DOI:
10.1039/D0RA08261A
(Paper)
RSC Adv., 2020,
10, 39687-39692
Synthesis of 5-hydroxymethylfurfural from monosaccharides catalyzed by superacid VNU-11–SO4 in 1-ethyl-3-methylimidazolium chloride ionic liquid†
Received
27th September 2020
, Accepted 19th October 2020
First published on 30th October 2020
Abstract
Superacid VNU-11–SO4, a modified metal–organic framework by post-synthetic treatment with a sulfuric acid solution, has been considered as a promising heterogeneous catalyst in the isomerization of glucose to fructose and further dehydration to form 5-hydroxymethylfurfural (HMF) due to its possession of both Lewis and Brønsted acid sites. In this work, we focused on using VNU-11–SO4 for the optimization of the conversion of fructose and glucose into HMF using an ionic liquid as a green solvent. The highest yields of HMF from glucose and fructose could be obtained in 28% (140 °C, 8 h) and 86% (110 °C, 3 h), respectively, with the use of VNU-11–SO4 catalyst in 1-ethyl-3-methylimidazolium chloride ionic liquid. Recycling examination of the catalyst showed only a slight decrease in the HMF yield, implying its potential industrial application in biomass transformation.
1. Introduction
5-Hydroxymethylfurfural is a useful furan ring that can produce an important intermediate to biofuels and commodity chemicals.1–3 In recent years, the preparation of HMF from carbohydrates has been extensively studied. Generally, HMF is mainly produced via dehydration reactions of monosaccharides using homogeneous (e.g., H2SO4, HCl, AlCl3, and CrCl3) or heterogeneous catalysts (e.g., zirconium oxide, zeolites, metal–organic frameworks) in a biphasic system.3–10 Although the homogeneous acidic catalysts are efficient in the process,11 some methods suffer from intrinsic drawbacks, e.g., an expensive catalyst with high loading, long reaction time, low yield, difficulty in the recovery of catalyst, and toxic reactants. The heterogeneous catalysts prove prominent advantages of stability and reusability promising as potential alternatives.12–15 Besides, water, dimethylsulfoxide (DMSO), N,N-dimethylformamide (DMF), N,N-dimethylacetamide (DMA), N-methyl pyrrolidone (NMP), acetonitrile, ionic liquids (ILs), deep eutectic (DES) and biphasic systems have proven as suitable solvents for the dissolution of sugar.16,17 Among these, ILs and DES are becoming increasingly important due to the unique properties related to design flexible structure and reusability.16,18–20
Metal–organic frameworks (MOFs) are attracted considerable interest in porous crystalline materials composed of organic ligands and inorganic metal clusters. These materials have been received with widespread attention for several fields, including gas storage and separation,21,22 catalysis,23,24 sensing,25,26 drug delivery,27,28 proton, and ion conduction.29,30 With the prominent features such as low density, the large pore sizes, and thermal stability, MOFs have recently appeared as ability heterogeneous catalytic for organic transformations.31,32 In HMF production, the materials had multi-utility catalytic simultaneously shown better activity and selectivity for the isomerization/dehydration of glucose, in particular, Lewis acidity, Brønsted acidity, and uniform structured nanoscale cavities in MOF could be readily introduced for biomass conversion.33–37 Sulfated MOF-808 and VNU-11 were known as the first superacid MOF on Zr- and Hf-MOF by anchoring sulfate groups onto the metal clusters of secondary building units. The coexisting Brønsted and Lewis acidities in superacid MOF were also capable of catalyzing reaction, including Friedel–Crafts acylation, esterification, isomerization, and heterocyclization.38,39 The reaction proceeded smoothly and afforded the expected product in high yield in the presence of superacid MOF and ionic liquid under mild condition.
Herein, we demonstrate a strategy using superacid VNU-11–SO4 for the preparation of HMF from monosaccharides in ILs (Scheme 1). The VNU-11–SO4 was proved to be an efficient catalyst for dehydration of glucose and fructose into HMF in 1-ethyl-3-methylimidazolium chloride ([Emim]Cl). To the best of our knowledge, this is the first report of utilizing VNU-11–SO4 in the conversion of monosaccharides to HMF in ionic liquids.
