DOI:
10.1039/D0RA06254E
(Paper)
RSC Adv., 2020,
10, 32833-32842
Quality characteristics of infusion and health consequences: a comparative study between orthodox and CTC green teas†
Received
18th July 2020
, Accepted 13th August 2020
First published on 3rd September 2020
Abstract
The present study compares the effects of two green tea processing techniques, viz. orthodox and CTC (curl, tear and crush) on the quality parameters and sensory profiles under the geographical and climatic conditions of Assam, India. The results showed that CTC green tea infusions had 13.3, 7.5, 7.1, 9.8, 5.4, 17.3, 17.1 and 18.6% more total polyphenol, total catechin, (−)-epigallocatechin-3-gallate (EGCG), (−)-epicatechin-3-gallate (ECG), (−)-epigallocatechin (EGC), (−)-epicatechin (EC), water extract and theanine level, respectively than the infusions prepared from orthodox green tea. The sensory evaluation preferred the orthodox over CTC processing mode. Risk assessment with daily consumption of five cups (10 g) of green tea reveals that the EGCG level is free from the risk of hepatotoxicity and caffeine will not inflict any health hazard.
1. Introduction
The potential health beneficial activities have made green tea widely popular. The popularity and demand for green tea in India are increasing at a high rate after Asian countries like China and Japan. Food and nutritional sciences have given in-depth attention to a better understanding of its chemical constituents and their benefits.1 Many researchers have demonstrated the beneficial effects of green tea consumption on human health over many diseases. Epidemiological study reveals direct relationship of green tea consumption with enhanced human leukocyte activity,2 reduced risk of Parkinson's disease3 and Alzheimer's disease.4 The most dominant green tea catechin (−)-epigallocatechin-3-gallate has been demonstrated effective against viral infection,5 various types of cancers in a number of in vitro studies.6,7 Clinical studies on green tea demonstrate its effectiveness in cognitive dysfunction,8 modulation of brain activity.9 These medicinal effects can be attributed to its flavanol (also called catechin) contents which are the major group of polyphenolic compounds present in tea. The principal flavanols present in green tea are (−)-epicatechin (EC), (−)-epigallocatechin (EGC), (−)-epicatechin-3-gallate (ECG) and (−)-epigallocatechin-3-gallate (EGCG).10 The polyphenolic compounds account for 24–36% of total biochemical contents in tea.10 Furthermore, green tea is an unfermented tea and its infusion has higher content of polyphenols than other types of fermented teas such as black and oolong tea.11 Fermented teas are reasonably expected to have a similar level of polyphenols as that of unfermented teas. However, the complex and polymeric nature of most of the polyphenols, formed during the fermentation stage, has prevented chemical characterization.12 The taste of green tea infusions primarily comprises astringency, bitterness and umami.13
Several researchers reported green tea processing with some modifications in the conventional orthodox processing method, and their effect on biochemical constituents.10,14,15 In orthodox processing of green tea, first deactivation of endogenous enzymes, mainly polyphenol oxidase and peroxidase is carried out. This deactivation of fresh leaf enzymes is the key step in green tea processing which prevents the oxidation of catechins. This could be achieved either by steaming or pan firing of green leaves.16 However, these enzyme deactivation processes drastically affect the quality of green tea, particularly the aroma and physical appearance. Typically, in the steaming process, freshly plucked leaves are subjected to hot steam of water at around 102 °C for a certain amount of time (1–2 min).15 On the other hand, in the pan firing process, freshly plucked leaves undergo withering for 2–3 h, where endogenous enzymes affect the chemical composition of the leaves,15,16 followed by pan-fired at 220 °C for 5 minutes.14–16 The steamed or pan-fired leaves are then rolled for 30–60 minutes for rupture of the leaf tissues as well as to have the desired shape and finally dried at 90–110 °C for 5–15 minutes.
CTC is widely used in black tea manufacturing process. The process involves five different steps consisting of withering, rolling, CTC, fermentation and drying. In the CTC step, the rolled leaves are subjected to crushing, tearing and curling in a CTC machine. The advantages of CTC black tea manufacturing are lower fermentation time and higher extraction efficiency. Very limited literatures are available where CTC step has been introduced for green tea processing. Barbora and Saha introduced CTC after the initial step of steaming or pan firing for green tea processing.17 The authors reported more cuppage (also known as number of cups) and shorter processing period for CTC green tea over the orthodox. However, no biochemical data was reported by the authors. Kilel et al. also introduced the CTC step in green tea processed from purple colored tea clones of Kenya.18 To the best of our knowledge, report on the comparative study of orthodox and CTC green tea processing is very limited in terms of biochemical constituents and their subsequent transfer into the infusion. Moreover, this aspect in the scenario of Assam's geographical and climatic condition has never been addressed. Therefore, the present investigation was aimed to determine the change in biochemical content on introducing CTC during green tea manufacturing over conventional orthodox processing and transfer rate of bioactive compounds from tea to its infusion. Organoleptic evaluation (also known as sensory evaluation) of both types of tea and the risk assessment of the daily intake level of caffeine and EGCG were also carried out.
2. Materials and methods
2.1. Chemicals
Gallic acid monohydrate (≥98.0%), caffeine (anhydrous, 99%), (−)-epigallocatechin-3-gallate (≥95%), (−)-epicatechin-3-gallate (≥95%, HPLC), (−)-epigallocatechin (≥95%, HPLC), (−)-epicatechin (≥90%, HPLC), (+)-catechin (≥95%, HPLC) and L-theanine (≥98%, HPLC) were procured from Sigma Aldrich, India. Folin–Ciocalteu reagent (LR) was purchased from Himedia (HiMedia Laboratories, India). Acetic acid (HPLC Grade), acetonitrile (HPLC Grade), sodium carbonate and all other chemicals were obtained from Merck KGaA, Darmstadt, Germany.
2.2. Instrumentation
A Dionex, Ultimate 3000 UPLC system fitted with Luna 5 μ phenylhexyl phenomenax column (4.5 mm × 250 mm) maintained at 25 ± 0.5 °C and UV-vis detector was used for determination of catechin, caffeine and theanine. The detector wavelength was set at 278 nm for catechin and caffeine quantification. For theanine, it was set at 210 nm. For UV-vis spectroscopy, Varian, Cary 50 Conc was used. Millipore Milli-Q Synthesis water purification system from Merck, Germany was used for Milli-Q water in all experiments. A fluid bed drier (FBD) from Teacraft, United Kingdom was used for enzyme deactivation and drying of green tea. A CTC roller with 10 TPI (teeth per inch) was used for CTC green tea processing. A Thermo Scientific, Orion Star A214 ion selective electrode (ISE) meter was used for quantification of fluoride and chloride. For flame photometry, a Systronics India Ltd flame photometer (Model 128) was used.
