DOI:
10.1039/D0RA04008H
(Paper)
RSC Adv., 2020,
10, 25358-25363
Synthesis of polyhydroquinolines and propargylamines through one-pot multicomponent reactions using an acidic ionic liquid immobilized onto magnetic Fe3O4 as an efficient heterogeneous catalyst under solvent-free sonication†
Received
4th May 2020
, Accepted 28th June 2020
First published on 3rd July 2020
Abstract
A nano-sized Fe3O4-supported Lewis acid ionic liquid catalyst for the synthesis of polyhydroquinolines and propargylamines under ultrasound irradiation has been developed. LAIL@MNP was synthesized from imidazolium chlorozincate(II) ionic liquid grafted onto the surface of Fe3O4 nanoparticles and evaluated by FT-IR, TGA, SEM, Raman, TEM, ICP-OES, and EDS. The multicomponent synthesis of polyhydroquinolines and propargylamines proceeded smoothly to afford the desired products in high yields. LAIL@MNP can be separated easily from the reaction mixture and reused for several runs without a significant degradation in catalytic activity.
Introduction
The application of heterogeneous catalysts in organic synthesis has attracted much attention due to the prominent features of these catalysts, such as high efficiency, simple recovery, and reusability.1 However, the major limitation of heterogeneous catalysts is their low catalytic activity and selectivity because of slow dispersibility in the solvent.2 Magnetic nanoparticles (MNPs) are known as a new type of catalyst support because of their easy synthesis, good stability, high surface area, and facile separation from the reaction mixture.3 Currently, there are many reports about the catalytic performance of Fe3O4@ZrO2/SO42−MNPs,4 Fe3O4@SiO2–SO3H MNPs,5,6 CoFe2O4@SiO2–SO3H MNPs,7 Fe3O4@ ZrO2–Pr–SO3H MNPs,8 and CuFe2O4 @SO3H MNPs9 in organic synthesis. Recently, Lewis acidic ionic liquids supported on magnetic nanoparticles have been extensively studied in organic synthesis due to high efficiency, simple recovery by an external magnet, excellent thermal stability, and reuse without loss of the activity.10–14
Polyhydroquinoline heterocycles (PHQ) have received considerable interest owing to their pharmacological activities such as anticancer,15 antiplasmodial,16 antiatherosclerotic,17 antibacterial,18 antiproliferative,19 antimalarial,20 calcium antagonists,21 and antidiabetic properties22 (Scheme 1). Various methods have been developed to synthesize polyhydroquinolines,23–25 pyridines,26 pyridylalanines,27 pyrroles,28 furans,28 etc. via the multicomponent Hantzsch reaction. Recently, different catalysts for the synthesis of PHQ from aldehydes, β-dicarbonyl compounds, active methylene compounds, and ammonium acetate have been reported including metal triflates,29 zeolites,30 ionic liquids,31–33 nanoparticles,34–40 heterogeneous nanocatalyst,41,42 metal halide,43–45 organic solid acid,46 enantioselective organocatalysis,47 nanocomposites,48 Brönsted acidic.49
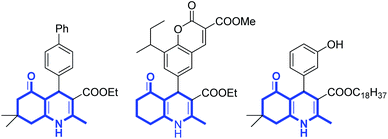 |
| Scheme 1 Some bioactive polyhydroquinolines. | |
Propargylamine derivatives are widely used in the pharmaceutical industry (Scheme 2),50 which are known as precursors in the formation of quinolines,51 oxazoles,52 imidazoles,53 indolizines,54 oxazolidinones,55 aza-anthraquinone,56 phenanthrolines,57 pyrroles,58 pyrrolidines,59 dihydropyridine,60 and pyridocoumarins.61 Moreover, some propargylamine derivatives had been committed as energetic anti-apoptotic agents62 and useful in treating Parkinson's disease patients.63 There were several classical methods for the preparation of propargylamines, and the straightforward and efficient method was A3-coupling from alkynes, aldehydes, and amines.64
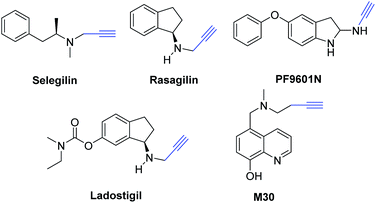 |
| Scheme 2 Some bioactive propargylamines. | |
As a continuation of previous work involving the application of Lewis acidic ionic liquids supported onto magnetic nanoparticles in organic synthesis,65 we report herein the use of the material as a recyclable catalyst for multicomponent reactions in the synthesis of polyhydroquinolines through Hantzsch condensation and propargylamines through A3-coupling reaction under solvent-free sonication.
