DOI:
10.1039/D0RA03642K
(Paper)
RSC Adv., 2020,
10, 23372-23378
Synthesis, crystal structures, and luminescent properties of Zn(II), Cd(II), Eu(III) complexes and detection of Fe(III) ions based on a diacylhydrazone Schiff base†
Received
23rd April 2020
, Accepted 13th June 2020
First published on 18th June 2020
Abstract
Acylhydrazone Schiff bases are rich in N and O atoms to coordinate with metal ions to form multidentate complexes. In this study, a novel diacylhydrazone Schiff base (N′1E,N′4E)-N′1,N′4-bis(2-hydroxy-5-nitrobenzylidene)succinohydrazide (H4L) was synthesized from the condensation of nitrosalicylaldehyde and succinic dihydrazide. The interactions of H4L with common monovalent, divalent and trivalent metal ions were investigated by ultraviolet spectroscopy and fluorescence spectroscopy. The results showed that H4L had no obvious effect on the monovalent metal ions (Li+, Na+, K+), but reacted with most divalent and trivalent metal ions, and showed single selectivity in the fluorescence recognition of Fe3+ ions. More importantly, three kinds of binuclear molecular structures, [Zn2(H2L)2]·5DMF (Zn-L), [Cd2(H2L)2]·DMF·H2O (Cd-L) and [Eu2(H2L)3]·6DMSO (Eu-L), have been studied to further illustrate the interaction mode of diacylhydrazone Schiff base and metal ions. In addition, the optical properties of these crystallized complexes have been studied in DMF solution.
Introduction
The acylhydrazone Schiff base is the product formed by condensation reaction of hydrazine derivatives with aldehydes or ketone derivatives.1 According to the number of acylhydrazone groups (–NH–CO–), it can be divided into monoacylhydrazone, diacylhydrazone and polyacylhydrazone Schiff base. As a peculiar kind of Schiff base, the diacylhydrazone Schiff base formed by succinic dihydrazide not only shows good stability because of the p–π conjugated system2,3 but also provides greater flexibility in three-dimensional space on account of the free rotation of the C–C single bond in the succinoyl fraction4 so that this kind of ligand exhibits strong coordination abilities and good characteristics of forming a variety of complex structures. Particularly, acylhydrazone metal complexes have found extensive application in diverse fields, such as magnetism,5–8 catalytic performance,9–12 biological function13,14 and molecular recognition.15–18 And the application of rare earth metal complexes in ion recognition has attracted more and more attention due to their near infrared luminescence properties.19,20 Scores of acylhydrazone metal complexes based on aromatic rings have been reported in the past few years. Nevertheless, merely a few studies on metal complexes of diacylhydrazone Schiff base containing flexible C–C single bond in the molecule, especially rare earth metal complexes have been reported. In 2013, Duan's group reported three kinds of diacylhydrazone Schiff bases and got their complexes based on Ce(III), which can be used to recognize Mg2+ and Al3+, respectively.21
In recent years, we have designed and synthesized series of monoacylhydrazone Schiff bases in our group. A variety of transition metals complexes based on them have indicated that this kind of ligands possessed the advantage of constructing different forms of structures.22–25 To extend our research, we designed and synthesized a new diacylhydrazone Schiff base (N′1E,N′4E)-N′1,N′4-bis(2-hydroxy-5-nitrobenzylidene)succinohy-drazide (H4L) (Scheme 1) by the condensation of nitrosalicylaldehyde and succinic dihydrazide. Even more importantly, we got three novel binuclear complexes Zn-L, Cd-L, Eu-L, and studied the structures and optical properties of them. It was worth mentioning that Zn(II) and Cd(II) are coordinated with two ligands to form butterfly-like structures while Eu(III) is coordinated with three ligands to form a complex with a cavity in the middle.
 |
| Scheme 1 Synthetic route of H4L. | |
Herein, we also investigated the interaction of H4L with common metal ions by using ultraviolet spectroscopy and fluorescence spectrum, and found that H4L has a distinct fluorescence quenching in the presence of Fe3+ ions. Already well known, Fe3+ ions play critical roles in living organisms, and are essential elements in many biochemical process.26–29 However, excess iron ions in human body will cause hemochromatosis and diabetes etc.30 Moreover, for H4L, its molecular structure is relatively simple, and the synthesis only requires one step of reaction. These advantages confirm its potential in the practical application of Fe3+ detection in the future.