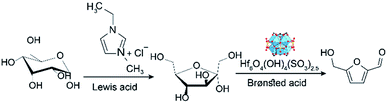 |
| Scheme 1 The glucose conversion into HMF using superacid MOF in ionic liquids. | |
2. Experimental
2.1. Materials and instrumentations
All chemicals were purchased commercial suppliers and used without further purification. HPLC analyses were recorded on Agilent Technologies 1260 Infinity high-performance liquid chromatography InertSustain C18 (4.6 × 150 mm) column, the size of particles is 5 μm, equipped with a UV photodiode array detector. For more details about chemicals and instruments, please see in ESI.†
2.2. Preparation of VNU-11–SO4
VNU-11 and VNU-11–SO4 were synthesized from reported literature.34 A mixture of HfOCl2·8H2O (7.5 mmol, 3.10 g) and the tricarboxylic acid H3BTC (2.5 mmol, 537 mg) in 320 mL solvent mixture of DMF and formic acid (v/v = 1
:
1) were added in 500 mL capped bottle and heated in an oven at 120 °C for 3 days under static conditions. After cooling the bottle to room temperature, the precipitates were collected by centrifugation. The products were washed with DMF, deionized water, and anhydrous acetone separately 3 times for three days. Then, the material was dried under reduced pressure at room temperature for 24 h and at 150 °C for 24 h to collect activated VNU-11.
To obtain VNU-11–SO4, activated VNU-11 (200 mg, 0.109 mmol) was treated with 20 mL of H2SO4 0.1 M and for 24 h. After 24 h, the solid was centrifuged and washed with 60 mL deionized water in 3 times per day for three days. Then, the sample was washed quickly, exchanged with 100 mL anhydrous acetone and immersed in 20 mL anhydrous chloroform for 3 days (3 times per day). Finally, VNU-11–SO4 was dried under reduced pressure at room temperature for 24 h and at 150 °C for 24 h to collect activated VNU-11–SO4.
2.3. Preparation of DESs
The deep eutectic solvents (DESs), a new type of ionic liquids, including choline chloride/phenol (DES 1), choline chloride/ethylene glycol (DES 2) were prepared through the procedure reported in our previous literature.13,40 DESs were synthesized easily by the formation of hydrogen bonding between phenol or ethylene glycol with choline chloride (please see in Section 3, ESI†).
2.4. Catalytic study
In a typical experiment, glucose or fructose (1 mmol) was dissolved in [Emim]Cl (6 mmol), and then the VNU-11–SO4 catalyst (20 mg) was added. The reaction mixture was heated in a magnetic stirrer with an oil bath for 24 h. Samples were taken from the reaction mixture at a period of time for HPLC analysis to study the effect of catalyst, reaction times, and reaction temperatures on the yield of the product. The isolation of HMF from [Emim]Cl was performed by liquid–liquid extraction with a mixture of ethyl acetate/diethyl ether. The structure of HMF was characterized by 1H NMR and MS.
3. Results and discussion
3.1. Catalysts characterization of VNU-11–SO4
The VNU-11 and VNU-11–SO4 were synthesized with a modification of the previous method and the detailed procedure reported in ESI.†38 The Hf-MOFs were prepared via the reaction between hafnium salt and H3BTC in N,N-dimethylformamide as a solvent, and formic acid as a modulator. After the removal of the guest molecules by evacuation at 150 °C for 24 h, the structure of the material was determined by PXRD patterns and N2 sorption isotherms to confirm the successful activation and phase purity of the materials (Fig. 1a and d). TGA measurements were carried out for the VNU-11 pristine and VNU-11–SO4 sample under airflow (Fig. 1b).