2.3. Plucking of tea leaves
The tender two leaves and the terminal bud from cultivars TV1, 9, 18, 20, 22, 23 and 25, Happy Valley 39, RR17/144, Ging186 and 482/12 grown under the identical environmental condition and soil nutrient management were hand-plucked from experimental Tea Estate (T.E.) of Tocklai Tea Research Institute (TTRI), Assam, India which lies between 26°43′14′′N and 94°11′54′′E and located at an elevation of 96.5 meters above mean sea level with an average precipitation of 2036 mm. Sample from each cultivar consisted of three biological replicates and green tea processing was carried out for each replicate sample.
2.4. Green tea processing
The fresh samples were subjected to hot air blow in the FBD set at 100 ± 5 °C for six minutes for enzyme deactivation. The deactivated samples were allowed to cool immediately with the exposure of cold air followed by hand-rolling for 30 minutes. The mass samples were divided into two parts. One part of the rolled samples was subjected to 3 cut CTC and then dried in the FBD at 90 ± 2 °C for twenty minutes to have the CTC green tea. The other part of the rolled leaves was dried under identical conditions to have the orthodox green tea.
2.5. Sensory evaluation of tea infusion
Green tea infusions were assessed by a tasting panel consisting of nine members aged between 23 and 55 years. The panellists were trained to quantify and evaluate the intensity of bitterness, sweetness, astringency and umami taste using deionised water solution of caffeine (1 mM), sucrose (50 mM), EGCG (0.8 mM), and sodium glutamate (3 mM), respectively for standardisation of judgments.19 A 10-point scale was used for scoring, where 8–10 indicates ‘extremely strong’, 6–8 indicates ‘strong’, 4–6 indicates ‘neutral’, 2–4 indicates ‘weak’ and 0–2 indicates ‘extremely weak’.11 Tea infusions were prepared by brewing 2 g green tea in 150 mL boiled water for 3 minutes. The tea was served in white porcelain bowls at room temperature. For the tasting, 5 mL infusion was held for 20 s in the oral cavity by the panellists and scores for different tastes were drawn. For preventing previous taste interference the panellists cleansed their palates with skimmed milk and flushed oral cavity thrice with water before tasting the next infusion.19 Three replications were performed for each evaluation on three different days with sample orders being randomised in each day.
Determination protocol of catechin, caffeine, theanine, water extract as well as calibration standard are available in ESI.† Besides these, transfer rate of bioactive compounds and determination of sodium, potassium, fluoride and chloride in tea infusion are also available in ESI.†
2.6. Risk assessment
Estimated daily intake (EDI) of caffeine and catechins on consuming five cups (10 g) of green tea in milligram per kilogram body weight (mg kg−1 BW) per day was calculated by the following formula |
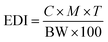 | (1) |
where C is the mean concentration of the biochemical compound of interest in green tea (mg g−1); M is the weight of consumed green tea per day (10 g); T is the transfer rate in percentage and BW is the average body weight for men (67.4 kg) and women (64.9 kg).20
2.7 Statistical analysis
Analysis of variance (ANOVA) was performed by using SPSS software version 17.00 (SPSS Inc., Chicago, IL). Tukey's multiple comparison test was used to get the differences between means and the differences were considered significant at p ≤ 0.05 and p ≤ 0.01. For each sample, all data were reported as the mean ± standard error (SE) with three independent replications. Pearson correlations were drawn using SPSS software version 17.00 (SPSS Inc., Chicago, IL) among the different taste attributes and contributing biochemical contents to study the association among them.
3. Results and discussion
3.1. Total polyphenol
Total polyphenol (TP) refers to flavanols (catechins), catechin gallates, flavonoid glycosides and phenolic acids. The polyphenols impart astringency taste to green tea infusion. The TP contents in CTC and orthodox green teas, in infusions, and their extent of transfer into infusions are presented in Fig. 1(a). TP content varied from 199.7 ± 3.4 mg g−1 in RR17/144 to 258.2 ± 1.4 mg g−1 in Ging186. The highest TP level in Ging186 observed significant difference (p ≤ 0.05) from that in TV9, 20, 23, 25 and RR17/144. Available literature reported TP level in green tea in the range from 208 to 259 mg g−1,18 182.6 to 296.4 mg g−1
21 determined using Folin–Ciocalteu reagent. Wang and Ruan reported TP level in Longjing green tea in the range from 211.2 to 281.2 mg g−1 using a FeSO4–NaKC4H4O6 dyeing solution and phosphate buffer.22 These observations are in agreement with the current findings. However, Kilel et al. reported that two traditionally used clones, Yabukita and Hanlu for green tea manufacturing in Japan and China, had lower levels of TP at 172 mg g−1 and 197 mg g−1, respectively.18 The CTC step significantly influenced the transfer rate of TP to infusion in all the cultivars. As the introduction of CTC in green tea processing resulted in increased surface area of the leaves that is exposed to the solvent, it facilitated the transfer of bioactive compounds into infusions.23 An enhanced average transfer rate of 13.3% was observed in CTC green tea. The TP level in CTC green tea infusion (CGTI) was in the range between 81.8 ± 2.1 and 120.7 ± 1.4 mg g−1 (equivalent to 1151–1652 mg L−1 GAE), whereas in orthodox green tea infusion (OGTI) it was between 53.6 ± 1.9 and 92.4 ± 1.3 mg g−1 (equivalent to 783–1265 mg L−1 GAE). The highest and lowest TP level was in TV1 and TV9, respectively, for both processing modes. These results support the findings of Liu et al. (500–1700 mg L−1 GAE, Folin–Ciocalteu reagent method) where the effect of particle size and brewing condition on TP level in green tea infusions were demonstrated.23 Farakte et al. reported a variation of rate constants from 0.257 to 0.685 min−1 while preparing tea infusion with a varying particle size of tea.24 Smaller particle size had higher extraction leading to faster infusion. During the CTC step, massive rupture of cell membranes exposes the biochemical contents. The biochemical components at the exposed surface can easily get dissolved in water. Furthermore, the transfer of compounds from tea to its infusion depends on its concentration in leaf and solubility.25 The dissolution of TP in water observes different phases of rapid and slow rate. The extraction of TP increases with time. The varying dissolution phases may be attributed to different solubility of the polyphenolic substances present in tea leaves.25 The current results also support the TP levels in green tea infusions (880–2560 mg L−1 GAE, Folin–Ciocalteu reagent method) reported by Komes et al.26
