DOI:
10.1039/D0RA05848C
(Paper)
RSC Adv., 2020,
10, 31022-31026
Palladium nanoparticles as efficient catalyst for C–S bond formation reactions†
Received
4th July 2020
, Accepted 14th August 2020
First published on 21st August 2020
Abstract
The development of green, economical and sustainable chemical processes is one of the primary challenges in organic synthesis. Herein, we report an efficient and heterogeneous palladium-catalyzed sulfonylation of vinyl cyclic carbonates with sodium sulfinates via decarboxylative cross-coupling. Both aliphatic and aromatic sulfinate salts react with various vinyl cyclic carbonates to deliver the desired allylic sulfones featuring tri- and even tetrasubstituted olefin scaffolds in high yields with excellent selectivity. The process needs only 2 mol% of Pd2(dba)3 and the in situ formed palladium nano-particles are found to be the active catalyst.
Introduction
The development of green, economical and sustainable organic processes that can be performed under ambient conditions in which the desired products can be obtained by the utilization of simple and readily available starting materials is one of the most important objectives in organic synthesis. Efforts to develop green processes and our ongoing interest in nano-catalysis, we focused to develop a practical and effective method for the formation of allylic sulfones since they have an important role in pharmaceuticals and biologically active drug agents,1 such as anticancer,1a antibacterial,1b and weed control herbicides.1c Moreover, there have been several reports on the use of substituted allylic sulfones in asymmetric catalytic processes, providing useful synthesis of chiral sulfones.2 Therefore; the development of effective methodologies to synthesize allylic sulfones has drawn considerable interest both in organic reactions3 and in the pharmaceutical industry.4 Reported methods included the use of transition metal-catalysed allylic substitution,5 nucleophilic/radical addition,6 hydrosulfination of allenes,7 or alkynes,8 and many others9 by using toxic and expensive ligands. From the view point of economy and environmental concerns, the development of greener synthetic procedures using readily available, environmentally benign and inexpensive feedstock is highly appealing.
Since the initial reports,10 many groups have found interest in vinyl cyclic carbonate chemistry to develop useful synthetic procedures for the synthesis of valuable building blocks.11 Among them, Kleij and Yang's et al. have together reported the homogeneous palladium-catalysed thiolation, sulfonylation of vinyl cyclic carbonates to form allylic sulfones (Scheme 1a).12 Regardless of their importance, these methods have few drawbacks. For example, the need of the bulky ligands to accomplish smooth conversions and the use of odorous thiols makes them limited towards the green and economical synthesis. The fast-growing field of palladium-nanocatalysis13 could solve these issues, which has increasing interest in both industry field and organic reactions, due to its stability, recyclability and unique reactivity. Furthermore, two-dimensional nano-materials has become an important platform to design efficient single site catalysts for various applications, such as, CO2 reduction and CO oxidation etc.14
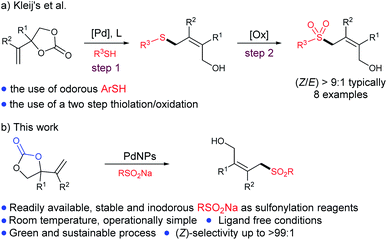 |
| Scheme 1 Cross coupling of vinyl cyclic carbonates for C–S bond formation. (a) Homogeneous catalysis (b) heterogeneous catalysis. | |
In this context, we have proposed a “green”, so-called “ligandless”, palladium nano-catalyst, which has shown good reactivity without the addition of any external ligand or stabilizer. We planned to examine the catalytic activity of PdNPs in C–S bond formation reactions, since very few reports are available for nanoparticle-catalyzed C–S bond formation,15 and, to the best of our knowledge, no report is existing for PdNPs-catalyzed C–S bond formation reaction, which involves no additional stabilizer and or external ligand at ambient temperature.16 In our continuing interest in green chemistry17 and efforts towards the formation of quaternary all-carbon centers,18 we herein report an external ligand-free palladium-catalyzed C–S bond formation reaction for the synthesis of allylic sulfones featuring tri- and or tetrasubstituted olefin scaffold in excellent yields and stereoselectivities at room temperature (Scheme 1b).