Experimental
Materials and measurements
All chemicals used in synthesis were obtained from commercial sources without further refinement. FT-IR spectrum was recorded in the VERTEX 80V FT-IR spectrometer as KBr pellets in the 4000–400 cm−1 region. 1H NMR and 13C NMR spectra were recorded on a Bruker ARX400 spectrometer (400 MHz) instrument in DMSO-d6 with Me4Si as the internal standard. The UV-vis absorption spectra were examined on a PERSEE TU-1950 spectrophotometer in the wavelength range of 190–650 nm and the solvent used to register the UV-visible spectra was DMF solution. The photoluminescence spectra were recorded by using a Hitachi F-7000 luminescence spectrophotometer equipped with a 450 W xenon lamp as the excitation source. The photomultiplier tube voltage was 700 V, the scan speed was 1200 nm min−1, and the slit width was 5.0 nm. The powder X-ray diffraction (PXRD) patterns of the samples were measured using a Japan Rigaku D/Max γ A X-ray diffractometer equipped with graphitemonochromatized Mo-Kα radiation (λ = 0.71073 Å). Thermo-gravimetric analyses (TGA) were carried out on a SEIKO TG/DTA 6200 thermal analyzer from room temperature to 650 °C at a ramp rate of 10 °C min−1 in a flowing 50 mL min−1 nitrogen atmosphere. The mass spectra was recorded by using an AGILENT Liquid Chromatograph Mass Spectrometer (Q-TOF 6520).
Preparation of the ligand H4L
An ethanol (20 mL) solution of 5-nitrosalicylaldehyde (3.677 g, 20 mmol) was added dropwise to an ethanol (20 mL) solution of succinic dihydrazide (1.462 g, 10 mmol) with stirring ceaselessly. The addition of one drop of concentrated hydrochloric acid induced a color change to yellow along with immediate precipitation. After that, the mixture was refluxed for 8 hours with continuous stirring and subsequently cooled to room temperature. A yellow solid was collected by vacuum filtration, washed with ethanol and diethyl ether several times, and dried in air. Yield: (73%). Element analysis results of C18H16N6O8 (444.10), theoretical value (%): C, 48.65; H, 3.63; N, 18.91. Experimental value (%): C, 48.83; H, 3.72; N, 18.44. Selected IR (KBr pellet, cm−1): ν (O–H) 3288; ν (C
O) 1647; ν (C
N) 1557(s). 1H NMR (400 MHz, DMSO-d6) δ (ppm) 12.31 (s, 2H), 11.92 (s, 2H), 9.09 (s, 2H), 8.54–8.46 (m, 4H), 7.18–7.05 (m, 2H), 3.00 (t, J = 4.0 Hz, 2H), 2.60 (t, J = 4.0 Hz, 2H). 13C NMR (175 MHz, DMSO-d6) δ (ppm) 175.61, 174.16, 168.79, 168.45, 164.16, 162.97, 162.19, 160.51, 143.13, 140.32, 137.98, 137.80, 128.65, 126.74, 124.39, 117.83, 29.17, 27.18.
Preparation of complexes
A solution of H4L (22 mg, 0.05 mmol) in 2 mL DMF containing 20 drops of NaOH (0.1 M) methanol was added in the solution of Zn(NO3)2·6H2O (30 mg, 0.1 mmol) in 9 mL of methanol with continuous stirring for ca. 30 min. Then, the resulting solution was filtered and remained undisturbed at room temperature to give a yellow solution. A yellow single crystal suitable for X-ray analysis was produced by slow evaporation of mother liquor for three days. For C51H63Zn2N17O21 (Zn-L), yield: 69%.
A solution of H4L (22 mg, 0.05 mmol) in 3 mL DMF containing 30 drops of NaOH (0.1 M) methanol was added in the solution of Cd(NO3)2·4H2O (31 mg, 0.1 mmol) in 8 mL of methanol with continuous stirring for ca. 30 min. The resulting solution was then filtered and remained undisturbed at room temperature. A yellow green single crystal suitable for X-ray analysis was produced by slow evaporation of mother liquor for three days. For C39H37Cd2N13O18 (Cd-L), yield: 67%.
A solution of Eu(CH3COO)3·H2O (12 mg, 0.05 mmol) in 3 mL DMSO was added by solution of H4L (13 mg, 0.05 mmol) in 3 mL DMSO. The reaction solution was filtrated after stirring with ca. 20 min. Yellow single crystals suitable for X-ray diffraction analysis were obtained from isopropanol vapor diffusion into the filtrate for several days. For C66H78Eu2N18O30S6 (Eu-L), yield: 78%.
