DOI:
10.1039/D0RA07017C
(Paper)
RSC Adv., 2020,
10, 34712-34718
Co0 superparamagnetic nanoparticles stabilized by an organic layer coating with antimicrobial activity
Received
14th August 2020
, Accepted 11th September 2020
First published on 18th September 2020
Abstract
Cobalt (Co) is one of the most promising materials in nanotechnology due to its superior magnetic properties. However, due to the high cytotoxicity of cobalt, the activity in biological systems has been little studied. In this work, we report the structural, morphological, and magnetic properties of cobalt nanoparticles stabilized with an organic layer (Co0@C-NPs) and its potential antimicrobial activity. The Co0@C-NPs were obtained from solvothermal conditions and characterized by X-ray powder diffraction, electronic microscopy, and magnetic measurements. The organic layer was analysed by thermogravimetric analysis, Scanning Electron Microscopy, Energy Dispersive Spectrometer, and Fourier Transform Infrared Spectroscopy. From the TEM image, an organic coating layer is observed around Co0 where this coating prevents NPs from oxidation allowing it to remain stable until 400 °C. Surface composition studies by SEM/EDS allowed the identification of carbon, oxygen, and cobalt elements present in the organic layer. This result was corroborated later by FITR analysis. Preliminary antibacterial properties were also investigated, which showed that the cobalt nanoparticles are active against Staphylococcus aureus after 1 h of exposure. The superparamagnetic properties and organic coating Co0@C-NPs could be biocompatible with biological systems, but more research is needed to apply these nanoparticles in biomedical products.
Introduction
Magnetic nanoparticles (MNPs) have attracted attention due to their potential applications in a wide range of technologies, including environmental remediation, catalysis, magnetic fluids, and magnetic resonance imaging.1–3 In the field of nanotechnology and nanomedicine, magnetic nanoparticles are of interest to researchers in studies of hyperthermia therapy, temperature-triggered drug release,4,5 and cytotoxicity on cancer cells,6 because of their unique physical and chemical properties.7,8 Small sizes and large surface area to volume ratios make nanomaterials very reactive and enable them to interact with the cell wall and genome of bacteria, leading to their antimicrobial activity.9 Among the MNPs, cobalt (Co) is one of the most promising materials and has attracted a great deal of interest for many years from researchers from a wide range of fields for different applications.10 Co nanoparticles exhibit high resistance to oxidation, corrosion, and wear.11 Most importantly, Co-NPs also have an intrinsic advantage in biomedical related fields.10 Due to their superior magnetic properties, Co nanoparticles could be a promising candidate for biomedical applications substituting the almost uniquely used biocompatible iron oxide nanoparticles.5 The activity in biological systems of Co-NPs and nanocomposites has been little studied. Because Co has high cytotoxicity, its passivation with an inert shell is a necessary prerequisite.6 However, some publications show that cobalt nanoparticles and cobalt oxide nanoparticles have been used as antitumor, anticancer, and antimicrobial agents.7,10–12 Also, they have been tested as nanotherapeutic agents against the proliferation of Acanthamoeba castellani,13 an amoeba that can be found in most ecosystems and is responsible for causing many diseases in humans.14 Other studies have published positive results of the antimicrobial action of cobalt cellulose nanocomposite against Gram-positive and Gram-negative bacteria.15 Staphylococcus aureus is a pathogen responsible for serious infections, which can often be treated with broad-spectrum antibiotics. However, due to the growing concern of antimicrobial resistance, alternative antimicrobials are needed.15 Besides, little information is available in the literature on the antimicrobial activity of metallic cobalt nanoparticles (Co0-NPs). However, studies show the use of complex nanostructured systems based on cobalt, such as, for example; rare earth element (REE) cerium (Ce3+) doped CoFe2O4 nanoparticles,16 nitrogen-rich carbon-coated bismuth/cobalt nanoparticles,17 cobalt ferrite-chitosan,18 and Co-doped TiO2 nanostructures,19 which have presented good antimicrobial behavior in Gram-positive and Gram-negative bacteria. Many synthetic methods have been developed to prepare MNPs, including the thermal decomposition method,20 hydrothermal microemulsion process,21 high temperature solution phase method,22 and solvothermal process.23 Solvothermal synthesis has allowed obtaining stable cobalt nanoparticles (Co0-NPs) with sizes smaller than 20 nm and spherical shape.24 Thus, the aim of this study was to develop stable and superparamagnetic Co0@C-NPs through solvothermal technique and analyzed its antimicrobial activity against Staphylococcus aureus.