Results and discussion
With the expectation to obtain trisubstituted allylic sulfone 3aa, we selected vinyl cyclic carbonate 1a and sodium benzenesulfinate 2a as model substrates. To our delight, the expected product 3aa can be obtained in 12% isolated yield when the reaction was conducted in THF at room temperature using PdCl2 as a catalyst (Table 1, entry 1). The effect of palladium-catalysts was screened, with the attempt to improving the yield and selectivity. Different palladium-catalysts were used under ligand free conditions at room temperature (Table 1, entries 2–4). Among them, only Pd2(dba)3 provided the desired trisubstituted allylic sulfone at an 92% isolated yield with >19
:
1 Z/E selectivity (Table 1, entry 4). The reaction was also performed in pure water, but only 42% of the desired product was isolated (Table 1, entry 5). The reaction efficiency did not improve further in other solvents (Table 1, entries 6–9). Furthermore, no desired product was detected when sodium benzenesulfinate was replaced by benzenesulfinic acid, which means the reaction worked as a simple nucleophilic addition.
Table 1 Optimization of the reaction conditionsa
With the optimized reaction condition (Table 1, entry 4), the generality of the substrate scope of the decarboxylative cross-coupling reaction was investigated and the results are summarized in Table 2. A variety of sodium sulfinates (2) reacted effectively to provide the desired allylic sulfones (3) in high yield with excellent (Z)-selectivity. Both electron-donating and electron-withdrawing substituents on phenyl ring of sulfinate salts could react with vinyl cyclic carbonate 1a, affording the corresponding products in excellent yields and stereoselectivities (Table 2, entries 2–12). The (Z)-configuration of allylic sulfone 3ab was confirmed by X-ray analysis (see ESI† for details). In addition, sulfinate salts with bulky naphthyl and heteroaryl moieties were also tolerated under the optimized conditions supported by the formation of products 3am–3ao (Table 2, entries 13–15).
Table 2 Sodium sulfinate substrate scopea
Furthermore, it is noteworthy that the alkyl sulfinate salts, which has posed great challenge in the synthesis of allylic sulfones due to their low reactivity, were also suitable nucleophiles in our reaction conditions.5–9 Both primary and secondary alkyl sulfinate salts worked well under the optimized condition providing the corresponding allylic sulfones in high yield with excellent regioselectivities (Table 2, entries 16–19). Notably, upon employing a more functionalized sodium sulfinate 2t as the sulfonylation partner, the linear allylic sulfone 3at was isolated in 82% yield with 10
:
1 (Z)-selectivity. The reaction leading to allylic sulfone 3aa was easily scaled up to gram scale without any loss of the stereoselectivity and yield (5 mmol scale: 1.3 g, 90% yield, >19
:
1 Z/E, Table 2, entry 1).
As described in Table 3, various vinyl cyclic carbonates were tested under the optimized conditions to further examine the reaction scope. A variety of phenyl-substituted vinyl cyclic carbonates, with different electronic and steric properties, were tolerated to provide the desired allylic sulfones (3ba–3na) in high yields with excellent stereoselectivities. Vinyl cyclic carbonates with bulky naphthyl group also worked well to provide allylic sulfones 3oa and 3pa in high yields with excellent (Z)-selectivity. Vinyl cyclic carbonate with versatile furan moiety also reacted smoothly to furnish the desired product 3qa in high yield with high stereoselectivity (86% yield, 15
:
