DOI:
10.1039/D0SC01249A
(Edge Article)
Chem. Sci., 2020,
11, 9209-9217
A synthetic glycan array containing Cryptococcus neoformans glucuronoxylomannan capsular polysaccharide fragments allows the mapping of protective epitopes†
Received
28th February 2020
, Accepted 29th July 2020
First published on 7th August 2020
Abstract
A convergent synthetic strategy to Cryptococcus neoformans glucuronoxylomannan (GXM) capsular polysaccharide part structures was developed based on di-, tri-, tetra-, penta- and hexasaccharide thioglycoside building blocks. The approach permitted the synthesis of a library of spacer-containing serotype A and D related GXM oligosaccharide structures, ranging from di- to octadecasaccharides. Ten deprotected GXM compounds (mono- to decasaccharide) were printed onto microarray plates and screened with seventeen mouse monoclonal antibodies (mAbs) to GXM. For the first time a GXM oligosaccharide structure (a serotype A decasaccharide), capable of being recognized by neutralizing forms of these GXM-specific mAbs, has been identified, offering insight into the binding epitopes of a range of protective monoclonal antibodies and furthering our efforts to develop semi-synthetic conjugate vaccine candidates against C. neoformans.
Introduction
Cryptococcus neoformans is an environmental yeast that can cause systemic infection in the immunocompromised and it is estimated that 1 million infections occur annually, leading to 600
000 deaths.1 Goldman et al. hypothesised that infection occurs in early childhood, going unrecognised, and infection is cleared or enters a state of latency.2 However, if the infection remerges, it is a major health risk for those undergoing solid organ transplant, chemotherapy and HIV/AIDS patients.1,3,4 Therapies currently used to treat this fungal infection are witnessing rising resistance,5 and therefore research into new therapeutics to fight cryptococcal infections is required.6
C. neoformans is unique among pathogenic fungi in that its polysaccharide capsule is essential for virulence in mammals.7 The capsule is composed of several constituents, including mannoproteins, β-glucans, galactoxylomannan (GalXM) and glucuronoxylomannan (GXM). The latter, which accounts for approximately 90–95% of the capsule mass, is released into the tissue during infection,2 causing a myriad of effects in the host, including interference with phagocytosis and leukocyte migration.8,9 The GXM is a heteropolymer and does not consist of repeating units as in bacterial polysaccharides, but rather contains 6 repeating motifs, 4 of which can be used to serotype C. neoformans (Fig. 1). However, these motifs occur in various ratios depending on both strain and batch of the microbe. The GXM structure was analysed by Cherniak and co-workers using nuclear magnetic resonance (NMR) spectroscopy.10–12 They concluded that this polysaccharide consisted of a α-(1 →3)-mannose backbone containing β-(1 → 2) and β-(1 → 4) xylose branches and a β-(1 → 2) glucuronic acid branch every three mannoses.10–12 The GXM is further functionalized by a heterogeneous 6-O-acetyl substitution pattern along the mannan backbone.13,14 The acetylation is known to be important for the antibody–antigen interactions and virulence with an estimated average of 2 acetates per mannose triad (serotype A and D).14,15 This heterogeneity of the GXM severely complicates its structural analysis as well as structure–activity investigations, e.g., its use in conjugate vaccines, where the stated heterogeneity has shown to lead to irreproducible effects in vaccination in murine models.16,17 Also, reducing ends are not obviously available in the GXM CPS,18 why CPS-based conjugate vaccines candidates have been constructed using methods, e.g., cyanogen bromide activation, resulting in complex cross-linked conjugates.