Results and discussion
Synthesis of LAIL@MNP
The LAIL@MNP was synthesized following a previously reported procedure.65 The magnetic nanoparticle supported ionic liquid catalyst was prepared in a few steps. MNPs were obtained by a simple co-precipitation method in the presence of KOH solution. Next, the imidazole chloride ionic liquid was synthesized from 3-chloroethoxypropylsilane and imidazole. Then, imidazole-functionalized magnetic Fe3O4 nanoparticle (IL@MNP) was reacted with ZnCl2 to afford the LAIL@MNP. Characterization of the synthesized nano-Fe3O4 and LAIL@MNP was determined using Fourier transform infrared (FT-IR) spectroscopy, scanning electron microscopy (SEM), transmission electron microscopy (TEM), thermo-gravimetric analysis (TGA), energy dispersive spectrum (EDS), and Raman spectrum (ESI, Fig. S1–S4†). The amount of zinc element in LAIL@MNP was found to be 0.3 mmol g−1 by ICP-MS.
Synthesis of polyhydroquinolines
The multicomponent reaction is one of the attractive tools in the synthesis of bioactive compounds.66,67 The catalytic activity of LAIL@MNP-catalyzed was performed the synthesis of polyhydroquinolines via Hantzsch reaction, four-component condensation reactions of dimedone, ethyl acetoacetate, ammonium acetate and aldehydes under solvent-free sonication. As shown from Table S1 (please see in ESI†), the condensation reaction between benzaldehyde, dimedone, ethyl acetoacetate, and ammonium acetate in the presence of LAIL@NMP (15 mg) provided in 68% yield under sonication at room temperature for 60 min (Table S1,† entry 5). Interestingly, the excellent yield was observed when the reaction 80 °C within 45 min (Table S1,† entry 9). Then, the loading of LAIL@NMP was examined in various weights ranging from 1 mg to 20 mg, and the best yield was attained with 15 mg of LAIL@NMP. Table 1 shows that LAIL@MNP is also a suitable catalyst for the synthesis of polyhydroquinolines.
Table 1 Comparative effectiveness for the four-component synthesis of polyhydroquinolines
Entry |
Catalyst |
Temperature (°C) |
Time (h) |
Yield (%) |
1 |
Yb(OTf)3/EtOH (5 mol%) |
r.t. |
5 |
90 (ref. 29) |
2 |
p-TSA/EtOH (10 mol%) |
r.t. |
2 |
93 (ref. 68) |
3 |
Scolecite/EtOH (200 mg) |
reflux |
0.75 |
93 (ref. 69) |
4 |
Hf(NPf2)4/C10H18 (1 mol%) |
60 |
3 |
95 (ref. 24) |
5 |
SSA/solvent-free (0.20 mmol, 0.08 g) |
60 |
0.75 |
92 (ref. 70) |
6 |
Sc(OTf)3/EtOH (5 mol%, 25 mg) |
r.t. |
4 |
93 (ref. 23) |
7 |
DBH/solvent-free (10 mol%) |
130 |
0.5 |
90 (ref. 71) |
8 |
Nano Pd-(0)/THF (2 step) |
reflux |
4.3 |
87 (ref. 35) |
9 |
Cs2.5H0.5PW12O40/melting metal (0.01 mmol) |
110 |
0.1 |
92 (ref. 72) |
10 |
Present work: LAIL@MNP (15 mg), solvent-free |
80 |
0.75 |
97 |
LAIL@NMP catalyzed the Hanzsch condensation of aldehyde (1.0 mmol), dimedone (1.0 mmol), ethyl acetoacetate (1.0 mmol), and ammonium acetate (2.0 mmol) solvent-free sonication. As shown in Table 2, the reaction was proceeded smoothly with cyclohexanecarbaldehyde to provide the product in 88% yield was for 45 min under sonication. Ortho- or para-substituted benzaldehydes containing electron-poor groups such as chloro and fluoro exhibited weaker activity than benzaldehyde. For those bearing electron-rich groups such as methyl, methoxy, and tert-butyl at the para position, the yields were nearly equal to that of benzaldehyde. Substrates containing o-substituted polar functional groups, such as –OH and –COOH, afforded the respective products whose yields were 20% less than that of benzaldehyde. The method was also efficient for furan aldehydes to give the desired products in 79–89% yields.