X-ray crystal structure determinations
Diffraction intensity for the complexes were collected on a Rigaku RAXIS-RAPID CCD diffractometer equipped with a graphitemonochromatic MoKα radiation (l ¼ 0.71073 Å) using a ω scan mode at 153 ± 2 K (complexes Zn-L and Cd-L), 296 ± 2 K (Eu-L). The structures were solved by direct methods and refined using full-matrix least square techniques on F2 with the program SHELXL (complexes Cd-L and Eu-L) and Olex2 (three complexes). Crystallographic data were shown in Table 1.
Table 1 Crystal data and structure refinement of the complexes
Complexes |
Zn-L |
Cd-L |
Eu-L |
Formula |
C51H63Zn2N17O21 |
C39H37Cd2N13O21 |
C66H69Eu2N18O30S6 |
M/mol−1 |
1380.92 |
1248.61 |
2090.67 |
T/K |
153(2) |
153(2) |
296(2) |
Crystal system |
Monoclinic |
Triclinic |
Orthorhombic |
Space group |
C2/c |
P![[1 with combining macron]](https://www.rsc.org/images/entities/char_0031_0304.gif) |
Pbcn |
a/Å |
15.6845(13) |
12.091(2) |
27.308(6) |
b/Å |
18.3664(15) |
12.459(3) |
19.968(5) |
c/Å |
21.3297(12) |
17.130(3) |
16.327(3) |
α/° |
90 |
90.42(3) |
90 |
β/° |
100.584(7) |
104.13(3) |
90 |
γ/° |
90 |
95.41(3) |
90 |
V/Å3 |
6039.8(8) |
2490.0(9) |
8903(3) |
Z |
8 |
2 |
4 |
ρ/mg·cm−3 |
1.519 |
1.665 |
1.560 |
μ(Mo-Kα)/mm−1 |
0.885 |
0.945 |
1.623 |
F (000) |
2864 |
1252 |
4212 |
S (GOF) |
1.043 |
1.042 |
1.031 |
R1[I > 2σ(I)] |
0.0570 |
0.0377 |
0.0728 |
wR2[I > 2σ(I)] |
0.1026 |
0.1003 |
0.1676 |
Δρmax, min/eÅ−3 |
0.979, −1.010 |
1.444, −0.552 |
1.640,−1.141 |
Results and discussion
Crystal structure of [Zn2(H2L)2]·5DMF (Zn-L)
X-ray crystallographic analysis reveals that Zn-L crystallizes in the monoclinic space group C2/c. As shown in Fig. 1, the binary core unit of Zn-L contains two Zn(II) ions, two partially deprotonated [H2L]2− ligands and five solvent DMF molecules. Moreover, it possesses a 2-fold axis of symmetry, in addition the atoms N9, C29 of one DMF molecule lies on the 2-fold axis. Each Zn(II) shows 6-coordination with O on the two phenolic hydroxyl groups, N on the imines and O on the two carbonyl groups of two ligands to form stable five-membered rings and six-membered rings. The coordination environment is Zn–N2O4, including Zn–O bonds fall in the range 2.016(Zn1–O3) to 2.243(Zn1–O2) Å and Zn–N fall in the range 2.086 (Zn1–N1) to 2.088 (Zn1–N4) Å to form a rhombic metallacycle with a Zn⋯Zn separation of 6.44 Å, making the double-nucleus structure looks like a butterfly. The dihedral angles between the two tridentate chelators in one bridge ligand both are about 88°. There are almost only Van der Waals interactions between the binuclear complexes to arrange into 3D structure. The free DMF molecules exist in the structure by forming hydrogen bonds with uncoordinated N on the amide groups in the ligands.
