DOI:
10.1039/C9SC05101E
(Edge Article)
Chem. Sci., 2020,
11, 2472-2478
Palladium catalysed C–H arylation of pyrenes: access to a new class of exfoliating agents for water-based graphene dispersions†
Received
9th October 2019
, Accepted 23rd January 2020
First published on 28th January 2020
Abstract
A new and diverse family of pyrene derivatives was synthesised via palladium-catalysed C–H ortho-arylation of pyrene-1-carboxylic acid. The strategy affords easy access to a broad scope of 2-substituted and 1,2-disubstituted pyrenes. The C1-substituent can be easily transformed into carboxylic acid, iodide, alkynyl, aryl or alkyl functionalities. This approach gives access to arylated pyrene ammonium salts, which outperformed their non-arylated parent compound during aqueous Liquid Phase Exfoliation (LPE) of graphite and compare favourably to state-of-the-art sodium pyrene-1-sulfonate PS1. This allowed the production of concentrated and stable suspensions of graphene flakes in water.
Introduction
During the last few years graphene has emerged as a revolutionary material attracting much attention due to its unique properties and multiple applications.1 The first isolation of graphene by Geim and coworkers in 2004 using the “Scotch-tape method” allowed its identification and spurred many others to further investigate the material and develop new and more efficient methods of production. On this regard, LPE offers a straightforward approach to obtain graphene dispersions directly from graphite.2 LPE is based on exposing directly the material to a solvent with a surface tension that favours an increase in the total area of graphite crystallites.2a Typically solvents used are NMP and DMF. However, LPE in water is also possible by using an exfoliating agent.3 This also allows to non-covalently functionalise the graphene flakes in situ, by tuning their surface chemistry depending on the exfoliating agent used.2i
Non-covalent functionalisation of graphene offers an opportunity to add new motifs and tune the surface chemistry of graphene without disrupting its inherent conjugation and thus, its high conductivity.3 In this context, pyrene derivatives have arisen as a privileged scaffold due to its strong affinity for graphene.4 For instance, several types of pyrene derivatives (Fig. 1) have been used to develop graphene-based biosensors,5 water-based electronic inks,6 composite materials,7 light-harvesting devices,8 among many.
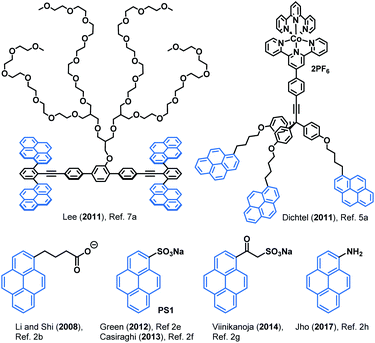 |
| Fig. 1 Selected pyrene-based compounds used in graphene non-covalent functionalisation and liquid phase exfoliation. | |
Although reports on pyrene-functionalised graphene flakes are very abundant, examples of cationic pyrene-derived surfactants used to obtain suspensions of positively charged graphene flakes are scarce and require further investigation.2e,f Most methods of pyrene derivatisation rely on electrophilic aromatic substitutions and its clear preference to yield 1-substituted pyrene derivatives.9 Nonetheless, several 2-substituted pyrenes have been produced after Marder and co-workers unveiled a method to generate 2-borylated and 2,7-diborylated pyrene,10 which can be extensively derivatised to obtain aryl, alkyl, alkynyl and amino derivatives, among others.10b Only a few methods have been reported for the directed C–H arylation of pyrene derivatives (Scheme 1).11 Notably, the work of Zhong and co-workers reports a scope of 2-arylated and 2,7-diarylated products when one or two 2-pyridyl groups are present in the 1- or in the 1- and 6-positions, respectively. Moreover, the directed C–H alkynylation, alkenylation and borylation of aromatics have also been tested in pyrene substrates but only a handful of examples have been reported.12–14 In order to access a varied family of pyrene derivatives that can find use among the multiple applications outlined above we envisioned that commercially available pyrene-1-carboxylic acid (1) would offer a flexible platform to that end. The palladium-catalysed C–H ortho-arylation of aryl carboxylic acids offers an oxygen and moisture-compatible way to derivatise these common feedstocks while choosing the fate of the acid moiety, which can be preserved or removed during the arylation reaction by judicious choice of reagents and conditions.15–17 Thus, the use of a silver-salt additive can lead to decarboxylated products,16 whilst replacing it with a tetramethylammonium salt, the ortho-arylated carboxylic acids are obtained.17b,c
