DOI:
10.1039/C9SC04062E
(Edge Article)
Chem. Sci., 2020,
11, 1411-1417
A denitrogenative palladium-catalyzed cascade for regioselective synthesis of fluorenes†
Received
14th August 2019
, Accepted 17th December 2019
First published on 17th December 2019
Abstract
We herein report a denitrogenative palladium-catalyzed cascade for the modular and regioselective synthesis of polysubstituted fluorenes. Hydrazone facilitates the Pd(II) to Pd(IV) oxidative addition in a Catellani pathway and is also the methylene synthon in the proposed reaction. Aryl iodides and 2-bromoarylaldehyde hydrazones undergo a norbornene-controlled tandem reaction sequence to give a broad scope of fluorenes in the presence of a palladium catalyst. The method described is scalable and adaptable to a three-component reaction with in situ generation of the hydrazone group. Preliminary mechanistic investigations have been conducted.
Introduction
Regioselective functionalization of organic molecules is one of the most important, yet challenging objectives in modern synthetic organic chemistry.1 In this regard, the Catellani reaction, which was discovered by the Catellani group in 1997,2 is a powerful and versatile tool for constructing polysubstituted arenes in a regioselective fashion via palladium/norbornene cooperative catalysis.3 The transformation is a tandem difunctionalization of aryl iodides which installs nucleophile ([E]-X) and electrophile ([Nu]-Y) at both ipso- and ortho-positions (Fig. 1A).2,3 After 20 years of advancements, a large number of chemical transformations have been successfully incorporated into the Catellani reaction manifold, which includes Mizoroki–Heck reaction,2,4 Sonogashira coupling,5 Suzuki coupling,6 enolate coupling,7 amination,8 borylation,9 cyanation,10 direct C–H arylation,11 thiolation12 and others.13 Recent contributions in this field have also revealed that Catellani-type reactions can be initiated by Pd(II) species with starting materials other than aryl iodides.14 Amongst the Pd(0) or Pd(II)-initiated Catellani cycles, directing/chelating groups were often used to facilitate the Pd(II)–Pd(IV) oxidative addition or to affect selective arene C–H activation.15 Nevertheless, these assisting groups often remain in the product structure and may not always be a subsequent synthetic handle for future derivatizations. In order to address this pitfall, our group have recently demonstrated the utilization of a deciduous carboxyl group16 to facilitate the Pd(II)/(IV) oxidative addition in Catellani-type aromatic π-extension reactions (Fig. 1B).17 As part of our continuing efforts in Pd/norbornene chemistry, we aim to explore new methods to access other chemical classes. Hydrazones have been reported to be a useful directing group in Pd-, Rh- or Ir-catalyzed reactions (Fig. 1C),18 and we sought to adopt it as an assisting group to facilitate the heterobiaryl formation in the Catellani cycle. During our investigations, we found that aryl iodides reacted with 2-bromoarylaldehyde hydrazone to give fluorenes under typical Catellani reaction conditions. Herein we report the development and results of a novel denitrogenative palladium-catalyzed cascade for the modular and regioselective synthesis of fluorenes (Fig. 1D).
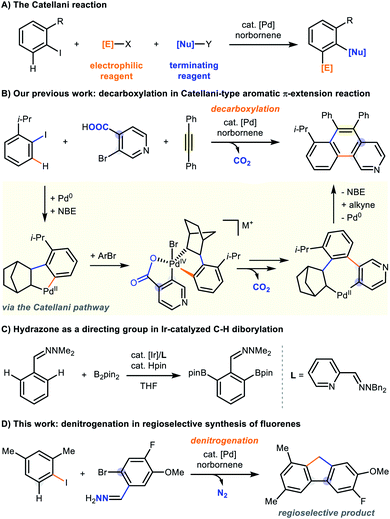 |
| Fig. 1 (A) A typical Catellani reaction; (B) previous work: decarboxylative aromatic π-extension reactions; (C) hydrazone as a directing group in Ir-catalyzed C–H borylations; (d) this work: Pd-catalyzed norbornene-mediated denitrogenative synthesis of fluorenes. | |
Although fluorene and its derivatives are ubiquitous structural motifs in light-emitting optoelectronics,19 organic semiconductors,20 nanostructures,21 functional polymers,20c,22 chemosensors19a,23 and bioactive compounds,24 we identified a multitude of challenges on their syntheses based on literature precedents. Traditional synthesis of substituted fluorenes mostly rely on the post-functionalization of 9H-fluorene via lengthy sequences (Fig. 2A),20a while transition metal-catalyzed reactions have also been developed to efficiently prepare fluorenes in one pot (Fig. 2B).25 Construction of the methylene group employs intramolecular direct C sp3–H activation or cleavage of a C sp3–X bond, but these biaryl substrates require pre-activation and inherently offset the methods' accessibility and scope.20c,26 These pre-functionalized substrates are typically prepared in multiple synthetic steps and the C9 (methylene) position is often substituted with functional groups, which cannot be readily converted into other moieties, such as alkyl chains that are important for liquid crystal formation.19a,27 Recently, impressive carbenoid cross-coupling strategies have been disclosed by the groups of Wang, Hu and others.28 However, the formation of regioisomers was still possible due to the unselective oxidative addition of C–Br/Cl bond and C–H activation. Although cross-dehydrogenative coupling (CDC) is useful in the preparation of heterocycles (Fig. 2C),29 it is not applicable for accessing fluorenes owing to the lack of directing abilities of the methylene group in the diarylmethane scaffold.