Table 2 The synthesis of various polyhydroquinolines using LAIL@MNP under solvent-free sonicationa
Aldehyde (1 mmol), ethyl acetoacetate (1.0 mmol), dimedone (1 mmol), and ammonium acetate (2.0 mmol) in the presence of LAIL@MNP (15 mg) under solvent-free sonication. Yields are isolated yield. |
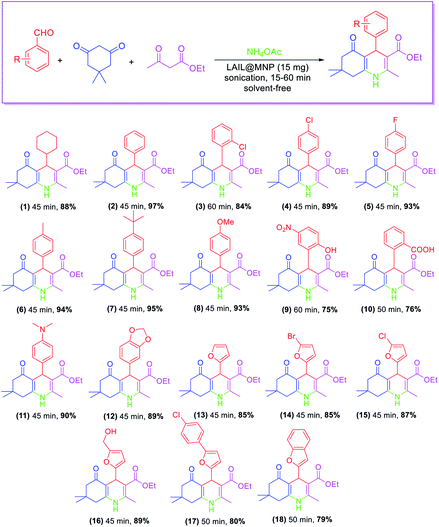 |
A plausible mechanism for the synthesis of polyhydroquinolines using LAIL@NMP was demonstrated in Scheme 3. The zinc species of LAIL@MNP catalyst coordinated with oxygen on the carbonyl group of benzaldehyde which enhanced the electrophilicity of carbonyl carbon, providing rise to a better nucleophilic attack of dimedone to benzaldehyde to produce an intermediate (A). In the second step, β-ketoester (ethyl acetoacetate) is activated by acid catalysis with the release of ammonia from ammonium acetate (NH4OAc) to produce enamine (B) along with CH3COOH as a by-product. Then, the Michael additive reaction occurs between (A) and (B), followed by the cyclization creating intermediate (C) and (D). Finally, the deprotonation of (D) provides the Hantzsch 1,4-dihydropyridines.
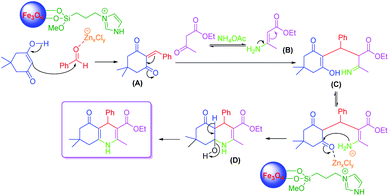 |
| Scheme 3 Proposed mechanism for one-pot four-component synthesis of polyhydroquinolines. | |
Synthesis of propargylamines
The catalytic activity of LAIL@MNP was demonstrated through a one-pot multicomponent reaction of phenylacetylene, piperidine, and aldehydes under solvent-free sonication. The effect of various parameters including time, temperature, amount of catalyst, and different solvents was investigated. As can be seen from Table S3,† the reaction of phenylacetylene (1.5 mmol), piperidine (1.2 mmol) and benzaldehyde (1.0 mmol) was conducted at 30 °C or 80 °C under sonication. Interestingly, a yield of propargylamine was obtained at 80 °C for 45 min in the presence of LAIL@MNP. The excellent yield of (1,3-diphenylprop-2-yn-1-yl)piperidine was achieved at an optimized molar ratio 1
:
1.5
:
1.2 of benzaldehyde, phenylacetylene, and piperidine (entry 19). The effect of catalytic loading and solvent was also examined (entries 24–27). The results showed that the use of 10 mg of LAIL@NMP afforded the highest yield of the product under solvent-free sonication (Table S4†). The current method was compared with other reports (Table 3), and LAIL@MNP catalyst demonstrated good catalytic performance in the preparation of propargylamines.