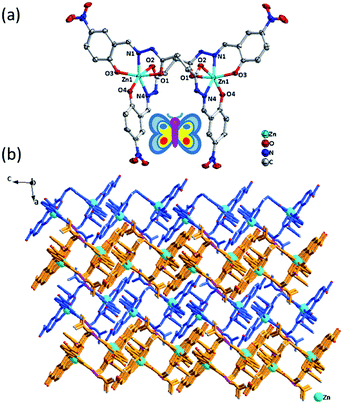 |
| Fig. 1 Crystal structure of Zn-L. (a) ORTEP picture of binuclear Zn-L combined with coordination bond, solvent molecules and anions are omitted for clarity, (b) the H-bonding linking the molecule of DMF with the binuclear structure (purple dashed lines) and 3D supramolecular structure of Zn-L from b axis. | |
Crystal structure of [Cd2(H2L)2]·DMF·H2O (Cd-L)
As shown in Fig. 2, X-ray crystallographic analysis reveals that Cd-L is a binuclear molecule and crystallizes in the triclinic space group P
, and the binary core unit contains two Cd(II) ion, two partially deprotonated [H2L]2− ligands, solvent DMF molecules (including a partially occupied and unordered DMF) and H2O molecule. Similar to complex Zn-L, each Cd(II) exhibits 6-coordination with O on two phenolic hydroxyl groups, N on two imines and O on two carbonyl groups in two ligands to form stable five-membered rings and six-membered rings in the coordination environment of Cd–N2O4. The dihedral angles between the two tridentate chelators in one bridge ligand are about 85° and 80°, respectively. Thus, Cd-L demonstrates the similar double-nucleus structure with butterfly shape and the distance of Cd⋯Cd is ca. 6.54 Å. The four uncoordinated imine groups don't deprotonate, making them easy to be donors of hydrogen bonds. N10 on imine group of the binuclear structure and O4 on phenolic hydroxyl in the other binuclear unit form an N–H⋯O hydrogen bond to come into being dimers. The distance between adjacent Cd in the two binuclear structures forming dimer is about 6.01 Å. Furthermore, each dimer interacts with two neighbours to form 1D chains by H-bonding between imine and terminal nitro group (N3–H3⋯O16: 2.049, 2.808, 141.31). In addition, the adjacent 1D chains are further assembled by four kinds of hydrogen bonds (N4–H4⋯O17: 2.056, 2.835, 144.15; N9–H9⋯O18: 2.013, 2.791, 144.15; O18–H18A⋯O17: 1.975, 2.824, 177.94; O18–H18⋯O10: 1.963, 2.813, 177.32) between ligands, DMF and H2O (Fig. 2c) to form a 3D supramolecular structure (Fig. 2d).
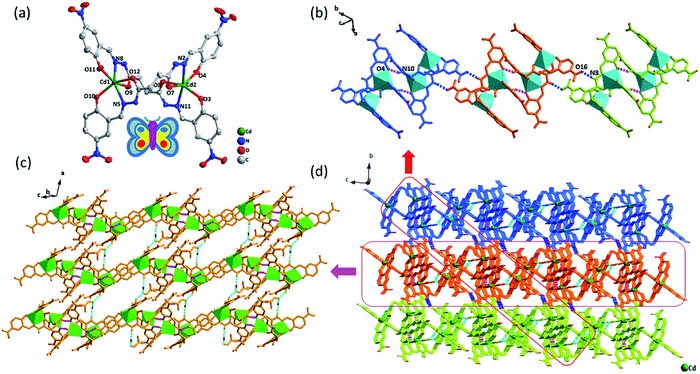 |
| Fig. 2 Crystal structure of Cd-L. (a) ORTEP picture of binuclear Cd-L combined with coordination bond, solvent molecules and anions are omitted for clarity, (b) The H-bonding linking between binuclear structures to form dimer (N10–H10⋯O4: purple dashed lines)and 1D chain structure (N3–H3⋯O16: blue dashed lines) of Cd-L, (c) 2D plane linked by four kinds of hydrogen bonding (light blue dashed lines), (d) 3D supramolecular structure of Cd-L from a axis. | |
Crystal structure of [Eu2(H2L)3]·6DMSO (Eu-L)
A single crystal X-ray analysis of compound Eu-L reveals that it crystallizes in the orthorhombic space group Pbcn. As shown in Fig. 3, the molecule of the compound Eu-L comprises three deprotonated ligands and two Eu(III) ions. The two Eu(III) ions are coordinated with three equivalent NOO tridentate chelators of three ligands to form a three-crown structure at both ends that cannot completely overlap, which shows that Eu-L doesn't have an ideal C3 symmetry (Fig. S3†). In fact, the compound Eu-L just possesses a 2-fold axis of symmetry. Each ligand bridges two Eu(III) ions in a twist manner with the Eu⋯Eu separation being 6.5219(12) Å. Three dihedral angles between the two tridentate chelators in one bridge ligand is about 86°, 83° and 80°. The opening of the cavity within the binuclear structure is a rhombus of size about 6.8 × 6.5 Å2. The radius of the cavity is about 2.25 Å, and the closed separation from the cavity center to the oxygen atom of the carbonyl in the ligand is about 2.30 Å.