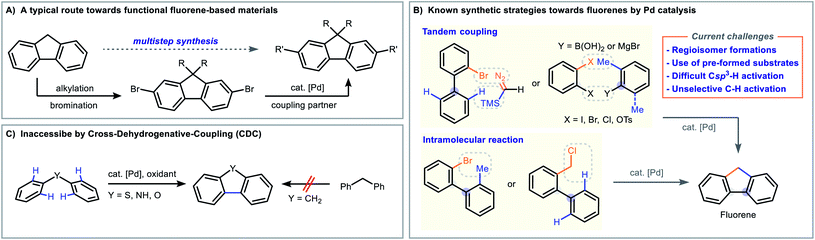 |
| Fig. 2 Approaches and difficulties of fluorene syntheses; (A) traditional multistep synthesis of fluorenes; (B) Pd-catalyzed tandem or intramolecular synthesis of fluorenes; (C) lack of directing abilities of methylene group in fluorene. | |
Results and discussion
At the outset of our investigation, we found that the reaction between 2-bromobenzaldehyde hydrazone (2a) and 2-iodotoluene has given fluorene 3a in 38% yield in the presence of a Pd(OAc)2/PPh3 catalyst and norbornene (Table 1, entry 1). An optimization study of reaction parameters was then undertaken with a survey of phosphine ligands, solvents bases and stoichiometry of reagents. Selected entries of our optimization have been shown in Table 1 (please see Tables S1–S3 in ESI† for detailed optimization data). Amongst the trialkyl or triaryl monophosphine ligands screened (entries 1–4), PCyPh2 provided the highest product yield (74%, entry 3). Either increasing or decreasing the electron richness of the phosphorus donor led to a drop in product yield. Bulky cataCXium A and other biaryl phosphine ligands showed inferior activity in this comparison (entries 5–7). Bidentate phosphine such as DPPF was not suitable for this reaction (entry 8). Cs2CO3 gave a similar yield to that of K2CO3 and the later one was chosen for further study because of its economical attractiveness (entry 3 vs. 9). Gratifyingly, the product yield was further increased to 80% when DMF was employed as solvent (entry 3 vs. 11). Other solvents gave comparable performances to that of toluene (entries 12–13), except acetonitrile (entry 14). A control experiment without norbornene was carried out and desired product was not observed (entry 15).
Table 1 Selected entries for reaction optimizationa

|
Entry |
Ligand |
Base |
Solvent |
% Yieldb |
Reaction conditions: Pd(OAc)2 (10 mol%), ligand (25 mol%), 2-iodotoluene (0.2 mmol), (2-bromobenzylidene)hydrazine (0.24 mmol), norbornene (0.3 mmol), base (0.6 mmol), solvent (0.1 M), 130 °C for 18 h under N2.
Calibrated GC-FID yields.
12.5 mol% of DPPF was used.
Reaction without norbornene.
