DOI:
10.1039/D0RA06913B
(Paper)
RSC Adv., 2020,
10, 33450-33454
Regio- and stereoselective thiocyanatothiolation of alkynes and alkenes by using NH4SCN and N-thiosuccinimides†
Received
11th August 2020
, Accepted 1st September 2020
First published on 10th September 2020
Abstract
A highly regioselective thiocyanatothiolation of alkynes and alkenes assisted by hydrogen bonding under simple and mild conditions is developed. Our thiocyanatothiolation reagents are readily available ammonium thiocyanate and N-thiosuccinimides. This metal-free system offers good chemical yields for a wide range of alkyne and alkene substrates with good functional group tolerance.
Sulfur-containing molecules are ubiquitous structural motifs and widely exist in natural products,1,2 pharmaceuticals3,4 and agrochemicals.5–7 Examples include the nonsteroidal anti-inflammatory drug Sulindac,8 the basal-cell carcinoma treatment drug Vismodegib,9 and drugs for the treatment of Parkinson's disease.10 Therefore, efficient introduction of sulfur into organic molecules has drawn much attention.11–15 And numerous approaches for the formation of C–S bonds have been developed.16–20 The most used organosulfur sources for the formation of C–S bonds are thiols and thiophenols, which have an unpleasant smell. Recently, inorganic metal sulfides have been extensively used to construct C–S bonds, such as sodium metabisulfite,21 K2S,22 Na2S23 and Na2S2O3.24 Compared to thiols and thiophenols, inorganic metal sulfides are cheaper and generally stable. Thus, introduction of sulfur-containing groups into molecules by using inorganic metal sulfides is one of the desired approaches. Among them, thiocyanates commonly serve as important precursors for the preparation of thioethers,25 trifluoromethyl sulfides,26 heteroaromatic compounds.27 In general, the sources of SCN used to introduce a sulfur-containing group into molecules are thiocyanate salts28–35 such as KSCN, NaSCN, AgSCN and NH4SCN. For example, thiocyanate salts were employed in thiocyanation of bromoalkenes via photocatalysis (Scheme 1a).36 Besides, the vinyl thiocyanates could be also obtained by thiocyanation of haloalkynes (Scheme 1b),37 iodothiocyanation of alkynes (Scheme 1c).38 Obviously, difunctionalization of alkynes is the most straightforward protocol to prepare vinyl thiocyanates.
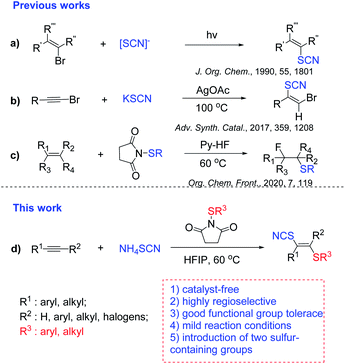 |
| Scheme 1 Methods for thiocyanatothiolation of alkynes and alkenes. | |
Recently, our group has focused on hydrogen-bonding network or cluster39 assisted transformations such as hydrofluorination of ynamides40 and alkenes,41 the addition of sulfonic acids to haloalkynes,42 fluorothiolation of alkenes,20 dihalogenation of alkynes43 and hydrochlorination of alkynes,44–46halothiolation of alkynes.47 Along this line, herein, we are glad to report a hydrogen bond network-enabled regio- and stereoselective thiocyanatothiolation of alkynes using NH4SCN and N-thiosuccinimides.