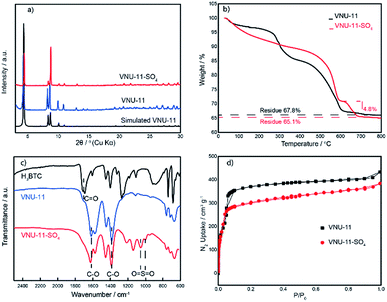 |
| Fig. 1 (a) PXRD patterns, (b) TGA curves, (c) FT-IR and (d) N2 isotherms of VNU-11 (blue) and VNU-11–SO4 (red) samples. The filled and opened symbols represent the adsorption and desorption processes, respectively. | |
The TGA curve of VNU-11 and VNU-11–SO4 showed two distinct weight loss steps, corresponding to the evaporation of organic solvents and water adsorbed on the surface (∼3% and 10% for VNU-11 and VNU-11–SO4, respectively) and to the decomposition of the framework (29.2% and 24.9% at 250–600 °C for VNU-11 and VNU-11–SO4, respectively). The temperature range of 600–800 °C of the VNU-11–SO4 sample was shown the decomposition of the anhydrous compound Hf(SO4)2 to give the end product HfO2 of decomposition.41 In the range temperature 600–700 °C, the mass loss was 4.8%, which corresponded to a release of SO2. This value was consistent with the percentage of theoretical mass SO2 lost from VNU-11–(SO4)2.5 (4.2%).
Fig. 1c shows the FT-IR spectra of the H3BTC, VNU-11, and VNU-11–SO4 samples. The characteristic peaks in the range of 1610–552 cm−1 in the VNU-11 spectrum were ascribed to C
O vibrations (1610 cm−1), C–O stretching (1400 cm−1), and Zr–O modes (760 cm−1). The presence of sulfonic groups in the VNU-11–SO4 structure resulted in identical signals at approximately 1000 and 1050 cm−1. The acidities of VNU-11–SO4 were characterized by using the Hammett indicator test. The color change observed of VNU-11–SO4 in 2,4-dinitrofluorobenzene as with a value of Ho ≤ −14.5 indicated a higher acidity than the original VNU-11 as well as sulfuric acid (Ho = −12).
3.2. Catalytic study
3.2.1. Optimization of solvent and catalyst loading. As reported in the literature, fructose or glucose is often used in the synthesis of HMF. Glucose molecules need to undergo the isomerization and finish with the dehydration to form HMF. In addition, organic solvents such as DMSO, IL 1-butyl-3-methyl-imidazolium chloride [Bmim]Cl, IL 1-ethyl-3-methyl-imidazolium chloride [Emim]Cl, were effective solvents for this reaction. To evaluate the role of catalytic active sites in each conversion, the reactions were investigated by heating fructose or glucose at reflux with the screening solvents and amount of VNU-11–SO4 (Table 1).
Table 1 Optimization of solvent and catalytic loading in the synthesis of 5-HMFa
Entry |
Monosaccharides |
Solvents |
VNU-11–SO4 (mg) |
HMF yield (%) |
Reaction condition: 1 mmol of fructose or glucose, 6 mmol solvent. At 80 °C for 24 h. At 140 °C for 3 h. Deep eutectic solvent between choline chloride and phenol was prepared at a 1 : 4 molar ratio. Deep eutectic solvent between choline chloride and ethylene glycol was prepared at a 1 : 2 molar ratio. |
1 |
Fructoseb |
[Emim]Cl |
0 |
0 |
2 |
[Emim]Cl |
10 |
51.0 |
3 |
[Emim]Cl |
20 |
86.5 |
4 |
[Emim]Cl |
30 |
90.5 |
5 |
DMSO |
30 |
18.5 |
6 |
Glucosec |
[Emim]Cl |
0 |
0 |
7 |
[Emim]Cl |
10 |
14.5 |
8 |
[Emim]Cl |
20 |
14.8 |
9 |
[Emim]Cl |
25 |
23.7 |
10 |
[Emim]Cl |
30 |
28.5 |
11 |
No solvent |
10 |
0 |
12 |
DMSO |
10 |
7.6 |
13 |
DES 1d |
10 |
5.9 |
14 |
DES 2e |
10 |
3.5 |
The catalytic activity of the VNU-11–SO4 catalyst was investigated for dehydration of fructose under screening the amount of catalyst from 0–30 mg in [Emim]Cl and DMSO. A high HMF yield of 90% could be achieved under 1 mmol fructose, 6 mmol [Emim]Cl as solvent at 80 °C for 24 h when using 0.025 mmol (30 mg) of VNU-11–SO4 (Table 1, entry 4), but the conversion of glucose was not more than 30% at 140 °C for 3 h (Table 1, entry 10). As seen in Table 1, the HMF was not detected in the absence of the catalyst (Table 1, entry 1). This can be seen that the strong acidity of the SO3H group in superacid VNU-11–SO4 could promote for dehydration of fructose to achieve the high HMF yield and the catalytic system between [Emim]Cl and Hf-clusters could further an isomerization of glucose to fructose.
