DOI:
10.1039/D0RA06147F
(Paper)
RSC Adv., 2020,
10, 37439-37448
Simultaneous estimation of dimenhydrinate, cinnarizine and their toxic impurities benzophenone and diphenylmethylpiperazine; in silico toxicity profiling of impurities
Received
14th July 2020
, Accepted 24th September 2020
First published on 9th October 2020
Abstract
The British Pharmacopeia (BP) reported that the carcinogenic and hepatotoxic, benzophenone (BZP) is a dimenhydrinate (DMH) impurity. On the other hand, cinnarizine (CIN) is reported to have five impurities (A–E). The toxicity profile of CIN impurities was studied and the in silico data revealed that impurity A [1-(diphenylmethyl)piperazine] (DPP) was the most toxic CIN impurity, and hence it was selected during this work. TLC-densitometric method was developed for separation and simultaneous quantitation of DMH, CIN and their toxic impurities. In the proposed method hexane
:
ethanol
:
acetone
:
glacial acetic acid (7
:
3
:
0.7
:
0.5, by volume) with UV scanning at 225 nm were used. Method validation was carried out according to ICH guidelines and linearity was achieved in the range 0.2–4, 0.5–5, 0.1–2.0, and 0.05–2.2 μg per band for DMH, CIN, BZP and DPP, respectively. On the application of the method to pharmaceutical formulation, no interference from additives was observed. The greenness of the method was evaluated using the analytical eco-scale and the results revealed the low negative environmental impact of the developed method.
1. Introduction
Dimenhydrinate (DMH), is the theoclate salt of diphenhydramine (DIP) that is chemically named, 1-((2-(benzhydryloxy)ethyl)(methyl)amino)-8-chloro-3-methyl-3,7-dihydro-1H-purine-2,6-dione, (Fig. 1a).1 DMH has antihistaminic and antimuscarinic activities with significant sedative and addictive effects.1–3 Furthermore, the antiemetic DMH is traditionally used in the treatment of motion sickness and peripheral vestibular vertigo.4
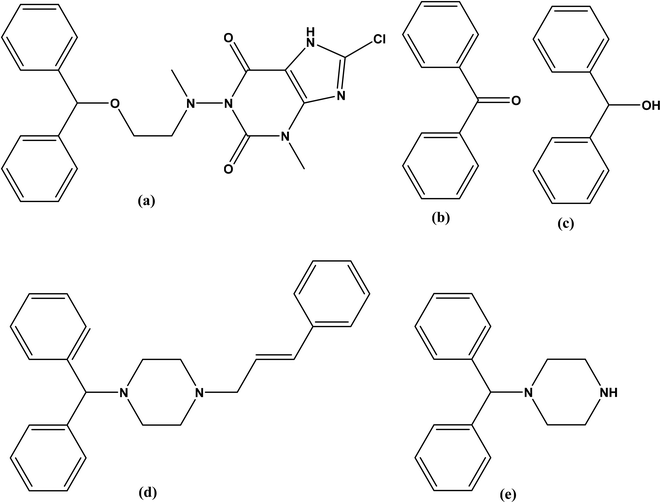 |
| Fig. 1 Chemical structures of (a) dimenhydrinate, (b) benzophenone (dimenhydrinate impurity). (c) benzhydrol, (d) cinnarizine and, (e) 1-diphenylmethyl piperazine (cinnarizine impurity). | |
Although DMH has several official impurities,5 only toxicity assessment was available for benzophenone (BZP), (Fig. 1b) and benzhydrol (Fig. 1c). Benzophenone is a precursor of DMH that is reported to cause cancer6,7 and hepatocellular necrosis7,8 in addition to its potential toxicity on aquatic life.9 Reviewing the reported toxicity studies of BZP and BZH revealed higher and wider toxic effects for BZP.10–13
Cinnarizine (CIN), 1-(diphenylmethyl)-4-(3-phenyl-2-propenyl)piperazine (Fig. 1d) is a piperazine derivative with antihistaminic and calcium channel blocking activities. It improves the cerebral blood flow therefore, it is used in the treatment of cerebral arteriosclerosis, cerebral apoplexy, and post-traumatic cerebral signs. Additionally, CIN is used for the management of nausea, vomiting, vertigo, motion sickness, and Meniere's disease.14–16
British Pharmacopeia (BP)5 stated that CIN has several impurities (A–E). Till now, the toxicity of these impurities is not studied in any published article. The compound 1-(diphenylmethyl)piperazine (DPP) shown in Fig. 1e, is the impurity A of CIN.
Recently, providing all the necessary information about impurities and harmful effects on human health gained immense interest during the development of new drug molecules. The presence of these undesirable substances, even in trifling quantity may affect the safety and potency of pharmaceutical products.17 Toxicity profiling of impurities is laborious, time-consuming, expensive, and of ethical concerns, from this point, many computational approaches for virtual toxicity profiling were developed, they collect databases containing ADME and toxicities data to train physiologically-based pharmacokinetics model and toxicity predictions.18 Nowadays, numerous in silico-ADMET/Tox softwares are widely used which saves time and cost.