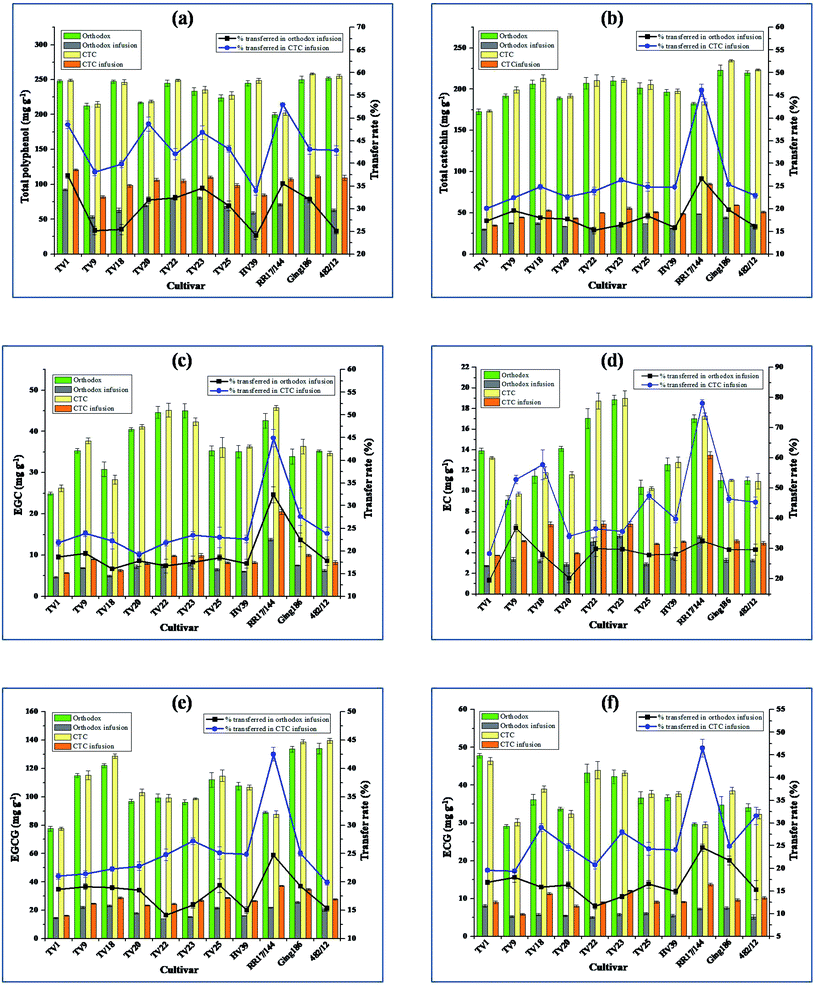 |
| Fig. 1 Average content (mg g−1) of total polyphenol (a), total catechin (b), EGC (c), EC (d), EGCG (e), and ECG (f) in CTC and orthodox green tea, in infusion, and their per cent transfer into infusions (error bars specify the standard error). | |
3.2. Total catechin
As evident all green tea samples proved to be a rich source of phenolic compounds. The total catechin (TC) contents in CTC and orthodox green teas, in infusions, and along with their transfer rate into infusion are presented in Fig. 1(b). The highest TC content of green teas was detected in Ging186 (234.5 ± 1.2 mg g−1) which is significantly different (p ≤ 0.05) from all others except 482/12 and the lowest TC was detected in TV1 (172.9 ± 3.2 mg g−1). Unlike our findings, Kilel et al. reported TC content in green tea processed from purple leaf colored cultivars of Kenya in the range between 52 and 120 mg g−1 using HPLC.18 They also demonstrated two standard green tea clones, Yabukita and Hanlu with TC contents of 94.1 and 123.0 mg g−1, respectively. The catechin (flavanol) content in fresh tea leaves is affected by sunlight intensity, temperature, rainfall, harvesting season, and other environmental factors. Therefore, the geographical origin of the growing region of tea cultivar plays a critical role in its biochemical contents which is reflected in the quality of processed tea.27,28 With increasing sunshine hour biosynthesis of flavanol increases to protect the plant from UV rays' damage.29 Moreover, the genetic diversity of tea plant is another important factor which affects its biochemical contents. However, the environment of the growing region is predominant in controlling the biochemical composition over that of genetic characteristics of the cultivar.29
The CTC step significantly influenced the transfer rate of TC into infusion in all the cultivars. The average transfer rates of TC into infusion were found to be 25.8% and 18.3% for CTC and orthodox green tea, respectively. TC content in CGTIs ranged from 34.8 ± 0.8 mg g−1 in TV1 to 84.9 ± 1.1 mg g−1 in RR17/144 (equivalent to 463.9–1133.1 mg L−1), whereas in OGTIs it was from 29.9 ± 0.9 mg g−1 in TV1 to 48.6 ± 0.3 mg g−1 in RR17/144 (equivalent to 399.1–647.6 mg L−1). The present results of TC content are comparable with other documented results such as 350.45–1073.15 mg L−1;26 398–1127 mg L−1;30 571–1416 mg L−1
31 determined using HPLC. From infusion kinetics study of CTC tea with varying particle size, Farakte et al. demonstrated that infusion prepared with tea (particle size 0.36 mm) observed 90% extraction in 3 min indicating its very fast extraction rate whereas tea with particle sizes 0.63, 0.83 and 1.17 mm had around 80% extraction in 3, 4 and 5 min, respectively.24 The diffusion of biochemical compounds from the complex tea matrix affects the infusion rate. This diffusion process becomes easier with reduced size of tea granules as the diffusion path gets shortened.
3.3. Non gallated catechins
Non gallated catechins comprise of (−)-epigallocatechin (EGC) and (−)-epicatechin (EC). The EGC and EC contents in green tea, in infusion, and its extent of transfer into infusion are presented in Fig. 1(c) and (d), respectively. Non-gallated catechins impart less astringency as compared to its gallated counterpart. EGC contributes a slight sweet after taste, even at a concentration level as low as 0.1% (w/v).18 It was found that RR17/144 (45.7 ± 0.6 mg g−1) and TV22 (45.2 ± 1.7 mg g−1) had the highest content of non-gallated catechin EGC, while TV1 (24.9 ± 0.4 mg g−1) had the lowest. Green tea processed from cultivar TV23 had the highest EC content with 19.0 ± 0.7 mg g−1 and TV9 had the lowest with 9.1 ± 0.4 mg g−1. In line with our results, Kilel et al. reported EGC and EC levels of green tea processed from Kenyan tea cultivars in the range between 4.0 and 61.2 mg g−1; and between 2.0 and 18.0 mg g−1, respectively.18 Dai et al. found EGC and EC level in Huangshan Maofeng green tea much lower than the current study at 11.32 and 8.68 mg g−1, respectively using HPLC.21 CGTIs had higher EGC levels in the range between 5.7 ± 0.1 and 20.5 ± 0.6 mg g−1 (equivalent to 76.1–273.3 mg L−1) than OGTIs in the range between 4.7 ± 0.2 and 13.7 ± 0.3 mg g−1 (equivalent to 62.0–183.2 mg L−1). EC levels in CGTIs varied from 3.7 ± 0.1 to 13.5 ± 0.4 mg g−1 (equivalent to 49.9–179.6 mg L−1) whereas in OGTIs it was from 2.7 ± 0.1 to 5.6 ± 0.2 mg g−1 (equivalent to 36.3–74.8 mg L−1). These results are consistent with previously published EGC and EC levels in tea infusion in the range from 69.71 to 279.87 mg L−1 and 48.80 to 169.16 mg L−1, respectively.26 The data reported here also supports the earlier documented EGC (34.9–291 mg L−1) and EC (19.3–154 mg L−1) levels in green tea infusions using HPLC.23
The processing mode had a significant influence on the transfer rate of non-gallated catechins into the infusion. The higher extraction level of non gallated catechins in CGTIs is probably due to reduced pore diffusion path of the tea matrix in CTC green tea. Green tea processed from cultivar RR17/144 had the highest EGC transfer rate with 44.9 ± 1.9 and 32.4 ± 1.8% for CTC and orthodox mode, respectively. The highest EC transfer rate (78.1 ± 1.3%) was observed for CTC green tea processed from RR17/144.