Initially, according to the previous report,20 we started the investigation of thiocyanatothiolation protocol using NH4SCN and N-(phenylthio)succinimide as thiolation reagents in DCM under air and carried out the reaction at 60 °C (Table 1). To our delight, the desired product 3a was obtained in 42% yield without any isomers found in the reaction mixture detected by GC-MS (Table 1, entry 1). Screening of solvents indicated that this transformation could not proceed in the polar solvents, such as acetone, THF, dioxane, i-PrOH, DMF (Table 1, entries 3–6) probably due to the solvation of electrophiles while moderate yield could be obtained in non-polar solvent (Table 1, entry 2). Strong hydrogen-bond donor solvents such as hexafluoro-2-propanol (HFIP), could form an H-bond network activating the electrophiles through a strong hydrogen bonding interaction.48 In order to enhance the H-bond interaction between the hydroxyl and 2, so AcOH was chosen to compare with HFIP (Table 1, entry 7). Along this line, hydrogen-bond donor solvents were used and further optimization of hydrogen-bond donor solvents indicated that HFIP was superior to AcOH and trifluoroethanol (Table 1, entries 7–9). Moreover, a screening of thiocyanate salts showed that NH4SCN was the best SCN source for this transformation compared with lithium thiocyanate, sodium thiocyanate and potassium thiocyanate (Table 1, entries 10–12). Additionally, decreasing the temperature from 60 °C to room temperature resulted in a lower yield (Table 1, entry 13) and the reaction yield was not improved significantly by raising the temperature from 60 °C to 80 °C (Table 1, entry 14).
Table 1 Optimization for the reaction conditions

|
Entrya |
[SCN] |
Solvent |
Temp. (°C) |
Yieldb (%) |
Reaction conditions: 1 (0.1 mmol), 2 (0.12 mmol), NH4SCN (0.2 mmol), solvent (0.5 mL), under air for 12 h at 60 °C. Determined by GC. |
1 |
NH4SCN |
DCM |
60 |
42 |
2 |
NH4SCN |
DCE |
60 |
47 |
3 |
NH4SCN |
THF |
60 |
0 |
4 |
NH4SCN |
Acetone |
60 |
0 |
5 |
NH4SCN |
DMF |
60 |
0 |
6 |
NH4SCN |
iPrOH |
60 |
0 |
7 |
NH4SCN |
AcOH |
60 |
24 |
8 |
NH4SCN |
TFE |
60 |
18 |
9 |
NH4SCN |
HFIP |
60 |
87 |
10 |
LiSCN |
HFIP |
60 |
36 |
11 |
NaSCN |
HFIP |
60 |
42 |
12 |
KSCN |
HFIP |
60 |
49 |
13 |
NH4SCN |
HFIP |
25 |
63 |
14 |
NH4SCN |
HFIP |
80 |
83 |
With the optimized conditions in hand, we next turned our attention to explore the substrate scope (Table 2). Firstly, N-(p-methoxyphenylthio)succinimide was used as electrophile to explore the scope of alkynes. In general, the reaction proceeded well to provide the desired products 3 in moderate to excellent yields with satisfactory regio- and stereoselectivity. Diverse aryl alkynes containing electron-donating groups such as isopropyl, hydroxy, methoxy, hydroxyethyl, tert-butyl and trifluoromethoxy groups (Table 2, 3e–3g and 3m–3p) at the ortho, meta, or para positions of aryl rings all reacted with N-thiosuccinimides to give the corresponding adducts in moderate to excellent yields. Besides, halide substitutes (F, Cl, Br) (Table 2, 3b, 3j–3l and 3w) and electron-withdrawing groups such as cyano and ester (Table 2, 3h and 3i) on phenyl ring were well tolerated. Furthermore, asymmetric or symmetrical internal alkynes also could be transformed into vinyl thiocyanates (Table 2, 3r, 3s and 3w) without any isomers. Remarkably, vinyl thiocyanates containing halogens could be obtained by using haloalkynes (Table 2, 3t–3v). Additionally, slightly low yields were observed for fused aromatic such as naphthalene and heterocyclic aromatic (Table 2, 3x and 3y). Due to good functional-group tolerance, derivatives of diacetone-D-glucose (Table 2, 3z), natural products L-menthol (Table 2, 3aa) and pharmaceuticals such as zaltoprofen (Table 2, 3ab) also worked well.