The environmental consciousness increased during the last few decades leading to emerging of the green analytical chemistry concept. The main principles of this concept are the reduction or elimination of the use and the generation of hazardous substances during the whole analytical procedures. Various green chemistry metrics are developed to evaluate the greenness criteria of such procedures. Eco-scale is a semiquantitative tool proposed by Van Aken et al.19 which is used to evaluate different analytical parameters: the amount of reagents, their hazards, energy, and waste.20
Potentiometric and argentometric titration were recommended for the determination of DMH in BP5 and EP,21 respectively. For the same purpose, USP22 described an HPLC method using a mobile phase of ammonium acetate: methanol (80
:
20 v/v) flowed at a rate of 1.5 mL min−1 with UV detection at 229 nm. Additionally, BP5 and European Pharmacopeia (EP)21 developed a potentiometric titration method for estimation of CIN. However, the DMH and CIN combination is not officially included in any pharmacopeia.
A literature survey revealed that DMH was analyzed by spectrophotometric,23 electrochemical24,25 and by chromatographic methods.26–28 Similarly, CIN was determined spectrophotometrically,29,30 electrochemically31,32 and by different chromatographic methods33–39 either alone or in combination with other drugs and related impurities. On the other hand, DMH and CIN were simultaneously assayed by spectrophotometric,40,41 TLC-densitometric42,43 and HPLC43,44 methods. Moreover, a ternary mixture of DMH, CIN and DPP was analyzed by TLC-densitometric45 and HPLC45 methods.
Benzophenone, was reported to have the highest toxicity among the DMH impurities. However, no available literature for the toxicity assessment of CIN impurities (A–E). Thus, this work aims to use PreADMET application for the first time to expect the toxicity profile of CIN impurities (A–E). The TLC-densitometry is considered a sensitive, accurate, and reliable analytical tool that is cost and time effective.46,47 Additionally, no method was reported for the simultaneous determination of DMH, CIN, and their impurities (BZP and DPP). Hence, the current work was also aimed to develop a TLC-densitometric for simultaneous determination of DMH, CIN, and their toxic impurities. The method validation was carried out following ICH guidelines.48
2. Experimental
All experiments complies with the ýrelevant laws and institutional guidelines of Beni-Suef University and approved by ýthe Safety and Occupational Health Committee at Faculty of Pharmacy, Beni-Suef University.
2.1. Materials and reagents
Cinnarizine pure sample was obtained as a gift from Amoun Pharmaceuticals (Cairo, Egypt) with a purity of 100.26% according to the analysis results of the reported method,45 DMH was kindly supplied from Kahira Pharmaceuticals and Chemical Industries Company (Cairo, Egypt) with a purity of 99.10% according to the reported method.45 Benzophenone and DPP were purchased from Sigma Aldrich, Chemie GmbH (Darmstadt, Germany) with claimed purity of 97% and 99%, respectively according to the manufacturing certificates of analysis. Methanol, ethanol, acetone, hexane, methylene chloride, chloroform, and ethylacetate were of HPLC grade (Fisher, Loughborough, UK) and were purchased from Sigma Aldrich Chemie GmbH (Darmstadt, Germany). Orthophosphoric acid, glacial acetic acid, formic acid, triethylamine, and ammonia solution (33%) were bought from EL-Nasr pharmaceutical, Chemical Co., Abu Zabaal, Cairo, Egypt and were of analytical grade. Amocerebral® tablets, (batch no. 6221025030658) were manufactured by Amoun pharmaceutical company (Cairo, Egypt) each tablet was labeled to contain 20 mg of DMH and 10 mg of CIN.
2.2. Softwares
Toxicity profiling was carried out using version 2.0 of PreADMET online program software. The toxicity profiling includes testing of acute algae, daphnia and fish toxicity, Ames test for mutagenicity testing of several Salmonella typhimurium strains. Carcinogenicity testing is also included through 2 years carcinogenicity bioassay in rats and mice in addition to in vitro human ether-a-go-go related gene channel (hERG) inhibition testing.49
2.3. Solutions
(a) Stock standard solutions of DMH, BZP, CIN, and DPP were prepared by accurate weighing of 0.1 g of each and dissolving in methanol to obtain a 100 mL stock solution of 1 mg mL−1 each.
(b) The stock solution of Amocerebral® tablets was prepared by weighing the content of ten tablets, then grinding them and mixing the resulted powder well. An amount of the tablets powder equivalent to 50 mg DMH and 25 mg CIN was weighed and transferred to a 25 mL volumetric flask. Methanol (10 mL) was then added and the solution was ultrasonicated for 30 min, cooled, the volume was adjusted with methanol and finally, the solution was filtered.
2.4. Instruments
Linomat V applicator with a 100 μL syringe was used to apply the samples. The TLC scanner model 3S/N (Camag, Muttenz, Switzerland) was used for scanning and the scanner was controlled with WinCATS software (version 3.15). TLC aluminum plates (20 × 10 cm) coated with 0.25 mm Silica gel 60 F254 (Merck, Darmstadt, Germany) were used as a stationary phase. Also, a UV lamp with a short wavelength of 254 nm (Vilber Lourmat, Cedex, France) was used during the optimization of the developed method.
Digital balance (Sartorius, Germany) and Sonix TVSS-series ultrasonicator (CA, USA) were used.
2.5. Chromatographic conditions
TLC-densitometric method was conducted by applying the studied compounds to TLC plates (20 × 10 cm with 250 μm thickness and 5 μm particle size) as bands of 3 mm width using Camag Linomat-V applicator. The bands were applied at 5 mm intervals and 15 mm from the bottom edge of the plate. Linear ascending development was performed to 8 cm in a chromatographic jar that was previously saturated for 30 min with a solvent mixture of hexane
:
ethanol
:
acetone
:
glacial acetic acid (7
:
3
:
0.7
:
0.5, by volume) at a temperature of 25 °C and the separated bands were UV scanned at 225 nm.