1 Z/E).
Table 3 Vinyl cyclic carbonate substrate scopea,b,c
Reaction conditions: 1a (0.20 mmol), 2 (0.30 mmol), Pd2(dba)3 (2 mol%), THF (1.0 mL), rt, 15 h. The yields are of isolated materials for the stereoisomers. Determined by 1H NMR of crude reaction mixture. The (Z)-configuration of 3ab was determined by X-ray crystallography, those of the other products were assigned by analogy (see ESI for more details). |
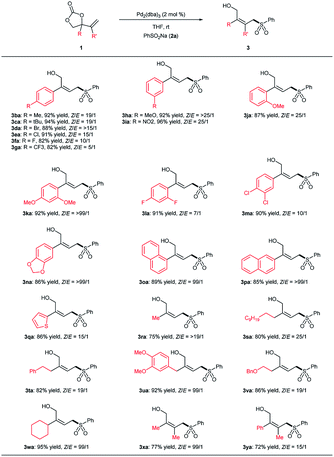 |
Furthermore, the conditions were also suitable for the reaction of alkyl substituted vinyl cyclic carbonates (2ra–2ya), providing the corresponding products in high yields and excellent stereoselectivities. In the case of methyl-substituted cyclic carbonate 1r, the formation of branched allylic sulfone was observed which affected the linear product yield. With vinyl carbonate bearing longer alkyl chain, the expected allylic sulfone was also obtained in high yield and regioselectivity (80% yield, >25
:
1 Z/E, 3sa). Vinyl cyclic carbonates 1t, 1u and 1v that have different substituents in the alkyl chain were allowed to react with sulfinate salt 2a, good yield and stereoselectivity were obtained (3ta, 3ua and 3va). In addition, cyclic carbonate with cyclohexyl-substituent afforded the desired product in high yield (95% yield, 3wa) with excellent (Z)-selectivity. Furthermore, the substitution of alkyl group (R = Me) at the β-position of the olefin scaffold is workable with excellent stereoselectivity (3xa and 3ya).
To demonstrate the synthetic use of the present methodology, gram scale synthesis was carried out (Fig. 1a). Compound 3aa was synthesized from 1a and 2a (5.0 mmol scale) over Pd2(dba)3 (2 mol%) at an 90% yield (1.3 g). At the end of reaction, the mixture was filtered and washed to give a dark black solid, from which palladium nano-particles were observed with size of 5–70 nm upon TEM analysis.19 Furthermore, we evaluated the recyclability of these free-formed palladium nano-particles, replacing Pd2(dba)3, showing a small decline in the catalytic activity in a test reaction (Fig. 1b) which clearly indicate that catalyst is free from any sort of agglomeration. Notably, a small decrease in the recovered PdNPs might be attributed due to workup and recovery procedures.
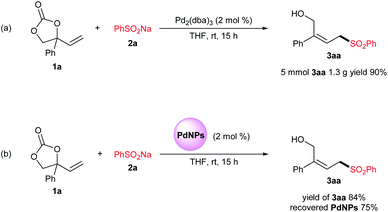 |
| Fig. 1 (a) Gram-scale synthesis and (b) recyclability of PdNPs. | |
In order to gain insight into the reaction mechanism, especially for the high activity of the palladium catalyst, transmission electron microscopy (TEM) analysis was carried out in this decarboxylative cross coupling reaction.19 The Pd(0) precursor serve as source of PdNPs due to the decomposition of Pd2(dba)3 in the solid state to release (dba) and formation of Pd black: Pd2(dba)3 → dba + [Pd].20 Analysis by TEM confirmed the presence of palladium nano-particles with dimensions of 5–70 nm as shown in Fig. 2. These results revealed that Pd2(dba)3 was reduced to form palladium nano-particles and the PdNPs are likely stabilized by dba.
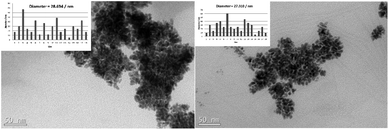 |
| Fig. 2 TEM images of PdNPs for the coupling of 1a with 2a in THF. A sample taken after 30 minutes (right). A sample taken after 15 hours (left). | |
Conclusions
In conclusion we have developed a palladium nanoparticle catalyzed cross-coupling of vinyl cyclic carbonates with sodium sulfinates under external ligand free conditions and without the addition of any stabilizer at room temperature. We are able to construct allylic sulfones in high yield and (Z)-selectivity. We found that a broad range of substitution is tolerated on either coupling partner. Taken together with its cost efficient, environmentally friendly, broad substrate scope, chemo-selectivity, readily available and stable starting materials and operational simplicity, this green methodology will find practical use in synthetic setting.
Conflicts of interest
The authors declare no conflicts of interest.