|
1 |
PPh3 |
K2CO3 |
Toluene |
38 |
2 |
PCy3 |
K2CO3 |
Toluene |
57 |
3 |
PCyPh2 |
K2CO3 |
Toluene |
74 |
4 |
P(4-OMeC6H4)3 |
K2CO3 |
Toluene |
43 |
5 |
cataCXium A |
K2CO3 |
Toluene |
13 |
6 |
XPhos |
K2CO3 |
Toluene |
37 |
7 |
PPh2-DavePhos |
K2CO3 |
Toluene |
45 |
8c |
DPPF |
K2CO3 |
Toluene |
31 |
9 |
PCyPh2 |
Cs2CO3 |
Toluene |
73 |
10 |
PCyPh2 |
Na2CO3 |
Toluene |
5 |
11 |
PCyPh2 |
K2CO3 |
DMF |
80 |
12 |
PCyPh2 |
K2CO3 |
NMP |
78 |
13 |
PCyPh2 |
K2CO3 |
1,4-Dioxane |
76 |
14 |
PCyPh2 |
K2CO3 |
CH3CN |
35 |
15d |
PCyPh2 |
K2CO3 |
DMF |
0 |
|
Having identified an optimal reaction conditions, we then studied the reaction scope of our reaction. Aryl iodides with different electron richness and steric bulkiness were first tested (Table 2, 3a–3l, 3q). Electron-rich and – neutral aryl iodides were converted to the desired products in 68–82% isolated yields (3a–3e, 3h–3i). However, substrates bearing an electron-withdrawing group such as –CF3 caused a drop in yield and 3f was isolated in 48% yield. Aryl iodides with sterically hindered ortho-substituents such as phenyl or isopropyl groups underwent the transformation smoothly to afford the corresponding fluorenes 3g and 3t in moderate-to-good yields. Heteroatom-containing and polyaromatic substrates were tolerated (3j–3l) and it is worth to mention that polyaromatic hydrocarbons (PAHs) contribute important properties in light-emitting devices and fluorophores.30 We next turned our attention to the scope of aryl hydrazones 2. We were pleased that the transformations proceeded efficiently to give multi-substituted fluorenes 3l–3z with complete regioselectivity (up to 90% yield). The structure of 3s was determined by single crystal X-ray analysis.31 Aryl hydrazones containing electron-donating and -withdrawing groups were applicable substrates (3m–3s). Polycyclic fluoreno[1,3]dioxoles 3t and 3u were isolated in 80% yield. The alkyl aryl ether (3w) and acetal group (3y) can be readily transformed into phenol and aldehyde, respectively. Chloro group (3x) was compatible and provides access to further functionalizations by palladium-catalyzed cross-couplings.32 Estrone-derived aryl iodide also reacted under the standard conditions to provide 3z in 75% isolated yield. Interestingly, reaction of aryl iodides without ortho-substituents, or with small ortho-substituents such as fluorine, gave norbornene-embedded heptagon compounds,33 in which it showed that the steric hindrance exerted by the ortho-substituents is critical for the extrusion of norbornene and thus the five-membered ring formation. Further study of this outcome is currently underway in our laboratory.
Reaction conditions: Pd(OAc)2 (10 mol%), PCyPh2 (25 mol%), aryl iodide (0.2 mmol), 2 (0.24 mmol), norbornene (0.3 mmol), K2CO3 (0.6 mmol), DMF (0.1 M), 130 °C for 18 h under N2. Isolated yields were reported.
|
|
To demonstrate the synthetic utility of our method in a wider scope, we carried out a gram-scale synthesis and 1.26 g of fluorene 3a was successfully obtained under standard reaction conditions (Scheme 1A). A diiodide substrate underwent the transformation successfully to form a dialkyl ether-linked difluorene 4 in 51% yield (Scheme 1B). To circumvent the access of hydrazones in this tandem reaction, we sought to adapt our system to a three-component reaction with in situ generation of the aryl hydrazone using 2-bromobenzaldehyde and hydrazine (Scheme 1C). All commercially available materials were employed and the desired product 3a was given in 73% isolated yield, in which the yield only decreased slightly in comparison to entry 3a of Table 2.
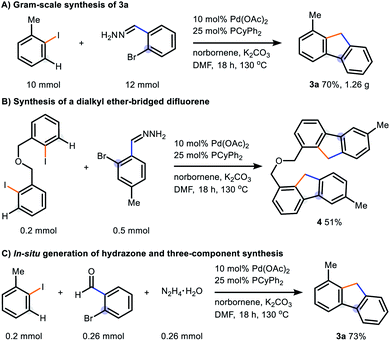 |
| Scheme 1 Synthetic utilities of our reaction; (A) scalability of our reaction; (B) reaction of a diiodide substrate; (C) in situ generation of hydrazone in a three-component reaction. | |
A mechanistic proposal of our reaction is illustrated in Scheme 2. The cascade initiates with the oxidative addition of 2-iodotoluene to Pd(0), followed by a migratory insertion of NBE and palladation of the ortho-C–H bond to give the ANP (aryl-norbornene palladacycle). Based on our previous works and literature reports, it is reasonable to hypothesize the formation of a Pd(IV) intermediate A from ANP.3,17 The hydrazone chelates to the Pd(II) and assists with the oxidative addition of 2a by allowing the C–Br bond to sit near the Pd centre. The reductive elimination of A generates the biarylpalladium(II) species B in a regioselective fashion and subsequent β-carbon elimination of norbornene gives the key intermediate C. We have observed a trace amount of intermediate E on GC-MS during the optimization studies, which suggests a possible C–H activation on the hydrazone to form palladacycle D. The Wolff–Kishner reduction of E gives the final product 3a.34 Alternatively, another possible pathway could be similar to the cross-coupling of Umpolung aldehydes, which were very recently reported by Li and co-workers.35 The deprotonation of hydrazone forms a carbon nucleophile, which upon substitution with the bromide would yield palladacycle F. Reductive elimination of F regenerates Pd(0) and affords intermediate G, which undergoes denitrogenation to give 3a under a basic condition. Although a deuterium labelling study was attempted (Scheme S1†), the results were not conclusive due to the H/D exchange nature of the C9-proton (pKa = 22.6 in DMSO for fluorene) under basic conditions.36 The Wolff–Kishner reduction was also tested by subjecting hydrazone 5 under standard reaction conditions in the absence of palladium catalyst (Scheme 3A) and 50% yield of fluorene 6 was obtained. However, the addition of 10 mol% Pd catalyst to the system increased the product yield of 6 to 73%, in which it is possible that the reduction could be assisted by a palladium process. Control experiments regarding the reaction pathway were carried out (Scheme 3B). An N-tosyl hydrazone 7 was subjected to the reaction system but no desired product was obtained, indicating the absence of a carbene migratory insertion within intermediate C. When the ketone-derived hydrazone 8 was used, no corresponding product was detected, in which it reveals the importance of the C–H site for intramolecular C–H activation by the Pd(II) centre.