Table 3 Comparison methods for the preparation of propargylamines
Entry |
Catalytic system |
Temp. (°C) |
Time (h) |
Yield (%) |
1 |
Nano CuO/toluene (10 mol%) |
90 |
5 |
84 (ref. 73) |
2 |
Cu NPs/TiO2 (0.5% mol) |
70 |
7 |
90 (ref. 74) |
3 |
Cu2I(pip)2/toluene (0.4%) |
110 |
2 |
98 (ref. 75) |
4 |
Cu@MOF-5-C/toluene (20 mg) |
110 |
6 |
96 (ref. 76) |
5 |
Cu/Al/toluene (0.12 mmol) |
100 |
22 |
94 (ref. 77) |
6 |
Present method: LAIL@MNP (10 mg), solvent-free sonication |
80 |
0.75 |
85 |
The scope of the substrate was explored with some aromatic aldehydes. Under the optimal conditions, the reaction proceeded smoothly to produce the corresponding propargylamines in good to excellent yields. The reaction was carried out with aryl aldehydes bearing an electron-donating or electron-withdrawing group. p-Methylbenzaldehyde was proceeded smoothly under optimized conditions. However, p-tertbutylbenzaldehyde reacted slowly and gave the desired product in moderate yield for 60 min. The aldehydes with halo or hydroxyl substituents in the para position were also reactive with prolonged reaction time to 60–70 min. The heterocyclic aldehyde, such as furfural and pyridine-4-carbaldehyde, afforded the desired products in acceptable yields (Table 4).
Table 4 LAIL@NMP-catalyzed for the synthesis of propargylaminesa
Reaction conditions: phenylacetylene (1.5 mmol), piperidine (1.2 mmol), and aldehyde (1.0 mmol) under solvent-free ultrasound irradiation. The conversion was determined by GC-MS based on aldehyde. Isolated yield was calculated based on the obtained amount after flash column chromatography. |
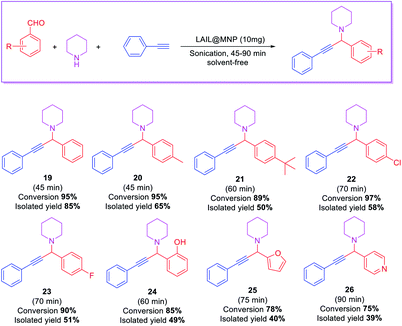 |
A proposed mechanism for the preparation of propargylamine was demonstrated in Scheme 4. The zinc species of LAIL@MNP catalyst coordinated with oxygen on the carbonyl group of benzaldehyde, which enhanced the electrophilicity of carbonyl carbon, providing rise to a better nucleophilic attack of piperidine to benzaldehyde to produce intermediate products (E). This intermediate product was dehydrated to form benzilidenpiperidinium ion (F). Next, phenylacetylene reacted with benzilidenpiperidinium ion (F) to form the desired product.
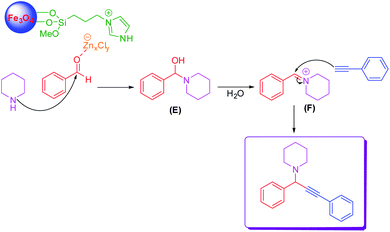 |
| Scheme 4 A plausible mechanism for the synthesis of propargylamines. | |
The recyclability of LAIL@MNP was tested in the preparation of propargylamine under optimal reaction conditions (Fig. 1). After completion, the reaction mixture was diluted with ethyl acetate (30 mL) and the LAIL@MNP was removed by an external magnet. The LAIL@MNP was then washed with ethyl acetate (3 × 3 mL), ethanol (3 × 3 mL), and dried in vacuo. The activity of the recovered catalyst was assessed five consecutive recycling tests. The FT-IR spectrum of recovered LAIL@MNP suggested no significant change in functionality (please see ESI, Fig. S7†).
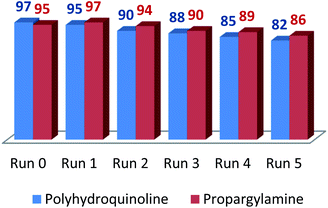 |
| Fig. 1 Recycling of LAIL@MNP for the synthesis of polyhydroquinolines. | |
Experimental
Synthesis of LAIL@MNP
LAIL@MNP was prepared according to a procedure reported previously in the literature.78,79 The LAIL@MNP has been evaluated by FT-IR, Raman, TGA, SEM, TEM, ICP-OES, and EDS (please see in ESI†).