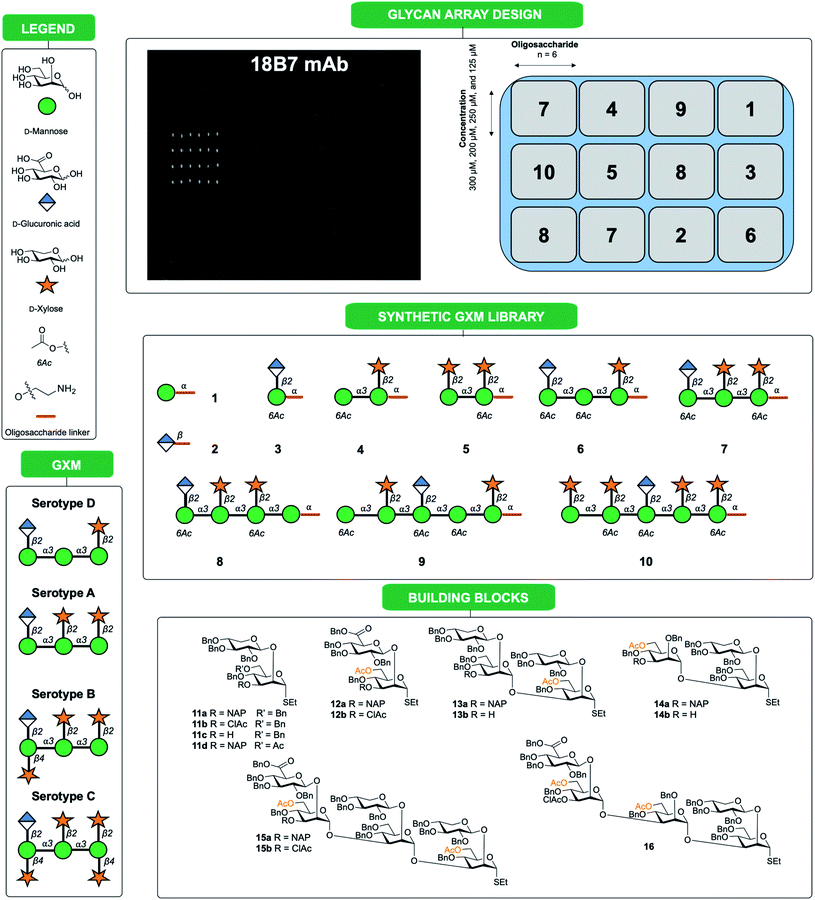 |
| Fig. 1 Synthetic oligosaccharides used to create a library of GXM structures. Glycans are depicted according to symbol nomenclature for glycans (SNFG).43 | |
Hence, access to well-defined synthetic spacer-equipped GXM glycans is almost certainly a requirement to map binding specificities of monoclonal antibodies (mAbs). This is essential to reveal protective epitopes that would enable selection of lead candidates in efforts to develop semi-synthetic vaccine candidates against C. neoformans infections, noting our previously attempt using a synthetic heptasaccharide conjugated to human serum album (HSA), which was found to elicit a non-protective immune response in mice.19,20 Since so far none of the smaller structures synthesized (up to heptasaccharide) have been recognized by any of the protective (neutralizing) mAbs available, we hypothesized that larger structures, able of presenting conformational epitopes, were required.
In this work we present a convergent synthetic strategy (Fig. 1) and synthesis of a library of GXM oligosaccharide structures, up to an octadecasaccharide. The GXM fragments are based on serotype A and D motifs (Fig. 1), as serotype A and D are responsible for the vast majority of human infections globally.21 The synthetic strategy allows for target structures to contain acetyl groups, which are known to be important for virulence.14 The acetylation pattern chosen was based on tentative NMR analysis suggesting a partly different acetylation arrangement between serotype A and D. A selection of these synthetic structures was used to create a first GXM glycan array containing 10 structures (Fig. 1), which was then screened with 17 anti-GXM mAbs, both protective and non-protective, to investigate their binding specificities and to seek lead candidates for vaccine development.