Experimental
Materials
All the starting materials were commercially available reagents of analytical grade and were used without further purification.
Synthesis of Co0@C-NPs
The Co0@C-NPs were obtained using solvothermal synthesis in the same synthetic conditions, as reported by Paredes-Garcia et al.23 Due to the high stability obtained in nickel nanoparticles synthesis. The NPs were obtained from L-2-amino-3-hydroxypropanoic acid (L-serine) and CoCl2·6H2O in a molar ratio of 1
:
1, using DMF in a 23 mL Teflon-lined stainless steel autoclave.
Characterization
The Co0@C-NPs were characterized by powder X-ray diffraction (PXRD) using a Bruker diffractometer, model D8 Advance with Cu Kα1 radiation and Bragg–Brentano geometry in the 5 ≤ 2q ≤ 80 range. The morphological features of nanoparticles were observed by Transmission Electron Microscope (TEM) model JEM-1001L and Scanning Electron Microscope (SEM) Zeiss EVO MA10 equiped with an Oxford X-Act Energy Dispersive Spectrometer (EDS). A Vibrating Sample Magnetometer (VSM) PPMS Dyna Cool 9T was used to characterize the magnetic properties of as-prepared nanoparticles. The nanoparticles stability was study through Thermogravimetric Analysis (TGA) on PerkinElmer Thermogravimetric Analyzer, model TGA4000. The FTIR spectroscopy technique was used for identify and characterize the organic coating on Fourier Transform Infrared Spectrometer Jasco FT/IR-4600.
Antibacterial activity
Staphylococcus aureus (ATCC 25923) was provided by the Chilean Institute of Public Health (ISP). The strain was cultured overnight in trypticase soy broth (TSB) at 37 °C. Afterwards, the culture was diluted 100-fold with TSB and incubated at 200 rpm until obtaining an optical density (OD) 600 nm of mid-log phase. Cells were then washed and recovered by centrifugation at 1000 × g for 2 min. The resultant pellet was resuspended in 1% TSB in 10 mM phosphate buffer saline (PBS) and adjusted to 1 × 107 CFU mL−1 (between 0.1 to 0.7 corresponding to 1 × 107 CFU mL−1). Then was exposed to 0.5, 1, and 2 mM Co0@C-NPs concentrations for 1 h at optimum growth temperature. Following exposure, the bacteria culture was diluted ten-fold with the same buffer and incubated for 18 h in fresh TSB media. Independent experiments were repeated a minimum of three times.25
Scanning Electron Microscopy (SEM) of Gram positive bacteria
Aliquots 100 μL of mid-log phase S. aureus was harvested by centrifugation at 3000 × g for 5 min and resuspended in HEPES buffer 10 mM with 1%TSB. The cell suspension was incubated at 37 °C for 30 minutes with the Co0@C-NPs at 0.5 and 2 mM. After incubation, the cells were centrifuged and washed 3 times at 4000 × g for 5 min with PBS and resuspended in the same buffer. The bacterial pellet was deposited on a glass coverslip and Gram stained. Afterwards it was sputter coated with approximately 30 nm thickness of gold. Finally, the microscopic examination was performed in a Carl Zeiss EVO MA 10 scanning electron microscope at 20 kV.
Statistical analysis
All values obtain throught antimicrobial assay were expressed as mean ± S.E. Statistical differences between means were calculated with GraphPad Prism 6 software by using the Student's t test correcting for differences in sample variance.