Synthesis of polyhydroquinolines
In a typical experiment, a reaction mixture of aldehyde (1.0 mmol, 0.106 g), ethyl acetoacetate (1.0 mmol, 0.130 g), dimedone (1.0 mmol, 0.140 g), ammonium acetate (2.0 mmol, 0.144 g), and LAIL@MNP (15 mg) was sonicated at 80 °C. After completion, 10 mL ethyl acetate was added and the LAIL@MNP was separated from an organic phase by an external magnet. The ethyl acetate layer was extracted wit water (3 × 15 mL), dried with anhydrous Na2SO4 and evaporated under vacuum. The residue was recrystallized from hot ethanol to give polyhydroquinoline. Synthesized products were confirmed by 1H, 13C NMR, and MS.
Synthesis of propargylamines
In a typical experiment, a reaction mixture of aldehyde (1.0 mmol, 0.106 g), phenylacetylene (1.5 mmol, 0.153 g), piperidine (1.2 mmol, 0.102 g), and LAIL@MNP (10 mg) was sonicated at 80 °C. After completion, 10 mL ethyl acetate was added, and the LAIL@MNP was separated from an organic phase by an external magnet. The organic solution was then dried with anhydrous Na2SO4. The solvent was removed under vacuum, and the crude product was purified through column chromatography using n-hexane/ethyl acetate (9/1) to provide pure propargylamine. Synthesized products were confirmed by 1H, 13C NMR, and MS.
Conclusions
In summary, we have developed a recyclable and efficient LAIL@MNP catalyst for the synthesis of polyhydroquinolines and propargylamines. The present method demonstrated a facile and green approach toward polyhydroquinolines and propargylamines under ultrasound irradiation. The LAIL@MNP can be quickly recovered and reused without a considerable decline in catalytic activity.
Conflicts of interest
There are no conflicts of interests to declare.
Acknowledgements
Hai Truong Nguyen acknowledges the Domestic Master/PhD Scholarship Programme of Vingroup Innovation Foundation (VINIF.2019.TS.20) for polyhydroquilonine derivatives synthesis and characterization. Phuong Hoang Tran acknowledges the Vietnam National University – Ho Chi Minh City (VNU-HCM) through Project No. 562-2020-18-01 for catalytic investigation for propargylamine synthesis and LAIL@MNP preparation characterization.
Notes and references
- N. Mizuno and M. Misono, Chem. Rev., 1998, 98, 199–218 CrossRef CAS PubMed.
- Y.-M. Wang, V. Ulrich, G. F. Donnelly, F. Lorenzini, A. C. Marr and P. C. Marr, ACS Sustainable Chem. Eng., 2015, 3, 792–796 CrossRef CAS.
- V. Polshettiwar, R. Luque, A. Fihri, H. Zhu, M. Bouhrara and J. M. Basset, Chem. Rev., 2011, 111, 3036–3075 CrossRef CAS.
- Y. K. Tailor, S. Khandelwal, R. Gopal, E. Rushell, A. Prajapati and M. Kumar, ChemistrySelect, 2017, 2, 11055–11061 CrossRef CAS.
- A. R. Kiasat and J. Davarpanah, J. Mol. Catal. A: Chem., 2013, 373, 46–54 CrossRef CAS.
- J. Safari and Z. Zarnegar, RSC Adv., 2015, 5, 17738–17745 RSC.
- X.-N. Zhao, G.-F. Hu, M. Tang, T.-T. Shi, X.-L. Guo, T.-T. Li and Z.-H. Zhang, RSC Adv., 2014, 4, 51089–51097 RSC.
- A. Tadjarodi, R. Khodikar and H. Ghafuri, RSC Adv., 2016, 6, 63480–63487 RSC.
- S. Swami, A. Agarwala and R. Shrivastava, New J. Chem., 2016, 40, 9788–9794 RSC.