Results and discussion
The synthesis is a continuation of our earlier work on serotype A and D GXM structures, based mainly on disaccharide building blocks 11 (Xyl-β-(1 → 2)-Man) and 12 (GlcA-β-(1 → 2)-Man), which are both ethyl thiosaccharides orthogonally protected in the 3-position (Fig. 1).22–27 To permit the presence of acetates in target structures, benzyl groups were used as permanent protecting groups and initially allyl ethers and subsequently 2-naphthylmethyl (NAP) ethers were employed as temporary protecting groups.26,27 These strategically designed disaccharide building blocks have been effectively used for the synthesis of structures up to a heptasaccharide in size, with all glycosylation products obtained with total 1,2-trans selectivity.19
Thioglycoside block synthesis
To facilitate the synthesis of extended structures, larger building blocks were sought. As reported previously, a serotype A tetrasaccharide (13) was synthesized from two Xyl-β-(1 → 2)-Man building blocks (11a + 11d) by converting one of the thiosaccharides to a trichloroacetimidate donor and one to a 3-OH acceptor, and then performing an orthogonal glycosylation.24 Very high glycosylation yields were obtained using a reversed glycosylation procedure, i.e., first mixing the acceptor and the promoter, and then adding the donor dropwise. The same approach was used to effectively obtain a serotype D trisaccharide (14). For continued synthesis of larger thioglycoside building blocks the NAP groups in blocks 13a and 14a were removed to obtain the 3-OH acceptors 13b (68%) and 14b (72%), respectively.24 As noted by other researchers the chemoselectivity was not complete and benzyl groups were simultaneously cleaved, thus, lowering the yield.28 This prompted us to investigate alternative orthogonal 3-O-protecting groups, since we anticipated that this problem may become even more pronounced on larger structures containing an even higher number of benzyl groups. A monochloroacetyl (AcCl) group was selected, the NAP group of disaccharide 12a was cleaved and subsequently the 3-OH was acylated with monochloroacetyl chloride to give thioglycoside 12b. This structure was then converted to its corresponding trichloroacetimidate donor 17b for orthogonal glycosylation optimization (Scheme 1).
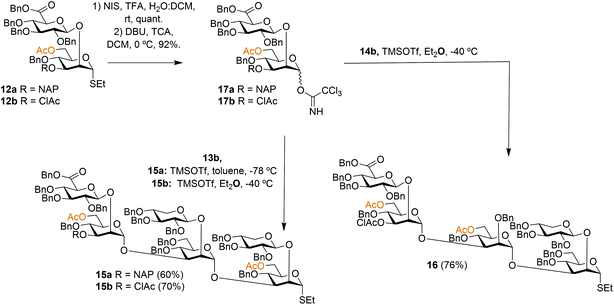 |
| Scheme 1 Synthesis of hexa- and pentasaccharide thioglycoside building blocks. | |
To afford a serotype A triad donor block, glycosylations with donor 17a and acceptor 13b were attempted, using the optimized conditions developed for the synthesis of 13a, i.e., an inverse glycosylation in toluene with TBDMSOTf as a promoter. However, this gave a complex mixture from which the desired product 15a could only be isolated in low yields (15–29%). Changing the promoter of the glycosylation to TMSOTf allowed the synthesis of 15a in a 60% yield. Glycosylations with the 3-O-ClAc protected donor 17b and 13b using the same promoter (TMSOTf) gave even higher yields of hexasaccharide 15b, in the range of 70%. For this reason, donor 17b was selected for the synthesis of the serotype D triad. Glycosylations of trisaccharide acceptor 14b with donor 17b afforded pentathiosaccharide 16 in 76% yield.
Spacer-equipped acceptor block synthesis
Though the synthesis of the larger thiosaccharide building blocks 15 and 16 was successful, it was considered more efficient, especially on a larger scale, to synthesise the corresponding spacer-equipped reducing end structures using a stepwise approach, rather than through a direct glycosylation of blocks 15 and 16 with a spacer. Thus, spacer-equipped disaccharide acceptors 18a and 18b were prepared in a similar manner to that described for the corresponding thioethyl disaccharides 11 (see ESI†). Given the chemoselectivity issues experienced when cleaving NAP groups, it was decided to also here investigate a chloroacetyl as a temporary protecting group. Therefore, the NAP group of 11a was converted to a chloroacetyl group (→11b, 2 steps, 54%). DMTST-promoted glycosylations29 of 18a with either donor 11a or 11b gave tetrasaccharides 19a and 19b in very high yields of 89% and 95%, respectively and total 1,2-trans selectivity (Scheme 2A). Removal of the NAP group from 19a yielded acceptor 19c in a 64–80% yield, while removal of the chloroacetate gave 19c in a 95% yield. Acceptor 19c was then glycosylated with either NAP-protected donor 12a or ClAc-protected donor 12b to afford hexasaccharides 20a (79%) and 20b (74%), respectively. As anticipated, removal of the NAP group was significantly lower yielding for this larger structure, affording acceptor 20c in only a 43% yield. In contrast, removal of the ClAc group produced 20c in a 90% yield, highlighting the benefit of employing the chloroacetate over the NAP group, especially for the synthesis of structurally larger acceptors.