3.4. Gallated catechins
Gallated catechins consist of (−)-epigallocatechin-3-gallate (EGCG) and (−)-epicatechin-3-gallate (ECG). In addition to astringency, ECG can contribute to a bitter after taste. The levels of EGCG and ECG in green tea, in infusion, and their transfer rate into infusion are presented in Fig. 1(e) and (f), respectively. EGCG level in green teas varied from 77.5 ± 1.8 mg g−1 in TV1 to 139.5 ± 1.8 mg g−1 in cultivar 482/12. Green tea processed from TV1 had the highest level of ECG with 47.8 ± 0.6 mg g−1. The EGCG and ECG levels from the present study are at variance with some available literature such as 19–62 mg g−1 (EGCG) and 10–30 mg g−1 (ECG);18 70.76 mg g−1 (EGCG) and 27.08 mg g−1 (ECG),21 where the levels are much lower. The effect of processing method had a strong influence in content of these catechins in infusions. The cultivar RR17/144 had the highest water extraction efficiency of gallated catechins for both modes of processing. EGCG level in CGTIs it was in the range between 16.3 ± 0.4 and 37.3 ± 0.3 mg g−1(equivalent to 217.1–497.2 mg L−1), while in OGTIs it was between 14.0 ± 0.2 and 25.8 ± 0.7 mg g−1 (equivalent to 186.9–343.5 mg L−1). ECG levels were in the range between 5.9 ± 0.2 and 13.7 ± 0.4 mg g−1 (equivalent to 78.0–182.9 mg L−1); and between 5.0 ± 0.2 and 8.1 ± 0.3 mg g−1 (equivalent to 66.9–107.7 mg L−1) in CGTIs and OGTIs, respectively. The present results are in conformity with literature available EGCG and ECG levels in green tea infusion in the range between 94.54 and 357.07 mg L−1; and between below detectable limit (BDL) and 310.80 mg L−1, respectively.26 Liu et al. reported a much wider range of EGCG (201–1289 mg L−1) and ECG (48.5–435 mg L−1) in Xihu Longjing green tea infusions in different brewing conditions and with different particle sizes.23 The highest EGCG transfer rate was observed in RR17/144 with 42.5 ± 1.2% and 24.7 ± 0.3% for CTC and orthodox green tea, respectively, while for ECG it was 46.5 ± 2.0% and 24.6 ± 0.8% for respective modes of processing in the same cultivar. The gallated catechins contributed 60–78% to total catechin in green tea infusions. This observation is in agreement with Xu et al. who reported 75.17% contribution of gallated catechin towards total catechin concentration.31 A thorough disruption of cell membranes in the CTC process efficiently exposes the catechins.32 The higher surface area for smaller tea particles enhances the infusion rate owing to dissolution of compounds on surface.
3.5. Caffeine
Green tea has been a rich source of caffeine.26 Caffeine level in tea leaf decreases with increasing maturity.18 The caffeine content in CTC and orthodox green teas, in infusions, and its extent of transfer into infusions are presented in Fig. 2(a). The caffeine content in green teas was in the range between 30.0 ± 0.1 mg g−1 in Ging186 and 55.0 ± 0.6 mg g−1 in TV9. Caffeine content in TV9 green tea was significantly different (p ≤ 0.05) from all others except TV18. The caffeine level in the teas being reported here is higher than some earlier literature cited levels determined using HPLC.18,21,22,33 Dai et al. found caffeine content in Huangshan Maofeng, a famous Chinese green tea, at 32.88 mg g−1.21 The caffeine level in Ziyang green tea, a selenium enriched Chinese green tea, was demonstrated in the range between 26.71 mg g−1 and 38.06 mg g−1.33 In Kenya, green teas processed from purple color cultivars had caffeine level in the range between 11.6 and 22.0 mg g−1.18 The authors also reported that Chinese variety Hanlu origin green tea had 24.2 mg g−1 caffeine. Longjing green tea has caffeine content in the range from 26 mg g−1 to 38.50 mg g−1.22 The caffeine levels in CGTIs and OGTIs did not represent any significant difference. The level of caffeine in CGTIs was between 12.8 ± 0.5 and 24.5 ± 1.1 mg g−1 (equivalent to 170.1–326.5 mg L−1) whereas in OGTIs it was between 11.0 ± 0.2 and 21.1 ± 0.9 mg g−1 (equivalent to 146.4–281.6 mg L−1). The caffeine contents in infusions are strongly supported by earlier reports such as 99.21–300.0 mg L−1;26 141–338 mg L−1;30 139–823 mg L−1)
23 determined using HPLC.