2.6. Construction of calibration curves CIN, DPP, DMH and BZP
Different samples of DMH, BZP, CIN, and DPP in the range of 40–220, 10–200, 50–500, and 5–220 μg mL−1 were prepared in methanol in separate series of 10 mL volumetric flasks using their respective stock solutions (1 mg mL−1). 10 μL of each sample was applied in triplicate to TLC plates. Ascending development was carried out following the instructions listed above.
The calibration curves were constructed by plotting area under the peak against the corresponding analyte concentrations from which the regression equations were created.
2.7. Application to the pharmaceutical formulation
Different dilutions of Amocerebral® tablets within the linearity ranges of the developed method were prepared in methanol using the previously prepared stock solution (2 mg mL−1 DMH and 1 mg mL−1 CIN). The instructions followed for the construction of calibration curves were applied and the previously computed regression equations were used to calculate the concentrations of the studied drugs in the prepared samples. Additionally, the accuracy of the developed method was tested using the standard addition technique.
3. Results and discussion
Nowadays, developing a method for determination of drug-related impurities is attractive to many researchers especially when they exhibit certain toxicity. Although toxicity profiling is the cornerstone of the drug development process, it requires a huge amount of time, energy, and cost. That is why the use of computational tools for toxicity prediction is rapidly grown.
To date, no available analytical method for the simultaneous determination of DMH, CIN, and their pharmacopeial impurities. Besides, no toxicity profiling study was published for CIN impurities. Therefore, in the current work, the toxicity profiling of CIN impurities was studied using PreADMET application in addition to developing and optimizing a TLC-densitometric method for the simultaneous determination of the quaternary mixtures (DMH, BZP, CIN, and DPP). The greenness of the developed method was evaluated using the analytical eco-scale.
The developed method has the advantages of being novel, green, time and cost-effective. Besides, it more sensitive and selective in comparison to the reported method.45 Table 1.
Table 1 Comparison of the developed and reported methods for determination of the selected drugs
Method |
Linearity range (μg per band) or (μg mL−1) |
Mobile phase |
Comments |
Developed TLC |
DMH |
0.2–4.0 |
Hexane : ethanol : acetone : glacial acetic acid (7 : 3 : 0.7 : 0.5, v/v/v/v) |
The developed method has the advantages of: (1) toxicity profiling of CIN impurities. (2) Higher sensitivity comparing to other reported chromatographic methods. (3) Better selectivity as it simultaneously determined BZP with the ternary mixture of DMH, CIN and DPP. (4). Lower cost, time and solvent consumption in comparison to the reported HPLC method |
BZP |
0.1–2.0 |
CIN |
0.5–5.0 |
DPP |
0.05–2.2 |
Reported TLC41 |
DMH |
0.2–2.0 |
Chloroform : methanol : glacial acetic acid : ammonia solution (33%), (9.5 : 0.5 : 0.1 : 0.1, v/v/v/v) |
CIN |
0.4–1.6 |
DPP |
0.1–1.0 |
Reported HPLC41 |
DMH |
3.0–30.0 |
0.05 M KH2PO4 (pH = 3) : methanol (35 : 65, v/v) |
CIN |
2.0–20.0 |
DPP |
1.0–10.0 |
3.1. In silico toxicity profiling of cinnarizine impurities
Examination of the preADMET toxicity screening results for CIN and its impurities (A–E) are shown in Table 2. The results revealed that all impurities showed positive AMES mutagenicity to only one of salmonella strains except DPP which is a mutagen for both TA1535_10RLI and TA1535_NA strains. Moreover, DPP showed the highest toxicity against algae, daphnia, and fish. Additionally, all the impurities showed positive carcinogenicity for either rat, mice, or both. Also, all of them have a medium risk for hERG_ inhibition. From these results, we can conclude that DPP was the most toxic CIN impurity and hence it was selected as an example of CIN toxic impurities to be quantified using the developed TLC-densitometric method.