Results and discussion
Characterization of Co0@C-NPs
X-ray powder diffraction. The chemical nature and crystallinity of the synthesized product were identified by X-ray powder diffraction. Fig. 1 show the diffraction patterns obtained for the Co0@C-NPs. The nanoparticles display three peaks of different intensity at 2q values of 44.2; 51.5 and 75.8° which correspond to the Miller Indices (111), (200), (220) respectively, and are characteristic of face centered cubic Co0 (JCPDS file no. 15-0806, cubic system, spatial group: Fm3m, a = 3.5447 Å). Only one metallic phase is observed.
 |
| Fig. 1 Powder diffraction pattern of Co0@C-NPs. | |
TEM and SEM microscopy analysis of Co0@C-NPs
Morphological and structural details of Co0@C-NPs were investigated by scanning and transmission electron microscopy. The observation of TEM images of these nanoparticles revealed that all the particles have spherical shape with an average diameter less than 100 nm. Besides, the TEM micrographic (Fig. 2A and B) permits the observation of a thin organic coating layer. This layer was supposedly associated with the amino acid used as a starting material in a previous study, which was also responsible for the stability of the nickel nanoparticles and prevented their oxidation.23,24 Fig. 2C and D show the SEM images for Co0@C-NPs at two different magnifications. It is observed that they are forming homogeneous metallic agglomerates with spherical shapes and sizes around 2 μm in diameter.
 |
| Fig. 2 Morphology of Co0@C-NPs. (A) and (B) TEM images of Co@C0-NPs. The organic layer is show in red arrows. (C) and (D) shows the SEM images for Co@C0-NPs at two different magnifications. | |
In order to investigate the surface composition of the organic layer attached to the synthesized Co0@C-NPs organic layer SEM/EDS and FTIR analysis were carried out. Through spectra obtained by SEM/EDS we observed peaks corresponding to carbon, oxygen and cobalt elements (Fig. 3).
 |
| Fig. 3 Characterization of Co0@C-NPs organic layer. SEM/EDS for Co0@C-NPs is shown in (A). This image was used to Co0@C-NPs surface composition studies where was obtained two spectra: 6 (B) and 7 (C). Both showed intensity peak for carbon, oxygen and cobalt elements. | |
The presence of organic material on the Co0@C-NPs surface was confirmed by FTIR spectrum (Fig. 4), where we can see characteristic bands for the functional groups of amino acid. The peak noticed at 3428 cm−1 could be attributed to (–NH stretching)26 1632 cm−1 and 1456 cm−1 vibration band could be are ascribed to COO- asymmetric (uas) and COO– symmetric (us) stretching respectively.27–29
 |
| Fig. 4 Characterization of Co0@C-NPs organic layer. FTIR spectra of Co0@C-NPs. | |
Thermogravimetric analysis
In order to investigate the thermal stability, Co0@C-NPs were subjected to thermogravimetric analysis (TGA) from 30 to 800 °C under air and nitrogen condition at the heating rate of 10 °C min−1. TGA curves show that Co0@C-NPs are stable until 400 °C (Fig. 5). Over this temperature was observed a significant mass increase of 30.32% and 26.00% in air and nitrogen atmosphere, respectively. This mass increase could be attributed to the generation of cobalt oxides due to the decomposition of organic material present in the Co0@C-NPs surface, which is corroborated with the results obtained through TEM, SEM/EDS images and FTIR spectrum.
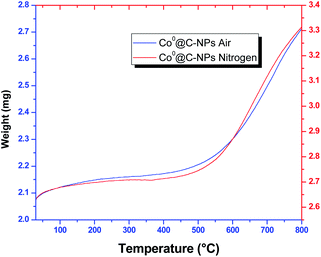 |
| Fig. 5 TGA curve of Co0@C-NPs. | |
Magnetic characterization
The magnetic behaviour for Co0@C-NPs was investigated by vibrating sample magnetometer at 300 K under an external magnetic field sweeping from +20 to −20 kOe. The magnetic hysteresis loop for Co0 nanoparticles is shown in Fig. 6.