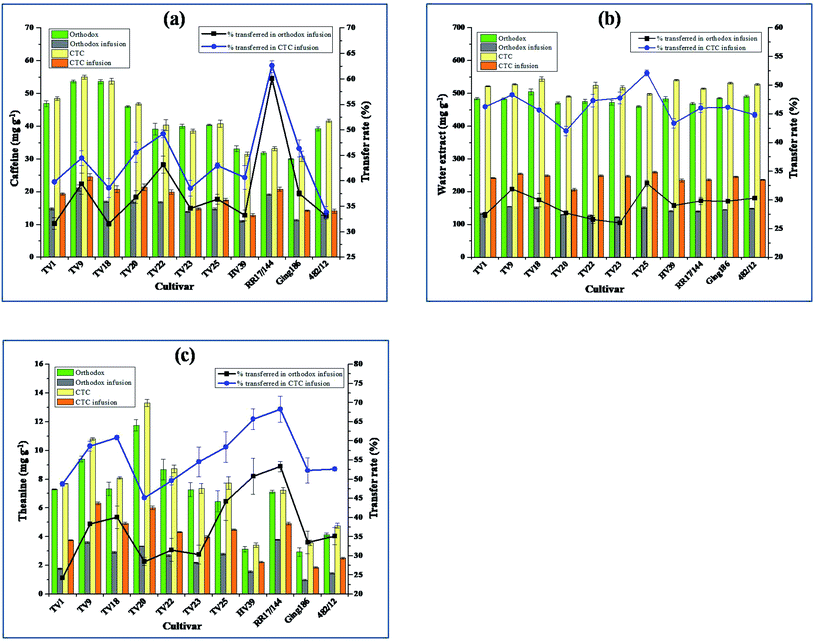 |
| Fig. 2 Average content (mg g−1) of caffeine (a), water extract (b), and theanine (c) in CTC and orthodox green tea, in infusion, and their per cent transfer into infusions (error bars specify the standard error). | |
3.6. Water extract
Water extract (WE) level was significantly influenced by the processing methods. The CTC green teas contain 17.12% (on average) more WE than their orthodox counterparts. WE levels in CTC and orthodox green teas, in infusions, and their transfer rate into infusion are summarized in Fig. 2(b). WE in CTC green teas varied from 490.7 ± 2.5 mg g−1 in TV20 to 544.0 ± 6.8 mg g−1 in TV18. WE content in TV18 CTC green tea was significantly different (p ≤ 0.05) from TV1, 20, 23, 25 and RR17/144. In orthodox green teas, WE was highest for TV18 (504.5 ± 9.3 mg g−1) and lowest for TV25 (459.5 ± 2.6 mg g−1). The highest content was significantly different (p ≤ 0.05) from TV20, 22, 23, 25 and RR17/144. WE levels in the present study are much higher than the minimum limit of 320 mg g−1 as defined by Food Safety and Standard Authority of India.34 The WE levels in the present study are higher than that reported in Longjing green tea (372.10–446.70 mg g−1) determined using ISO 9768:1994 method.22 The infusions from both types of teas were also estimated for the WE contents. The level of WE in CGTIs were in the range between 206.2 ± 4.5 and 259.2 ± 2.3 mg g−1 (equivalent to 2749–3455 mg L−1), whereas in OGTIs it was between 122.6 ± 1.6 and 154.1 ± 0.7 mg g−1 (equivalent to 1634–2055 mg L−1). The higher surface area for CTC green tea particles led to higher extraction rate which is due to dissolution of biochemical compounds on surface.24,35
3.7. Theanine
Theanine was found in all green tea samples and their contents in green tea, in infusion, and its transfer rate into infusion are presented in Fig. 2(c). Theanine content in green teas varied from 2.9 ± 0.2 mg g−1 in Ging186 to 13.3 ± 0.3 mg g−1 in TV20. The cultivar TV20 had significantly higher (p ≤ 0.05) theanine content from all other cultivars. In line with our results, Too et al. reported theanine level in the range between 1.4 and 15.0 mg g−1 in green tea manufactured from Kenyan tea cultivars using HPLC.36 Kilel et al. reported a higher level of theanine (15.0–21.8 mg g−1) in green tea processed from purple tea cultivars of Kenya.18 Theanine content in Longjing green tea varied between 5.68 and 17.36 mg g−1.22 Theanine contents in infusions were positively influenced by CTC step during processing. The CGTIs had 19% (on average) more theanine content than OGTIs. Theanine levels in CGTIs were in the range between 1.9 ± 0.1 and 6.3 ± 0.1 mg g−1 (equivalent to 24.7–84.4 mg L−1), whereas in OGTIs it was between 1.0 ± 0.1 and 3.8 ± 0.1 mg g−1 (equivalent to 12.9–50.5 mg L−1). The stripping of the leaf epidermis and maceration during CTC step led to microstructural changes in cells which efficiently exposed its components.37 The findings in the present work support the reported theanine level (2.58–4.23 mg g−1) in twenty-seven famous commercial teas of UK.38 Liu et al. reported higher levels of theanine (38.5–272 mg L−1) in green tea infusions determined by derivatization of infusion using 2,4-dinitrofluorobenzene, and detected at 360 nm using HPLC.23
3.8. Sensory evaluation
The overall taste of green tea is characterized by the basic tastes comprising bitterness, astringency and umami,39 with astringency and bitterness being the primary ones.11 The overall acceptability of the CTC green teas (score: 6.1–8.0) was slightly lower than its orthodox counterpart (score: 6.5–8.6) (Table 1). The difference in taste profile can be attributed to the difference in biochemical constituents contributing to taste. The gallated and non-gallated catechins had a profound effect on the intensities of astringency, bitterness and sweetness as reported by Cao et al.40 The intensities of astringency (R2 = 0.89, p ≤ 0.01 for both orthodox and CTC); and bitterness (R2 = 0.78, p ≤ 0.01 for orthodox and R2 = 0.72, p ≤ 0.01 for CTC) were positively correlated with the concentration of gallated catechins. Non gallated catechins had positive correlation with sweetness (R2 = 0.88, p ≤ 0.01 for orthodox and R2 = 0.71, p ≤ 0.01 for CTC) and overall acceptability (R2 = 0.85, p ≤ 0.01 for orthodox and R2 = 0.77, p ≤ 0.01 for CTC). The scores for umami taste had strong positive correlation (R2 = 0.98, p ≤ 0.01 for orthodox and R2 = 0.96, p ≤ 0.01 for CTC) with theanine content. Previous studies also reported a profound effect of free amino acids present in infusion on umami taste.11
Table 1 Taste attributes of orthodox and CTC green tea infusionsa
Cultivar |
Orthodox |
CTC |
Bitterness |
Astringency |
Umami |
Sweetness |
Overall acceptability |
Bitterness |
Astringency |
Umami |
Sweetness |
Overall acceptability |
Values are means (±SE) of three replications. |
TV1 |
2.30 ± 0.06 |
5.10 ± 0.21 |
6.50 ± 0.23 |
4.10 ± 0.06 |
6.80 ± 0.06 |
2.20 ± 0.06 |
5.20 ± 0.29 |
5.90 ± 0.21 |
3.70 ± 0.12 |
6.50 ± 0.35 |
TV9 |
2.30 ± 0.15 |
6.00 ± 0.06 |
7.90 ± 0.35 |
4.80 ± 0.06 |
6.80 ± 0.23 |
2.30 ± 0.06 |
5.90 ± 0.17 |
7.70 ± 0.23 |
4.50 ± 0.23 |
6.30 ± 0.17 |
TV18 |
2.40 ± 0.12 |
6.10 ± 0.29 |
6.60 ± 0.40 |
4.60 ± 0.12 |
6.50 ± 0.12 |
2.20 ± 0.17 |
6.20 ± 0.17 |
6.30 ± 0.29 |
4.50 ± 0.12 |
6.20 ± 0.17 |
TV20 |
2.20 ± 0.06 |
5.70 ± 0.12 |
8.20 ± 0.12 |
5.90 ± 0.12 |
8.40 ± 0.23 |
2.10 ± 0.06 |
5.80 ± 0.23 |
8.00 ± 0.17 |
5.30 ± 0.23 |
8.00 ± 0.17 |
TV22 |
2.20 ± 0.15 |
5.90 ± 0.21 |
7.50 ± 0.49 |
6.20 ± 0.17 |
7.90 ± 0.12 |
2.00 ± 0.12 |
5.80 ± 0.12 |
7.00 ± 0.40 |
5.90 ± 0.23 |
7.70 ± 0.17 |
TV23 |
2.20 ± 0.12 |
5.80 ± 0.21 |
6.50 ± 0.17 |
6.60 ± 0.23 |
8.40 ± 0.17 |
2.20 ± 0.17 |
6.00 ± 0.29 |
6.50 ± 0.17 |
6.40 ± 0.17 |
8.00 ± 0.29 |
TV25 |
2.40 ± 0.17 |
6.10 ± 0.17 |
5.90 ± 0.15 |
5.70 ± 0.12 |
7.20 ± 0.17 |
2.30 ± 0.12 |