 |
| Scheme 1 Overview of C–H arylation of pyrene derivatives. | |
Results and discussion
The feasibility of the proposed approach was first assessed using pyrene-1-carboxylic acid (1) and 3,5-iodoxylene (2a). After screening of the reaction parameters,18 both the 2-arylated pyrenecarboxylic acid 3a and the corresponding decarboxylated 2-arylpyrene 5a could be obtained in good yields (Table 1, entries 3a and 5a). Arylated carboxylic acids 3 were obtained in very good yields regardless of the substituents in iodoarenes 2. Both electron-withdrawing and electron-donating functionalities were broadly tolerated; haloarenes were also obtained in good yields (entries 3g–j) and the use of trifluoromethylated aryl iodides led to excellent yields (entries 3k and 3m). Ester-substituted products, which are amenable for further derivatisation, were also obtained in good yields (entries 3o and 3p). On the other hand, products of decarboxylative C–H arylation 5 were obtained in moderate to good yields in most cases and only in two particular cases the decarboxylative coupling proved inefficient (entries 5k and 5n). Nonetheless, the desired products could still be obtained in good yields by decarboxylation of the corresponding carboxylic acid.19 Importantly, this decarboxylative coupling can be a useful one-step alternative to access 2-arylpyrenes. Finally, using a modified protocol of our recent iododecarboxylation of carboxylic acids,20 arylated pyrene acids 3 could be further derivatised into the corresponding iodoarenes 4,18 which grant access to a whole new family of unexplored pyrene derivatives (vide infra). In this case yields ranged from moderate to good, although as expected, the best yields were obtained among the most electron-rich substrates (4a–e). To our delight, some substrates bearing electron withdrawing groups and halogen substituents also gave very satisfactory results (4h, 4i, 4m and 4o). In general, products 3–5 were obtained in good yields and reactions could be scaled up with ease (entries 3a, 3b, 3e, 3p, 4a, 4b and 4e).
Table 1 Scope of C–H arylation of pyrene-1-carboxylic acid (1) and subsequent iododecarboxylation of 2-arylpyrene-1-carboxylic acids 3a
All yields are isolated.
Reaction run at 1.00–3.00 mmol scale; see ESI for further details.
Conditions: 3 (20 mg), Ag2CO3 (1 equiv.), DMSO (0.07 M), 140 °C, 15 h.
|
|
Alternatively, arylation products 3 could be obtained in good yields using aryl bromide electrophiles under ruthenium catalysis (Table 2).21 This system also offered an opportunity to introduce both electron-rich and electron-deficient heteroaromatic substituents in the pyrene ring and obtain heteroaryl-substituted pyrene carboxylic acids in moderate to good yields, while the same heteroaryl electrophiles failed to engage in both the Pd catalyzed decarboxylative and non-decarboxylative cross-coupling reactions.
Table 2 Use of aryl bromides and heteroarylation of pyrene-1-carboxylic acid
NMR yield determined using nitromethane as internal standard.
Isolated yield.
|
|
Arylated iodopyrenes 4 offer a platform for further derivatisation using well stablished cross-coupling reactions (Table 3). This was first validated with the preparation of a series of alkynylated derivatives 6 using primary aryl alkynes and trimethylsilylacetylene in Sonogashira couplings,18 which gave the desired products in good yields with both trimethylacetylene and a range of arylalkynes. Alkynylated derivatives have been previously used in the design and synthesis of extended polyaromatic hydrocarbons, such as graphene nanoribbons.22 Alternatively, 1,2-diarylated pyrenes 7 were obtained in good yields after coupling the same iodopyrenes 4 with arylboronic acids using a Suzuki coupling. Furthermore, these products can be useful in the synthesis of even more substituted pyrene compounds. For instance, C–H borylation was used to obtain bisarylated pyreneboronic ester 8 in good yield and exquisite regioselectivity, offering a route to 1,2,7-trisubstituted pyrenes. Pyrene-2-boronic esters have previously been reported to be versatile intermediates for extensive further manipulation.10b
Table 3 Derivatisation of arylated iodopyrenesa
All yields are isolated.