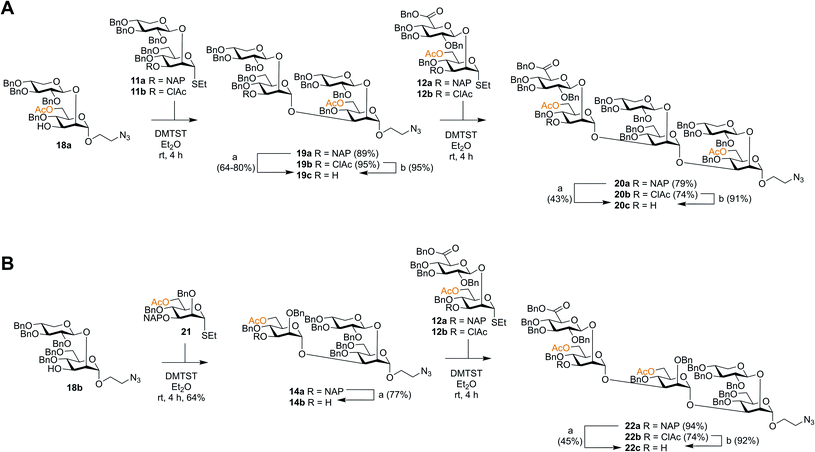 |
| Scheme 2 Synthesis of spacer-equipped GXM oligosaccharides. (A) Serotype A hexasaccharide acceptor (B) serotype D pentasaccharide acceptor. Reagents and conditions: (a) DDQ, DCM : H2O, rt; (b) thiourea, NaHCO3, TBAI, dry. THF, 65 °C. | |
Serotype D structures were synthesised by coupling disaccharide acceptor 18b with monosaccharide donor 21 using DMTST as promoter which gave an α/β-mixture (3
:
1) from which the pure α-anomer 14a could be isolated in a 64% yield (Scheme 2B). We have earlier encountered the formation of anomeric mixtures in our GXM structure syntheses, but only in glycosylations employing 2-O-benzyl protected (monosaccharide) donors,24 all donors possessing a 2-O-glycosidic moiety have displayed complete α-selectivity. In this case, the anomers were quite easily separated and, for this reason, no major efforts were performed to improve the stereoselectivity of the glycosylation. Removal of the NAP group afforded trisaccharide acceptor 14b (77%), which was then glycosylated with thioglycoside donor 12a to give pentasaccharide 22a in a 94% yield. Again, removal of the NAP group from 22a to afford acceptor 22c was quite low yielding (45%). Consequently, donor 12b was utilized to give pentasaccharide 22b in a lower glycosylation yield of 74%, but a higher deprotection yield of 92% to form acceptor 22c. This improved the overall yield of the two-step process from 42% (NAP) to 68% (ClAc).
Block assembly
With this arsenal of building blocks available, their assembly was then attempted (Schemes 3 and 4). Although problems had been encountered in the formation of some of the building blocks (especially 12, 13, and 15), their assembly was straightforward. The same glycosylation conditions (DMTST, Et2O, rt) developed for the disaccharide thioglycoside donors proved to be universally effective for all donor–acceptor combinations. In addition, the selective removal of the chloroacetyl group proved to be consistently high yielding, all contributing to an effective synthetic approach to GXM oligosaccharides. Although NAP protected building blocks 11a, 13a, and 14a were still utilised, they were introduced as terminal ends of the larger structures. As such, the NAP group act as a permanent protecting group rather than a temporary one, and thus their low yielding selective removal was not an issue.