Table 2 Toxicity profiling of cinnarizine and its impurities using toxicity prediction of PreADMET application45
Compound |
CIN |
Imp A (DPP) |
Imp B |
Imp C |
Imp D |
Imp E |
Algae_at |
0.015 |
0.085 |
0.024 |
0.003 |
0.001 |
0.002 |
Ames_test |
Mutagen |
Mutagen |
Mutagen |
Mutagen |
Mutagen |
Mutagen |
Carcino_mouse |
+ |
− |
− |
+ |
+ |
− |
Carcino_rat |
+ |
+ |
+ |
+ |
− |
+ |
Daphnia_at |
0.008 |
0.153 |
0.046 |
0.001 |
0.000 |
0.003 |
hERG_inhibition |
Medium risk |
Medium risk |
Medium risk |
Medium risk |
Medium risk |
Medium risk |
Medaka_at |
0.000 |
0.035 |
0.003 |
1.11 × 10−6 |
8.17 × 10−7 |
2.00 × 10−4 |
Minnow_at |
0.002 |
0.080 |
0.020 |
0.001 |
4.470 × 10−5 |
3.00 × 10−4 |
TA100_10RLI |
− |
− |
+ |
+ |
− |
− |
TA100_NA |
− |
− |
− |
− |
− |
− |
TA1535_10RLI |
+ |
+ |
− |
− |
+ |
+ |
TA1535_NA |
− |
+ |
− |
− |
− |
− |
3.2. Optimization of the mobile phase composition
Several trials were performed to achieve complete separation among the four studied compounds. Trials started with ethanol: ethylacetate (8
:
2, 2
:
8, and 5
:
5, v/v) where the peaks of CIN, DMH, and BZP appeared with the solvent front in all these trials. After that, nonpolar solvents were tested in a trial to enhance the retention of these rapidly eluted compounds. Different ratios of hexane
:
ethanol, chloroform
:
ethanol, and methylene chloride
:
ethanol were checked and the best non polar solvent was hexane in the ratio of (7
:
3, v/v) where the retention of DMH, CIN and BZP was improved but unresolved peaks for DMH and CIN were observed, besides, DPP appeared near the baseline. On the other hand, lower volumes of hexane significantly affected the separation of other components. The effect of mobile phase pH on the separation efficacy was then tested by separate addition of different volumes of formic acid, ammonia solution (33%), triethylamine, orthophosphoric acid, and glacial acetic acid to the optimum developing system. It was noticed that the addition of 0.5 mL glacial acetic acid was sufficient to improve the separation between CIN and DMH. Additionally, different volumes of acetone were tested (0.3–0.8 mL) to improve the Rf value of DPP without affecting the separation among the four compounds. Finally, complete separation was achieved upon using a mobile phase mixture of hexane
:
ethanol
:
acetone
:
glacial acetic acid (7
:
3
:
0.7
:
0.5, by volume).
3.2.1. Selection of detection wavelength. To improve the sensitivity of the developed method, different scanning wavelengths were tried (215, 225, and 254 nm). The highest sensitivity with low noise for all the scanned components was observed on scanning at 225 nm as shown in Fig. 2.
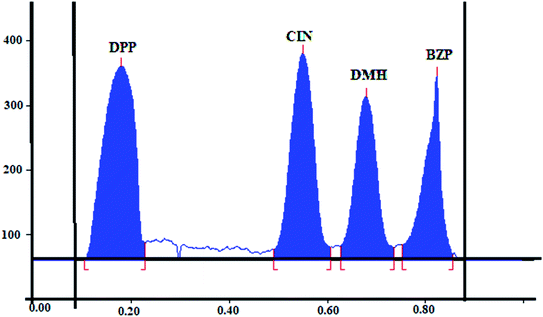 |
| Fig. 2 2D TLC chromatogram of a mixture of 1-diphenylmethyl piperazine, cinnarizine, dimenhydrinate and benzophenone using hexane : ethanol : acetone : glacial acetic acid (7 : 3 : 0.7 : 0.5, by volume) as developing system and scanned at 225 nm. | |
3.2.2. Slit dimensions of scanning light beam. Different slit dimensions were checked and 3 × 0.3 mm2 was proved to be the slit dimension that provided the highest sensitivity for all the studied drugs.Finally, suitable resolution and acceptable peaks were attained where the Rf values were 0.18, 0.55, 0.68, and 0.82 for DPP, CIN, DMH and BZP, respectively. Fig. 2.
3.3. Methods validation
Method validation was carried out according to guidelines for method validation from the International Conference on Harmonization (2005).48 It was performed to estimate selectivity, linearity, sensitivity, accuracy, and precision.
3.3.1. Linearity of calibration curves. Calibration curves were linear over the concentration ranges of 0.2–4, 0.5–5, 0.05–2.2 μg per band for DMH, CIN and DPP, respectively with correlation coefficients (r) of 0.9996, 0.9999, and 0.9998, respectively. While, a polynomial calibration curve of BZP was plotted over the range of 0.1–2.0 μg per band, with correlation coefficient of 0.9997. The calculated regression parameters including the slopes and intercepts are presented in Table 3.
Table 3 Regression and analytical parameters of the proposed TLC-densitometric method
Parameters |
DMH |
BZP |
CIN |
DPP |
Mean recoveries of six concentrations of pure CIN, DPP, DMH and BZ. The x2 coefficient (m) of the polynomial regression equation for BZP: A = mx2 + nx + k. Where A is the peak area of the analyte and k is the intercept. The x coefficient (n) of the polynomial regression equation for BZP: A = mx2 + nx + k. Where A is the peak area of the analyte and k is the intercept. The inter-day precision (n = 9), average SD of three different concentrations repeated three times within one. The inter-day precision (n = 9), average SD of three different concentrations repeated three times on three successive days. |
Calibration range (μg per band)a |
0.2–4.0 |
0.1–2.0 |
0.5–5.0 |
0.05–2.2 |
Slope |
51.1250 |
−1.9080b |
67.0600 |
19.9170 |
91.0881c |
Intercept |
20.0660 |
129.1200 |
8.9100 |
12.4050 |
Correlation coefficient |
0.9996 |
0.9997 |
0.9999 |
0.9998 |
Accuracy |
99.95 |
100.20 |
99.99 |
99.59 |
Repeatability (% RSD)d |
1.09 |
1.32 |
1.41 |
1.16 |
Intermediate precision (% RSD)e |
1.62 |
1.59 |
1.55 |
1.25 |
Limit of detection |
0.05 |
0.03 |
0.11 |
0.01 |
Limit of quantification |
0.15 |
0.09 |
0.33 |
0.03 |
3.3.2. Accuracy and precision. Accuracy of the developed method was evaluated by analyzing different concentrations of DMH, BZP, CIN, and DPP in their linearity ranges following the instructions of the method and calculated as percentage recovery. Good results were obtained and are given in Table 3. Additionally, the standard addition technique was applied to amocerebral® tablets to assess the accuracy of the proposed method, the good recoveries obtained suggested no interference from excipients (Table 4).