 |
| Fig. 6 Magnetization curve of Co0@C-NPs measured at 300 K. | |
The magnetic parameters values obtained from Co0@C-NPs such as saturation magnetization (Ms), coercivity (Hc), remnant magnetization (Mr) and ratio of the remnant to saturation magnetization (Mr/Ms) are 148.6 (emu g−1), 56 (Oe), 3.95 (emu g−1) and 0.027 respectively. The results demonstrate that the magnetization curve shows typical superparamagnetic behavior with very low values in both remnant magnetization and coercivity. The value of Ms for Co0@C-NPs is lower than multidomain bulk particles of Co (168 emu g−1).30 This is due to the size in which the nanoparticles are obtained. The coercive force (Hc = 56 Oe) and residual magnetization (Mr = 3.95 emu g−1) are small, which indicates the Co0@C-NPs are close to superparamagnetic. Furthermore, the ratio of Mr/Ms = 0.027, is very small, which is typical for superparamagnetic behaviour.31 Superparamagnetic nanoparticles exhibit a monodomain structure with a high magnetic susceptibility exclusively under an external magnetic field. This unique property combining with a biocompatible capability is very promising because allow applying this kind of nanostructure in several biomedical application like theragnostic, hyperthermia tumor therapy, drug delivery, and magnetic resonance imaging.32–34
Antimicrobial effect of Co0@C-NPs in bacteria
Since it has been shown that bacteria are becoming resistant to antimicrobial agents, it is necessary to find novel materials with antimicrobial activity. Nanoparticles are being used with this purpose.35,36 The Co based nanoparticles has been studies against Staphylococcus aureus, these include cobalt ferrite nanoparticles37–40 and cobalt oxide nanoparticles,41,42 but there are a few investigation that used only metallic cobalt nanoparticles without conjugated at other nanoparticles or nanomaterials. For this reason, in this study, we evaluated the antimicrobial properties of Co0@C-NPs synthesized by a solvothermal technique against Gram-positive bacteria (S. aureus) (Fig. 7). At 0.5 and 1 mM of Co0@C-NPs, no significant inhibition was observed in the growth of S. aureus, but at 2 mM, it was observed that Co0@C-NPs have major antimicrobial activity against this strain (Fig. 7), inhibiting the amount of bacteria from 0 up to 5.5 × 103 CFU mL−1 after 1 h of exposition. In two investigations have been demostrated that Co0-NPs have antimicrobial activity. One of them have described that cobalt nanoparticles, in the range of 20-49 nm synthetized by adopting an eco method, showed activity against S. aureus above 25 mg mL−1,43 which is 200 to 400 times higher than what we used in this research. The second investigation, cobalt nanoparticles of 10 nm synthetized by chemical methods are capable of killing 1 × 102 CFU mL−1 of Escherichia coli after 3 h of exposition at 1.86 mM (0.11 mg mL−1), which is a lower inhibition than was obtained in this investigation.36
 |
| Fig. 7 Antimicrobial activity of Co0@C-NPs. Growth inhibition of S. aureus in the absence of Co0@C-NPs and in the presence of Co0@C-NPs concentrations of 0.5, 1 and 2 mM after 1 hour of exposure. The standard error was determined from the results of two experiments carried out in triplicate. | |
A SEM study was performed for the direct observation of cell morphological changes after Co0@C-NPs treatment at 0.5 and 2 mM. The untreated S. aureus cells were used as a control, exhibiting a smooth and intact surface (Fig. 8), but when we treated S. aureus with Co0@C-NPs at 0.5 mM, it was observed that NPs form an aggregate-like structure on the bacteria surface. It could be presumed that this is an interaction observed between clustering of NPs and the cell surface that occurs during co-incubation and is not reflective of damage induced to the bacteria membrane as previously described by Sousa et al.44 At 2 mM a similar aggregate structure was observed but with fewer NPs. Probably, since there is a higher concentration of Co0@C-NPs, it is easier for them to aggregate and precipitate at the time of centrifugation, leaving individual NPs or apparently loose NPs aggregates44 on the bacteria surface. Further research is necessary to demonstrate if Co0@C-NPs have effects on bacterial surfaces or on intracellular components of bacteria at different times.