Table 2 Scope for thiocyanatothiolation of alkynes and N-arylsulfenylsuccinimidesa,b
Reaction conditions: 1 (0.1 mmol), 2 (0.12 mmol), NH4SCN (0.2 mmol), HFIP (0.5 mL), under air for 12 h at 60 °C. Isolated yield. Ar = Ph. Determined by NMR. |
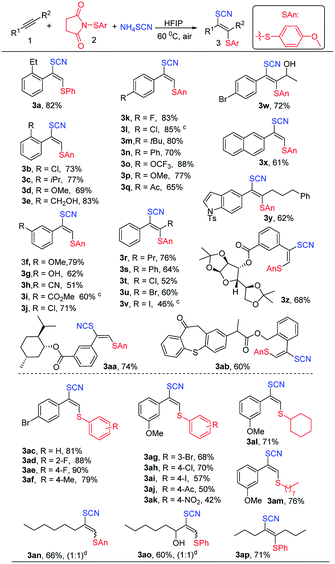 |
Next, we started to explore the scope of N-arylsulfenylsuccinimides. Various N-arylsulfenylsuccinimides can be obtained easily by the method in ESI.† To our delight, the introduction of electron-donating groups or halide substitutes to the phenyl ring of N-arylsulfenylsuccinimides had little influence on this reaction, providing the corresponding products in 57–90% yields (Table 2, 3ac–3ak) while electron-withdrawing groups on the phenyl ring such as acetyl or nitro resulted in lower yields (Table 2, 3aj and 3ak) probably due to the decrease of electrophilicity of N-arylsulfenylsuccinimides. Notably, the scope of N-sulfenylsuccinimides could be extended to N-alkylsulfenylsuccinimides (Table 2, 3al and 3am), affording the desired products with good yields and high selectivity. Unfortunately, the thiocyanatothiolated products (Table 2, 3an and 3ao) with poor stereoselectivity (Z/E = 1
:
1) were obtained when the unsymmetrically aliphatic alkynes were employed. We speculated that the steric hindrance of the aliphatic side chain maybe is small, resulting in a low Z/E ratios.
Encouraged by the success of thiocyanatothiolation of alkynes, we next turned our focus to the thiocyanatothiolation of alkenes. Under the optimized conditions, no product was observed. And the HFIP as nucleophile replaced the NH4SCN, giving hexafluoroisopropanol thiolated product. As result, non-polar solvent DCE was used to avoid the hexafluoroisopropanol thiolat of alkenes. To our delight, the thiocyanatothiolation of alkenes could proceed smoothly though moderate or lower chemical yields were obtained. Among them, aromatic alkenes gave moderate yields without any isomers (Table 3, 5a–5e) and aliphatic alkenes gave lower yields (Table 3, 5f–5h).
Table 3 Scope for thiocyanatothiolation of alkenesa,b
Reaction conditions: 4 (0.1 mmol), 2 (0.12 mmol), NH4SCN (0.2 mmol), DCE (1.0 mL), under air for 12 h at 60 °C. Isolated yield. |
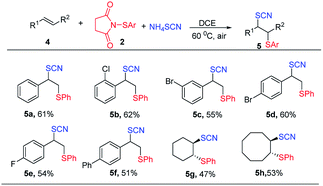 |
To demonstrate the scalability of this protocol, a gram-scale reaction of 1,1′-biphenyl-4-ethynyl (6 mmol) with N-(4-bromo thio)succinimide was carried out, and the corresponding product 3aq was obtained in 62% yield (Scheme 2).
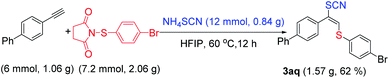 |
| Scheme 2 Gram-scale preparation of 3aq. | |
To identify the configuration, the single crystal of product 3aq was cultivated by solvent evaporation. And the regio- and stereoselectivity of products were further confirmed the X-ray crystallographic analysis of the obtained product 3aq (Fig. 1).
