaction of tetrahydro pyranopyrazole 3a with aq. HBr in the presence of aq. H2O2 leads to the formation of novel and highly diversified tetrasubstituted olefins with dicarbonyl functionality (4a) in very good yields via cleavage of six membered cyclic ether (pyran) ring as shown in Scheme 3 and the results are summarized in Table 3.
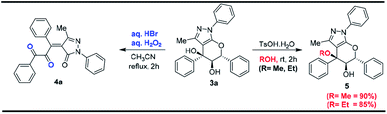 |
| Scheme 3 Functional group transformation of 3a. | |
Table 3 Substrate scope of densely functionalized tetrasubstituted olefinsa,b
Reaction conditions: tetrahydropyrano pyrazole 3a (1 equiv.), aq. HBr (0.2 equiv.), aq. H2O2 (3.0 equiv.) and CH3CN (5 ml) at reflux temperature. Isolated yields of the pure products. |
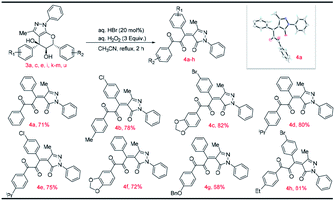 |
The plausible mechanism for the synthesis of tetrahydropyrano pyrazole 3a using racemic chalcone epoxide 1a and pyrazolone 2a is shown in Scheme 4. Pyrazolone 2a can serve as a nucleophilic component to react with the electrophilic racemic chalcone epoxide 1a, which was mediated by KOBut. First, the C-nucleophile of the enolate 2a reacts with the carbonyl group of the chalcone epoxide 1a giving rise to intermediate A. Subsequently, the intermediate A undergoes abstraction of the proton by a base that lead to enolate and the enolate oxygen attack on the β-position of the epoxide to give the cyclic product which undergoes protonation to provide the desired product 3a as shown in Scheme 4.
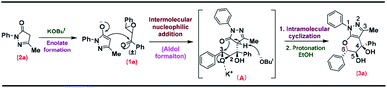 |
| Scheme 4 The proposed reaction pathway for the preparation of racemic tetrahydropyranopyrazoles. | |
The proposed mechanism for the formation of densely functionalized tetrasubstituted olefins is given in Scheme 5. The reaction of hydrobromic acid with aqueous hydrogen peroxide generates the hypobromous acid.21 Hypobromous acid reacts with the tertiary alcohol in pyranopyrazole 3a to give intermediate I. The intermediate I is then transformed into intermediate II by the removal of hypobromite ion. Pyran ring opening takes place by the attack of hypobromite ion which gives intermediate III. The intermediate III loses a molecule of HBr to give the intermediate IV. Reaction of hypobromous acid with the secondary alcohol in intermediate IV provided intermediate V through the loss of the water molecule. The intermediate V loses a molecule of HBr to give the tetrasubstituted olefin 4a as given in Scheme 3.
 |
| Scheme 5 Proposed mechanism for the formation of densely functionalized tetrasubstituted olefins. | |
All the newly synthesized compounds were characterized by 1H-NMR, 13C-NMR and HRMS techniques. The structure of the compounds 3a and 4a were further confirmed by single crystal X-ray diffraction (XRD) method and the ORTEP diagram22 of the compounds 3a and 4a are shown in Table 2 and 3
Conclusions
In conclusion, we have successfully developed an efficient cascade annulation reaction (CAR) for the construction of highly functionalized pyranopyrazole scaffolds with wide variety of substrate scope using chalcone epoxides for the first time. This unprecedented domino protocol is highly diastereoselective in nature. Interestingly, this reaction creates a six membered pyran ring with three contiguous stereogenic centers which include one quaternary chiral center in a unique manner. Moreover, the pyranopyrazoles were successfully manipulated to access novel tetra substituted olefins having dicarbonyl functionality which may act as valuable synthetic building blocks.
Conflicts of interest
There are no conflicts to declare.
Acknowledgements
We thank DST-SERB Ref. No.: (CRG/2019/006323) for the financial support. We also acknowledge the DST-FIST for the ESI-HRMS facility. Manickam Surendar thanks UGC for the scholarship.
Notes and references
-
(a) Y. Zheng, L. Cui, Y. Wang and Z. J. Zhou, Org. Chem., 2016, 81, 4340 CrossRef CAS PubMed;
(b) A. Ansari, A. Ali, M. Asif and Shamsuzzaman, New J. Chem., 2017, 41, 16 RSC;
(c) W. Li, R. Ruzi and K. Ablajan, Tetrahedron, 2017, 73, 164 CrossRef CAS;
(d) J. V. Faria, P. F. Vegi, A. G. C. Miguita, M. S. dos Santos, N. Boechat and A. M. R. Bernardino, Bioorg. Med. Chem., 2017, 25, 5891 CrossRef CAS PubMed;
(e) S. G. Kücųükgüzel and S. Şenkardes, Eur. J. Med. Chem., 2015, 97, 786 CrossRef PubMed.