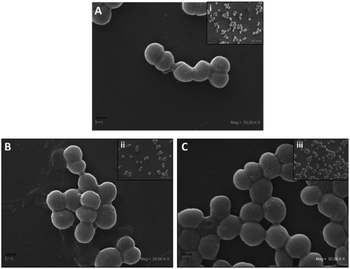 |
| Fig. 8 Scanning electron microscopy of S. aureus. SEM micrographs of Staphylococcus aureus (A) without Co0@C-NPs, (B) in the presence of Co0@C-NPs at 0.5 mM, and (C) in the presence of Co0@C-NPs at 2 mM. | |
Given that Co0@C-NPs have a thin organic coating layer associated to L-serine amino acid and DMF which is responsible for their stability and prevention of oxidation, the effect of metallic cobalt in bacterial growth-inhibition may be explained for the generation of oxidation products derived from L-serine amino acid in agreement to general reaction of oxidation for α-amino acids: R·CH(NH2)·CO2H + O Þ R·CHO + NH3 + CO2 described by Hough et al.45,46 and oxidation of DMF to a carboxylic acid as well as the reduction of Ni2+ (or Co2+) to Ni (or Co), which can be briefly formulated as follows: HCONMe2 + Ni2+(or Co2+) + H2O Þ Ni(or Co) + Me2NCOOH + 2H+ described by Zhang et al.,46 where functional groups of Me2NCOOH could be able to form hydrogen bonds with the bacterial cell wall.
On the other hand, it has been described that different types of nanoparticles generate reactive oxygen species (ROS), that could damage bacterial DNA, cell membranes (lipid peroxidation) and cause protein dysfunction.15,35,47,48 In this study, cobalt ions could be released from metallic cobalt nanoclusters which could interact with thiol groups on bacterial enzymes leading to cell death, as previously described by Alahmadi et al.47 Further experiments are essential before concluding about the antibacterial behavior of such oxidation products generated in the synthesis of Co0@C-NPs, which may lead to the release of cobalt ions or possibly both. Experiments will be important for determining the potential use of these nanoparticles as a new antimicrobial agent to combat bacterial infections.
Conclusions
In conclusion, we report the structural, morphological and magnetic properties of cobalt nanoparticles stabilized with an organic layer (Co0@C-NPs) and its potential antimicrobial activity. The solvothermal technique permits us to obtain stable and spherical fcc-Co0 with an organic layer that avoids the oxidation process until 400 °C. The elemental analysis confirm the presence of carbon and oxygen elements associated to organic layer and through spectroscopy was identified functional groups of amino acid like –NH and COO–.
Furthermore, this study reveals promissory results given that Co0@C-NPs shows antimicrobial activity against S. aureus at 2 mM, being 55 times more effective in less time than the previous studies. SEM analysis shows that Co0@C-NPs form an aggregate-like structure probably due interaction observed between clustering of NPs and the cell surface. Further research is necessary to establish the mechanism of action of the Co0@C-NPs.
The organic coating Co0@C-NPs and superparamagnetic property could be biocompatible with biological systems and in the future could be use in several biomedical application without additional processes required such as encapsulation, but previously must be evaluated its biosafety through in vitro biological assay.
Conflicts of interest
The authors declare that they have no competing interest.
Acknowledgements
This work was funded by project DIUA 151-2019 from the Dirección de Investigación of Universidad Autónoma de Chile and FONDECYT project No. 11170244 and No. 11170544. Financiamiento Basal para Centros Científicos y Tecnológicos de Excelencia AFB180001 and CONICYT-FONDEQUIP/PPMS/EQM130086-UNAB.
Notes and references
- A. H. Lu, E. L. Salabas and F. Schüth, Magnetic nanoparticles: Synthesis, protection, functionalization, and application, Angew. Chem. Int. Ed., 2007, 46(8), 1222–1244 CrossRef CAS.
- A. Gual, C. Godard, S. Castillón, D. Curulla-Ferré and C. Claver, Colloidal Ru, Co and Fe-nanoparticles. Synthesis and application as nanocatalysts in the Fischer-Tropsch process, Catal. Today, 2012, 183(1), 154–171 CrossRef CAS.