Table 4 Statistical analysis of proposed TLC-densitometric and the reported methods for the determination of dimenhydrinate and cinnarizine in its dosage form and results of standard addition technique
Parameter |
Proposed method |
Reported HPLC method41 |
DMH |
CIN |
DMH |
CIN |
The values between parentheses correspond to the theoretical values of t and F (p = 0.05). Average of three determinations. |
Mean |
99.84 |
99.98 |
100.21 |
100.26 |
SD |
1.69 |
0.77 |
2.29 |
0.88 |
t-Test (2.228)a |
0.32 |
0.59 |
— |
— |
F-Value (5.050)a |
1.83 |
1.30 |
— |
— |
Standard addition (mean ± SD)b |
98.87 ± 0.36 |
96.95 ± 1.99 |
|
|
The precision was assessed by measuring the intra and interday variations. Intraday variation (repeatability) was calculated by analyzing separately three concentrations of CIN and DMH (0.6, 2.6, and 3.4 μg per band), DPP (0.1, 1.3, and 2 μg per band) and BZP (0.20, 1, and 1.5 μg per band). Samples were analyzed three times on the same day following the instructions of the method and results were expressed as RSD (%). Interday variations (intermediate precision) were confirmed by analyzing the previous concentrations on three consecutive days. Acceptable results and low RSD were obtained (Table 3).
3.3.3. Limits of detection and quantitation. Sensitivity of the developed method was proved by calculation of the limits of detection (LOD) and quantitation (LOQ). They were calculated using the following equations: LOD = 3.3 × SD/slope and LOQ = 10 × SD/slope. The standard deviation (SD) was calculated from the lower part of the calibration curve, while the slope referred to the slope of the calibration curve for each analyte. The proposed method showed high sensitivity as demonstrated by LOD and LOQ values given in (Table 3)
3.3.4. Selectivity. Selectivity of the proposed method was proven by the complete separation between the four analytes shown in Fig. 2. Additionally, values of resolution and selectivity factors were within the acceptable limits ensuring complete separation among the four components (Table 5).
Table 5 Summary of parameters required for system suitability testing of the proposed TLC-densitometric method
Parameters |
DPP |
CIN |
DMH |
BZP |
Reference values46 |
Rf |
0.18 |
|
0.55 |
|
0.68 |
|
0.82 |
— |
Tailing factor |
0.88 |
|
1.00 |
|
1.08 |
|
0.85 |
<2 |
Resolution |
|
3.35 |
|
1.88 |
|
2.00 |
|
>1.5 |
Selectivity |
|
3.35 |
|
1.24 |
|
1.18 |
|
>1 |
K′ capacity |
0.82 |
|
0.45 |
|
0.32 |
|
018 |
|
3.3.5. Robustness. Robustness is characterized as the method's ability to tolerate minor changes intended in system parameters such as changing the percentage of acetic acid (±0.05 mL) and saturation time (±5 min). The effect of these changes on Rf value was calculated as the SD. It was found that changing in the studied parameters had no significant effect on the Rf values of the separated peaks (Table 6)
Table 6 Results of testing method robustness
Parameter |
Rf |
DPP |
CIN |
DMH |
BZP |
Saturation (min) time ± 5 min |
25.00 |
0.17 |
0.53 |
0.67 |
0.82 |
30.00 |
0.18 |
0.55 |
0.68 |
0.82 |
35.00 |
0.20 |
0.57 |
0.71 |
0.84 |
SD |
0.02 |
0.02 |
0.02 |
0.01 |
Volume of acetic acid ± 0.05 mL |
0.40 |
0.21 |
0.58 |
0.71 |
0.83 |
0.50 |
0.18 |
0.55 |
0.68 |
0.82 |
0.60 |
0.16 |
0.52 |
0.66 |
0.8 |
SD |
0.03 |
0.03 |
0.03 |
0.02 |
3.3.6. System suitability testing parameters. System suitability was evaluated by calculating the capacity factor (K′), tailing factor (T), selectivity (α) and resolution (Rs) factors. All measured parameters (Table 5) were within acceptable ranges,50 indicating the high selectivity of the method established and ensuring its validity.
3.3.7. Application of the method. The developed method was successfully applied to the available dosage form; amocerebral® tablet. The results presented in Table 4 revealed the suitability of the developed method for the simultaneous determination of DMH and CIN in their available dosage form without interfering from tablets excipients. Furthermore, the application of standard addition technique confirmed the accuracy of the method.
4. Greenness assessment
Green analytical chemistry is designed to eliminate or reduce the amount of toxic solvents produced and consumed daily worldwide. Eco-scale provides a semiquantitative tool for assessment of the greenness of an analytical procedure, which is preferable than other qualitative ones (NEMI, Efactor) considering the environmental consequences and the energy consumption during the life cycle of the analytical method.
Eco-scale was used to assess and easily compare the proposed and reported methods greenness, the calculated eco-scale scores of the developed method was >75 meaning excellent green analysis.51 (Table 7).