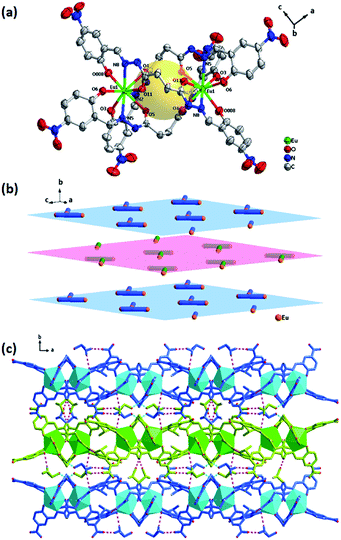 |
| Fig. 3 Crystal structure of Eu-L. (a) ORTEP picture of binuclear Eu-L combined with coordination bond, solvent molecules and anions are omitted for clarity, (b) simplified stacking diagram of Eu-L, the metal is drawn in orange, the virtual bond between Eu(III) of one binuclear is drawn in blue and green respectively, (c) 3D supramolecular structure linked by hydrogen bonding (C–H⋯O: purple dashed lines; N–H⋯O: light blue dashed lines)of Eu-L from c axis. The different colors represent different layers. | |
Interestingly, the binuclear structures are interlaced with each other (Fig. 3b) and interconnected by nonclassical hydrogen bonds such as C–H⋯O interaction (C30–H30C⋯O6 2.629 3.528
156.13; C29–H29B⋯O2 2.302 3.203
155.95) between the -OHphenol and the methyl of DMSO to form 2D sheets. Then each layer is further connected by the N–H⋯O interaction involving the amide N–H and the O of the DMSO extending the network in the three dimension. This 3D framework architecture is further supported by the hydrogen bonds of binuclear structures with other two DMSO molecules (Fig. 3c).
As a complement, the lengths (Å) and angles (deg) of hydrogen bonds and selected bonds data for the complexes Zn-L, Cd-L and Eu-L can be seen in Tables S1–S4†. The structures from the other viewing direction of Zn-L, Cd-L and Eu-L are shown in Fig. S4–S6.†
Powder X-ray diffraction (PXRD) analysis
To prove the purity and crystallinity of the bulk materials, the three complexes were employed towards the PXRD analysis, shown in Fig. 4. Notably, the experimental PXRD pattern of Zn-L, Cd-L and Eu-L matches quite well with that of the simulated pattern obtained from the Mercury, which shows rational crystalline phase purity of the bulk sample.
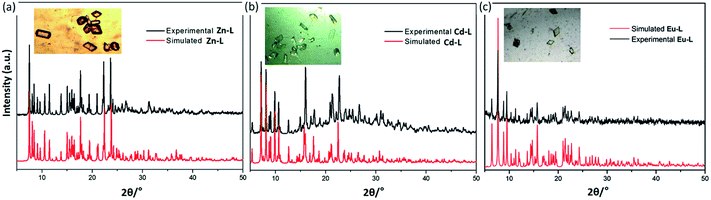 |
| Fig. 4 The XRPD patterns of complexes Zn-L, Cd-L and Eu-L. | |
Optical properties
UV-vis absorption studies of ligand and complexes. The ligand H4L and complexes have good solubility in DMF solution. The UV-vis spectrum of the free H4L in DMF solution exhibits a major absorption band at 287 nm attributed to the conjugate system formed by the co-plane of benzene ring and C
N bond, the maximum absorption peak (λmax) located at 450 nm corresponding to the π–π* and n–π* transitions.31 The addition of divalent metal ions(Ca2+, Mg2+, Ba2+, Mn2+, Co2+, Cu2+ and Zn2+) and trivalent metal ions(Al3+, Cr3+, Fe3+ and Eu3+) to the solution of H4L (20 μM) significantly change the λmax of pure H4L (Fig. S8†). Remarkably, it shows same equivalence points after the addition of Eu3+ compared with divalent metal ions, such as Zn2+, indicating the similar coordination pattern between them (see Fig. 5). It can clearly be seen that the result matches the UV-vis spectrum of the complexes, and is consistent with the previous structural analysis. Whereas the addition of monovalent cations (Li+, Na+ and K+) to the H4L solution do not impose obvious change to the absorption spectrum.