- W. Baaziz, S. Begin-Colin, B. P. Pichon, I. Florea, O. Ersen and S. Zafeiratos, et al., High-Density Monodispersed Cobalt Nanoparticles Filled into Multiwalled Carbon Nanotubes, Chem. Mater., 2012, 24(9), 1549–1551 CrossRef CAS.
- C. Dey, K. Baishya, A. Ghosh, M. M. Goswami and A. Ghosh, J. Magn. Magn. Mater., 2016, 427, 168–174 CrossRef.
- A. Kotoulas and C. Sarafidis, Carbon-encapsulated cobalt nanoparticles: synthesis , properties , and magnetic particle hyperthermia efficiency, J. Nanopart. Res., 2017, 19, 399 CrossRef.
- Z. Abdullaeva, E. Omurzak, C. Iwamoto and T. Mashimo, Onion-like carbon-encapsulated Co , Ni , and Fe magnetic nanoparticles with low cytotoxicity synthesized by a pulsed plasma in a liquid, Carbon, 2011, 50(5), 1776–1785 CrossRef.
- V. Dogra, G. Kaur, S. Jindal, R. Kumar, S. Kumar and N. Kumar, Science of the Total Environment Bactericidal effects of metallosurfactants based cobalt oxide/hydroxide nanoparticles against Staphylococcus aureus, Sci. Total Environ., 2019, 681, 350–364 CrossRef CAS.
- J. Z. Hilt, Magnetic nanoparticles in biomedicine: synthesis , functionalization and applications Review, Nanomedicine, 2010, 5, 1401–1414 CrossRef.
- D. K. Henderson, Managing Methicillin-Resistant Staphylococci: A Paradigm for Preventing Nosocomial Transmission of Resistant Organisms, Am. J. Med, 2006, 119, 45–52 CrossRef.
- S. M. Ansari, R. D. Bhor, K. R. Pai, D. Sen, S. Mazumder and K. Ghosh, et al., Cobalt Nanoparticles for Biomedical Applications: Facile Synthesis , Physiochemical Characterization , Cytotoxicity Behavior and Biocompatibility, Appl. Surf. Sci., 2017, 414, 171–187 CrossRef CAS.
- M. Alagiri, C. Muthamizhchelvan and S. B. A. Hamid, Synthesis of superparamagnetic cobalt nanoparticles through solvothermal process, J. Mater. Sci. Mater. Electron., 2013, 24(11), 4157–4160 CrossRef CAS.
- S. Khan, A. A. Ansari, A. Arif, K. Rehan, O. Al and O. Wael, et al., In vitro evaluation of anticancer and antibacterial activities of cobalt oxide nanoparticles, J. Biol. Inorg Chem., 2015, 20(8), 1319–1326 CrossRef CAS.
- A. Anwar, A. Numan, R. Siddiqui, M. Khalid and N. A. Khan, Cobalt nanoparticles as novel nanotherapeutics against Acanthamoeba castellanii, Parasites Vectors, 2019, 1–10 Search PubMed.
- J. C. Castrill and L. P. Orozco, spp. como parásitos patógenos y oportunistas, Rev. Chil. infectol., 2013, 30, 147–155 CrossRef.
- N. S. Alahmadi, J. W. Betts, F. Cheng, M. G. Francesconi and S. M. Kelly, Cellulose magnetic nanocomposites, RSC Adv., 2017, 7, 20020–20026 RSC.
- K. Elayakumar, A. Dinesh, A. Manikandan, M. Palanivelu, G. Kavitha, S. Prakash, R. Thilak Kumar, S. Jaganathan and A. Baykal, Structural, morphological, enhanced magnetic properties and antibacterial bio-medical activity of rare earth element (REE) Cerium (Ce3+) doped CoFe2O4 nanoparticles, J. Magn. Magn. Mater., 2018, 476, 157–165 CrossRef.
- R. Wang, B. Zhang, Z. Liang, Y. He, Z. Wang and X. Ma, et al., Environmental Insights into rapid photodynamic inactivation mechanism of Staphylococcus aureus via rational design of multifunctional nitrogen-rich carbon-coated bismuth/cobalt nanoparticles, Appl. Catal., B, 2019, 241, 167–177 CrossRef CAS.