K2CO3 replaced by Na2CO3 (7.2 equiv.).
EtOH added as co-solvent.
|
|
Next, we embarked in the derivatisation of carboxylic acids 3 to obtain positively charged pyrene derivatives that can be used in the production of sable water-based graphene suspensions (Table 4). To that end, carboxylic acids 3b, 3p and 3k were reduced to benzylic alcohols 9, brominated and subsequently transformed into the corresponding trimethylammonium bromide salts 10a, 10b and 10d, respectively. Alternatively, 3p was fully reduced into the corresponding diol after reaction of both the carboxylic acid and ester groups, allowing the formation of the corresponding dicationic salt 10c.18 For comparison, non-arylated pyrene cation 11 was also prepared and used as control.18
Table 4 Synthesis of arylated pyrene cationsa
All yields are isolated.
In this case, the carboxylic acid was selectively reduced in a two-step procedure: (a) SOCl2, 70 °C; (b) NaBH4, THF, 50 °C.
|
|
It is very interesting to assess the exfoliation efficiency of the set of aryl-substituted pyrenes 10a–c and compare them with unsubstituted pyrene cation 11. Pyrene derivatives have been widely used as exfoliating agents; however, the underlying nature of the interaction of the pyrene derivatives with graphene sheets during exfoliation is not completely understood. Thus, it is important to gain more insight on how to design a pyrene-based exfoliating agent that would allow the highest exfoliation efficiency, both in terms of concentration and relative percentage of single-layer graphene in the dispersion.
Pyrene cations 10a–c and 11 were then tested for their ability to exfoliate graphite in water to produce stable aqueous graphene dispersions. Higher concentrations of graphene were obtained when using arylated pyrene cations 10, according to UV-Vis spectroscopy analysis, as opposed to non-arylated analogue 11 (Table 5).18 Compounds 10a and 10c gave very similar concentrations of exfoliated graphene despite the extra ammonium group in 10c. However, the ester-bearing cation 10b gave twice as concentrated graphene dispersions, presumably thanks to the higher electron deficiency of the ester-substituted pyrene derivative. This trend was further confirmed by 10d, another electron-poor analogue, which gave a concentration of aqueous graphene similar to that of 10b. The concentrations obtained with 10a–d were of the same order of magnitude than the one obtained with negatively charged sodium 1-pyrenesulfonate (PS1, see Fig. 1) under the same exfoliation conditions (110.9 μg mL−1).18PS1 is one of the most commonly used negatively charged organic surfactants for aqueous graphene exfoliation and has found application in different graphene-based technologies.2f,g,6
Table 5 Aqueous liquid phase exfoliation of graphite with pyrene cations
Compound |
Graphene concentrationa (μg mL−1) |
Zeta-potential (mV) |
Determined by UV-Vis spectroscopy.
|
10a
|
88.8 |
40.9 |
10b
|
170.3 |
37.0 |
10c
|
79.6 |
46.0 |
10d
|
143.3 |
39.0 |
11
|
18.1 |
40.5 |
Aqueous suspensions of exfoliated graphene were diluted (×10) for visual inspection. The pictures in Fig. 2 clearly illustrate the stark difference in graphene concentration between suspensions prepared with arylated pyrene cations 10a–d and non-arylated pyrene cation 11. As expected, the more concentrated suspensions, prepared with electron-poor compounds 10b and 10d, were darker than the rest.