Next, the influence solvents on the HMF production were tested, and the results were shown in Table 1. The investigation indicated the highest HMF yield was obtained in [Emim]Cl (Table 1, entries 4 and 7), which was higher than the results achieved in DMSO (Table 1, entries 5 and 12). Deep eutectic solvents, a new type of ionic liquids, were also tested as the solvents in the present method, but the low yields of HMF were observed by using DES 1 and DES 2 (Table 1, entries 13 and 14). The reaction did not proceed in the absence of a solvent (Table 1, entry 11). The high yield of HMF in [Emim]Cl could be explained by the high concentration of chloride anion in the reaction mixture to assist with the isomerization of glucose to fructose of Lewis acid sites on VNU-11–SO4.42 Therefore, [Emim]Cl was used as the solvent for glucose conversion to HMF.
3.2.2. Optimization of temperature and reaction time. After finding the positive role of [Emim]Cl for these reactions, the optimization of the effects of temperature (80–140 °C) and reaction times (0.5–24 h) was screened in both cases of fructose and glucose. For the dehydration of fructose, when the temperature was raised from 80–140 °C, the yield of 5-HMF increased significantly and reached an 82% yield of 5-HMF at 140 °C. The results in Fig. 2 demonstrated that the highest yield of HMF was found at 80 °C and 24 h. At the higher temperature 110 °C, the yield of 83% HMF stead increased after 3 h, uphold magnitude in 2 h, and then slipped from 80% to 70% at the time reaction prolong from 6 h to 24 h. The same phenomenon almost occurred at 140 °C, but the magnitude of a stronger decline than at 110 °C. Reduce the HMF yield could be caused by the decomposition of 5-HMF at the high temperature. The synthesis of HMF from fructose was also carried out on a 10 mmol scale, and the HMF yield was almost the same as that on the 1 mmol scale (84.2% vs. 90.5%).
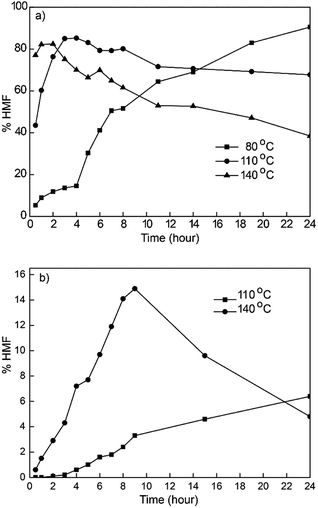 |
| Fig. 2 The effect of temperature and time reaction of fructose (a) and glucose (b) conversion to HMF. Reaction condition: 1 mmol monosaccharides, 6 mmol [Emim]Cl and 30 mg VNU-11–SO4. | |
In a previous study, high temperatures were essential for the dehydration of glucose. Fig. 2 shows the effect of time (110–140 °C) and temperature (0.5 –24 h) on the yield of HMF from the conversion of glucose in [Emim]Cl.