- Z. E. Eldine, W. M. A. Rouby and El. PT CR., Mater. Sci. Eng. C, 2018, 91, 361–371 CrossRef.
- M. Hosseini-zori, Journal of Photochemistry & Photobiology , B: Biology Co-doped TiO2 nanostructures as a strong antibacterial agent and self- cleaning cover: Synthesis , characterization and investigation of photocatalytic activity under UV irradiation, J. Photochem. Photobiol. B Biol., 2018, 178, 512–520 CrossRef CAS.
- I. C. Communication and A. D. Khalaji, Cobalt oxide nanoparticles by solid-state decomposition: Synthesis and characterization, Chem. Commun., 2019, 7, 113–116 Search PubMed.
- J. Yang, C. Lin, Z. Wang and J. Lin, In(OH)3 and In2O3 nanorod bundles and spheres: Microemulsion-mediated hydrothermal synthesis and luminescence properties, Inorg. Chem., 2006, 45(22), 8973–8979 CrossRef CAS.
- Y. Su, X. OuYang and J. Tang, Spectra study and size control of cobalt nanoparticles passivated with oleic acid and triphenylphosphine, Appl. Surf. Sci., 2010, 256(8), 2353–2356 CrossRef CAS.
- V. Paredes-Garcia, C. Cruz, N. Toledo, J. Denardin, D. Venegas-Yazigi, C. Castillo, E. Spodine and Z. Luo, Effect of the different synthetic parameters on the morphology and magnetic properties of nickel nanoparticles, New J. Chem., 2014, 38(2), 837–844 RSC.
- C. Castillo, K. Seguin, P. Aguirre, A. D. C. Viegas and E. Spodine, Nickel nanocomposites: magnetic and catalytic, RSC Adv., 2015, 5, 63073–63079 RSC.
- M. Azkargorta, J. Soria, C. Ojeda, F. Guzman, A. Acera, I. Iloro, T. Suárez and F. Elortza, Human basal tear peptidome characterization by CID , HCD and ETD followed by in silico and in vitro analyses for antimicrobial peptide identification, J. Proteome Res., 2015, 14(6), 2649–2658 CrossRef CAS.
- A. B. Tamboli and N. N. Maldar, Synthesis and characterization of processable aromatic poly (ether ether ketone amide) s modified by phenoxy, Polym Bull, 2020, 0123456789 Search PubMed.
- W. Lee, C. Loo, A. V. Zavgorodniy and R. Rohanizadeh, High protein adsorptive capacity of amino acid-functionalized hydroxyapatite, J. Biomed. Mater. Res., 2012, 1–11 CAS.
- M. Nageshwari, C. R. T. Kumari, G. Vinitha, S. Muthu and M. L. Caroline, Phys. B Condens. Matter, 2018, 541, 32–42 CrossRef CAS.
- K. Tõnsuaadu, M. Gruselle, F. Kriisa, A. Trikkel, P. Gredin and D. Villemin, Dependence of the interaction mechanisms between l - serine and O - phospho - l - serine with calcium hydroxyapatite and copper modified hydroxyapatite in relation with the acidity of aqueous medium, J. Biol. Inorg Chem., 2018, 0123456789 Search PubMed.
- G. Zhu, X. Wei, C. Xia and Y. Ye, Solution route to single crystalline dendritic cobalt nanostructures coated with carbon shells, Carbon, 2007, 45, 1160–1166 CrossRef CAS.
- M. Galaburda, V. Bogatyrov, O. Oranska and V. Gun, Synthesis and characterization of carbon composites containing Fe , Co , Ni nanoparticles, J. Therm. Anal. Calorim., 2015, 122, 553–561 CrossRef CAS.
- S. Talluri and R. R. Malla, Superparamagnetic Iron Oxide Nanoparticles ( SPIONs ) for Diagnosis and Treatment of Breast , Ovarian and Cervical Cancers, Curr. Drug Metab., 2019, 20, 12 Search PubMed.
- E. J. Guggenheim, J. Z. Rappoport and I. Lynch, Mechanisms for cellular uptake of nanosized clinical MRI contrast agents, Nanotoxicology, 2020, 1–29 Search PubMed.