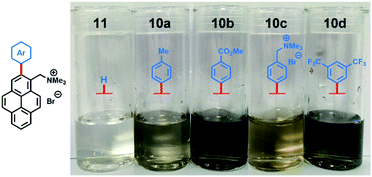 |
| Fig. 2 Pictures of diluted (×10) aqueous dispersions of exfoliated graphene with pyrene cations 10a–d and 11. | |
A plausible explanation for the results obtained with 2-arylated pyrene cations 10 is that steric clashes result in the benzylic ammonium group to be oriented perpendicular to the plane of the pyrene ring, locking the molecule in a favourable conformation (Fig. 3A). Due to the increased sterics, there is restricted rotation around the C sp2–C sp3 bond leading to the emergence of axial chirality. This hypothesis is supported by 1H-NMR analysis, which shows that the benzylic protons in the 2-arylpyrenes 10 are diastereotopic, suggesting that the ammonium group is positioned preferably perpendicular to the pyrene ring plane, thus favouring π-stacking to graphene with one face of the molecule and enhancing interaction with water on the side where the ammonium group points away from the graphene flake (Fig. 3B). This is in sharp contrast with the benzylic protons in non-arylated compound 11, which appear as a singlet in the corresponding 1H-NMR, consistent with free rotation along the C sp2–C sp3 bond. Moreover, complexation of aromatic molecules bearing bi-aryl moieties with graphene has been reported to increase planarity of those molecules, leading to increased π-stacking interactions.23
 |
| Fig. 3 Differences in benzylic protons between pyrene cations in this study. (A) Rotamers along the C sp2–C sp3 bond of arylated (10a–d) and non-arylated (11) pyrene cations. (B) 1H-NMR signals of benzylic protons in compounds 10a–d and 11 in methanol-d4. The diastereotopic benzylic protons of arylated compounds 10a–d indicate that the ammonium group is locked pointing away off the pyrene ring plane. The second set of benzylic protons of compound 10c appear outside the shown range. See ESI† for full spectra. | |
The exfoliated graphene nanosheets were characterised by atomic force microscopy (AFM) for analysis of lateral size. Fig. 4 shows that most of the nanosheets have a lateral size of few hundreds of nanometers. Due to the adsorbed pyrene derivatives, the number of layers of nanosheets cannot be reliably estimated from AFM. Thus, we performed Raman analysis using a protocol that allows to qualitatively determining the number of single-layers, few-layers (less than 10 layers) and residual graphite (>10 layers) by analyzing the symmetry of the Raman 2D peak of several flakes.24 The Raman results showed that all the graphene dispersions prepared in this study consist of mostly single-layer and few-layer graphene nanosheets, showing effective exfoliation of graphite using pyrene derivatives.18 The stability of the exfoliated graphene aqueous dispersion was investigated by zeta-potential measurements, which are typically used as a measure of stability of colloidal suspensions. All graphene dispersions showed a zeta-potential absolute value higher than 30 mV (Table 5), confirming electrostatic stabilization. This was also supported by visual inspection as no noticeable sedimentation was observed over several months of storage at room temperature.
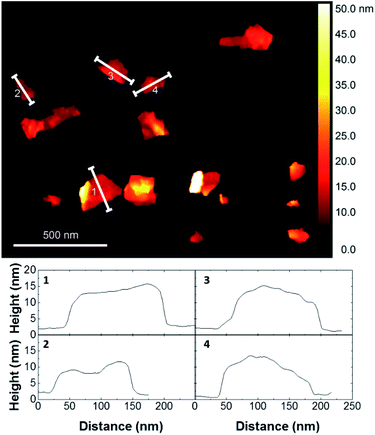 |
| Fig. 4 Representative AFM image of graphene flakes prepared with pyrene cation 10b and cross-section analyses of several flakes. See ESI† for AFM images of graphene flakes exfoliated with all pyrene cations. | |
Conclusions
In conclusion, we have developed a versatile strategy for the synthesis of 2-arylated pyrenes, including 1,2-disubstituted derivatives with several functionalities easily introduced in position 1, such as carboxylic acid, iodide, alkynyl, aryl and alkyl (benzylic alcohols, bromides and ammonium salts). This approach also offers a one-step alternative to the synthesis of 2-aryl pyrenes and opens new possibilities for the design and synthesis of unprecedented functional pyrene-based materials, previously unexplored due to its synthetic inaccessibility. Importantly, the cationic arylated pyrene ammonium salts accessible by this method can be used to obtain stable aqueous graphene suspensions by liquid phase exfoliation of graphite, composed mostly of single- and few-layer graphene flakes, and of concentrations comparable to those achieved with state-of-the-art anionic pyrene exfoliators.
Conflicts of interest
The authors declare no conflict of interest.
Acknowledgements
We would like to thank the EPSRC for funding the 2D Health pro-ject (EP/P00119X/1). MZ acknowledges the Hewlett-Packard Company for financial support in the framework of the Graphene NowNano Doctoral Training Center. VN acknowledges NPL London for financial support in the framework of the Graphene NowNano Doctoral Training Center.