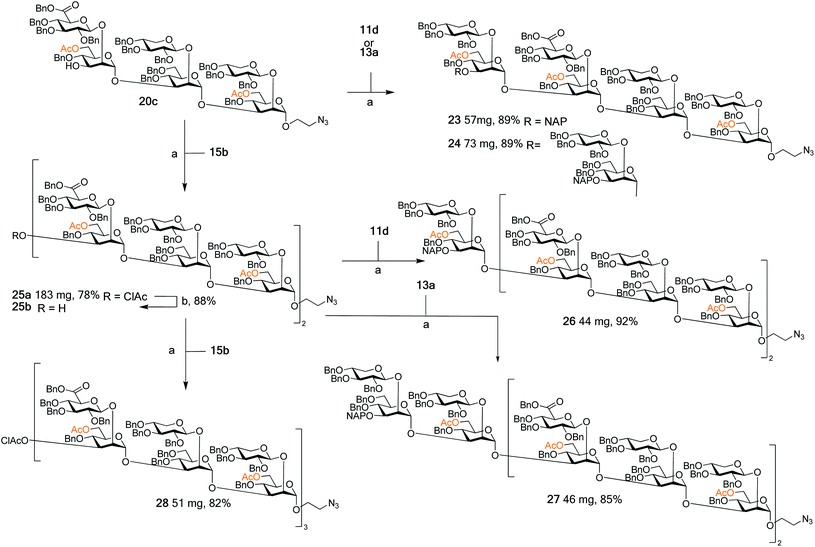 |
| Scheme 3 Assembly of spacer-equipped serotype A structures. Reagents and conditions: (a) DMTST, Et2O, rt; α-only; (b) thiourea, NaHCO3, TBAI, dry. THF, 65 °C. | |
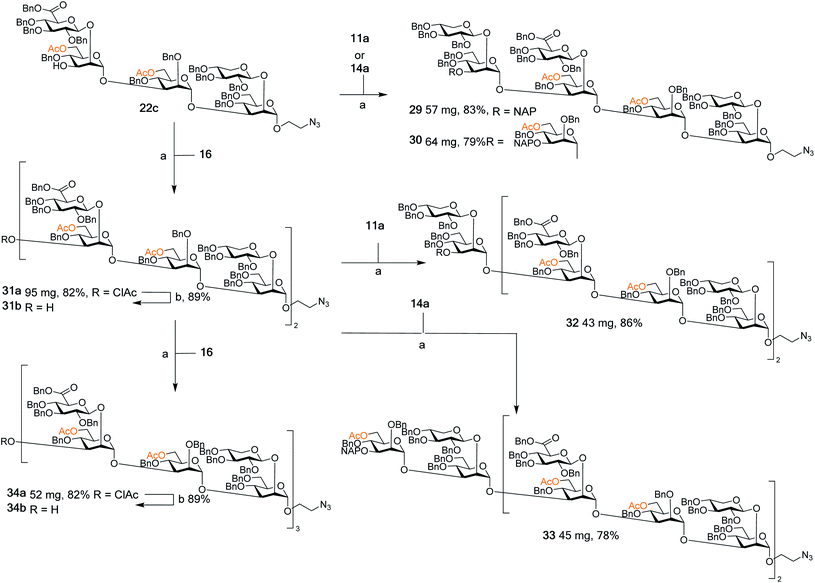 |
| Scheme 4 Assembly of spacer-equipped serotype D fragments. Reagents and conditions (a) DMTST, Et2O, rt, α-only; (b) thiourea, NaHCO3, TBAI, dry. THF, 65 °C. | |
The assembly of serotype A structures is summarized in Scheme 3. Glycosylation yields were reproducibly high (78–92%), and the removal of the chloroacetyl protecting groups was performed in an 88% yield. These factors contributed to an efficient block synthesis of a library of serotype A related structures, e.g., octadecasaccharide 28 was synthesized on a 50 mg scale from building blocks 15b(x2) and 20c in only three steps with an overall yield of 56%. Assembly of the serotype D series is summarized in Scheme 4. Again, the glycosylation yields were consistently high (78–86%), as were the chloroacetyl removals; 89% (→31b) and 89% (→34b). For example, pentadecasaccharide 34a was assembled on a 50 mg scale from the building blocks 16(x2) and 22c in three steps in an overall yield of 60%.