Acknowledgements
This work was supported by the “Fundamental Research Funds for Central Universities” (No. 1191329135), Key Laboratory Construction Program of Xi'an Municipal Bureau of Science and Technology (No. 201805056ZD7CG40) and the starting fund of Xi'an Jiao Tong University (No. 7121182002). We thank the Instrumental Analysis Center of Xi'an Jiao Tong University for HRMS analysis.
Notes and references
-
(a) C. Pabba, B. T. Gregg, D. B. Kitchen, Z. J. Chen and A. Judkins, Bioorg. Med. Chem. Lett., 2011, 21, 324 CrossRef CAS PubMed
;
(b) F. Reck, B. F. Zhou, M. Girardot, G. Kern, C. J. Eyermann, N. J. Hales, R. R. Ramsay and G. W. Gravestock, J. Med. Chem., 2005, 48, 499 CrossRef CAS PubMed
;
(c) N. Neamati, G. W. Kabalka, B. Venkataiah and R. W. O. Dayam, US Pat., 0203224, 2007
;
(d) M. Muehlebach, W. Lutz, J. Wenger, J. Finney, C. J. Mathews and D. W. O. Fawke, WO patent, 110308, 2008
;
(e) N. A. Mcgrath, M. Brichacek and J. T. Njardarson, J. Chem. Educ., 2010, 87, 1348 CrossRef CAS
. -
(a) K. M. H. Lim and T. J. Hayashi, J. Am. Chem. Soc., 2012, 134, 5056 CrossRef PubMed
;
(b) W. J. Yue, C. Y. Zhang and L. Yin, Science, 2019, 14, 88 CAS
;
(c) T. Zhou, B. K. Peters, M. F. Maldonado, T. Govender and P. G. Andersson, J. Am. Chem. Soc., 2012, 134, 13592 CrossRef CAS PubMed
;
(d) B. K. Peters, T. Zhou, J. Rujirawanich, A. Cadu, T. Singh, W. Rabten, S. Kerdphon and P. G. Andersson, J. Am. Chem. Soc., 2014, 136, 16557 CrossRef CAS PubMed
;
(e) K. M. H. Lim and T. Hayashi, J. Am. Chem. Soc., 2015, 137, 3201 CrossRef CAS PubMed
. - For the application of allylic sulfones in organic synthesis, see:
(a) A. E. Awa, M. N. Noshi, X. M. Jourdin and P. L. Fuchs, Chem. Rev., 2009, 109, 2315 CrossRef PubMed
;
(b) P. Koschker and B. Breit, Acc. Chem. Res., 2016, 49, 1524 CrossRef CAS PubMed
;
(c) V. T. Nguyen, H. T. Dang, H. H. Phan, V. D. Nguyen, C. F. Hansen, H. D. Arman and O. V. Larionov, J. Am. Chem. Soc., 2018, 140, 8434 CrossRef CAS PubMed
;
(d) S. Kamijo, K. Kamijo, K. Maruoka and T. Murafuji, Org. Lett., 2016, 18, 6516 CrossRef CAS PubMed
;
(e) M. Y. Chang, M. H. Wu and Y. L. Chen, Org. Lett., 2013, 15, 2822 CrossRef CAS PubMed
;
(f) S. G. Pyne, D. M. David and Z. Dong, Tetrahedron Lett., 1998, 39, 8499 CrossRef CAS
;
(g) K. Anczkiewicz, M. Krolikiewicz, Z. Wrobel and K. Wojciechowski, Tetrahedron, 2015, 71, 3924 CrossRef CAS
. - For the application of allylic sulfones in drugs and related pharmaceuticals, see:
(a) T. Wang, A. Riegger, M. Lamla, S. Wiese, P. Oeckl, M. Otto, Y. Wu, S. Fischer, H. Barth, S. L. Kuan and T. Weil, Chem. Sci., 2016, 7, 3234 RSC
;