Notes and references
- For selected publications on graphene and graphene-based materials, see:
(a) K. S. Novoselov, A. K. Geim, S. V. Morozov, D. Jiang, Y. Zhang, S. V. Dubonos, I. V. Grigorieva and A. A. Firsov, Science, 2004, 306, 666 CrossRef CAS PubMed;
(b) J. Wu, W. Pisula and K. Müllen, Chem. Rev., 2007, 107, 718 CrossRef CAS PubMed;
(c) M. J. Allen, V. C. Tung and R. B. Kaner, Chem. Rev., 2010, 110, 132 CrossRef CAS PubMed;
(d) K. S. Novoselov, V. I. Fal'ko, L. Colombo, P. R. Gellert, M. G. Schwab and K. Kim, Nature, 2012, 490, 192 CrossRef CAS PubMed;
(e) K. Kostarelos and K. S. Novoselov, Nat. Nanotechnol., 2014, 9, 744 CrossRef CAS PubMed;
(f) G. Liu, W. Jin and N. Xu, Chem. Soc. Rev., 2015, 44, 5016 RSC;
(g) M. F. El-Kady, Y. Shao and R. B. Kaner, Nat. Rev. Mater., 2016, 1, 16033 CrossRef CAS;
(h) X. Yu, H. Cheng, M. Zhang, Y. Zhao, L. Qu and G. Shi, Nat. Rev., 2017, 2, 17046 CAS;
(i) G. Bottari, M. A. Herranz, L. Wibmer, M. Volland, L. Rodríguez-Pérez, D. M. Guldi, A. Hirsch, N. Martín, F. D'Souza and T. Torres, Chem. Soc. Rev., 2017, 46, 4464 RSC;
(j) K. Kostarelos, M. Vincent, C. Hebert and J. A. Garrido, Adv. Mater., 2017, 29, 1700909 CrossRef PubMed.
- For selected papers on liquid-phase exfoliation of graphene, see:
(a) Y. Hernandez, V. Nicolosi, M. Lotya, F. M. Blighe, Z. Sun, S. De, I. T. McGovern, B. Holland, M. Byrne, Y. K. Gun'ko, J. J. Boland, P. Niraj, G. Duesberg, S. Krishnamurthy, R. Goodhue, J. Hutchison, V. Scardaci, A. C. Ferrari and J. N. Coleman, Nat. Nanotechnol., 2008, 3, 563 CrossRef CAS PubMed;
(b) Y. Xu, G. Lu, C. Li and G. Shi, J. Am. Chem. Soc., 2008, 130, 5856 CrossRef CAS PubMed;
(c) M. Loyta, Y. Hernandez, P. J. King, R. J. Smith, V. Nicolosi, L. S. Karlsson, F. M. Blighe, S. De, Z. Wang, I. T. McGovern, G. S. Duesberg and J. N. Coleman, J. Am. Chem. Soc., 2009, 131, 3611 CrossRef PubMed;
(d) Q. Su, S. Pang, V. Alijani, C. Li, X. Feng and K. Müllen, Adv. Mater., 2009, 21, 3191 CrossRef CAS;
(e) D. Parviz, S. Das, H. S. T. Ahmed, F. Irin, S. Bhattacharia and M. J. Green, ACS Nano, 2012, 10, 8857 CrossRef PubMed;
(f) H. Yang, Y. Hernandez, A. Schlierf, A. Felten, A. Eckmann, S. Johal, P. Louette, J.-J. Pireaux, X. Feng, K. Müellen, V. Palermo and C. Casiraghi, Carbon, 2013, 53, 357 CrossRef CAS;
(g) A. Viinikanoja, J. Kauppila, P. Damlin, E. Mäkilä, J. Leiro, T. Ääritalo and J. Lukkari, Carbon, 2014, 68, 195 CrossRef CAS;
(h) J. Cho, I. Jeon, S. Y. Kim, S. Lim and J. Y. Jho, ACS Appl. Mater. Interfaces, 2017, 9, 27984 CrossRef CAS PubMed;
(i) A. Ciesielski and P. Samorì, Adv. Mater., 2016, 28, 6030 CrossRef CAS PubMed.