The best result of glucose conversion was obtained in 140 °C with 28.5% HMF yield for 8 h. A further increase in reaction time resulted in a noticeable decrease in HMF yield, presumably due to the decomposition of HMF (Fig. 2). At the lower temperature (110 °C), the yield of HMF increased slowly to 14% when extending the reaction time up to overnight, and the reaction did not proceed at 80 °C.
It is essential to compare our work with previous literature using MOFs as catalysts. The HMF yield from fructose was demonstrated in Table 2 (entries 1–7). The efficiency of the VNU-11–SO4 catalyst showed better activity than other MOFs, HMF yield of 86% could be achieved at 110 °C for only 3 h. In [Emim]Cl solvent, the activity of Brønsted acid MOF as PTA(3.0)/MIL-101 and VNU-11–SO4 were efficient for this reaction at the low temperature. The tolerable increase of HMF yields with longer reaction may be due to the sparing decomposition of HMF.
Table 2 The comparison of catalysts for the conversion of monosaccharides to HMF
Entry |
React |
Catalyst |
Solvent |
Time (h) |
Temperature (°C) |
HMF yield (%) |
Ref. |
1 |
Fructose |
VNU-11–SO4 |
[Emim]Cl |
24 |
80 |
90.5 |
This work |
2 |
VNU-11–SO4 |
DMSO |
24 |
80 |
18.5 |
This work |
3 |
PTA (3.0)/MIL-101 |
[Emim]Cl |
1 |
80 |
63 |
43 |
4 |
NUS-6 (Hf) |
DMSO |
3.5 |
100 |
98 |
34 |
5 |
VNU-11–SO4 |
[Emim]Cl |
3 |
110 |
86 |
This work |
6 |
VNU-11–SO4 |
DMSO |
3 |
110 |
31.2 |
This work |
7 |
MIL-101(Cr)–SO3H |
DMSO |
1 |
120 |
90 |
6 |
8 |
Glucose |
VNU-11–SO4 |
[Emim]Cl |
8 |
140 |
28.5 |
This work |
9 |
PO4/Nu-1000 |
Water/THF |
5 |
140 |
25 |
44 |
10 |
MIL-101(Cr)–SO3H |
GVC : water |
7 |
150 |
39.7 |
45 |
11 |
UiO-66 |
DMSO |
0.5 |
160 |
28 |
1 |
12 |
UiO-66 |
DMSO/water |
0.5 |
160 |
37 |
1 |
The summary for the progression of glucose conversion over a variety of catalysts and solvent was shown in Table 2, entries 8–12. At the high temperature of 160 °C, UiO-66 provided the desired product in a moderate yield (37%) of HMF in a mixture of DMSO and water. MIL-101(Cr)–SO3H gave a much higher yield of HMF for 7 h at 150 °C, compared with the yield using VNU-11–SO4 at 140 °C for 8 h.
3.2.3. Recyclability of VNU-11–SO4. The recyclability of the catalyst VNU-11–SO4 was investigated in the reaction for the synthesis of HMF. After completion of the reaction, the mixture was diluted with organic solvents and VNU-11–SO4 was collected via centrifugation, washed with dichloromethane and acetone, dried in reduced pressure. PXRD patterns of the fresh and reused catalysts after the three cycles indicated the structure of VNU-11–SO4 has remained mostly unchanged (Fig. 3). The S
O coordination in the structure after the reaction was confirmed by FT-IR (Fig. S2, ESI†) to deny the possibility of the leaching SO4 from the catalyst in the reaction mixture.
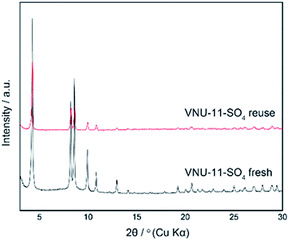 |
| Fig. 3 PXRD analysis of VNU-11–SO4 fresh (black) and after (red) the glucose conversion. | |
The leaching test was examined by ICP-OES and the hafnium content in the reaction mixture was less than 2 ppm, indicating negligibly slight leaching occurred from the catalyst. The recovered VNU-11–SO4 was reused three times with a slight decrease in the HMF yield. The recyclability of VNU-11–SO4 promises the potential application in industrial processes.