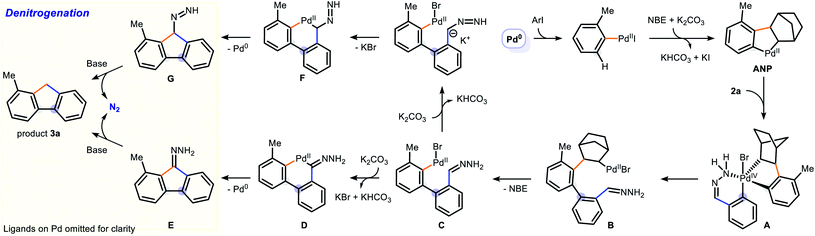 |
| Scheme 2 Mechanistic proposal. | |
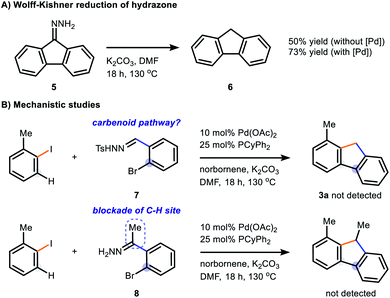 |
| Scheme 3 (A) Wolff-Kishner reaction of 9-fluorenone hydrazone under standard conditions as shown in Table 2, [Pd] = 10 mol% Pd(OAc)2 and 25 mol% PCyPh2; (B) mechanistic studies. | |
Conclusions
In summary, a denitrogenative palladium-catalyzed cascade has been developed to allow for facile assembly of polysubstituted fluorenes. Hydrazone was utilized as a deciduous chelating group to facilitate the Pd(II)/Pd(IV) oxidative addition in a Catellani cycle while it also served as a methylene synthon for the fluorene C-9 carbon. This modular synthesis exhibited complete regioselectivity and provided fluorenes with various substitution patterns in good-to-excellent product yields. In situ generation of hydrazones from aldehydes has also been illustrated in a three-component cross-coupling reaction. We believe that the reaction scope, as well as the use of a commercial catalyst and easily available reactants, would render our method attractive and versatile for synthetic applications in material science, medicinal chemistry and organic chemistry.
Conflicts of interest
There are no conflicts to declare.
Acknowledgements
We thank RGC of Hong Kong (GRF: 14307418) for financial support. CUHK direct grant (4053269, 4053322, 4442122) is gratefully acknowledged. We thank Dr Pui Ying Choy (CUHK) for helpful discussions. We also thank the financial support from Innovation and Technology Commission (HKSAR, China) to the State Key Laboratory of Synthetic Chemistry.
Notes and references
-
(a) J. F. Hartwig, Chem. Soc. Rev., 2011, 40, 1992 RSC;
(b) Y. Segawa, T. Maekawa and K. Itami, Angew. Chem., Int. Ed., 2015, 54, 66 CrossRef CAS PubMed;
(c) H. Ito, K. Ozaki and K. Itami, Angew. Chem., Int. Ed., 2017, 56, 11144 CrossRef CAS PubMed;
(d) J. Jeon, H. Ryu, C. Lee, D. Cho, M.-H. Baik and S. Hong, J. Am. Chem. Soc., 2019, 141, 10048 CrossRef CAS PubMed.
- M. Catellani, F. Frignani and A. Rangoni, Angew. Chem., Int. Ed., 1997, 36, 119 CrossRef CAS.
- Recent reviews on the Catellani reaction:
(a) M. Catellani, E. Motti and N. Della Ca', Acc. Chem. Res., 2008, 41, 1512 CrossRef CAS PubMed;
(b) A. Martins, B. Mariampillai and M. Lautens, Top. Curr. Chem., 2009, 292, 1 CrossRef PubMed;
(c) R. Ferraccioli, Synthesis, 2013, 45, 581 CrossRef CAS;
(d) J. Ye and M. Lautens, Nat. Chem., 2015, 7, 863 CrossRef CAS PubMed;
(e) N. Della Ca', M. Fontana, E. Motti and M. Catellani, Acc. Chem. Res., 2016, 49, 1389 CrossRef PubMed;
(f) J. Wang and G. Dong, Chem. Rev., 2019, 119, 7478 CrossRef CAS PubMed.