Global deprotection and array printing
Following assembly, the final steps required to attain the target structures involved removing the benzyl ethers and reducing the azide present on the spacer to an amino group, which would be employed to not only immobilise structures on array plates, but also as a means for conjugation to carrier proteins to form glycoconjugate vaccine candidates. A one-step global deprotection, catalytic hydrogenolysis (H2 20–40 bar, Pd/C) to remove benzyl (and NAP) ethers, and concomitantly reduce the azide, was performed. Though saturation side-products of the benzyl and naphthylmethyl protecting groups occurred during hydrogenolysis,30 it was possible to obtain pure target structures following purification via reversed phase chromatography (yields 37–85%). A number of structures ranging in size from mono- to decasaccharides (Fig. 1, compounds 1–10) were selected for preparation of an initial glycan array. Using a previously reported method,31,32 the compounds 1 to 10 were printed at four different concentrations (300 μM, 250 μM, 200 μM and 125 μM) in six replicates (Fig. 1) onto glass slides coated with different types of reactive functional groups; N-hydroxysuccinimide esters (hydrogel slides) or epoxides (epoxy slides). Both of these functionalities being capable of reacting with the terminal amino group of the synthesized C. neoformans fragments and would allow insight into the optimal choice of glass slide for investigating these antigen–antibody interactions. Following printing, the slides were screened with 17 anti-GXM monoclonal antibodies (Fig. 2).
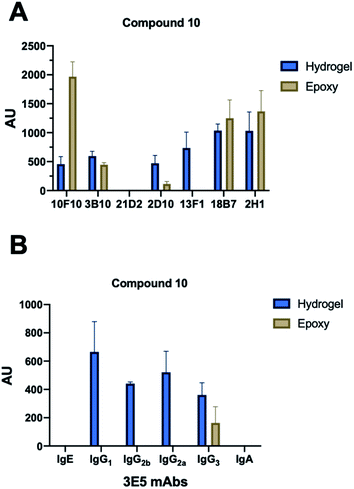 |
| Fig. 2 Array screening. Summary of microarray results from synthetic glycan library. (A) Summary of mAbs that recognize compound 10. All incubations were carried out at 10 μg mL−1 with exceptions indicated in parentheses. 10F10–IgM (100 μg mL−1), 3B10–IgG1, 21D2–IgM (100 μg mL−1), 2D10–IgM, 13F1–IgM, 18B7–IgG1 and 2H1–IgG1. (B) Effect of isotype switch on glycan recognition in 3E5 mAbs. Family contained IgG1, IgG2a, IgG2b, IgG3, IgE and IgA isotypes. Bars represent the mean ±SD. Each antibody experiment was repeated in triplicate. | |
Microarray screening
With each hybridization, a set of matching slides was screened, one epoxy slide and one hydrogel slide so results could be compared between the two surface coatings. A total of 17 different mAbs to GXM were tested, these murine mAbs were generated from B cells harvested from the spleen on mice immunized with a GXM-tetanus toxoid conjugate vaccine,33 while isotype switch variants were obtained through in vitro isotype switching (Fig. 2).34 While four concentrations of each glycan were printed onto the microarrays, no concentration dependence was observed for antibody binding in this range. Most mAbs showed similar compound specificities between the hydrogel and epoxy coatings (Fig. 2A), but the 4H3 family exhibited a preference to the epoxy-coated arrays with perplexingly little to no binding observed on hydrogel slides. The epoxy-coated slides also tended to produce a higher background signal making it more difficult in some arrays to determine binding. Within individual arrays, some mAbs clearly bound more strongly to certain compounds than others.