6.30 ± 0.29 |
5.50 ± 0.23 |
6.30 ± 0.29 |
7.00 ± 0.23 |
HV39 |
2.40 ± 0.06 |
6.00 ± 0.12 |
3.90 ± 0.06 |
5.80 ± 0.06 |
7.80 ± 0.35 |
2.40 ± 0.12 |
6.20 ± 0.23 |
3.70 ± 0.06 |
5.80 ± 0.29 |
6.90 ± 0.35 |
RR17/144 |
2.20 ± 0.12 |
4.80 ± 0.06 |
6.20 ± 0.17 |
6.00 ± 0.12 |
8.60 ± 0.35 |
2.10 ± 0.06 |
5.00 ± 0.17 |
6.00 ± 0.23 |
6.10 ± 0.17 |
7.90 ± 0.23 |
Ging186 |
3.50 ± 0.10 |
6.30 ± 0.20 |
3.50 ± 0.06 |
5.00 ± 0.17 |
6.90 ± 0.12 |
3.30 ± 0.06 |
6.40 ± 0.17 |
3.40 ± 0.12 |
5.10 ± 0.06 |
6.10 ± 0.23 |
482/12 |
3.90 ± 0.15 |
6.20 ± 0.25 |
4.30 ± 0.12 |
5.70 ± 0.12 |
7.80 ± 0.23 |
3.80 ± 0.12 |
6.30 ± 0.12 |
4.30 ± 0.23 |
5.60 ± 0.17 |
7.50 ± 0.12 |
3.9. Fluoride and chloride content
Fluoride prevents dental caries, although excessive exposure to high levels may lead to skeletal and dental fluorosis.41 The CGTIs had higher levels of fluoride than OGTIs (please see ESI Table S1†). Fluoride content ranged from 0.5 to 1.1 mg L−1 (mean 0.83 mg L−1) in CGTIs, whereas it was from 0.4 to 0.9 mg L−1 (mean 0.63 mg L−1) in OGTIs. Based on World Health Organisation (WHO) guideline on fluoride in drinking water (1.5 μg mL−1) all the green tea samples are safe for human consumption with respect to fluoride content.42 The fluoride content, determined using fluoride selective electrode, in infusions of green tea available in Portugal was much higher (0.80–2.0 mg L−1, mean 1.20 mg L−1) than the results being reported here.30 In a study conducted in Republic of Ireland, Waugh et al. reported mean fluoride content of 3.3 mg L−1 in commercially available black tea samples demonstrating the risk of health hazards from fluoride exposure.43 The mean chloride contents were determined to be 19.02 and 14.24 mg L−1 in CGTIs and OGTIs, respectively (please see ESI Table S1†). The current results are in disparity with previously documented work which reported much higher chloride content (86.0 mg L−1) in green tea infusions in Romania using ion chromatography.44
3.10. Sodium and potassium content
The CGTIs had a higher content of sodium and potassium than OGTIs (please see ESI Table S1†). Sodium contents were in the range from 1.2 to 2.6 mg L−1 (mean 1.67 mg L−1) in CGTIs, whereas it was from 1.0 ± 0.1 to 2.1 ± 0.1 mg L−1 (mean 1.28 mg L−1) in OGTIs. Fernandez et al. reported a similar level of sodium content (0.4–3.3 mg L−1) in twenty green tea samples from different geographical origin using ICP-AES.45 Reto et al. demonstrated a much higher level of sodium (36–69 mg L−1) in the infusions of green tea commercialized in Portugal.30 The variation in concentration of sodium can be attributed to its high extractability and wide range of its content in green leaves. Islam and Ebihara determined sodium and potassium content in Japanese green tea using combined PGA (neutron-induced prompt gamma-ray analysis) and INAA (instrumental neutron activation analysis) technique. The authors reported sodium level in the range 5.16–53.6 μg g−1 with an extraction efficiency of 59% to the infusion (equivalent to 0.03–0.32 mg L−1) which is much lower than the present findings.46 The potassium contents were in the range between 138.7 ± 4.7 and 281.6 ± 5.9 mg L−1 (mean 225.79 mg L−1) in CGTIs, whereas it was between 89.3 ± 2.7 and 179.5 ± 3.1 mg L−1 (mean of 152.89 mg L−1) in OGTIs. Our findings are in conformity with a previous report of potassium level in the range from 94 to 259 mg L−1 determined using ICP-AES.45 Potassium level in Japanese green tea was demonstrated in the range 15
400 to 21
900 μg g−1 with an infusion transfer rate of 71% (equivalent to 109.34–155.49 mg L−1) which is in agreement with the current results.46 However, Reto et al. reported much lower mean potassium content (114.77 mg L−1) in nine Portuguese tea samples.30
3.11. Risk assessment
The EDI results are presented in Fig. 3(a) and (b) for caffeine and EGCG, respectively. The average EDI of caffeine in mg kg−1 BW was 2.70 and 2.29 for men; 2.80 and 2.37 and for women in CTC and orthodox green tea, respectively. Nawrot et al. reported that daily caffeine intake within the level of 6 mg kg−1 BW for a healthy adult had no adverse health effect like toxicity, increased cancer risk, cardiovascular effects, decreased male fertility, etc.47 The authors also suggested that women fertility is not affected on consuming caffeine within the level of 4.6 mg per kg BW per day and children should not consume beyond 2.5 mg per kg BW per day. The EDI levels of caffeine in the present study are well within the limit of risk as suggested by Nawrot et al.47
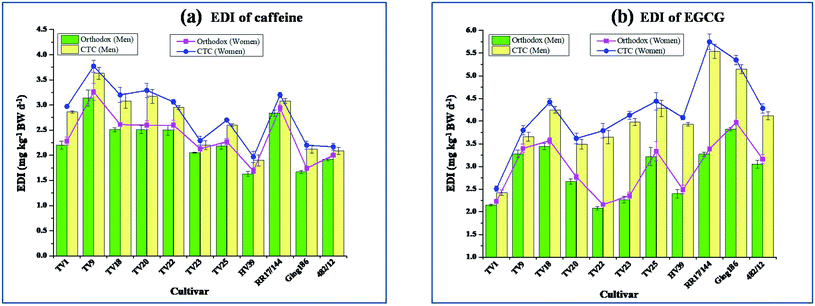 |
| Fig. 3 Estimated daily intake (EDI) of caffeine (a), and EGCG (b) for both men and women on consumption of five cups (equivalent to 10 g) of green tea [average body weight (BW) considered for men and women were 67.4 and 64.9 kg, respectively]. | |
The average EDI of EGCG in mg kg−1 BW was 4.04 (equivalent to 272.30 mg per day) and 2.88 (equivalent to 194.11 mg per day) for men; 4.19 (equivalent to 271.93 mg per day) and 2.99 (equivalent to 194.05 mg per day) for women in CTC and orthodox green tea, respectively. EGCG is reportedly suspected as one of the causes for liver toxicity in human.48 European Food Safety Authority (EFSA) on their study on the association of EGCG intake and hepatotoxicity demonstrated that a small group of population experienced an elevated level of ALT (alanine aminotransferase) and AST (aspartate aminotransferase) on consuming a dose of 800 mg per day for a period extending beyond 4 months.49 A dose of 316 mg per day did not show any elevation of serum levels of transaminases. The EFSA panel did not find any evidence of ALT level elevation at a dose of 700 mg EGCG per day. Dekant et al. proposed an EGCG dose of 300 mg per day as safe for human consumption.48 The data from the present study revealed the safety of these green teas from the risk of hepatotoxicity.