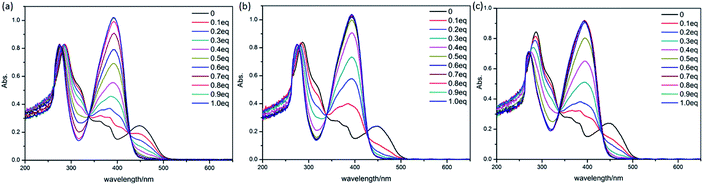 |
| Fig. 5 UV-vis titration spectra of Zn2+ (a), Cd2+ (b) and Eu3+ (c) to H4L in DMF. | |
Fluorescent properties of ligand and complexes. The photoluminescent behaviours of three complexes as well as the free ligand H4L were investigated in the solution of DMF at room temperature (Fig. 6). The fluorescence emission spectra of pure H4L exhibits dual emission at λem values of 320 nm (accompanied by two shoulder peaks at 307 nm, 331 nm) and 417 nm, upon irradiation at 290 nm, which may be caused by the π–π* transition of the conjugated system in the Schiff base ligand. Apparently, the emission of complexes Zn-L and Cd-L are similar to that of the ligand, and the emission at 424 nm of complex Eu-L is similar to that of the ligand, indicating that the binuclear complexes have an L-based emission. As for the complex Zn-L, compared to the free ligand, the intensity of the shoulder peaks is reduced, and the peak at 420 nm has a visible redshift, which can be attributed to π–π* intraligand (IL) transitions of the ligand and ligand-to-metal charge transfer.22 Fluorescence spectra of Cd-L shows similar changes with Zn-L, which may be due to their similar molecular structures.32 Due to the complexation of H4L with Eu(III) ions, the intensity of the peak at 417 nm is reduced, accompanied by a slight redshift, and a new peak centred on λem = 615 nm appears, which is the characteristic peak of the Eu(III) complex.33,34 In the meanwhile, the complex Eu-L exhibits a red luminescence while the free ligand H4L shows a blue-green luminescence in DMF solution under the irradiation of λ = 375 nm ultraviolet light.
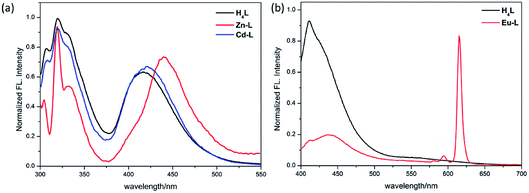 |
| Fig. 6 PL spectra of H4L, Zn-L, Cd-L in DMF with 20 mM (λex = 290 nm) (a), PL spectra and photos of H4L and Eu-L in DMF with 20 Mm (λex = 390 nm) (b). | |
Fluorescence recognition of Fe3+ ions
As shown in Fig. 7a, after the addition of different metal ions including Li+, Na+, K+, Ca2+, Mg2+, Ba2+, Al3+, Cr3+, Mn2+, Fe3+, Co2+, Ni2+, Cu2+, Zn2+, Cd2+ and Hg2+ to H4L solutions, only Fe3+ ions cause the apparent fluorescence quenching of H4L. Differently, the addition of Mg2+, Al3+, Zn2+ result in different degrees of weak fluorescence enhancement.
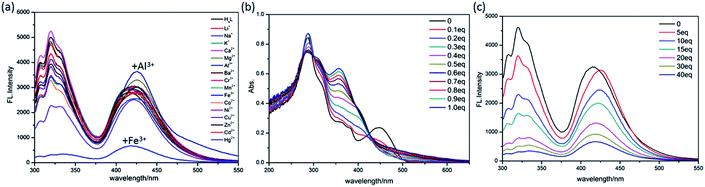 |
| Fig. 7 Fluorescence intensity after adding 40 equiv. of different metal ions to H4L solution (2 × 10−5 M, DMF) (λex = 290 nm) (a); UV-vis titration spectra of Fe3+ to H4L in DMF (b); The change of the fluorescence spectra of H4L (2 × 10−5 M, DMF) upon gradual addition of Fe3+ ions (c). | |
Although the single crystal structure of Fe-L has not been obtained after many efforts on preparing high quality of single crystals, the coordination interaction between Fe3+ and H4L has been investigated by UV-vis titration of H4L (20 μM) by incremental addition of Fe3+ ions (0.03 M) in DMF solution (Fig. 7b). By addition of Fe3+ ions into H4L, the absorption band of H4L at 450 nm disappears. These changes are along with the appearance of two clear isosbestic points at 425 nm and 495 nm. Moreover, the absorption band of H4L at 287 nm shows a slight redshift. These changes are accompanied by the appearance of two clear isosbestic points at 270 nm and 290 nm, which indicate that Fe3+ ions complexed with H4L.