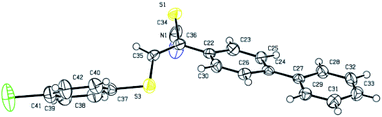 |
| Fig. 1 Single crystal structure of 3aq. | |
Based on our previous work,47 a plausible reaction pathway was proposed in Scheme 3. The interaction of HFIP hydrogen bonding linear aggregates48 with sulfenylation reagent 2a may strongly activate the sulfenylation reagent, which generates the active intermediate B (Scheme 3). Sequentially, a sulfonium C is produced from intermediate B with an alkyne, followed by a nucleophilic attack of SCN anion to obtain the products 3.
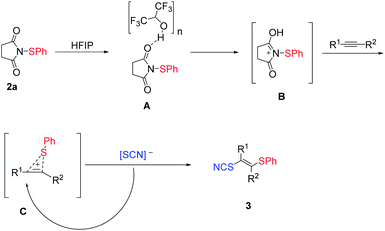 |
| Scheme 3 Plausible mechanism. | |
Conclusions
In summary, we have developed a widely applicable regio- and stereoselective thiocyanatothiolation of alkynes and alkenes under simple and mild conditions. This metal-free system offers good chemical yields and functional group tolerance. At present, the fluorinated reagent HFIP, which is not a green solvent, is indeed a limitation of this method, but as scientific research continues, we believe that green fluorinated reagents can be discovered. Other similar thiolation systems are currently investigated in our laboratory.
Conflicts of interest
There are no conflicts to declare.
Acknowledgements
We are grateful to the National Science Foundation of China (NSFC-21672035), Jiangsu Vocational College of Medicine (20186104) and Jiangsu Provincial Higher Education Natural Science Foundation (19KJB350010) for financial support.
Notes and references
- M. Fontecave, S. Ollagnier-de-Choudens and E. Mulliez, Biological Radical Sulfur Insertion Reactions, Chem. Rev., 2003, 103, 2149–2166 CrossRef CAS.
- S. Oida, Y. Tajima, T. Konosu, Y. Nakamura, A. Somada, T. Tanaka, S. Habuki, T. Harasaki, Y. Kamai, T. Fukuoka, S. Ohya and H. Yasuda, Synthesis and Antifungal Activities of R-102557 and Related Dioxane-Triazole Derivatives, Chem. Pharm. Bull., 2000, 48, 694–707 CrossRef CAS.
- E. A. Ilardi, E. Vitaku and J. T. Njardarson, Data-Mining for Sulfur and Fluorine: An Evaluation of Pharmaceuticals To Reveal Opportunities for Drug Design and Discovery, J. Med. Chem., 2014, 57, 2832–2842 CrossRef CAS.
- I. P. Beletskaya and V. P. Ananikov, Transition-Metal-Catalyzed C−S, C−Se, and C−Te Bond Formation via Cross-Coupling and Atom-Economic Addition Reactions, Chem. Rev., 2011, 111, 1596–1636 CrossRef CAS.
- C. Jacob, E. Battaglia, T. Burkholz, D. Peng, D. Bagrel and M. Montenarh, Control of Oxidative Posttranslational Cysteine Modifications: From Intricate Chemistry to Widespread Biological and Medical Applications, Chem. Res. Toxicol., 2012, 25, 588–604 Search PubMed.
- J. E. Casida, Unexpected Metabolic Reactions and Secondary Targets of Pesticide Action, J. Agric. Food Chem., 2016, 64, 4471–4477 CrossRef CAS.
- J. E. Casida and K. A. Durkin, Pesticide Chemical Research in Toxicology: Lessons from Nature, Chem. Res. Toxicol., 2017, 30, 94–104 Search PubMed.
- A. A. Constantinescu, K. Caliskan, O. C. Manintveld, R. van Domburg, L. Jewbali and A. H. M. M. Balk, Weaning from inotropic support and concomitant beta-blocker therapy in severely ill heart failure patients: take the time in order to improve prognosis, Eur. J. Heart Failure, 2014, 16, 435–443 CrossRef CAS.