Based on the previous reports13,46 and our work, the isomerization of glucose and dehydration of fructose into HMF in the presence of [Emim]Cl solvent were demonstrated the sample evidence of VNU-11–SO4 as both of Brønsted and Lewis sites in the reaction mechanism (Scheme 2). In the first step, glucose was dissolved in [Emim]Cl solvent and isomerized to fructose by Lewis sites of VNU-11–SO4. Then, SO3H groups of superacid MOF acted as Brønsted acid for the dehydration process to obtain HMF.
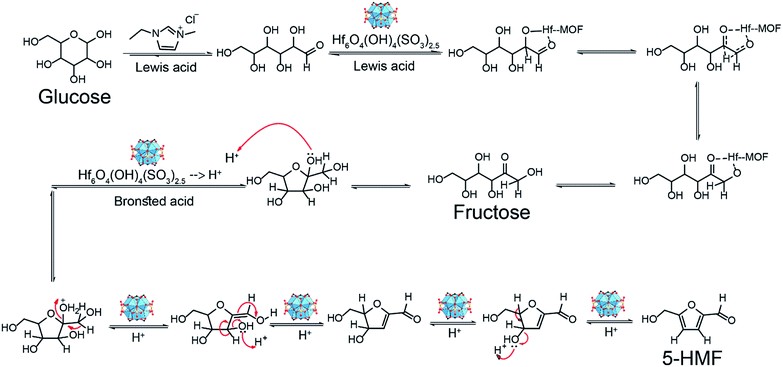 |
| Scheme 2 Dehydration reaction between glucose under VNU-11(Hf) catalyst and [Emim]Cl solvent. | |
4. Conclusion
The heterogeneously catalytic capability of superacid MOF was tested in dehydration of glucose and fructose to HMF in [Emim]Cl ionic liquid and DMSO. As a result, the VNU-11–SO4 showed a highly efficient conversion of glucose to fructose and further formation of HMF. Moreover, VNU-11–SO4 could be recycled three times without a significant decrease in reactivity. Compared to other MOFs, VNU-11–SO4 was a better catalyst and exhibited excellent performance in the 28% and 90% yield towards HMF from large amount of glucose and fructose, respectively, at the low temperature. This is the first example of HMF synthesis catalyzed by superacid MOF in ionic liquids. The method is a promising route for the large-scale production of HMF from biomass.
Conflicts of interest
There are no conflicts to declare.
Acknowledgements
This research was funded by Vietnam National University, Ho Chi Minh City (VNU-HCM) under grant number 562-2020-18-01. Linh Ho Thuy Nguyen acknowledges TX-2020-50-01 for VNU-11–SO4 catalytic synthesis and characterization.
Notes and references
- J. Gong, M. J. Katz and F. M. Kerton, RSC Adv., 2018, 8, 31618–31627 RSC.
- H. Xia, S. Xu, H. Hu, J. An and C. Li, RSC Adv., 2018, 8, 30875–30886 RSC.
- R.-J. van Putten, J. C. van der Waal, E. de Jong, C. B. Rasrendra, H. J. Heeres and J. G. de Vries, Chem. Rev., 2013, 113, 1499–1597 CrossRef CAS.
- F. Menegazzo, E. Ghedini and M. Signoretto, Molecules, 2018, 23, 2201 CrossRef.
- W. Guo, H. J. Heeres and J. Yue, Chem. Eng. J., 2020, 381, 122754 CrossRef CAS.
- J. Chen, K. Li, L. Chen, R. Liu, X. Huang and D. Ye, Green Chem., 2014, 16, 2490–2499 RSC.
- A. A. Rosatella, S. P. Simeonov, R. F. M. Frade and C. A. M. Afonso, Green Chem., 2011, 13, 754 RSC.