The turn-off of the fluorescence of H4L by Fe3+ ions is explained with the help of a fluorescence emission titration (Fig. 7c). Addition of incremental amounts of Fe3+ ions lead to a fluorescence quenching of the ligand solution, which may be caused by the complexation of H4L with Fe3+ ions, resulting in the charge transfer from ligand to the half-filled 3d orbital of Fe3+ ions.35,36 As shown in the mass spectra (Fig. S9†), the peak assigned to [Fe2(HL)2-H+]+ helps us to speculate the coordination mode of H4L with Fe3+ ions in solution, which is similar with complexes Zn-L, Cd-L and Eu-L with single crystal structural analysis comprehensively (Fig. S10†). In addition, anti-interference experiment (Fig. 8) reveals the absence of any interference with the detection of Fe3+ ions by the inclusion of metal ions, indicating the excellent selectivity of H4L for Fe3+ ions.
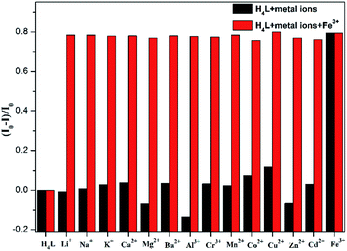 |
| Fig. 8 Fluorescence intensity at 420 nm of H4L (in DMF solution, 20 μM) containing 40 equiv. of iron ions and 40 equiv. of other competing ions. I0 represents the fluorescence intensity of the ligand solution, and I represents the fluorescence intensity of the solution after adding metal ions. | |
Conclusions
In summary, we have successfully demonstrated the synthesis and characterizations of a new diacylhydrazone Schiff base (H4L), which contains Schiff base (–CH
N–), imine group (–NH), nitro group (–NO2) and phenolic hydroxyl group (–OH). It can nicely coordinate with divalent and trivalent metal ions. In addition, three new binuclear coordination compounds namely Zn-L, Cd-L and Eu-L have been synthesized based on H4L. Among them, Zn(II) or Cd(II) can coordinate with two ligands respectively to show a butterfly shape, while Eu(III) can coordinate with three ligands. What's more, the ligand H4L shows a “turn-off” fluorescence sense for Fe3+ ions in DMF solution with high selectivity, which provides a potential of detection applications in the future.
Conflicts of interest
The authors confirm that there are no conflicts of interest to declare.
Acknowledgements
This work was financially supported by the National Natural Science Foundation of China (No. 21471017).
References
- J. Fan, S. Zhang, Y. Xu, N. Wei, B. Wan, L. Qian and Y. Liu, Carbohydr. Polym., 2020, 228, 115379 CrossRef CAS PubMed.
- R. Dwivedi, D. P. Singh, S. Singh, A. K. Singh, B. S. Chauhan, S. Srikrishna and V. P. Singh, Org. Biomol. Chem., 2019, 17, 7497–7506 RSC.
- L. Wu, P. Wu, D. Guo, W. Fu, D. Li and T. Luo, Croat. Chem. Acta, 2015, 88, 1–6 CrossRef CAS.
- R. Borthakur, A. Kumar and R. A. Lal, Spectrochim. Acta, Part A, 2014, 118, 94–101 CrossRef CAS PubMed.
- A. Adhikary, S. Goswami, J. A. Sheikh and S. Konar, Eur. J. Inorg. Chem., 2014, 2014, 963–967 CrossRef CAS.
- S. Xue, L. Zhao, Y. N. Guo and J. Tang, Dalton Trans., 2012, 41, 351–353 RSC.
- A. Adhikary, J. A. Sheikh, S. Biswas and S. Konar, Dalton Trans., 2014, 43, 9334–9343 RSC.
- U. Golla, A. Adhikary, A. K. Mondal, R. S. Tomar and S. Konar, Dalton Trans., 2016, 45, 11849–11863 RSC.
- S. D. Kurbah, A. Kumar, I. Syiemlieh, M. Asthana and R. A. Lal, Inorg. Chem. Commun., 2017, 86, 39–43 CrossRef CAS.
- R. Arunachalam, E. Chinnaraja, A. Valkonen, K. Rissanen, S. K. Sen, R. Natarajan and P. S. Subramanian, Inorg. Chem., 2018, 57, 11414–11421 CrossRef CAS PubMed.
- C. He, J. Wang, L. Zhao, T. Liu, J. Zhang and C. Duan, Chem. Commun., 2013, 49, 627–629 RSC.
- W. Zhu, X. Wu, C. He and C. Duan, Tetrahedron, 2013, 69, 10477–10481 CrossRef CAS.
- S. D. Kurbah, A. Kumar, I. Syiemlieh and R. A. Lal, Polyhedron, 2018, 139, 80–88 CrossRef CAS.