- Y. Xiao, Superparamagnetic nanoparticles for biomedical applications, J. Mater. Chem., 2019, 8, 354–367 RSC.
- Y. N. Slavin, J. Asnis, U. O. Häfeli and H. Bach, Metal nanoparticles: understanding the mechanisms behind antibacterial activity, J. Nanobiotechnol., 2017, 1–20 Search PubMed.
- S. Hatamie, M. Nouri, S. K. Karandikar, A. Kulkarni, S. D. Dhole, D. M. Phase and S. Kale, Complexes of cobalt nanoparticles and polyfunctional curcumin as antimicrobial agents, Mater. Sci. Eng. C, 2012, 32(2), 92–97 CrossRef CAS.
- N. Sanpo, C. C. Berndt, C. Wen and J. Wang, Transition metal-substituted cobalt ferrite nanoparticles for biomedical applications, Acta Biomater., 2013, 9(3), 5830–5837 CrossRef CAS.
- R. Žalnėravičius, A. Paškevičius, K. Mažeika and A. Jagminas, Appl. Surf. Sci., 2017, 435, 141–148 CrossRef.
- V. S. Kirankumar, Photocatalytic and antibacterial activity of bismuth and copper co- doped cobalt ferrite nanoparticles, J. Mater. Sci. Mater. Electron., 2018, 29, 8738–8746 CrossRef CAS.
- S. Rehman, M. A. Ansari, M. A. Alzohairy, M. N. Alomary, B. R. Jermy, R. Shahzad, N. Tashkandi and Z. H. Alsalem, Synthesized Neodymium-Substituted Cobalt, Processes, 2019, 7, 714 CrossRef CAS.
- A. Talha, M. Ovais, I. Ullah, M. Ali, Z. K. Shinwari and M. Maaza, Physical properties , biological applications and biocompatibility studies on biosynthesized single phase cobalt oxide (Co3O4) nanoparticles via Sageretia thea ( Osbeck .), Arabian J. Chem., 2020, 13(1), 606–619 CrossRef.
- V. Dogra, G. Kaur, S. Jindal, R. Kumar, S. Kumar, N. Kumar and N. K. Singhal, Bactericidal effects of metallosurfactants based cobalt oxide/hydroxide nanoparticles against Staphylococcus aureus, Sci. Total Environ., 2019, 681, 350–364 CrossRef CAS.
- O. Igwe and E. S. Ekebo, Biofabrication of cobalt nanoparticles using leaf extract of Chromonaela odorata and their potential antibacterial application, Res. J. Chem. Sci., 2018, 8, 1 11–17 Search PubMed.
- C. Sousa, D. Sequeira, Y. V. Kolen, I. M. Pinto and D. Y. Petrovykh, Analytical Protocols for Separation and Electron Microscopy of Nanoparticles Interacting with Bacterial Cells Analytical Protocols for Separation and Electron Microscopy of Nanoparticles Interacting with Bacterial Cells, Anal. Chem., 2015, 87, 4641–4648 CrossRef CAS.
- L. Hough and V. Slyke, The Periodate Oxidation of Amino Acids with Reference to Studies on Glycoproteins, Biochem, 1965, 17–24 Search PubMed.
- Z. Zhang, X. Chen, X. Zhang and C. Shi, Synthesis and magnetic properties of nickel and cobalt nanoparticles obtained in DMF solution, Solid State Commun., 2006, 139, 403–405 CrossRef CAS.
- A. A. Dayem, M. K. Hossain, S. B. Lee, K. Kim, S. K. Saha, G. Yang, H. Choi and S. Cho, The Role of Reactive Oxygen Species ( ROS ) in the Biological Activities of Metallic Nanoparticles, Int. J. Mol. Sci., 2017, 18, 120 CrossRef.
- L. Wang and C. Hu, The antimicrobial activity of nanoparticles: present situation and prospects for the future, Int. J. Nanomed., 2017, 1227–1249 CrossRef.
|
This journal is © The Royal Society of Chemistry 2020 |
Click here to see how this site uses Cookies. View our privacy policy here.