-
(a) M. Lautens and S. Piguel, Angew. Chem., Int. Ed., 2000, 39, 1045 CrossRef CAS;
(b) F. Faccini, E. Motti and M. Catellani, J. Am. Chem. Soc., 2004, 126, 78 CrossRef CAS PubMed.
- E. Motti, M. Rossetti, G. Bocelli and M. Catellani, J. Organomet. Chem., 2004, 689, 3741 CrossRef CAS.
-
(a) M. Catellni, E. Motti and M. Minari, Chem. Commun., 2000, 157 RSC;
(b) C. Ye, H. Zhu and Z. Chen, J. Org. Chem., 2014, 79, 8900 CrossRef CAS PubMed.
-
(a) G. Maestri, N. Della Ca' and M. Catellani, Chem. Commun., 2009, 4892 RSC;
(b) W. C. Fu, B. Zheng, Q. Zhao, W. T. K. Chan and F. Y. Kwong, Org. Lett., 2017, 19, 4335 CrossRef CAS PubMed.
-
(a) P. Thansandote, M. Raemy, A. Rudolph and M. Lautens, Org. Lett., 2007, 9, 5255 CrossRef CAS PubMed;
(b) D. A. Candito and M. Lautens, Org. Lett., 2010, 12, 3312 CrossRef CAS PubMed.
- H. Shi, D. J. Babinski and T. Ritter, J. Am. Chem. Soc., 2015, 137, 3775 CrossRef CAS PubMed.
-
(a) B. Mariampillai, D. Alberico, V. Bidau and M. Lautens, J. Am. Chem. Soc., 2006, 127, 14436 CrossRef PubMed;
(b) B. Luo, J.-M. Gao and M. Lautens, Org. Lett., 2016, 18, 4166 CrossRef CAS PubMed.
- C. Bressy, D. Alberico and M. Lautens, J. Am. Chem. Soc., 2005, 127, 13148 CrossRef CAS PubMed.
- F. Sun, M. Li, C. He, B. Wang, B. Li, X. Sui and Z. Gu, J. Am. Chem. Soc., 2016, 138, 7456 CrossRef CAS PubMed.
- Recent examples of Catellani-type reactions:
(a) Z. Dong and G. Dong, J. Am. Chem. Soc., 2013, 135, 18350 CrossRef CAS PubMed;
(b) P.-X. Zhou, Y.-Y. Ye, C. Liu, L.-B. Zhao, J.-Y. Hou, D.-Q. Chen, Q. Tang, A.-Q. Wang, J.-Y. Zhang, Q.-X. Huang, P.-F. Xu and Y.-M. Liang, ACS Catal., 2015, 5, 4927 CrossRef CAS;
(c) Z. Dong, J. Wang, Z. Ren and G. Dong, Angew. Chem., Int. Ed., 2015, 54, 12664 CrossRef CAS PubMed;
(d) X. Sui, L. Ding and Z. Gu, Chem. Commun., 2016, 52, 13999 RSC;
(e) L. Fan, J. Liu, L. Bai, Y. Wang and X. Luan, Angew. Chem., Int. Ed., 2017, 56, 14257 CrossRef CAS PubMed;
(f) G. Qian, M. Bai, S. Gao, H. Chen, S. Zhou, H.-G. Cheng, W. Yan and Q. Zhou, Angew. Chem., Int. Ed., 2018, 57, 10980 CrossRef CAS PubMed;
(g) Z. Dong, G. Lu, J. Wang, P. Liu and G. Dong, J. Am. Chem. Soc., 2018, 140, 8551 CrossRef CAS PubMed;
(h) L. Bai, J. Liu, W. Hu, K. Li, Y. Wang and X. Luan, Angew. Chem., Int. Ed., 2018, 57, 5151 CrossRef CAS PubMed;
(i) Y. Yang, B. Zhou, X. Zhu, G. Deng, Y. Liang and Y. Yang, Org. Lett., 2018, 20, 5402 CrossRef CAS PubMed;
(j) H. Zhou, W. Chen and Z. Chen, Org. Lett., 2018, 20, 2590 CrossRef CAS PubMed;
(k) J. Wang, R. Li, Z. Dong, P. Liu and G. Dong, Nat. Chem., 2018, 10, 866 CrossRef CAS PubMed;
(l) A. Whyte, M. E. Olson and M. Lautens, Org. Lett., 2018, 20, 345 CrossRef CAS PubMed;
(m) J. Nan, Y. Yuan, L. Bai, J. Liu and X. Luan, Org. Lett., 2018, 20, 7731 CrossRef CAS PubMed;
(n) A. Casnati, M. Fontana, G. Coruzzi, B. M. Aresta, N. Corriero, R. Maggi, G. Maestri, E. Motti and N. Della Ca', ChemCatChem, 2018, 10, 4346 CrossRef CAS;
(o) R. Li and G. Dong, Angew. Chem., Int. Ed., 2018, 57, 1967 Search PubMed;
(p) W. Li, W. Chen, B. Zhou, Y. Xu, G. Deng, Y. Liang and Y. Yang, Org. Lett., 2019, 21, 2718 CrossRef CAS PubMed;
(q) P. Wang, S. Chen, Z. Zhou, H.-G. Cheng and Q. Zhou, Org. Lett., 2019, 21, 3323 CrossRef CAS PubMed;
(r) S. Chen, P. Wang, H.-G. Cheng, C. Yang and Q. Zhou, Chem. Sci., 2019, 10, 8384–8389 RSC.