- Q. Yao, J. Qi, Y. Zheng, K. Qian, L. Wei, M. Maimaitiyiming, Z. Cheng and Y. Wang, J. Inorg. Biochem., 2019, 193, 1–8 CrossRef CAS PubMed.
- S. Chopra, J. Singh, H. Kaur, A. Singh, N. Singh and N. Kaur, Eur. J. Inorg. Chem., 2015, 2015, 4437–4442 CrossRef CAS.
- K. Santhiya, S. K. Sen, R. Natarajan, R. Shankar and B. Murugesapandian, J. Org. Chem., 2018, 83, 10770–10775 CrossRef CAS PubMed.
- S. Gao, L. Li, I. Vohra, D. Zha and L. You, R. Soc. Open Sci., 2017, 4, 170466 CrossRef PubMed.
- X. Ma, Y. Cui, S. Liu and J. Wu, Soft Matter, 2017, 13, 8027–8030 RSC.
- D. Shi, X. Yang, H. Chen, Y. Ma, D. Schipper and R. A. Jones, J. Mater. Chem. C, 2019, 7, 13425–13431 RSC.
- D. Shi, X. Yang, Z. Xiao, X. Liu, H. Chen, Y. Ma, D. Schipper and R. A. Jones, Nanoscale, 2020, 12, 1384–1388 RSC.
- L. Zhao, Y. Liu, C. He, J. Wang and C. Duan, Dalton Trans., 2014, 43, 335–343 RSC.
- B.-b. Tang, H. Ma, G.-z. Li, Y.-b. Wang, G. Anwar, R. F. Shi and H. Li, CrystEngComm, 2013, 15, 8069–8073 RSC.
- B.-b. Tang, X.-p. Sun, G.-l. Liu and H. Li, J. Mol. Struct., 2010, 984, 111–116 CrossRef CAS.
- G. Xu, B. Tang, L. Gu, P. Zhou and H. Li, J. Mol. Struct., 2016, 1120, 205–214 CrossRef CAS.
- G. Xu, B.-b. Tang, L. Hao, G.-l. Liu and H. Li, CrystEngComm, 2017, 19, 781–787 RSC.
- J. Kaplan and D. M. Ward, EBioMedicine, 2015, 2, 1582–1583 CrossRef PubMed.
- P. T. Lieu, M. Heiskala, P. A. Peterson and Y. Yong, Mol. Aspect. Med., 2001, 22, 1–87 CrossRef CAS.
- M. A. Alam, T. K. Pal, M. A. Mumit and M. A.-A.-A.-A. Islam, J. Sci. Res., 2012, 4, 635–647 CrossRef.
- X. Gong, X. Ding, N. Jiang, T. Zhong and G. Wang, Microchem. J., 2020, 152, 104351 CrossRef.
- M. W. Hentze, M. U. Muckenthaler, B. Galy and C. Camaschella, Cell, 2010, 142, 24–38 CrossRef CAS PubMed.
- D. Zhang, W. Zhao, Z. Feng, Y. Wu, C. Huo, L. He and W. Lu, E-Polymers, 2019, 19, 15–22 CAS.
- D. Majumdar, D. Das, S. S. Sreejith, S. Nag, S. Dey, S. Mondal, K. Bankura and D. Mishra, Inorg. Chim. Acta, 2019, 496, 119069 CrossRef CAS.
- L. Hou, Y. Song, Y. Xiao, R. Wu and L. Wang, Talanta, 2020, 209, 120534 CrossRef CAS PubMed.
- M. Saif, H. F. El-Shafiy, M. M. Mashaly, M. F. Eid, A. I. Nabeel and R. Fouad, J. Mol. Struct., 2018, 1161, 26–33 CrossRef CAS.
- Z. F. Pu, Q. L. Wen, Y. J. Yang, X. M. Cui, J. Ling, P. Liu and Q. E. Cao, Spectrochim. Acta, Part A, 2020, 229, 117944 CrossRef CAS PubMed.
- D. M. Arvapalli, A. T. Sheardy, K. C. Alapati and J. Wei, Talanta, 2020, 209, 120538 CrossRef CAS PubMed.
Footnote |
† Electronic supplementary information (ESI) available: Details about general methods, structure information, spectra (1H, 13C NMR, IR, UV-vis, fluorescence, mass spectra), PXRD, thermogravimetric analysis. CCDC 1989009–1989011. For ESI and crystallographic data in CIF or other electronic format see DOI: 10.1039/d0ra03642k |
|
This journal is © The Royal Society of Chemistry 2020 |
Click here to see how this site uses Cookies. View our privacy policy here.