- Y. Yang, C.-w. Hu and M. M. Abu-Omar, Green Chem., 2012, 14, 509–513 RSC.
- J. Yang, K. De Oliveira Vigier, Y. Gu and F. Jérôme, ChemSusChem, 2015, 8, 269–274 CrossRef CAS.
- K. Zeng, Z. Huang, J. Yang and Y. Gu, Chin. J. Catal., 2015, 36, 1606–1613 CrossRef CAS.
- P. Körner, D. Jung and A. Kruse, Green Chem., 2018, 20, 2231–2241 RSC.
- L. T. Mika, E. Cséfalvay and Á. Németh, Chem. Rev., 2018, 118, 505–613 CrossRef CAS.
- P. H. Tran and P. V. Tran, Fuel, 2019, 246, 18–23 CrossRef CAS.
- J. Tuteja, S. Nishimura and K. Ebitani, Bull. Chem. Soc. Jpn., 2012, 85, 275–281 CrossRef CAS.
- W. Niu, D. Wang, G. Yang, J. Sun, M. Wu, Y. Yoneyama and N. Tsubaki, Bull. Chem. Soc. Jpn., 2014, 87, 1124–1129 CrossRef CAS.
- S. Körner, J. Albert and C. Held, Front. Chem., 2019, 7, 661 CrossRef.
- F. D'Anna, S. Marullo, P. Vitale, C. Rizzo, P. Lo Meo and R. Noto, Appl. Catal., A, 2014, 482, 287–293 CrossRef.
- J. Zhang, Y. Xiao, Y. Zhong, N. Du and X. Huang, ACS Sustainable Chem. Eng., 2016, 4, 3995–4002 CrossRef CAS.
- C. Wu, L.-H. Lu, A.-Z. Peng, G.-K. Jia, C. Peng, Z. Cao, Z. Tang, W.-M. He and X. Xu, Green Chem., 2018, 20, 3683–3688 RSC.
- L.-Y. Xie, S. Peng, L.-H. Lu, J. Hu, W.-H. Bao, F. Zeng, Z. Tang, X. Xu and W.-M. He, ACS Sustainable Chem. Eng., 2018, 6, 7989–7994 CrossRef CAS.
- H. Li, K. Wang, Y. Sun, C. T. Lollar, J. Li and H.-C. Zhou, Mater. Today, 2018, 21, 108–121 CrossRef CAS.
- O. V. Gutov, W. Bury, D. A. Gomez-Gualdron, V. Krungleviciute, D. Fairen-Jimenez, J. E. Mondloch, A. A. Sarjeant, S. S. Al-Juaid, R. Q. Snurr, J. T. Hupp, T. Yildirim and O. K. Farha, Chem.–Eur. J., 2014, 20, 12389–12393 CrossRef CAS.
- Y. Bai, Y. Dou, L.-H. Xie, W. Rutledge, J.-R. Li and H.-C. Zhou, Chem. Soc. Rev., 2016, 45, 2327–2367 RSC.
- L. H. T. Nguyen, T. T. T. Nguyen, P. H. Tran, Y. Kawazoe, H. M. Le and T. L. H. Doan, J. Catal., 2019, 374, 110–117 CrossRef CAS.
- P. Kumar, A. Deep and K.-H. Kim, TrAC, Trends Anal. Chem., 2015, 73, 39–53 CrossRef CAS.
- D.-K. Nguyen, J.-H. Lee, T. L.-H. Doan, T.-B. Nguyen, S. Park, S. S. Kim and B. T. Phan, Appl. Surf. Sci., 2020, 523, 146487 CrossRef CAS.
- P. Horcajada, T. Chalati, C. Serre, B. Gillet, C. Sebrie, T. Baati, J. F. Eubank, D. Heurtaux, P. Clayette, C. Kreuz, J.-S. Chang, Y. K. Hwang, V. Marsaud, P.-N. Bories, L. Cynober, S. Gil, G. Ferey, P. Couvreur and R. Gref, Nat. Mater., 2010, 9, 172–178 CrossRef CAS.