4. Conclusion
This study provides comparative information on the quality components of orthodox and CTC green tea to judge the preferred processing mode with improved quality. The results demonstrated that CTC green teas had significantly high extraction efficiency of bioactive components than orthodox ones which led to a high content of total polyphenol, catechins, theanine and water extract in CTC green tea infusions. The extraction efficiency of total polyphenol, total catechin, theanine and water extract in CTC green tea were 13.3, 7.5, 18.6, and 17.1%, respectively, higher in comparison to orthodox green tea. This study provides a new fundamental basis of the economic efficiency of CTC green tea in the light of the higher transfer rate of bioactive compounds from tea to its infusion. The study also suggests that EGCG intake from consumption of five cups (10 g) of tea infusion a day is free from the risk of hepatotoxicity and caffeine intake from the same quantity of tea will not inflict any health hazard. Infusion prepared from orthodox green tea had preferred sensory qualities as compared with that of CTC green tea, with lower astringency, and higher umami and overall acceptability score. In spite of the slight low tasters score, the economic efficiency of the CTC green tea, in terms of cuppage, is thought provoking. The current study also found that the cultivars TV20, TV23, and RR17/144 had a high level of overall acceptability tasters score (>8.0).
Abbreviations
BW | Body weight |
CGTI | CTC green tea infusion |
CTC | Curl, tear and crush |
EC | (−)-Epicatechin |
ECG | (−)-Epicatechin-3-gallate |
EDI | Estimated daily intake |
EFSA | European Food Safety Authority |
EGC | (−)-Epigallocatechin |
EGCG | (−)-Epigallocatechin-3-gallate |
FBD | Fluid bed dryer |
GAE | Gallic acid equivalent |
OGTI | Orthodox green tea infusion |
TC | Total catechin |
TE | Tea estate |
TP | Total polyphenols |
TPI | Teeth per inch |
TTRI | Tocklai Tea Research Institute |
TV | Tocklai vegetative |
WE | Water extract |
Author contributions
H. Deka designed the research, collected green leaves from field, processed all green teas, carried out the biochemical analysis, statistical analysis, interpreted the results, and drafted the manuscript. T. Barman analysed the macroelements, fluoride, chloride and actively helped in editing the manuscript. P. P. Sarmah helped in preparing tables, figures and overall improvement of the manuscript. P. Tamuly arranged sensory evaluation of samples. A. Devi retouched the manuscript. R. K. Paul carried out statistical analysis and interpretation of the data set. T. Karak guided in preparing the manuscript, revised it for technical and intellectual content.
Conflicts of interest
The authors declare no conflict of interest.
Acknowledgements
Mr Prodip Saikia, Tocklai Tea Research Institute (TTRI) helped actively in green tea processing. We acknowledge the help of Dr Santanu Sabhapondit, TTRI in determination of theanine and Mr Nabajyoti Neog, TTRI in sample collection. The authors sincerely acknowledge Dr A. K. Barooah, Director, TTRI for his support. Finally, we would like to express our sincere thanks and gratitude to the two anonymous reviewers for their valuable suggestions that helped us a lot in improving this manuscript.
References
- W. Reygaert, Beverages, 2017, 3, 6 CrossRef.
- G. M. Lowe, K. Gana and K. Rahman, J. Complementary Integr. Med., 2015, 12, 277–282 CAS.
- X. Gao, A. Cassidy, M. A. Schwarzschild, E. B. Rimm and A. Ascherio, Neurology, 2012, 78, 1138–1145 CrossRef CAS.
- L. Yang, X. Jin, J. Yan, Y. Jin, W. Yu, H. Wu and S. Xu, Age Ageing, 2016, 45, 708–712 Search PubMed.
- Y. H. Lee, Y. H. Jang, Y. Kim, J. Kim and B. L. Seong, J. Biol. Eng., 2018, 12, 1–10 CrossRef.
- H. S. Oz, T. S. Chen, C. J. Mcclain and W. J. S. De Villiers, J. Nutr. Biochem., 2005, 16, 297–304 CrossRef CAS.
- H. S. Oz, C. J. Mcclain, H. T. Nagasawa, M. B. Ray, W. J. S. De Villiers and T. S. Chen, J. Biochem. Mol. Toxicol., 2004, 18, 361–368 CrossRef CAS.
- K. Ide, H. Yamada, N. Takuma, M. Park, N. Wakamiya, J. Nakase, Y. Ukawa and Y. M. Sagesaka, Nutrients, 2014, 6, 4032–4042 CrossRef.
- A. Scholey, L. A. Downey, J. Ciorciari, A. Pipingas, K. Nolidin, M. Finn, M. Wines, S. Catchlove, A. Terrens, E. Barlow, L. Gordon and C. Stough, Appetite, 2012, 58, 767–770 CrossRef CAS.
- L. Xing, H. Zhang, R. Qi, R. Tsao and Y. Mine, J. Agric. Food Chem., 2019, 67, 1029–1043 CrossRef CAS.
- Y. Q. Xu, W. Bin Ji, P. Yu, J. X. Chen, F. Wang and J. F. Yin, Food Chem., 2018, 248, 146–154 CrossRef CAS.
- M. E. Harbowy and D. A. Balentine, Crit. Rev. Plant Sci., 1997, 16, 415–480 CrossRef CAS.
- Y. N. Zhang, J. F. Yin, J. X. Chen, F. Wang, Q. Z. Du, Y. W. Jiang and Y. Q. Xu, Food Chem., 2016, 192, 470–476 CrossRef CAS.
- Y. Wang, Z. Kan, H. J. Thompson, T. Ling, C. T. Ho, D. Li and X. Wan, J. Agric. Food Chem., 2019, 67, 5423–5436 CrossRef CAS.