-
(a) T. D. Penning, J. J. Talley, S. R. Bertenshaw, J. S. Carter, P. W. Collins, S. Docter, M. J. Graneto, L. F. Lee, J. W. Malecha, J. M. Miyashiro, R. S. Rogers, D. J. Rogier, S. S. Yu, G. D. Anderson, E. G. Burton, J. N. Cogburn, S. A. Gregory, C. M. Koboldt, W. E. Perkins, K. Seibert, A. W. Veenhuizen, Y. Y. Zhang and P. C. J. Isakson, Med. Chem., 1997, 40, 1347 CrossRef CAS PubMed;
(b) N. K. Terrett, A. S. Bell, D. Brown and P. Ellis, Bioorg. Med. Chem. Lett., 1996, 6, 1819 CrossRef;
(c) C. Despotopoulou, L. Klier and P. Knochel, Org. Lett., 2009, 11, 3326 CrossRef CAS PubMed.
-
(a) B. M. Trost, A. C. Gutierrez and R. C. Livingston, Org. Lett., 2009, 11, 2539 CrossRef CAS PubMed;
(b) D. P. Steinhuebel and J. D. Bois, Org. Lett., 2002, 4, 293 CrossRef CAS PubMed.
-
(a) E. Kurosawa, A. Fukuzawa and T. Irie, Tetrahedron Lett., 1973, 14, 4135 CrossRef;
(b) M. Suzuki, K. Kurata and E. Kurosawa, Bull. Chem. Soc. Jpn., 1986, 59, 2953 CrossRef CAS;
(c) A. Fukuzawa and T. Masamune, Tetrahedron Lett., 1981, 22, 4081 CrossRef CAS;
(d) K. Audouze, E. O. Nielsen and D. J. Peters, Med. Chem., 2004, 47, 3089 CrossRef CAS PubMed;
(e) G. P. Penga, G. Tiana, X. F. Huanga and F. C. Lou, Phytochemistry, 2003, 63, 877 CrossRef;
(f) A. D. Rodriguez, O. M. Cobar and O. L. Padilla, J. Nat. Prod., 1997, 60, 915 CrossRef CAS PubMed.
-
(a) A. Shaabani, A. Sarvary and S. Keshipour, Tetrahedron, 2009, 65, 3492 CrossRef CAS;
(b) M. Aleem and A. Remaily, Tetrahedron, 2014, 70, 2971 CrossRef;
(c) S. Paul, K. Pradhan and A. R. Das, Tetrahedron, 2014, 70, 6088 CrossRef CAS;
(d) J. Xie, F. Sha and X. Wu, Tetrahedron, 2016, 72, 4047 CrossRef CAS;
(e) M. Seydimemet, K. Ablajan and M. Obul, Tetrahedron, 2016, 72, 7599 CrossRef CAS.
- J. Kim, D. Lee, C. Park, W. So, M. Jo, T. Ok, J. Kwon, S. Kong, S. Jo, Y. Kim, J. Choi, H. C. Kim, Y. Ko, I. Choi, Y. Park, J. Yoon, M. K. Ju, J. Kim, J. Lee and Z. No, ACS Med. Chem. Lett., 2012, 3, 678 CrossRef CAS PubMed.
-
(a) P. T. Mistry, N. R. Kamdar, D. D. Haveliwala and K. S. J. Patel, Heterocycl. Chem., 2012, 49, 349 CrossRef CAS;
(b) A. H. Mandour, E. R. El-Sawy, M. S. Ebaid and S. M. Hassan, Acta Pharm., 2012, 62, 15 CAS;
(c) P. Cherry, D. I. Starkey and N. K. Cobley, J. Med. Chem., 1998, 41, 787 CrossRef PubMed.
- R. Nagamallu and A. K. Kariyappa, Bioorg. Med. Chem. Lett., 2013, 23, 6406 CrossRef PubMed.
-
(a) J. L. Wang, D. Liu, Z. J. Zheng, S. Shan, X. Han, S. M. Srinivasula, C. M. Croce, E. S. Alnemri and Z. Huang, Proc. Natl. Acad. Sci. U. S. A., 2009, 97, 7124 CrossRef PubMed;
(b) J. Li, Y. F. Zhao, X. Y. Yuan and P. Gong, Molecules, 2006, 11, 574 CrossRef CAS PubMed.