- H.-G. Cheng, S. Chen, R. Chen and Q. Zhou, Angew. Chem., Int. Ed., 2019, 58, 5832 CrossRef CAS PubMed.
- For the use of directing groups in Catellani-type reactions:
(a) Y.-B. Zhao, B. Mariampillai, D. A. Candito, B. Laleu, M. Li and M. Lautens, Angew. Chem., Int. Ed., 2009, 48, 1849 CrossRef CAS PubMed;
(b) P. Thansandote, E. Cheng, K.-O. Feldmann and M. Lautens, J. Org. Chem., 2010, 75, 3495 CrossRef CAS PubMed;
(c) A. Martins, D. A. Candito and M. Lautens, Org. Lett., 2010, 12, 5186 CrossRef CAS PubMed;
(d) M. Larraufie, G. Maestri, A. Beaume, É. Derat, C. Ollivier, L. Fensterbank, C. Courillon, E. Lacôte, M. Catellani and M. Malacria, Angew. Chem., Int. Ed., 2011, 50, 12253 CrossRef CAS PubMed;
(e) Z. Dong, J. Wang and G. Dong, J. Am. Chem. Soc., 2015, 137, 5887 CrossRef CAS PubMed;
(f) J. Han, L. Zhang, Y. Zhu, Y. Zheng, X. Chen, Z.-B. Huang, D.-Q. Shi and Y. Zhao, Chem. Commun., 2016, 52, 6903 RSC;
(g) D. Rasina, A. Kahler-Quesada, S. Ziarelli, S. Warratz, H. Cao, S. Santoro, L. Ackermann and L. Vaccaro, Green Chem., 2016, 18, 5025 RSC;
(h) P.-X. Ling, K. Chen and B.-F. Shi, Chem. Commun., 2017, 53, 2166 RSC.
- Gooßen and co-workers first introduced carboxyl group as a deciduous directing group:
(a) L. Huang, A. Biafora, G. Zhang, V. Bragoni and L. J. Gooßen, Angew. Chem., Int. Ed., 2016, 55, 6933 CrossRef CAS PubMed;
(b) A. Biafora and L. J. Gooßen, Synlett, 2017, 28, 1885 CrossRef CAS. For selected examples on the use of deciduous carboxylates in transition metal-catalyzed reactions:
(c) J. Tang, D. Hackenberger and L. J. Gooßen, Angew. Chem., Int. Ed., 2016, 55, 11296 CrossRef CAS PubMed;
(d) A. Biafora, B. A. Khan, J. Bahri, J. M. Hewer and L. J. Gooßen, Org. Lett., 2017, 19, 1232 CrossRef CAS PubMed;
(e) K. R. Bettadapur, V. Lanke and K. R. Prabhu, Chem. Commun., 2017, 53, 6251 RSC;
(f) R. N. P. Tulichala, M. Shankar and K. C. K Swamy, J. Org. Chem., 2018, 83, 4375 CrossRef CAS PubMed.
-
(a) W. C. Fu, Z. Wang, W. T. K. Chan, Z. Lin and F. Y. Kwong, Angew. Chem., Int. Ed., 2017, 56, 7166 CrossRef CAS PubMed;
(b) Q. Zhao, W. C. Fu and F. Y. Kwong, Angew. Chem., Int. Ed., 2018, 57, 3381 CrossRef CAS PubMed.
-
(a) A. Ros, R. López-Rodríguez, B. Estepa, E. Álvarez, R. Fernández and J. M. Lassaletta, J. Am. Chem. Soc., 2012, 134, 4573 CrossRef CAS PubMed;
(b) Z. Huang, C. Wang and G. Dong, Angew. Chem., Int. Ed., 2016, 55, 5299 CrossRef CAS PubMed;
(c) P. Xu, G. Wang, Z. Wu, S. Li and C. Zhu, Chem. Sci., 2017, 8, 1303 RSC.