- A. M. Giannetti, H. Wong, G. J. P. Dijkgraaf, E. C. Dueber, D. F. Ortwine, B. J. Bravo, S. E. Gould, E. G. Plise, B. L. Lum, V. Malhi and R. A. Graham, Identification, Characterization, and Implications of Species-Dependent Plasma Protein Binding for the Oral Hedgehog Pathway Inhibitor Vismodegib (GDC-0449), J. Med. Chem., 2011, 54, 2592–2601 CrossRef CAS.
- S. F. Nielsen, E. Ø. Nielsen, G. M. Olsen, T. Liljefors and D. Peters, Novel Potent Ligands for the Central Nicotinic Acetylcholine Receptor: Synthesis, Receptor Binding, and 3D-QSAR Analysis, J. Med. Chem., 2000, 43, 2217–2226 CrossRef CAS.
- D. Huang, J. Chen, W. Dan, J. Ding, M. Liu and H. Wu, A Metal-Free Sulfenylation and Bromosulfenylation of Indoles: Controllable Synthesis of 3-Arylthioindoles and 2-Bromo-3-arylthioindoles, Adv. Synth. Catal., 2012, 354, 2123–2128 CrossRef CAS.
- C. J. Nalbandian, E. M. Miller, S. T. Toenjes and J. L. Gustafson, A conjugate Lewis base-Brønsted acid catalyst for the sulfenylation of nitrogen containing heterocycles under mild conditions, Chem. Commun., 2017, 53, 1494–1497 RSC.
- D. Zhu, Y. Gu, L. Lu and Q. Shen, N-Difluoromethylthiophthalimide: A Shelf-Stable, Electrophilic Reagent for Difluoromethylthiolation, J. Am. Chem. Soc., 2015, 137, 10547–10553 CrossRef CAS.
- S. Song, Y. Zhang, A. Yeerlan, B. Zhu, J. Liu and N. Jiao, Cs2CO3-Catalyzed Aerobic Oxidative Cross-Dehydrogenative Coupling of Thiols with Phosphonates and Arenes, Angew. Chem., Int. Ed., 2017, 56, 2487–2491 CrossRef CAS.
- S. Vásquez-Céspedes, A. Ferry, L. Candish and F. Glorius, Heterogeneously Catalyzed Direct C·H Thiolation of Heteroarenes, Angew. Chem., Int. Ed., 2015, 54, 5772–5776 CrossRef.
- S. Bhunia, G. G. Pawar, S. V. Kumar, Y. Jiang and D. Ma, Selected Copper-Based Reactions for C−N, C−O, C−S, and C−C Bond Formation, Angew. Chem., Int. Ed., 2017, 56, 16136–16179 CrossRef CAS.
- X. Li, L. Li, X. Mo and D. Mo, Transition-metal-free synthesis of thiocyanato- or nitro-arenes through diaryliodonium salts, Synth. Commun., 2016, 46, 963–970 CrossRef CAS.
- S. Liu, X. Zeng and B. Xu, Hydrogen-Bonding-Network-Assisted Regioselective Trifluoromethylthiolation and Sulfenylation of Electron-Rich (Hetero)arenes, Asian J. Org. Chem., 2019, 8, 1372–1375 CrossRef CAS.
- S. Liu, X. Zeng and B. Xu, Regio- and stereoselective halothiolation of alkynes using lithium halides and N-thiosuccinimides, Org. Chem. Front., 2020, 7, 1690–1695 RSC.
- S. Liu, X. Zeng and B. Xu, Practical fluorothiolation and difluorothiolation of alkenes using pyridine-HF and N-thiosuccinimides, Org. Chem. Front., 2020, 7, 119–125 RSC.
- M. Wang, Q. Fan and X. Jiang, Metal-free construction of primary sulfonamides through three diverse salts, Green Chem., 2018, 20, 5469–5473 RSC.
- W. Tan, C. Wang and X. Jiang, Green carbon disulfide surrogate via a combination of potassium sulfide and chloroform for benzothiazine-thione and benzothiazole-thione construction, Org. Chem. Front., 2018, 5, 2390–2394 RSC.