The microarray binding results provide the first information on the polysaccharide structures recognized by protective mAbs to C. neoformans GXM and give additional information on structures recognized by non-protective mAbs.19,20 mAbs to GXM have been categorized into five groups based on their molecular structure and idiotype reactivity.35 mAbs 2H1, 18B7, 2D10, 3B10, 13F1, 3E5, and 10F10 are Group II mAbs while mAb 4H3 and its isotype switched variants are Group V mAbs. Consistent with the molecular and idiotypic classification, the Group II and V mAbs manifested different reactivity with the oligosaccharide compounds. Compound 10 was bound by protective mAbs 2H1, 18B7, 2D10, and 3B10 when immobilized by both epoxy and hydrogel coatings. However, non-protective mAb 13F1, which is known to bind a different epitope from that recognized by the other Group II protective mAbs,36 bound compound 10 attached by the hydrogel but not epoxy coating. We interpret this result as consistent with mAb 13F1 having different epitope specificity and revealing differences in how the oligosaccharides are recognised depending on the glass slide used. In this regard, the epitopes recognized by some of these mAbs are conformational,37 suggesting a possible explanation for the differences in their reactivity with oligosaccharides depending on the glass surface used. The 3E5 mAb set was derived by in vitro isotype switching from an IgG3 hybridoma and structurally this mAb is classified as Class II. All of the IgG isotypes of 3E5 share the same variable gene sequence but differ in constant region. These exhibited similar binding profiles, binding best to decasaccharide compound 10. However, the 3E5 IgA and IgE isotype variants did not bind to any compounds on the array in contrast to their IgG variants, consistent with epitope recognition differences mediated by constant region effect, which had previously been reported by Janda et al.38
The 4H3 mAb family represents a group of isotype switched antibodies derived from 4H3 IgG3, which is not protective against C. neoformans.33 The 4H3 mAbs bound best to compound 8, a heptasaccharide, which we have previously investigated as a semi-synthetic vaccine candidate, and ultimately produced a non-protective immune response.19,20 Hence, we have the consistent result that this mAb is not protective and binds best to an oligosaccharide that elicited non-protective antibodies. Again, we observed with the 4H3 family of mAbs that isotype switching effected antigen recognition (Fig. 3), as we have reported previously.39 In contrast to the 4H3 mAbs, the IgG1, IgG2a, IgG2b, and IgG3 4H3 variants each demonstrated different reactivity with the oligosaccharide compound set consistent with the notion that isotype switching can affect the specificity of antibodies expressing identical variable region structures (for review40).
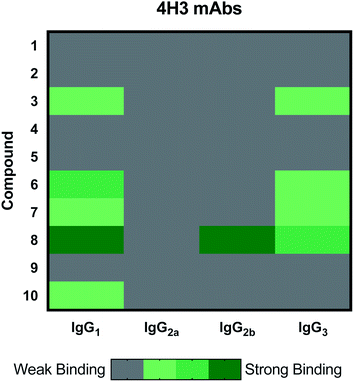 |
| Fig. 3 Heatmap depicting effect of isotype switching on epitope preference of 4H3 mAbs. Family contains IgG1, IgG2a, IgG2b and IgG3. All incubations were carried out at 10 μg mL−1. Each microarray scan was repeated three times. | |
Overall, these results indicated that structurally and functionally different mAbs to C. neoformans GXM bind to different polysaccharide motifs in the capsular polysaccharide. Most of the mAbs to GXM bound best, or only, to 10, a serotype A decasaccharide and this included two mAbs that have been shown to be protective in mice, 2H1 (IgG1) and 18B7 (IgG1).41,42 The finding that all protective mAbs bound to compound 10 immobilized by both the epoxy and hydrogel coatings suggests that it contains an epitope that could elicit useful antibody responses, making it a good candidate for evaluation in vaccination experiments. Further 10 was recently shown to adopt conformations of the native GXM, unlike smaller synthetic fragments.
Conclusions
The native GXM polysaccharide is too heterogeneous to be used in mAb epitope mapping, why synthetic glycans are essential tools in investigating the immunological properties of the C. neoformans capsule. Epitope mapping is important to understand the relationship between mAb binding and capsule reactivity and for the development of these reagents for therapeutic and vaccine use. We here present the design and implementation of an effective convergent synthetic strategy enabling access to an extended library of Cryptococcus neoformans GXM serotype A and D structures. The structures comprise the natural acetylation pattern, known to be of importance for virulence, adding severe challenges to the synthesis. In particular, the synthesis of large thioglycoside building blocks up to a hexasaccharide in size allowed the efficient assembly also of large GXM glycans, up to an octadecasaccharide. Part of this library (up to a decasaccharide) was deprotected and used in printing a first generation GXM glycan microarray, which was screened with a library of GXM mAbs, both non-protective and protective (neutralizing). For the first time ever the binding specificity of neutralizing GXM mAbs could be established. The screening revealed that a serotype A decasaccharide was recognized by several neutralizing mAbs, making this structure a most attractive candidate for continued conjugate vaccine development.
Conflicts of interest
There are no conflicts to declare.