-
(a) M. P. Aldred, A. J. Eastwood, S. M. Kelly, P. Vlachos, A. E. A. Contoret, S. R. Farrar, B. Mansoor, M. O'Neill and W. C. Tsoi, Chem. Mater., 2004, 16, 4928 CrossRef CAS;
(b)
J. U. Wallace and S. H. Chen, in Polyfluorenes Advances in Polymer Science, ed. U. Scherf and D. Neher, Springer, Berlin, Heidelberg, 2008, pp. 145–186 Search PubMed;
(c) R. Abbel, A. P. H. J. Schenning and E. W. Meijer, J. Polym. Sci., Part A: Polym. Chem., 2009, 47, 4215 CrossRef CAS.
-
(a) J. Locklin, M. M. Ling, A. Sung, M. E. Roberts and Z. Bao, Adv. Mater., 2006, 18, 2989 CrossRef CAS;
(b) T. Qi, Y. Liu, W. Qiu, H. Zhang, X. Gao, Y. Liu, K. Lu, C. Du, G. Yu and D. Zhu, J. Mater. Chem., 2008, 18, 1131 RSC;
(c) H. Chen, M. Hurhangee, M. Nikolka, W. Zhang, M. Kirkus, M. Neophytou, S. J. Cryer, D. Harkin, P. Hayoz, M. Abdi-Jalebi, C. R. McNeill, H. Sirringhaus and I. McCulloch, Adv. Mater., 2017, 29, 1702523 CrossRef PubMed.
-
(a) L. Wang, G.-W. Zhang, C.-J. Ou, L.-H. Xie, J.-Y. Lin, Y.-Y. Liu and W. Huang, Org. Lett., 2014, 16, 1748 CrossRef CAS PubMed;
(b) H. Zhong, C. Liu, Y. Wang, R. Wang and M. Hong, Chem. Sci., 2016, 7, 2188 RSC;
(c) L. Wang, B. Dong, R. Ge, F. Jiang and J. Xu, ACS Appl. Mater. Interfaces, 2017, 9, 7108 CrossRef CAS PubMed.
-
(a) A. Donat-Bouillud, I. Lévesque, Y. Tao, M. D'lorio, S. Beaupré, P. Blondin, M. Ranger, J. Bouchard and M. Leclerc, Chem. Mater., 2000, 12, 1931 CrossRef CAS;
(b) Q. Chen, J.-X. Wang, Q. Wang, N. Bian, Z.-H. Li, C.-G. Yan and B.-H. Han, Marcomolecules, 2011, 44, 7987 CrossRef CAS;
(c) K. Nakabayashi, T. Imai, M.-C. Fu, S. Ando, T. Higashihara and M. Ueda, Marcomolecules, 2016, 49, 5849 CrossRef CAS.
-
(a) S. Yao, H.-Y. Ahn, X. Wang, J. Fu, E. W. V. Stryland, D. J. Hagan and K. D. Belfield, J. Org. Chem., 2010, 75, 3965 CrossRef CAS PubMed;
(b) K. D. Belfield, M. V. Bondar, A. Frazer, A. R. Morales, O. D. Kachkovsky, I. A. Mikhailov, A. E. Masunov and O. V. Przhonska, J. Phys. Chem. B, 2010, 114, 9313 CrossRef CAS PubMed.
-
(a) G. Kumar, R. Agarwal and S. Swaminathan, Chem. Commun., 2012, 48, 2412 RSC;
(b) Y. Shi and S. Gao, Tetrahedron, 2016, 72, 1717 CrossRef CAS.
-
(a) K. Funchibe and T. Akiyama, J. Am. Chem. Soc., 2006, 128, 1434 CrossRef PubMed;
(b) N. Chernyak and V. Gevorgyan, J. Am. Chem. Soc., 2008, 130, 5636 CrossRef CAS PubMed;
(c) D. J. Gorin, I. D. G. Watson and F. D. Toste, J. Am. Chem. Soc., 2008, 130, 3736 CrossRef CAS PubMed;
(d) S. Zhu, L. Wu and X. Huang, Org. Biomol. Chem., 2012, 10, 3696 RSC;
(e) Z. Liu, H. Tan, L. Wang, T. Fu, Y. Xia, Y. Zhang and J. Wang, Angew. Chem., Int. Ed., 2015, 54, 3056 CrossRef CAS PubMed;
(f) Z.-J. Cai, F.-H. Li, S.-Y. Wang and S.-J. Ji, Org. Lett., 2016, 18, 4810 CrossRef CAS PubMed.
-
(a) S. J. Hwang, H. J. Kim and S. Chang, Org. Lett., 2009, 11, 4588 CrossRef CAS PubMed;
(b) C.-C. Hsiao, Y.-K. Lin, C.-J. Liu, T.-C. Wu and Y.-T. Wu, Adv. Synth. Catal., 2010, 352, 3267 CrossRef CAS.