- J. Wei, Y. Li and X. Jiang, Aqueous Compatible Protocol to Both Alkyl and Aryl Thioamide Synthesis, Org. Lett., 2016, 18, 340–343 CrossRef CAS.
- J. T. Reeves, K. Camara, Z. S. Han, Y. Xu, H. Lee, C. A. Busacca and C. H. Senanayake, The Reaction of Grignard Reagents with Bunte Salts: A Thiol-Free Synthesis of Sulfides, Org. Lett., 2014, 16, 1196–1199 CrossRef CAS.
- F. Ke, Y. Qu, Z. Jiang, Z. Li, D. Wu and X. Zhou, An Efficient Copper-Catalyzed Carbon−Sulfur Bond Formation Protocol in Water, Org. Lett., 2011, 13, 454–457 CrossRef CAS.
- B. Exner, B. Bayarmagnai, F. Jia and L. J. Goossen, Iron-Catalyzed Decarboxylation of Trifluoroacetate and Its Application to the Synthesis of Trifluoromethyl Thioethers, Chem.–Eur. J., 2015, 21, 17220–17223 CrossRef CAS.
- V. Aureggi and G. Sedelmeier, 1,3-Dipolar Cycloaddition: Click Chemistry for the Synthesis of 5-Substituted Tetrazoles from Organoaluminum Azides and Nitriles, Angew. Chem., Int. Ed., 2007, 46, 8440–8444 CrossRef CAS.
- H. Yang, X.-H. Duan, J.-F. Zhao and L.-N. Guo, Transition-Metal-Free Tandem Radical Thiocyanooxygenation of Olefinic Amides: A New Route to SCN-Containing Heterocycles, Org. Lett., 2015, 17, 1998–2001 CrossRef CAS.
- L.-N. Guo, Y.-R. Gu, H. Yang and J. Hu, Transition-metal free thiocyanooxygenation of functionalized alkenes: facile routes to SCN-containing dihydrofurans and lactones, Org. Biomol. Chem., 2016, 14, 3098–3104 RSC.
- B. Chen, S. Guo, X. Guo, G. Zhang and Y. Yu, Selective Access to 4-Substituted 2-Aminothiazoles and 4-Substituted 5-Thiocyano-2-aminothiazoles from Vinyl Azides and Potassium Thiocyanate Switched by Palladium and Iron Catalysts, Org. Lett., 2015, 17, 4698–4701 CrossRef CAS.
- Y. Chen, S. Wang, Q. Jiang, C. Cheng, X. Xiao and G. Zhu, Palladium-Catalyzed Site-Selective sp3 C–H Bond Thiocyanation of 2-Aminofurans, J. Org. Chem., 2018, 83, 716–722 CrossRef CAS.
- L. Zhen, K. Yuan, X.-y. Li, C. Zhang, J. Yang, H. Fan and L. Jiang, Cascade Reaction of Propargyl Amines with AgSCF3, as Well as One-Pot Reaction of Propargyl Amines, AgSCF3, and Di-tert-butyl Peroxide: Access to Allenyl Thiocyanates and Allenyl Trifluoromethylthioethers, Org. Lett., 2018, 20, 3109–3113 CrossRef CAS.
- Y.-F. Zeng, D.-H. Tan, Y. Chen, W.-X. Lv, X.-G. Liu, Q. Li and H. Wang, Direct radical trifluoromethylthiolation and thiocyanation of aryl alkynoate esters: mild and facile synthesis of 3-trifluoromethylthiolated and 3-thiocyanated coumarins, Org. Chem. Front., 2015, 2, 1511–1515 RSC.
- Q. Lin, W. Yang, Y. Yao, S. Chen, Y. Tan, D. Chen and D. Yang, Copper-Catalyzed Diastereoselective 1,2-Difunctionalization of Oxabenzonorbornadienes Leading to β-Thiocyanato Thioethers, Org. Lett., 2019, 21, 7244–7247 CrossRef CAS.