- Y. Zhang, Y. Zhao and C. Xia, J. Mol. Catal. A: Chem., 2009, 306, 107–112 CrossRef CAS.
- X. Zheng, S. Luo, L. Zhang and J.-P. Cheng, Green Chem., 2009, 11, 455–458 RSC.
- I. Cano, C. Martin, J. A. Fernandes, R. W. Lodge, J. Dupont, F. A. Casado-Carmona, R. Lucena, S. Cardenas, V. Sans and I. de Pedro, Appl. Catal., B, 2020, 260, 118110 CrossRef CAS.
- A. Alizadeh, M. Fakhari, M. M. Khodeai, G. Abdi and J. Amirian, RSC Adv., 2017, 7, 34972–34983 RSC.
- B. Atashkar, M. A. Zolfigol and S. Mallakpour, Mol. Catal., 2018, 452, 192–246 CrossRef CAS.
- L. R. Morgan, B. S. Jursic, C. L. Hooper, D. M. Neumann, K. Thangaraj and B. LeBlanc, Bioorg. Med. Chem. Lett., 2002, 12, 3407–3411 CrossRef CAS.
- P. Beagley, M. A. L. Blackie, K. Chibale, C. Clarkson, R. Meijboom, J. R. Moss, P. J. Smith and H. Su, Dalton Trans., 2003, 3046–3051 RSC.
- P. N. Kalaria, S. P. Satasia and D. K. Raval, Eur. J. Med. Chem., 2014, 78, 207–216 CrossRef CAS PubMed.
- N. Fokialakis, P. Magiatis, I. Chinou, S. Mitaku and F. Tillequin, Chem. Pharm. Bull., 2002, 50, 413–414 CrossRef CAS PubMed.
- J.-C. Liang, J.-L. Yeh, C.-S. Wang, S.-F. Liou, C.-H. Tsai and I.-J. Chen, Bioorg. Med. Chem., 2002, 10, 719–730 CrossRef CAS PubMed.
- A. Ryckebusch, R. Deprez-Poulain, L. Maes, M.-A. Debreu-Fontaine, E. Mouray, P. Grellier and C. Sergheraert, J. Med. Chem., 2003, 46, 542–557 CrossRef CAS PubMed.
- F. Bossert, H. Meyer and E. Wehinger, Angew. Chem., Int. Ed., 1981, 20, 762–769 CrossRef.
- A. Kumar, S. Sharma, V. D. Tripathi, R. A. Maurya, S. P. Srivastava, G. Bhatia, A. K. Tamrakar and A. K. Srivastava, Bioorg. Med. Chem., 2010, 18, 4138–4148 CrossRef CAS PubMed.
- J. L. Donelson, R. A. Gibbs and S. K. De, J. Mol. Catal. A: Chem., 2006, 256, 309–311 CrossRef CAS.
- M. Hong, C. Cai and W.-B. Yi, J. Fluorine Chem., 2010, 131, 111–114 CrossRef CAS.
- R. Surasani, D. Kalita, A. V. D. Rao, K. Yarbagi and K. B. Chandrasekhar, J. Fluorine Chem., 2012, 135, 91–96 CrossRef CAS.
- M. Nasr-Esfahani, B. Karami and M. Behzadi, J. Heterocycl. Chem., 2009, 46, 931–935 CrossRef CAS.
- A. Dondoni, A. Massi and M. Aldhoun, J. Org. Chem., 2007, 72, 7677–7687 CrossRef CAS PubMed.
- T. A. Moss and T. Nowak, Tetrahedron Lett., 2012, 53, 3056–3060 CrossRef CAS.
- L.-M. Wang, J. Sheng, L. Zhang, J.-W. Han, Z.-Y. Fan, H. Tian and C.-T. Qian, Tetrahedron, 2005, 61, 1539–1543 CrossRef CAS.
- S. S. Katkar, P. H. Mohite, L. S. Gadekar, B. R. Arbad and M. K. Lande, Green Chem. Lett. Rev., 2010, 3, 287–292 CrossRef CAS.
- M. M. Heravi, M. Saeedi, N. Karimi, M. Zakeri, Y. S. Beheshtiha and A. Davoodnia, Synth. Commun., 2010, 40, 523–529 CrossRef CAS.