Table 7 The penalty points for determination of Analytical Eco-Scale score of the developed and reported methods
Parameters |
Developed TLC-densitometric method |
Penalty points (PP) |
Reported TLC-densitometric41 |
Penalty points (PP) |
Reported HPLC41 |
Penalty points (PP) |
Reagents (PP of solvent = subtotal PP × number of pictogram × signal word) |
Hexane |
8 |
Chloroform |
4 |
0.05 M KH2PO4 solution |
0 |
Consumed volume/sample = 1.94 mL |
Consumed volume/sample = 2.63 mL |
Consumed volume = 2.45 mL |
Subtotal PP = 1 [solvent <10 mL] |
Subtotal PP = 1 [solvent <10 mL] |
Subtotal PP = 1 [solvent <10 mL] |
Signal word = 2 danger |
Signal word = 2 danger |
Signal word = 0 none |
No. of pictogram = 4 |
No. of pictogram = 2 |
No. of pictogram = 0 |
Ethanol |
4 |
Methanol |
6 |
Methanol |
6 |
Consumed volume/sample = 0.83 mL |
Consumed volume/sample = 0.14 mL |
Consumed volume/sample = 4.55 mL |
Subtotal PP = 1 [solvent <10 mL] |
Subtotal PP = 1 [solvent <10 mL] |
Subtotal PP = 1 [solvent <10 mL] |
Signal word = 2 danger |
Signal word = 2 danger |
Signal word = 2 danger |
No. of pictogram = 2 |
No. of pictogram = 3 |
No. of pictogram = 3 |
Acetone |
4 |
Ammonia solution (33%) |
6 |
|
|
Consumed volume/sample = 0.19 mL |
Consumed volume/sample = 0.03 mL |
Subtotal PP = 1 [solvent <10 mL] |
Subtotal PP = 1 [solvent <10 mL] |
Signal word = 2 danger |
Signal word = 2 danger |
No. of pictogram = 2 |
No. of pictogram = 3 |
Glacial acetic acid |
4 |
Glacial acetic acid |
4 |
Consumed volume = 0.14 mL |
Consumed volume = 0.03 mL |
Subtotal PP = 1 [solvent <10 mL] |
Subtotal PP = 1 [solvent <10 mL] |
Signal word = 2 danger |
Signal word = 2 danger |
No. of pictogram = 2 |
No. of pictogram = 2 |
Instruments |
Energy |
≤1.5 kW h per sample |
1 |
≤1.5 kW h per sample |
1 |
≤1.5 kW h per sample |
1 |
Occupational hazard |
Analytical process hermetization |
0 |
Analytical process hermetization |
0 |
Analytical process hermetization |
0 |
Wastes |
1–10 mL |
3 |
1–10 mL |
3 |
1–10 mL |
3 |
Total penalty points |
24 |
24 |
10 |
Analytical eco-scale total score |
76 |
76 |
90 |
5. Statistical analysis
The results obtained by the proposed TLC-densitometric method for amocerebral® tablets were statistically compared to the reported method.45 Using Student's t-test and variance ratio F-test at a 95% confidence level and the values of the calculated t and F were less than the tabulated ones (Table 4), revealing no significant difference with respect to accuracy and precision between the proposed method and the reported one.
6. Conclusion
Benzophenone was reported as a carcinogenic and hepatotoxic impurity of DMH. On the other hand, the in silico toxicity profiling was studied for CIN impurities and results showed that DPP (impurity A of CIN) had the highest toxicity among all CIN impurities (A–E). Likewise, a novel, accurate and precise TLC-densitometric method has been developed for the simultaneous determination of DMH, CIN and their toxic impurities; BZP and DPP, respectively. The method was cost and time-effective as many samples can be performed simultaneously using small quantities of solvents. Additionally, the proposed method was validated according to ICH guidelines and all results were within acceptable limits. The validated method has been successfully applied for the estimation of the active drugs in their pure forms and in their commercial dosage form. Furthermore, analytical eco-scale was used for evaluation of the greenness of the method where the results proved that the method had a low negative environmental impact.
Funding
This research did not receive any specific grant from funding agencies in the public, commercial, or not-for-profit sectors.
Conflicts of interest
The authors declare that they have no known competing for financial interests or personal relationships that could have appeared to influence the work reported in this paper. The authors declare the following financial interests/personal relationships which may be considered as potential competing interests.
References
- S. Budavaried, The Merck Index: an encyclopedia of Chemicals, Drugs and Biological Merck & Co. Inc, Whitehouse station, NJ, USA, 2012 Search PubMed.
- X. Wen, F. Di Paola and N. Chopra, Psychotropics in your medicine cabinet: a case study of dimenhydrinate use, J. Addict. Med., 2019, 13, 412–414 CrossRef.
- A. G. Halpert, M. C. Olmstead and R. J. Beninger, Dimenhydrinate produces a conditioned place preference in rats, Pharmacol., Biochem. Behav., 2003, 75, 173–179 CrossRef CAS.
- A. W. Scholtz, et al., Efficacy and safety of a fixed combination of cinnarizine 20 mg and dimenhydrinate 40 mg vs betahistine dihydrochloride 16 mg in patients with peripheral vestibular vertigo: a prospective, multinational, multicenter, double-blind, randomized, non-inferior, Clin. Drug Invest., 2019, 39, 1045–1056 CrossRef CAS.
- British Pharmacopoeia, Her Majesty's, The Stationery Office, London, 2014 Search PubMed.