- X. Zhang, L. Bu, Y. Qu, L. Wang, Y. Geng and F. Wang, J. Mater. Chem., 2009, 19, 399 RSC.
-
(a) C.-G. Dong and Q.-S. Hu, Org. Lett., 2006, 8, 5057 CrossRef CAS PubMed;
(b) T.-P. Liu, C.-H. Xing and Q.-S. Hu, Angew. Chem., Int. Ed., 2010, 49, 2909 CrossRef CAS PubMed;
(c) S. Xu, X. Shangguan, H. Li, Y. Zhang and J. Wang, J. Org. Chem., 2015, 80, 7779 CrossRef CAS PubMed;
(d) G. Shi, D. Chen, H. Jiang, Y. Zhang and Y. Zhang, Org. Lett., 2016, 18, 2958 CrossRef CAS PubMed;
(e) J. Song, W. Sun, Y. Li, F. Wei, C. Liu, Y. Qian and S. Chen, Chem.–Asian J., 2016, 11, 211 CrossRef CAS PubMed;
(f) S. Xu, R. Chen, Z. Fu, Q. Zhou, Y. Zhang and J. Wang, ACS Catal., 2017, 7, 1993 CrossRef CAS.
-
(a) B. Liégault, D. Lee, M. P. Huestis, D. R. Stuart and K. Fagnou, J. Org. Chem., 2008, 73, 5022 CrossRef PubMed;
(b) R. Che, Z. Wu, Z. Li, H. Xiang and X. Zhou, Chem.–Eur. J., 2014, 20, 7258 CrossRef CAS PubMed;
(c) D.-D. Guo, B. Li, D.-Y. Wang, Y.-R. Gao, S.-H. Guo, G.-F. Pan and Y.-Q. Wang, Org. Lett., 2017, 19, 798 CrossRef CAS PubMed.
-
(a) Z. Sun, Q. Ye, C. Chi and J. Wu, Chem. Soc. Rev., 2012, 41, 7857 RSC;
(b) M. Li, C. An, T. Marszalek, X. Guo, Y.-Z. Long, H. Yin, C. Gu, M. Baumgarten, W. Pisula and K. Müllen, Chem. Mater., 2015, 27, 2218 CrossRef CAS.
- The X-ray crystallographic data for 3s (CCDC 1833356).
-
(a) T. Jia, A. Bellomo, K. E. Baina, S. D. Dreher and P. J. Walsh, J. Am. Chem. Soc., 2013, 135, 3740 CrossRef CAS PubMed;
(b) J. Zhang, A. Bellomo, N. Trongsiriwat, T. Jia, P. J. Carroll, S. D. Dreher, M. T. Tudge, H. Yin, J. R. Robinson, E. J. Schelter and P. J. Walsh, J. Am. Chem. Soc., 2014, 136, 6276 CrossRef CAS PubMed.
- The general structure is 4b,6,7,8,8a,9-hexahydro-5H-5,8-methanotribenzo[a,c,e][7]annulene.
-
(a) N. Kishner, J. Russ. Phys.-Chem. Soc., 1911, 43, 582 Search PubMed;
(b) L. Wolff, Justus Liebigs Ann. Chem., 1912, 394, 86 CrossRef; Recent literature:
(c) M. E. Furrow and A. G. Myers, J. Am. Chem. Soc., 2004, 126, 5436 CrossRef CAS PubMed;
(d) X.-J. Dai and C.-J. Li, J. Am. Chem. Soc., 2016, 138, 5433 CrossRef CAS PubMed.
-
(a) D. Zhu, L. Lv, C.-C. Li, S. Ung, J. Gao and C.-J. Li, Angew. Chem., Int. Ed., 2018, 57, 16520 CrossRef CAS PubMed;
(b) L. Lv, D. Zhu, J. Tang, Z. Qiu, C.-C. Li, J. Gao and C.-J. Li, ACS Catal., 2018, 8, 4622 CrossRef CAS;
(c) D. Zhu, L. Lv, Z. Qiu and C.-J. Li, J. Org. Chem., 2019, 84, 6312 CrossRef CAS PubMed.
- F. G. Bordwell, Acc. Chem. Res., 1988, 21, 456 CrossRef CAS.
Footnotes |
† Electronic supplementary information (ESI) available: Detailed experimental procedures, 1H, 13C NMR spectra, and characterization data of all new compounds. CCDC 1833356. For ESI and crystallographic data in CIF or other electronic format see DOI: 10.1039/c9sc04062e |
‡ Present address: Department of Chemistry, Massachusetts Institute of Technology 77 Massachusetts Avenue, Cambridge, MA 02139 (USA). |
|
This journal is © The Royal Society of Chemistry 2020 |
Click here to see how this site uses Cookies. View our privacy policy here.