- M. Hong, C. Cai and W.-B. Yi, J. Fluorine Chem., 2010, 131, 111–114 CrossRef CAS.
- M. Tajbakhsh, H. Alinezhad, M. Norouzi, S. Baghery and M. Akbari, J. Mol. Liq., 2013, 177, 44–48 CrossRef CAS.
- S. B. Sapkal, K. F. Shelke, B. B. Shingate and M. S. Shingare, Tetrahedron Lett., 2009, 50, 1754–1756 CrossRef CAS.
- M. Saha and A. K. Pal, Tetrahedron Lett., 2011, 52, 4872–4877 CrossRef CAS.
- M. Abdollahi-Alibeik and A. Rezaeipoor-Anari, J. Magn. Magn. Mater., 2016, 398, 205–214 CrossRef CAS.
- S. T. Fardood, A. Ramazani and S. Moradi, J. Sol-Gel Sci. Technol., 2017, 82, 432–439 CrossRef CAS.
- M. Nasr-Esfahani, S. J. Hoseini, M. Montazerozohori, R. Mehrabi and H. Nasrabadi, J. Mol. Catal. Chem., 2014, 382, 99–105 CrossRef CAS.
- S. Taghavi Fardood, A. Ramazani, Z. Golfar and S. W. Joo, Appl. Organomet. Chem., 2017, 31, e3823 CrossRef.
- L. Saikia, D. Dutta and D. K. Dutta, Catal. Commun., 2012, 19, 1–4 CrossRef.
- A. Maleki, A. R. Akbarzade and A. R. Bhat, J. Nanostruct. Chem., 2017, 7, 309–316 CrossRef CAS.
- A. Ghorbani-Choghamarani, B. Tahmasbi, P. Moradi and N. Havasi, Appl. Organomet. Chem., 2016, 30, 619–625 CrossRef CAS.
- R. Surasani, D. Kalita, A. V. D. Rao, K. Yarbagi and K. B. Chandrasekhar, J. Fluorine Chem., 2012, 135, 91–96 CrossRef CAS.
- J. S. Yoo, T. J. Laughlin, J. J. Krob and R. S. Mohan, Tetrahedron Lett., 2015, 56, 4060–4062 CrossRef CAS.
- S. Ko and C.-F. Yao, Tetrahedron, 2006, 62, 7293–7299 CrossRef CAS.
- S. Mondal, B. C. Patra and A. Bhaumik, ChemCatChem, 2017, 9, 1469–1475 CrossRef CAS.
- C. G. Evans and J. E. Gestwicki, Org. Lett., 2009, 11, 2957–2959 CrossRef CAS PubMed.
- Z. Zarnegar, J. Safari and Z. M. Kafroudi, New J. Chem., 2015, 39, 1445–1451 RSC.
- O. Goli-Jolodar, F. Shirini and M. Seddighi, RSC Adv., 2016, 6, 26026–26037 RSC.
- T. K. Saha and R. Das, ChemistrySelect, 2018, 3, 147–169 CrossRef CAS.
- F. Xiao, Y. Chen, Y. Liu and J. Wang, Tetrahedron, 2008, 64, 2755–2761 CrossRef CAS.
- N. Shachat and J. J. Bagnell, J. Org. Chem., 1963, 28, 991–995 CrossRef.
- C. G. Overberger and J.-P. Anselme, J. Org. Chem., 1963, 28, 592–593 CrossRef CAS.
- B. Yan and Y. Liu, Org. Lett., 2007, 9, 4323–4326 CrossRef CAS PubMed.
- E.-S. Lee, H.-S. Yeom, J.-H. Hwang and S. Shin, Eur. J. Org. Chem., 2007, 2007, 3503–3507 CrossRef.
- C. Jiang, M. Xu, S. Wang, H. Wang and Z.-J. Yao, J. Org. Chem., 2010, 75, 4323–4325 CrossRef CAS PubMed.
- D. Shibata, E. Okada, J. Molette and M. Médebielle, Tetrahedron Lett., 2008, 49, 7161–7164 CrossRef CAS.