- For reviews on non-covalent functionalisation of graphene, see:
(a) V. Georgakilas, M. Otyepka, A. B. Bourlinos, V. Chandra, N. Kim, K. C. Kemp, P. Hobza, R. Zboril and K. S. Kim, Chem. Rev., 2012, 112, 6156 CrossRef CAS PubMed;
(b) V. Georgakilas, J. N. Tiwari, K. C. Kemp, J. A. Perman, A. B. Bourlinos, K. S. Kim and R. Zboril, Chem. Rev., 2016, 116, 5464 CrossRef CAS PubMed.
-
(a) H. Jaegfeldt, T. Kuwana and G. Johansson, J. Am. Chem. Soc., 1983, 105, 1805 CrossRef CAS;
(b) Y. H. Zhang, C. J. Liu, W. Q. Shi, Z. Q. Wang, L. M. Dai and X. Zhang, Langmuir, 2007, 23, 7911 CrossRef CAS PubMed.
- For selected references on the development of graphene-based biosensors using pyrene adducts, see:
(a) J. A. Mann, J. Rodríguez-López, H. D. Abruña and W. R. Dichtel, J. Am. Chem. Soc., 2011, 133, 17614 CrossRef CAS PubMed;
(b) T. Alava, J. A. Mann, C. Théodore, J. J. Benitez, W. R. Dichtel, J. M. Parpia and H. G. Craighed, Anal. Chem., 2013, 85, 2754 CrossRef CAS PubMed;
(c) M. Singh, M. Holzinger, M. Tabrizian, S. Winters, N. C. Berner, S. Cosnier and G. S. Duesberg, J. Am. Chem. Soc., 2015, 137, 2800 CrossRef CAS PubMed;
(d) S. Osella, M. Kiliszek, E. Harputlu, C. G. Unlu, K. Ocakoglu, J. Kargul and B. Trzaskowski, J. Mater. Chem. C, 2018, 6, 5046 RSC.
- For selected references on the production of graphene inks, see:
(a) A. Schlierf, H. Yang, E. Gebremedhn, E. Treossi, L. Ortolani, L. Chen, A. Minoia, V. Morandi, P. Samorì, C. Casiraghi, D. Beljonne and V. Palermo, Nanoscale, 2013, 5, 4205 RSC;
(b) D. McManus, S. Vranic, F. Withers, V. Sanchez-Romaguera, M. Macucci, H. Yang, R. Sorrentino, K. Parvez, S.-K. Son, G. Iannaccone, K. Kostarelos, G. Fiori and C. Casiraghi, Nat. Nanotechnol., 2017, 12, 343 CrossRef CAS PubMed.
- For selected publications on graphene-pyrene-based composite materials, see:
(a) D.-W. Lee, T. Kim and M. Lee, Chem. Commun., 2011, 47, 8259 RSC;
(b) J. Zhang, Y. Xu, L. Cui, A. Fu, W. Yang, C. Barrow and J. Liu, Composites, Part A, 2015, 71, 1 CrossRef.
- For selected publications on graphene-pyrene-based light harvesting materials, see:
(a) A. Roth, M.-E. Ragoussi, L. Wibmer, G. Katsukis, G. de la Torre, T. Torres and D. M. Guldi, Chem. Sci., 2014, 5, 3432 RSC;
(b) C. B. Kc, G. N. Lim and F. D'Souza, Angew. Chem., Int. Ed., 2015, 54, 5088 CrossRef CAS PubMed;
(c) M. Kiliszek, E. Harputlu, M. Szalkowski, D. Kowalska, C. G. Unlu, P. Haniewicz, M. Abram, K. Wiwatowski, J. Niedziółka-Jönsson, S. Maćkowski, K. Ocakoglu and J. Kargul, J. Mater. Chem. A, 2018, 6, 18615 RSC.
- For reviews on the synthesis of pyrene derivatives, see:
(a) T. M. Figueira-Duarte and K. Müllen, Chem. Rev., 2011, 111, 7260 CrossRef CAS PubMed;
(b) J. M. Casas-Solvas, J. D. Howgego and A. P. Davis, Org. Biomol. Chem., 2014, 12, 212 RSC;
(c) X. Feng, J.-Y. Hu, C. Redshaw and T. Yamato, Chem.–Eur. J., 2016, 22, 11898 CrossRef CAS PubMed.