-
(a) S. Prekupec, D. Makuc, J. Plavec, L. Suman, M. Kralj, K. Pavelic, J. Balzarini, E. D. Clercq, M. Mintas and S. J. RaicMalic, Med. Chem., 2007, 50, 3037 CrossRef CAS PubMed;
(b) M. E. A. Zaki, A. H. Saliman, O. A. Hickal and A. E. Z. Rashad, Biosci, 2006, 61, 1 CAS.
-
(a) M. Pineschi, Eur. J. Org. Chem., 2006, 4979 CrossRef CAS;
(b) R. E. Parker and N. S. Isaacs, Chem. Rev., 1959, 59, 737 CrossRef CAS;
(c) I. Erden, A. R. Katritzky, C. W. Rees and E. F. V. Scriven, Comprehensive Heterocyclic Chemistry II, Pergamon, Oxford, 1996, vol. 1A, p. 97 Search PubMed.
-
(a) C. Y. Huang and A. G. Doyle, Chem. Rev., 2014, 114, 8153 CrossRef CAS PubMed;
(b) S. Park, Chem.–Asian J., 2019, 14, 2048 CrossRef CAS PubMed;
(c) E. Thiery, J. Le Bras and J. Muzart, Chem.–Eur. J., 2009, 961 CAS.
-
(a) C. Huo, X. Xu, J. An, X. Jia, X. Wang and C. J. Wang, Org. Chem., 2012, 77, 8310 CrossRef CAS PubMed;
(b) T. Patonay, A. L. Vai and C. J. Nemes, Org. Chem., 1996, 61, 5375 CrossRef CAS;
(c) D. Black, N. Kumar and P. S. R. Mitchell, J. Org. Chem., 2002, 67, 2464 CrossRef CAS PubMed.
- D. Enders, A. Grossmann, B. Gieraths, M. Dü zdemir and C. Merkens, Org. Lett., 2012, 14, 4254 CrossRef CAS PubMed.
- M. Bakthadoss, D. Kannan and R. Selvakumar, Chem. Commun., 2013, 49, 10947 RSC.
- S. R. Yetra, S. Mondal, E. Suresh and A. T. Biju, Org. Lett., 2015, 17, 1417 CrossRef CAS PubMed.
-
(a) R. N. Kumarswamy and V. Kesavan, Org. Lett., 2016, 18, 1354 CrossRef PubMed;
(b) S. Liu, X. Bao and B. Wang, Chem. Commun., 2018, 54, 11515 RSC.
-
(a) M. Bakthadoss, G. Sivakumar and D. Kannan, Org. Lett., 2009, 11, 4466 CrossRef CAS PubMed;
(b) M. Bakthadoss and R. Selvakumar, J. Org. Chem., 2016, 81, 3391 CrossRef CAS PubMed;
(c) M. Bakthadoss, R. Selvakumar and M. Mushaf, Org. Biomol. Chem., 2019, 17, 4767 RSC;
(d) D. Basavaiah, M. Bakthadoss and S. Pandiaraju, Chem. Commun., 1998, 1639 RSC;
(e) M. Bakthadoss and M. Mushaf, RSC Adv., 2018, 8, 12152 RSC;
(f) M. Bakthadoss, A. Devaraj and D. Kannan, Eur. J. Org. Chem., 2014, 1505 CrossRef CAS;
(g) M. Bakthadoss and G. Murugan, Eur. J. Org. Chem., 2010, 5825 CrossRef CAS.
- E. J. Corey and F. Y. Zhang, Org. Lett., 1999, 1, 1287 CrossRef CAS PubMed.
- A. Kolocouris, J. Org. Chem., 2009, 74, 1842 CrossRef CAS PubMed.
-
(a) R. B. Daniel, P. Samuel, S. Shaik and R. Neumann, J. Am. Chem. Soc., 2003, 125, 12116 CrossRef PubMed;
(b) V. P. Sharma, S. L. Jain and B. Sain, Synlett, 2005, 1, 173 Search PubMed;
(c) R. D. Patil, G. Joshi and S. Adimurthy, Ind. Eng. Chem. Res., 2010, 49, 8100 CrossRef CAS.
- Structures were confirmed by single-crystal X-ray data. CCDC number for 3a and 4a are 1943125 and 1977496† respectively.
Footnote |
† Electronic supplementary information (ESI) available. CCDC 1943125 and 1977496. For ESI and crystallographic data in CIF or other electronic format see DOI: 10.1039/d0ra03400b |
|
This journal is © The Royal Society of Chemistry 2020 |
Click here to see how this site uses Cookies. View our privacy policy here.