(b) H. G. F. Richter, P. Angehrn, C. Hubschwerlen, M. Kania, J.-L. Specklin and F. K. Winkler, J. Med. Chem., 1996, 39, 3712 CrossRef CAS PubMed
. - For the synthesis of allylic sulfones via allylic substitution, see:
(a) B. M. Trost and N. R. Schmuff, J. Am. Chem. Soc., 1985, 107, 396 CrossRef CAS
;
(b) M. Jegelka and B. Plietker, Chem.–Eur. J., 2011, 17, 10417 CrossRef CAS PubMed
;
(c) X.-S. Wu, Y. Chen, M.-B. Li, M.-G. Zhou and S.-K. Tian, J. Am. Chem. Soc., 2012, 134, 14694 CrossRef CAS PubMed
;
(d) S. Chandrasekhar, V. Jagadeshwar, B. Saritha and C. Narsihmulu, J. Org. Chem., 2005, 70, 6506 CrossRef CAS PubMed
;
(e) F.-X. Felpin and Y. Landais, J. Org. Chem., 2005, 70, 6441 CrossRef CAS PubMed
;
(f) M. Jegelka and B. Plietker, Org. Lett., 2009, 11, 3462 CrossRef CAS PubMed
;
(g) M. Billamboz, F. Mangin, N. Drillaud, C. Chevrin-Villette, E. Banaszak-Léonard and C. Len, J. Org. Chem., 2014, 79, 493 CrossRef CAS PubMed
;
(h) X.-T. Ma, R.-H. Dai, J. Zhang, Y. Gu and S.-K. Tian, Adv. Synth. Catal., 2014, 356, 2984 CrossRef CAS
;
(i) T.-T. Wang, F.-X. Wang, F.-L. Yang and S.-K. Tian, Chem. Commun., 2014, 50, 3802 RSC
. - For nucleophilic and radical addition for allylic sulfones, see:
(a) X.-Q. Chu, H. Meng, X.-P. Xu and S.-J. Ji, Chem.–Eur. J., 2015, 21, 11359 CrossRef CAS PubMed
;
(b) L. Rajender Reddy, B. Hu, M. Prashad and K. Prasad, Angew. Chem., Int. Ed., 2009, 48, 172 CrossRef PubMed
;
(c) X.-Q. Chu, H. Meng, X.-P. Xu and S.-J. Ji, Chem.–Eur. J., 2015, 21, 11359 CrossRef CAS PubMed
;
(d) X. Li, X. Xu and Y. Tang, Org. Biomol. Chem., 2013, 11, 1739 RSC
;
(e) G. Zhang, L. Zhang, H. Yi, Y. Luo, X. Qi, C.-H. Tung, L.-H. Wu and A. Lei, Chem. Commun., 2016, 52, 10407 RSC
;
(f) R. Mao, Z. Yuan, R. Zhang, Y. Ding, X. Fan and X. Wu, Org. Chem. Front., 2016, 3, 1498 RSC
;
(g) S. Parisotto, G. Garreffa, C. Canepa, E. Diana, F. Pellegrino, E. Priola, C. Prandi, V. Maurino and A. Deagostino, ChemPhotoChem, 2017, 1, 56 CrossRef CAS
;
(h) X. Li, X. Xu and C. Zhou, Chem. Commun., 2012, 48, 12240 RSC
;
(i) J. Zhang, K. Zhou, G. Qiu and J. Wu, Org. Chem. Front., 2019, 6, 36 RSC
. - For hydrosulfination of allene, see:
(a) S. Kamijo, M. Al-Masum and Y. Yamamoto, Tetrahedron Lett., 1998, 39, 691 CrossRef CAS
;
(b) V. Khakyzadeh, Y. Wang and B. Breit, Chem. Commun., 2017, 53, 4966 RSC
. - For hydrosulfination of alkynes, see:
(a) K. Xu, V. Khakyzadeh, T. Bury and B. Breit, J. Am. Chem. Soc., 2014, 136, 16124 CrossRef CAS PubMed
;
(b) C.-J. Lu, H. Chen, D.-K. Chen, H. Wang, Z.-P. Yang, J. Gao and H. Jin, Org. Biomol. Chem., 2016, 14, 10833 RSC
. - For the synthesis of allylic sulfones through other types, see:
(a) H. M. Sheldrake and T. W. Wallace, Tetrahedron Lett., 2007, 48, 4407 CrossRef CAS
;
(b) P.-X. Zhou, Y.-Y. Ye, L.-B. Zhao, J.-Y. Hou, X. Kang, D.-Q. Chen, Q. Tang, J.-Y. Zhang, Q.-X. Huang, L. Zheng, J.-W. Ma, P.-F. Xu and Y.-M. Liang, Chem.–Eur. J., 2014, 20, 16093 CrossRef CAS PubMed
;
(c) J. Li, G. Qin, Y. Liu and H. Huang, Org. Chem. Front., 2016, 3, 259 RSC
;
(d) Y. Hou, Q. Shen, L. Zhu, Y. Han, Y. Zhao, M. Qin and P. Gong, RSC Adv., 2017, 7, 50372 RSC
;
(e) P.-X. Zhou, Y. Zhang, C. Ge, Y.-M. Liang and C. Li, J. Org. Chem., 2018, 83, 4762 CrossRef CAS PubMed
;
(f) C. Liu and Q. Wang, Angew. Chem., Int. Ed., 2018, 57, 4727 CrossRef CAS PubMed
;
(g) F. Xiao, C. Liu, D. Wang, H. Huang and G.-J. Deng, Green Chem., 2018, 20, 973 RSC
;
(h) A. Stikute, J. Luginina and M. Turks, Tetrahedron Lett., 2017, 58, 2727 CrossRef CAS
;
(i) X. Liu, X. Chen and J. T. Mohr, Org. Lett., 2015, 17, 3572 CrossRef CAS PubMed
. - For the initial work on substituted vinyl cyclic carbonates, see:
(a) A. Khan, R. Zheng, Y. Kan, J. Ye, J. Xing and Y. J. Zhang, Angew. Chem., Int. Ed., 2014, 53, 6439 CrossRef CAS PubMed
;
(b) A. Khan, L. Yang, J. Xu, L. Y. Jin and Y. J. Zhang, Angew. Chem., Int. Ed., 2014, 53, 11257 CrossRef CAS PubMed
;
(c) A. Khan, J. Xing, J. Zhao, Y. Kan, W. Zhang and Y. J. Zhang, Chem.–Eur. J., 2015, 21, 120 CrossRef CAS PubMed
. - For selected original examples, see:
(a) A. Khan, I. Khan, S. Khan, C. Zhao, Y. Mao, Y. Chen and Y. J. Zhang, J. Am. Chem. Soc., 2017, 139, 10733 CrossRef CAS PubMed
;
(b) A. Cai, W. Guo, L. Martínez-Rodríguez and A. W. Kleij, J. Am. Chem. Soc., 2016, 138, 14194 CrossRef CAS PubMed
;
(c) W. Guo, J. E. Kuniyil, J. E. Gómez, F. Maseras and A. W. Kleij, J. Am. Chem. Soc., 2018, 140, 3981 CrossRef CAS PubMed
;
(d) Z. Q. Rong, L. C. Yang, S. Liu, Z. Yu, Y. N. Wang, Z. Y. Tan, R. Z. Huang, Y. Lan and Y. Zhao, J. Am. Chem. Soc., 2017, 139, 15304 CrossRef CAS PubMed
;
(e) L. C. Yang, Z. Q. Rong, Y. N. Wang, Z. Y. Tan, M. Wang and Y. Zhao, Angew. Chem., Int. Ed., 2017, 56, 2927 CrossRef CAS PubMed
;
(f) S. Singa, T. Patra, C. G. Daniluc and F. Glorius, J. Am. Chem. Soc., 2018, 140, 3551 CrossRef PubMed
. -
(a) J. E. Gomez, W. Guo and A. W. Kleij, Org. Lett., 2016, 18, 6042 CrossRef CAS PubMed
;
(b) L. Deng, A. W. Kleij and W. Yang, Chem.–Eur. J., 2018, 24, 19156 CrossRef CAS PubMed
. - For selected reviews on palladium nano-catalysis, see:
(a) Á. Molnár, Chem. Rev., 2011, 111, 2251 CrossRef PubMed
;
(b) L. Yin and J. Liebscher, Chem. Rev., 2007, 107, 133 CrossRef CAS PubMed
;
(c) C. Deraedt and D. Astruc, Acc. Chem. Res., 2014, 47, 494 CrossRef CAS PubMed