-
(a) D. N. Coventry, A. S. Batsanov, A. E. Goeta, J. A. K. Howard, T. B. Marder and R. N. Perutz, Chem. Commun., 2005, 2172 RSC;
(b) A. G. Crawford, Z. Liu, I. A. I. Mkhalid, M.-H. Thibault, N. Schwarz, G. Alcaraz, A. Steffen, J. C. Collings, A. S. Batsanov, J. A. K. Howard and T. B. Marder, Chem.–Eur. J., 2012, 18, 5022 CrossRef CAS PubMed.
- For references on the C–H arylation of pyrene derivatives, see:
(a) K. Mochida, K. Kawasumi, Y. Segawa and K. Itami, J. Am. Chem. Soc., 2011, 133, 10716 CrossRef CAS PubMed;
(b) L. Ilies, E. Konno, Q. Chen and E. Nakamura, Asian J. Org. Chem., 2012, 1, 142 CrossRef CAS;
(c) Y.-Q. He and Y.-W. Zhong, Chem. Commun., 2015, 51, 3411 RSC;
(d) S. Rajkumar, S. Karthik and T. Gandhi, J. Org. Chem., 2015, 80, 5532 CrossRef CAS PubMed;
(e) K. D. Collins, R. Honeker, S. Vásquez-Céspedes, D.-T. D. Tang and F. Glorious, Chem. Sci., 2015, 6, 1816 RSC.
- For references on the C–H alkynylation of pyrene derivatives, see:
(a) G. Jiang, W. Hu, J. Li, C. Zhu, W. Wu and H. Jiang, Chem. Commun., 2018, 54, 1746 RSC;
(b) G. Liao, Q.-J. Yao, Z.-Z. Zhang, Y.-J. Wu, D.-Y. Huang and B.-F. Shi, Angew. Chem., Int. Ed., 2018, 57, 3661 CrossRef CAS PubMed;
(c) E. Tan, O. Quinonero, M. E. de Orbe and A. M. Echevarren, ACS Catal., 2018, 8, 2166 CrossRef CAS PubMed.
- For the directed C–H alkenylation of a pyrene derivatives, see: J. Zheng and S.-L. You, Angew. Chem., Int. Ed., 2014, 53, 13244 CrossRef CAS PubMed.
- For the directed C–H borylation of a pyrene derivative, see: A. Ros, B. Estepa, R. López-Rodríguez, E. Álvarez, R. Fernández and J. M. Lassaletta, Angew. Chem., Int. Ed., 2011, 50, 11724 CrossRef CAS PubMed.
- For reviews on the arylation of aromatic carboxylic acids, see:
(a) J. Cornella and I. Larrosa, Synthesis, 2012, 653 CAS;
(b) M. P. Drapeau and L. J. Gooßen, Chem.–Eur. J., 2016, 22, 18654 CrossRef PubMed;
(c) M. Font, J. Quibell, G. J. P. Perry and I. Larrosa, Chem. Commun., 2017, 53, 5584 RSC;
(d) G. J. Perry and I. Larrosa, Eur. J. Org. Chem., 2017, 25, 3517 CrossRef PubMed.
- For references on decarboxylative ortho-arylation of carboxylic acids, see:
(a) J. Cornella, M. Righi and I. Larrosa, Angew. Chem., Int. Ed., 2011, 50, 9429 CrossRef CAS PubMed;
(b) J. Luo, S. Preciado and I. Larrosa, J. Am. Chem. Soc., 2014, 136, 4109 CrossRef CAS PubMed;
(c) J. Luo, S. Preciado and I. Larrosa, Chem. Commun., 2015, 51, 3127 RSC;
(d) Y. Zhang, H. Zhao, M. Zhang and W. Su, Angew. Chem., Int. Ed., 2015, 54, 3817 CrossRef CAS PubMed;
(e) M. Font, A. R. A. Spencer and I. Larrosa, Chem. Sci., 2018, 9, 7133 RSC.