- Z. X. Han, M. M. Rana, G. F. Liu, M. J. Gao, D. X. Li, F. G. Wu, X. B. Li, X. C. Wan and S. Wei, Food Chem., 2016, 212, 739–748 CrossRef CAS.
- R. Baba and K. Kumazawa, J. Agric. Food Chem., 2014, 62, 8308–8313 CrossRef CAS.
- B. C. Barbora and S. C. Saha, Two Bud, 1995, 42, 12–13 Search PubMed.
- E. C. Kilel, A. K. Faraj, J. K. Wanyoko, F. N. Wachira and V. Mwingirwa, Food Chem., 2013, 141, 769–775 CrossRef CAS.
- A. Mao, H. Su, S. Fang, X. Chen, J. Ning, C. Ho and X. Wan, Int. J. Food Sci. Technol., 2018, 53, 2586–2594 CrossRef CAS.
- T. Barman, A. K. Barooah, B. C. Goswami, N. Sharma, S. Panja, P. Khare and T. Karak, Biol. Trace Elem. Res., 2020, 196, 318–329 CrossRef CAS.
- Q. Dai, S. Liu, Y. Jiang, J. Gao, H. Jin, Y. Zhang, Z. Zhang and T. Xia, J. Food Sci. Technol., 2019, 56, 4333–4348 CrossRef CAS.
- K. Wang and J. Ruan, Int. J. Food Sci. Technol., 2009, 44, 2476–2484 CrossRef CAS.
- Y. Liu, L. Luo, C. Liao, L. Chen, J. Wang and L. Zeng, Food Chem., 2018, 269, 24–34 CrossRef CAS.
- R. A. Farakte, G. Yadav, B. Joshi, A. W. Patwadhan and G. Singh, Int. J. Food Eng., 2016, 12, 1–16 CAS.
- C. D. Fernando and P. Soysa, Nutr. J., 2015, 14, 1–7 CrossRef CAS.
- D. Komes, D. Horžić, A. Belščak, K. K. Ganić and I. Vulić, Food Res. Int., 2010, 43, 167–176 CrossRef CAS.
- M. Wakamatsu, H. Yamanouchi, H. Sahara, T. Iwanaga, R. Kuroda, A. Yamamoto, Y. Minami, M. Sekijima, K. Yamada and K. Kajiya, Food Sci. Nutr., 2019, 7, 2769–2778 CAS.
- X. Q. Zheng, Y. Nie, Y. Gao, B. Huang, J. H. Ye, J. L. Lu and Y. R. Liang, J. Food Compos. Anal., 2018, 67, 29–37 CrossRef CAS.
- K. Wei, L. Wang, J. Zhou, W. He, J. Zeng, Y. Jiang and H. Cheng, Food Chem., 2011, 125, 44–48 CrossRef CAS.
- M. Reto, M. E. Figueira, H. M. Filipe and C. M. M. Almeida, Plant Foods Hum. Nutr., 2007, 62, 139–144 CrossRef CAS.
- Y. Q. Xu, Y. N. Zhang, J. X. Chen, F. Wang, Q. Z. Du and J. F. Yin, Food Chem., 2018, 258, 16–24 CrossRef CAS.
- P. O. Owuor and C. O. Othieno, J. Sci. Food Agric., 1989, 49, 87–94 CrossRef CAS.
- X. He, J. Li, W. Zhao, R. Liu, L. Zhang and X. Kong, Food Chem., 2015, 171, 405–411 CrossRef CAS.
- Food Safety and Standard Authority of India, Food safety and standards (food products standards and food additives) regulations, 2011 Search PubMed.
- R. S. S. Kumar, N. N. Muraleedharan, S. Murugesan, G. Kottur, M. P. Anand and A. Nishadh, Food Chem., 2011, 129, 117–124 CrossRef.
- T. C. Janet, W. K. John, K. Thomas, M. O. Kelvin and W. N. Francis, Food Nutr. Sci., 2015, 06, 1450–1459 CAS.
- P. K. Mahanta and M. Hazarika, J. Sci. Food Agric., 1985, 36, 1133–1139 CrossRef CAS.
- E. K. Keenan, M. D. A. Finnie, P. S. Jones, P. J. Rogers and C. M. Priestley, Food Chem., 2011, 125, 588–594 CrossRef CAS.
- J. F. Yin, Y. N. Zhang, Q. Z. Du, J. X. Chen, H. B. Yuan and Y. Q. Xu, Food Res. Int., 2014, 62, 941–946 CrossRef CAS.
- Q. Q. Cao, C. Zou, Y. H. Zhang, Q. Z. Du, J. F. Yin, J. Shi, S. Xue and Y. Q. Xu, Food Chem., 2019, 277, 432–437 CrossRef CAS.
- K. O. Moseti, T. Kinyanjui, J. K. Wanyoko and F. N. Wachira, Afr. Crop Sci. J., 2014, 22, 897–904 Search PubMed.
- World Health Organization, Guidelines for Drinking-water Quality, 4th edn, 2011 Search PubMed.
- D. T. Waugh, W. Potter, H. Limeback and M. Godfrey, Int. J. Environ. Res. Public Health, 2016, 13, 259 CrossRef.
- I. Mincă, A. M. Josceanu, R. D. Isopescu and C. Guran, UPB Sci. Bull. Ser. B Chem. Mater. Sci., 2013, 75, 65–78 Search PubMed.
- P. L. Fernández, F. Pablos, M. J. Martín and A. G. González, Food Chem., 2002, 76, 483–489 CrossRef.
- M. A. Islam and M. Ebihara, Arabian J. Chem., 2017, 10, S677–S682 CrossRef CAS.
- P. Nawrot, S. Jordan, J. Eastwood, J. Rotstein, A. Hugenholtz and M. Feeley, Food Addit. Contam., 2003, 20, 1–30 CrossRef CAS.
- W. Dekant, K. Fujii, E. Shibata, O. Morita and A. Shimotoyodome, Toxicol. Lett., 2017, 277, 104–108 CrossRef CAS.
- M. Younes, P. Aggett, F. Aguilar, R. Crebelli, B. Dusemund, M. J. Frutos, P. Galtier, D. Gott, U. Gundert-remy, J. Leblanc, I. T. Lillegaard, P. Moldeus, A. Mortensen, A. Oskarsson, I. Stankovic, I. Waalkens-berendsen, R. A. Woutersen, R. J. Andrade, C. Fortes, P. Mosesso, P. Restani, D. Arcella, F. Pizzo, C. Smeraldi and M. Wright, EFSA J., 2018, 16, 5239 Search PubMed.
Footnote |
† Electronic supplementary information (ESI) available. See DOI: 10.1039/d0ra06254e |
|
This journal is © The Royal Society of Chemistry 2020 |
Click here to see how this site uses Cookies. View our privacy policy here.