- M. C. Rhodes, et al., Carcinogenesis studies of benzophenone in rats and mice, Food Chem. Toxicol., 2007, 45, 843–851 CrossRef CAS.
- G. A. Burdock, D. H. Pence and R. A. Ford, Safety evaluation of benzophenone, Food Chem. Toxicol., 1991, 29, 741–750 CAS.
- Y. Nakagawa, T. Suzuki and S. Tayama, Metabolism and toxicity of benzophenone in isolated rat hepatocytes and estrogenic activity of its metabolites in MCF-7 cells, Toxicology, 2000, 156, 27–36 CrossRef CAS.
- https://www.fishersci.com/shop/products/benzophenone-crystalline-flakes-certified-fisher-chemical/B270500#?keyword=Benzophenones, 28/4/2020 21:34.
- C. S. M. Liew, X. Li, H. Zhang and H. K. Lee, A fully automated analytical platform integrating water sampling-miniscale-liquid-liquid extraction-full evaporation dynamic headspace concentration-gas chromatography-mass spectrometry for the analysis of ultraviolet filters, Anal. Chim. Acta, 2018, 1006, 33–41 CrossRef CAS.
- H. K. Jeon, Comparative toxicity related to metabolisms of benzophenone-type UV filters, potentially harmful to the environment and humans, Mol. Cell. Toxicol., 2017, 13, 337–343 CrossRef CAS.
- T. B. Adams, et al., The FEMA GRAS assessment of aromatic substituted secondary alcohols, ketones, and related esters used as flavor ingredients, Food Chem. Toxicol., 2007, 45, 171–201 CrossRef CAS.
- Y. Nakagawa and K. Tayama, Estrogenic potency of benzophenone and its metabolites in juvenile female rats, Arch. Toxicol., 2001, 75, 74–79 CrossRef CAS.
- J. E. F. Reynolds, Martindale, The Complete Drug Reference, The Pharmaceutical Press, London, 32nd edn, 1999, p. 406 Search PubMed.
- C. R. Gordon, et al., The effects of dimenhydrinate, cinnarizine and transdermal scopolamine on performance, J. Psychopharmacol., 2001, 15, 167–172 CrossRef CAS.
- M. V. Kirtane, A. Bhandari, P. Narang and R. Santani, Cinnarizine: a contemporary review, Indian J Otolaryngol Head Neck Surg., 2019, 71, 1060–1068 CrossRef.
- B. A. Olsen, B. C. Castle and D. P. Myers, Advances in HPLC technology for the determination of drug impurities, TrAC, Trends Anal. Chem., 2006, 25, 796–805 CrossRef CAS.
- A. B. Raies and V. B. Bajic, In silico toxicology: computational methods for the prediction of chemical toxicity, Wiley Interdiscip. Rev.: Comput. Mol. Sci., 2016, 6, 147–172 CAS.
- K. Van Aken, L. Strekowski and L. Patiny, EcoScale, a semi-quantitative tool to select an organic preparation based on economical and ecological parameters, Beilstein J. Org. Chem., 2006, 2, 3 Search PubMed.
- A. Gałuszka, Z. M. Migaszewski, P. Konieczka and J. Namieśnik, Analytical Eco-Scale for assessing the greenness of analytical procedures, TrAC, Trends Anal. Chem., 2012, 37, 61–72 CrossRef.
- European Pharmacopoeia, 6.0, European Directorate for the Quality of Medicines, 2014 Search PubMed.
- The United States Pharmacopoeia, 32th Revision, NF 27, The United States Pharmacopoeial Convention Inc., Rockville, MD, 2013 Search PubMed.
- A. Kar and G. I. Aniuha, Spectrophotometric determination of dimenhydrinate with Reinecke salt, J. Pharm. Sci., 1981, 70, 690 CrossRef CAS.
- J. Machado Freitas, et al., Development of a simple and fast electrochemical method for screening and stoichiometric determination of dimenhydrinate, Electroanalysis, 2014, 26, 1905–1911 CrossRef.
- R. M. Shubietah, A. Z. A. Zuhri and A. G. Fogg, Adsorptive stripping voltammetric determination of dimenhydrinate at a hanging mercury drop electrode, Microchim. Acta, 1999, 130, 165–171 CrossRef CAS.
- U. Döge and K. Eger, A simple HPLC-UV method for the determination of dimenhydrinate and related substances - Identification of an unknown impurity, Pharmazie, 2007, 62, 174–178 Search PubMed.
- B. V. Girish, S. Praveen and N. Kathyayini, Analytical Quality By Design For Development Of A Sensitive, Rapid And Stability Indicating Method For Estimation Of Impurities Of Dimenhydrinate In Its Pharmaceutical Formulation, Res. J. Pharm., Biol. Chem. Sci., 2016, 7, 1309–1322 CAS.
- T. S. Belal, K. M. Abdel-Hay and C. R. Clark, Selective determination of dimenhydrinate in presence of six of its related substances and potential impurities using a direct GC/MS method, J. Adv. Res., 2016, 7, 53–58 CrossRef CAS.
- H. Abdine, F. Belal and N. Zoman, Simple spectrophotometric determination of cinnarizine in its dosage forms, Farmaco, 2002, 57, 267–271 CrossRef CAS.
- S. M. Tawakkol, M. B. El-Zeiny and A. Hemdan, Full spectrum and selected spectrum based chemometric methods for the simultaneous determination of cinnarizine and dimenhydrinate in laboratory prepared mixtures and pharmaceutical dosage form, Spectrochim. Acta, Part A, 2017, 173, 892–896 CrossRef CAS.