- For references on the palladium-catalysed non-decarboxylative ortho-arylation of carboxylic acids, see:
(a) H. A. Chiong, Q.-N. Pham and O. Daugulis, J. Am. Chem. Soc., 2007, 129, 9879 CrossRef CAS PubMed;
(b) C. Arroniz, A. Ironmonger, G. Rassias and I. Larrosa, Org. Lett., 2013, 15, 910 CrossRef CAS PubMed;
(c) C. Arroniz, J. G. Denis, A. Ironmonger, G. Rassias and I. Larrosa, Chem. Sci., 2014, 5, 3509 RSC;
(d) C. Zhu, Y. Zhang, J. Kan, H. Zhao and W. Su, Org. Lett., 2015, 17, 3418 CrossRef CAS PubMed.
- See the ESI† for further details.
- J. Cornella, C. Sanchez, D. Banawa and I. Larrosa, Chem. Commun., 2009, 7176 RSC.
- G. J. P. Perry, J. M. Quibell, A. Panigrahi and I. Larrosa, J. Am. Chem. Soc., 2017, 139, 11527 CrossRef CAS PubMed.
- L. Huang and D. J. Weix, Org. Lett., 2016, 18, 5432 CrossRef CAS PubMed.
- For examples of the synthesis of polyaromatic hydrocarbons using alkynylated precursors, see:
(a) M. B. Goldfinger and T. M. Swager, J. Am. Chem. Soc., 1994, 116, 7895 CrossRef CAS;
(b) M. B. Goldfinger, K. B. Crawford and T. M. Swager, J. Am. Chem. Soc., 1997, 119, 4578 CrossRef CAS;
(c) R. K. Mohamed, S. Mondal, B. Gold, C. J. Evoniuk, T. Banerjee, K. Hanson and I. V. Alabugin, J. Am. Chem. Soc., 2015, 137, 6335 CrossRef CAS PubMed;
(d) W. Yang, J. H. S. K. Monteiro, A. de Bettercourt-Dias, V. J. Catalano and W. A. Chalifoux, Angew. Chem., Int. Ed., 2016, 55, 10427 CrossRef CAS;
(e) W. Yang, A. Lucotti, M. Tommasini and W. A. Chalifoux, J. Am. Chem. Soc., 2016, 138, 9137 CrossRef CAS PubMed;
(f) I. V. Alabugin and E. Gonzalez-Rodriguez, Acc. Chem. Res., 2018, 51, 1206 CrossRef CAS PubMed.
- Y. Xu, L. Zhao, H. Bai, W. Hong, C. Li and G. Shi, J. Am. Chem. Soc., 2009, 131, 13490 CrossRef CAS PubMed.
- For papers on the analysis of exfoliated graphene flakes, see:
(a) A. Ciesielski, S. Haar, M. El Gemayel, H. Yang, J. Clough, G. Melinte, M. Gobbi, E. Orgiu, M. V. Nardi, G. Ligorio, V. Palermo, N. Koch, O. Ersen, C. Casiraghi and P. Samorì, Angew. Chem., Int. Ed., 2014, 53, 10355 CrossRef CAS;
(b) A. Haar, A. Ciesielski, J. Clough, H. Yang, R. Mazzaro, F. Richard, S. Conti, N. Merstorf, M. Cecchini, V. Morandi, C. Casiraghi and P. Samorì, Small, 2015, 11, 1691 CrossRef PubMed;
(c) S. Haar, M. El Gemayel, Y. Shin, G. Melinte, M. A. Squillaci, O. Ersen, C. Casiraghi, A. Ciesielski and P. Samorì, Sci. Rep., 2015, 5, 16684 CrossRef CAS PubMed;
(d) S. Conti, M. G. del Rosso, A. Ciesielski, J. Weippert, A. Böttcher, Y. Shin, G. Melinte, O. Ersen, C. Casiraghi, X. Feng, K. Müllen, M. M. Kappes, P. Samorì and M. Cecchini, ChemPhysChem, 2016, 17, 352 CrossRef CAS PubMed;
(e) Y. Shin, E. Prestat, K.-G. Zhou, P. Gorgojo, K. Althumayri, W. Harrison, P. M. Budd, S. J. Haigh and C. Casiraghi, Carbon, 2016, 102, 357 CrossRef CAS.
Footnote |
† Electronic supplementary information (ESI) available. See DOI: 10.1039/c9sc05101e |
|
This journal is © The Royal Society of Chemistry 2020 |
Click here to see how this site uses Cookies. View our privacy policy here.