- Y. Yamamoto, H. Hayashi, T. Saigoku and H. Nishiyama, J. Am. Chem. Soc., 2005, 127, 10804–10805 CrossRef CAS PubMed.
- D. F. Harvey and D. M. Sigano, J. Org. Chem., 1996, 61, 2268–2272 CrossRef CAS.
- F. J. Fañanás, T. Arto, A. Mendoza and F. Rodríguez, Org. Lett., 2011, 13, 4184–4187 CrossRef PubMed.
- T. S. Symeonidis, M. G. Kallitsakis and K. E. Litinas, Tetrahedron Lett., 2011, 52, 5452–5455 CrossRef CAS.
- M. Naoi, W. Maruyama, Y. Akao, H. Yi and Y. Yamaoka, in Oxidative Stress and Neuroprotection, ed. H. Parvez and P. Riederer, Springer Vienna, Vienna, 2006, pp. 67–77, DOI:10.1007/978-3-211-33328-0_8.
- J. Leegwater-Kim and E. Bortan, Clin. Interventions Aging, 2010, 5, 149–156 CrossRef CAS PubMed.
- V. A. Peshkov, O. P. Pereshivko, A. A. Nechaev, A. A. Peshkov and E. V. Van der Eycken, Chem. Soc. Rev., 2018, 47, 3861–3898 RSC.
- H. T. Nguyen, N. P. T. Le, D. K. N. Chau and P. H. Tran, RSC Adv., 2018, 8, 35681–35688 RSC.
- M. M. Hooper and B. DeBoef, J. Chem. Educ., 2009, 86, 1077 CrossRef CAS.
- M. Shiri, Chem. Rev., 2012, 112, 3508–3549 CrossRef CAS PubMed.
- S. R. Cherkupally and R. Mekala, Chem. Pharm. Bull., 2008, 56, 1002–1004 CrossRef CAS PubMed.
- L. S. K. Gadekar, S. Santosh, S. R. Mane, B. R. Arbad and M. K. Lande, Bull. Korean Chem. Soc., 2009, 30, 2532–2534 CrossRef CAS.
- A. Mobinikhaledi, N. Foroughifar, M. A. B. Fard, H. Moghanian, S. Ebrahimi and M. Kalhor, Synth. Commun., 2009, 39, 1166–1174 CrossRef CAS.
- R. T. Behrooz Maleki, M. Kermanian and S. S. Ashrafi, J. Mex. Chem. Soc., 2013, 57, 290–297 Search PubMed.
- H. Khabazzadeh, E. T. Kermani, D. Afzali, A. Amiri and A. Jalaladini, Arabian J. Chem., 2012, 5, 167–172 CrossRef CAS.
- M. Lakshmi Kantam, S. Laha, J. Yadav and S. Bhargava, Tetrahedron Lett., 2008, 49, 3083–3086 CrossRef.
- M. J. Albaladejo, F. Alonso, Y. Moglie and M. Yus, Eur. J. Org. Chem., 2012, 2012, 3093–3104 CrossRef CAS.
- H.-B. Chen, Y. Zhao and Y. Liao, RSC Adv., 2015, 5, 37737–37741 RSC.
- S. Cheng, N. Shang, C. Feng, S. Gao, C. Wang and Z. Wang, Catal. Commun., 2017, 89, 91–95 CrossRef CAS.
- J. Dulle, K. Thirunavukkarasu, M. C. Mittelmeijer-Hazeleger, D. V. Andreeva, N. R. Shiju and G. Rothenberg, Green Chem., 2013, 15, 1238–1243 RSC.
- S. Nazari, S. Saadat, P. K. Fard, M. Gorjizadeh, E. R. Nezhad and M. Afshari, Monatsh. Chem., 2013, 144, 1877–1882 CrossRef CAS.
- H. T. Nguyen, N.-P. Thi Le, D.-K. Nguyen Chau and P. H. Tran, RSC Adv., 2018, 8, 35681–35688 RSC.
Footnote |
† Electronic supplementary information (ESI) available. See DOI: 10.1039/d0ra04008h |
|
This journal is © The Royal Society of Chemistry 2020 |
Click here to see how this site uses Cookies. View our privacy policy here.