;
(d) A. Fihri, M. Bouhrari, B. Nekoueishahraki, J.-M. Basset and V. Polshettiwar, Chem. Rev., 2011, 40, 5181 CAS
;
(e) A. Balanta, C. Godard and C. Claver, Chem. Soc. Rev., 2011, 40, 4973 RSC
;
(f) P. K. Verma, M. L. Shegavi, S. K. Bose and K. Geetharani, Org. Biomol. Chem., 2018, 16, 857 RSC
;
(g) M. O. Sydnes, Curr. Org. Chem., 2014, 18, 312 CrossRef CAS
;
(h) A. Bej, K. Ghosh, A. Sarkar and D. W. Knight, RSC Adv., 2016, 6, 11446 RSC
. - For selected examples on 2-dimentional material nano-catalysts, see:
(a) B. Song, Y. Zhou, H.-M. Yang, J.-H. Liao, L.-M. Yang, X.-B. Yang and E. Ganz, J. Am. Chem. Soc., 2019, 141, 3630 CrossRef CAS PubMed
;
(b) L.-M. Yang, V. Bačić, I. A. Popov, A. I. Boldyrev, T. Heine, T. Frauenheim and E. Ganz, J. Am. Chem. Soc., 2015, 137, 2757 CrossRef CAS PubMed
;
(c) J.-H. Liu, L.-M. Yang and E. Ganz, ACS Sustainable Chem. Eng., 2018, 6, 11 Search PubMed
;
(d) J.-H. Liu, L.-M. Yang and E. Ganz, J. Mater. Chem. A, 2019, 7, 11944 RSC
. - For general metal-nanoparticle catalysed C–S bond formation, see:
(a) Y. S. Panova, A. S. Kashin, M. G. Vorobev, E. S. Degtyareva and V. P. Ananikov, ACS Catal., 2016, 6, 3637 CrossRef CAS
;
(b) B. C. Ranu, A. Saha and R. Jana, Adv. Synth. Catal., 2007, 349, 2690 CrossRef CAS
;
(c) A. Y. Mitrofanov, A. V. Murashkina, I. Martín-García, F. Alonso and I. P. Beletskaya, Catal. Sci. Technol., 2017, 7, 4401 RSC
;
(d) Z. Taherinia and A. Ghorbani-Choghamarani, Can. J. Chem., 2019, 97, 46 CrossRef CAS
;
(e) A. Ghorbani-Choghmarani and Z. Taherinia, New J. Chem., 2017, 41, 9414 RSC
. - For palladium nano-particle catalysed C–S bond formation, see:
(a) R. Hemelaere, J. Desroches and J.-F. Paquin, Org. Lett., 2015, 17, 1770 CrossRef CAS PubMed
;
(b) A. Ghorbani-Choghmarani and Z. Taherinia, RSC Adv., 2016, 6, 59410 RSC
. -
(a) A. Khan, J. Zhang and S. Khan, Green Chem., 2020, 22, 4116 RSC
;
(b) Y. Mao, X. Zhai, A. Khan, J. Cheng, X. Wu and Y. J. Zhang, Tetrahedron Lett., 2016, 57, 3268 CrossRef CAS
. -
(a) M. Salman, Y. Xu, S. Khan, J. Zhang and A. Khan, Chem. Sci., 2020, 11, 5481 RSC
;
(b) A. Khan, M. Zhang and S. Khan, Angew. Chem., Int. Ed., 2020, 59, 1340 CrossRef CAS PubMed
. - See ESI† for more details.
-
(a) S. S. Zalesskiy and V. P. Ananikov, Organometallics, 2012, 31, 2302 CrossRef CAS
;
(b) D. N. Leonard and S. J. Franzen, J. Phys. Chem. C, 2009, 113, 12706 CrossRef CAS
.
Footnote |
† Electronic supplementary information (ESI) available. See DOI: 10.1039/d0ra05848c |
|
This journal is © The Royal Society of Chemistry 2020 |
Click here to see how this site uses Cookies. View our privacy policy here.