- L. He, Y. Liu, J. Lau, W. Fan, Q. Li, C. Zhang, P. Huang and X. Chen, Nanomedicine, 2019, 14, 1343–1365 CrossRef CAS.
- H. Furukawa, K. E. Cordova, M. O'Keeffe and O. M. Yaghi, Science, 2013, 341, 1230444 CrossRef.
- A. U. Czaja, N. Trukhan and U. Muller, Chem. Soc. Rev., 2009, 38, 1284–1293 RSC.
- V. I. Isaeva, O. M. Nefedov and L. M. Kustov, Catalysts, 2018, 8, 368 CrossRef.
- V. Pascanu, G. González Miera, A. K. Inge and B. Martín-Matute, J. Am. Chem. Soc., 2019, 141, 7223–7234 CrossRef CAS.
- Z. Xue, M.-G. Ma, Z. Li and T. Mu, RSC Adv., 2016, 6, 98874–98892 RSC.
- Z. Hu, Y. Peng, Y. Gao, Y. Qian, S. Ying, D. Yuan, S. Horike, N. Ogiwara, R. Babarao, Y. Wang, N. Yan and D. Zhao, Chem. Mater., 2016, 28, 2659–2667 CrossRef CAS.
- Z. Hu and D. Zhao, in Elaboration and Applications of Metal-Organic Frameworks, 2018, pp. 495–518, DOI:10.1142/9789813226739_0012.
- R. Insyani, D. Verma, S. M. Kim and J. Kim, Green Chem., 2017, 19, 2482–2490 RSC.
- Y.-T. Liao, B. M. Matsagar and K. C. W. Wu, ACS Sustainable Chem. Eng., 2018, 6, 13628–13643 CrossRef CAS.
- L. H. T. Nguyen, T. T. Nguyen, H. L. Nguyen, T. L. H. Doan and P. H. Tran, Catal. Sci. Technol., 2017, 7, 4346–4350 RSC.
- J. Jiang, F. Gándara, Y.-B. Zhang, K. Na, O. M. Yaghi and W. G. Klemperer, J. Am. Chem. Soc., 2014, 136, 12844–12847 CrossRef CAS.
- P. T. Nguyen, T.-D. T. Nguyen, V. S. Nguyen, D. T.-X. Dang, H. M. Le, T.-C. Wei and P. H. Tran, J. Mol. Liq., 2019, 277, 157–162 CrossRef CAS.
- S. Genieva, R. Yankova, G. Baikusheva-Dimitrova and N. Halachev, J. Therm. Anal. Calorim., 2016, 124, 1595–1600 CrossRef CAS.
- D.-J. Tao, Z. Cheng, F.-F. Chen, Z.-M. Li, N. Hu and X.-S. Chen, J. Chem. Eng. Data, 2013, 58, 1542–1548 CrossRef CAS.
- Y. Zhang, V. Degirmenci, C. Li and E. J. M. Hensen, ChemSusChem, 2011, 4, 59–64 CrossRef CAS.
- M. Yabushita, P. Li, T. Islamoglu, H. Kobayashi, A. Fukuoka, O. K. Farha and A. Katz, Ind. Eng. Chem. Res., 2017, 56, 7141–7148 CrossRef CAS.
- Y. Su, G. Chang, Z. Zhang, H. Xing, B. Su, Q. Yang, Q. Ren, Y. Yang and Z. Bao, AIChE J., 2016, 62, 4403–4417 CrossRef CAS.
- I. Elsayed, M. Mashaly, F. Eltaweel, M. A. Jackson and E. B. Hassan, Fuel, 2018, 221, 407–416 CrossRef CAS.
Footnotes |
† Electronic supplementary information (ESI) available. See DOI: 10.1039/d0ra08261a |
‡ These authors contributed equally. |
|
This journal is © The Royal Society of Chemistry 2020 |
Click here to see how this site uses Cookies. View our privacy policy here.