- X. Zeng, B. Chen, Z. Lu, G. B. Hammond and B. Xu, Homogeneous and Nanoparticle Gold-Catalyzed Hydrothiocyanation of Haloalkynes, Org. Lett., 2019, 21, 2772–2776 CrossRef CAS.
- T. Kitamura, S. Kobayashi and H. Taniguchi, Photolysis of vinyl halides. Reaction of photogenerated vinyl cations with cyanate and thiocyanate ions, J. Org. Chem., 1990, 55, 1801–1805 CrossRef CAS.
- G. Jiang, C. Zhu, J. Li, W. Wu and H. Jiang, Silver-Catalyzed Regio- and Stereoselective Thiocyanation of Haloalkynes: Access to (Z)-Vinyl Thiocyanates, Adv. Synth. Catal., 2017, 359, 1208–1212 CrossRef CAS.
- X. Zeng and L. Chen, Iodine-mediated regio- and stereoselective iodothiocyanation of alkynes in aqueous ethanol, Org. Biomol. Chem., 2018, 16, 7557–7560 RSC.
- T. Steiner, The Hydrogen Bond in the Solid State, Angew. Chem., Int. Ed., 2002, 41, 48–76 CrossRef CAS.
- X. Zeng, J. Li, C. K. Ng, G. B. Hammond and B. Xu, (Radio)fluoroclick Reaction Enabled by a Hydrogen-Bonding Cluster, Angew. Chem., Int. Ed., 2018, 57, 2924–2928 CrossRef CAS.
- Z. Lu, X. Zeng, G. B. Hammond and B. Xu, Widely Applicable Hydrofluorination of Alkenes via Bifunctional Activation of Hydrogen Fluoride, J. Am. Chem. Soc., 2017, 139, 18202–18205 CrossRef CAS.
- X. Zeng, S. Liu, Z. Shi and B. Xu, Hydrogen Bonding Cluster-Enabled Addition of Sulfonic Acids to Haloalkynes: Access to Both (E)- and (Z)-Alkenyl Sulfonates, Org. Lett., 2016, 18, 4770–4773 CrossRef CAS.
- X. Zeng, S. Liu, Y. Yang, Y. Yang, G. B. Hammond and B. Xu, Regio- and Stereoselective Synthesis of 1,2-Dihaloalkenes Using In-Situ-Generated ICl, IBr, BrCl, I2, and Br2, Chem, 2020, 6, 1018–1031 CAS.
- R. Ebule, S. Liang, G. B. Hammond and B. Xu, Chloride-Tolerant Gold(I)-Catalyzed Regioselective Hydrochlorination of Alkynes, ACS Catal., 2017, 7, 6798–6801 CrossRef CAS.
- X. Zeng, S. Liu, G. B. Hammond and B. Xu, Hydrogen-Bonding-Assisted Brønsted Acid and Gold Catalysis: Access to Both (E)- and (Z)-1,2-Haloalkenes via Hydrochlorination of Haloalkynes, ACS Catal., 2018, 8, 904–909 CrossRef CAS.
- J. Oliver-Meseguer, A. Doménech-Carbó, M. Boronat, A. Leyva-Pérez and A. Corma, Partial Reduction and Selective Transfer of Hydrogen Chloride on Catalytic Gold Nanoparticles, Angew. Chem., Int. Ed., 2017, 56, 6435–6439 CrossRef CAS.
- S. Liu, X. Zeng and B. Xu, Regio- and stereoselective halothiolation of alkynes using lithium halides and N-thiosuccinimides, Org. Chem. Front., 2020, 7, 1690–1695 RSC.
- S. Henkel, M. C. Misuraca, P. Troselj, J. Davidson and C. A. Hunter, Polarisation effects on the solvation properties of alcohols, Chem. Sci., 2018, 9, 88–99 RSC.
Footnote |
† Electronic supplementary information (ESI) available. CCDC 2022664. For ESI and crystallographic data in CIF or other electronic format see DOI: 10.1039/d0ra06913b |
|
This journal is © The Royal Society of Chemistry 2020 |
Click here to see how this site uses Cookies. View our privacy policy here.