- S. S. M. Hassan, R. M. Abdel-Aziz and A. B. Abbas, Potentiometric membrane sensors for the selective determination of cinnarizine in pharmaceutical preparations, Anal. Chim. Acta, 1996, 321, 47–52 CrossRef CAS.
- G. O. El-Sayed, S. A. Yasin and A. A. El Badawy, Voltammetric behavior and determination of cinnarizine in pharmaceutical formulations and serum, Anal. Lett., 2008, 41, 3021–3033 CrossRef CAS.
- S. S. M. Hassan, M. A. F. Elmosallamy and A. B. Abbas, LC and TLC determination of cinnarizine in pharmaceutical preparations and serum, J. Pharm. Biomed. Anal., 2002, 28, 711–719 CrossRef CAS.
- A. A. Heda, A. R. Sonawane, G. H. Naranje and P. K. Puranik, A rapid determination of cinnarizine in bulk and pharmaceutical dosage form by LC, E-J. Chem., 2010, 7, 1080–1084 CrossRef CAS.
- M. T. Rosseel and R. A. Lefebvre, Sensitive determination of cinnarizine in human plasma by high performance liquid chromatography and fluorescence detection, Chromatographia, 1993, 36, 356–358 CrossRef CAS.
- A. P. Argekar and S. J. Shah, Simultaneous determination of cinnarizine and domepiridone maleate from tablet dosage form by reverse phase ion pair high performance liquid chromatography, J. Pharm. Biomed. Anal., 1999, 19, 813–817 CrossRef CAS.
- A. El-Gindy, S. Emara and A. Mostafa, Spectrophotometric and LC determination of two binary mixtures containing antihistamins, Farmaco, 2004, 59, 713–722 CrossRef CAS.
- A. L. Rao, T. Prasanthi, C. Meenakshi, J. Banu, J. Mrunalini, M. C. S. Teja and V. Abhishek, Analytical Method Development and Validation for the Estimation of Cinnarizine by RP-HPLC in Bulk and Pharmaceutical Dosage Forms, Asian J. Pharm. Health Sci., 2019, 9, 2053–2058 CAS.
- C.-H. Zhang, W. Jin, S.-M. Zhang and Y.-J. Yang, Determination of related substances in cinnarizine by supercritical fluid chromatography, Chin. Pharm. J., 2018, 53, 1603–1607 Search PubMed.
- N. T. Lamie and A. M. Yehia, Development of normalized spectra manipulating spectrophotometric methods for simultaneous determination of dimenhydrinate and cinnarizine binary mixture, Spectrochim. Acta, Part A, 2015, 150, 142–150 CrossRef CAS.
- N. S. Abdelwahab, M. M. Abdelrahman, F. M. Salama and A. B. Ahmed, Determination of dimenhydrinate and cinnarizine in combined dosage form in presence of cinnarizine impurity, Eur. J. Chem., 2015, 6, 475–481 CrossRef CAS.
- D. S. El-Kafrawy and T. S. Belal, Validated HPTLC method for the simultaneous determination of cinnarizine and dimenhydrinate in their combined dosage form, J. Assoc. Arab Univ. Basic Appl. Sci., 2016, 19, 15–22 Search PubMed.
- N. T. Lamie and H. H. Monir, Simultaneous determination of cinnarizine and dimenhydrinate in binary mixture using chromatographic methods, J. Chromatogr. Sci., 2016, 54, 36–42 CAS.
- A. J. Nathan and A. Scobell, Development and validation of analytical method for simultaneous estimation of cinnarizine and dimenhydrinate in tablet dosage form, Int. J. Pharma Sci. Res., 2014, 5, 4815–4819 Search PubMed.
- A. B. Ahmed, N. S. Abdelwahab, M. M. Abdelrahman and F. M. Salama, Simultaneous determination of Dimenhydrinate, Cinnarizine and Cinnarizine impurity by TLC and HPLC chromatographic methods, Bull. Fac. Pharm., 2017, 55, 163–169 Search PubMed.
- M. Srivastava, High-performance thin-layer chromatography (HPTLC), Springer Science & Business Media, 2011, DOI:10.1007/978-3-642-14025-9.
- N. S. Abdelwahab, F. H. Edrees, N. H. Amin and A. S. Saad, Therapeutic drug monitoring of two co-administered drugs through development of two ecological chromatographic methods: in vivo application, Microchem. J., 2020, 104935 CrossRef CAS.
- ICH, Q2 (R1), Validation of Analytical Procedures: Text and Methodology, ICH Harmonized Tripartite Guideline, 2005 Search PubMed.
- Bioinformatics and Molecular Design Research Center, Seul, South Corea, PreADMET program, 2004, available from: URL: http://preadmet.bmdrc.org Search PubMed.
- B. Fried and B. Sherma Thin-Layer Chromatography, revised and expanded, CRC Press, 1999 Search PubMed.
- A. Gałuszka, Z. M. Migaszewski, P. Konieczka and J. Namieśnik, Analytical Eco-Scale for assessing the greenness of analytical procedures, TrAC, Trends Anal. Chem., 2012, 37, 61–72 CrossRef.
|
This journal is © The Royal Society of Chemistry 2020 |
Click here to see how this site uses Cookies. View our privacy policy here.