Acknowledgements
We thank Dr Yannick Ortin and Dr Jimmy Muldoon for NMR and MS support. L. G. was supported by Marie Curie European Fellowships (FP7-PEOPLE-2011-IEF, Project Number 299710). C. J. C. was funded by Irish Research Council postgraduate award (GOIPG/2016/998). A. C. was supported in part by NIH grants AI052733 16 and HL059842 19. S. O was supported by Science Foundation Ireland Award 13/IA/1959.
Notes and references
- B. J. Park, K. A. Wannemuehler, B. J. Marston, N. Govender, P. G. Pappas and T. M. Chiller, Estimation of the current global burden of cryptococcal meningitis among persons living with HIV/AIDS, AIDS, 2009, 23, 525–530 CrossRef PubMed.
- D. L. Goldman, H. Khine, J. Abadi, D. J. Lindenberg, La Pirofski, R. Niang and A. Casadevall, Serologic evidence for Cryptococcus neoformans infection in early childhood, Pediatrics, 2001, 107, 5 CrossRef PubMed.
- N. Singh, F. Dromer, J. R. Perfect and O. Lortholary, Cryptococcosis in solid organ transplant recipients: current state of the science, Clin. Infect. Dis., 2008, 47, 1321–1327 CrossRef PubMed.
- S. A. Schmalzle, U. K. Buchwald, B. L. Gilliam and D. J. Riedel, Mycoses, 2016, 59, 542–552 CrossRef PubMed.
- E. R. Block, A. E. Jennings and J. E. Bennett, 5-fluorocytosine resistance in Cryptococcus neoformans, Antimicrob. Agents Chemother., 1973, 3, 649–656 CrossRef CAS PubMed.
- M. C. Caballero, V. Dyke and F. L. Wormley, A Call to Arms: Quest for a Cryptococcal Vaccine, Trends Microbiol., 2018, 26, 436–446 CrossRef PubMed.
- A. Casadevall, C. Coelho, R. J. B. Cordero, Q. Dragotakes, E. Jung, R. Vij and M. P. Wear, The capsule of Cryptococcus neoformans, Virulence, 2018, 1–10 Search PubMed.
- Z. M. Dong and J. W. Murphy, Effects of the two varieties of Cryptococcus neoformans cells and culture filtrate antigens on neutrophil locomotion, Infect. Immun., 1995, 63, 2632–2644 CrossRef CAS PubMed.
- C. Retini, A. Vecchiarelli, C. Monari, C. Tascini, F. Bistoni and T. R. Kozel, Capsular polysaccharide of Cryptococcus neoformans induces proinflammatory cytokine release by human neutrophils, Infect. Immun., 1996, 64, 2897–2903 CrossRef CAS PubMed.
- R. Cherniak, H. Valafar, L. C. Morris and F. Valafar, Cryptococcus neoformans chemotyping by quantitative analysis of 1H nuclear magnetic resonance spectra of glucuronoxylomannans with a computer-simulated artificial neural network, Clin. Diagn. Lab. Immunol., 1998, 5, 146–159 CrossRef CAS PubMed.
- S. Sheng and R. Cherniak, Structure of the 13C-enriched O-deacetylated glucuronoxylomannan of Cryptococcus neoformans serotype A determined by NMR spectroscopy, Carbohydr. Res., 1997, 301, 33–40 CrossRef CAS PubMed.
- R. Cherniak and J. B. Sundstrom, Polysaccharide antigens of the capsule of Cryptococcus neoformans, Infect. Immun., 1994, 62, 1507–1512 CrossRef CAS PubMed.
- A. K. Bhattacharjee, J. E. Bennett and C. P. Glaudemans, Rev. Infect. Dis., 1984, 6, 619–624 CrossRef CAS PubMed.
- P. M. Ellerbroek, D. J. Lefeber, R. van Veghel, J. Scharringa, E. Brouwer, G. J. Gerwig, G. Janbon, A. I. M. Hoepelman and F. E. J. Coenjaerts, O-Acetylation of Cryptococcal Capsular Glucuronoxylomannan Is Essential for Interference with Neutrophil Migration, J. Immunol., 2004, 173, 7513–7520 CrossRef CAS PubMed.
- C. Crawford, M. P. Wear, D. F. Q. Smith, C. D'Errico, A. Casadevall and S. Oscarson, Antibodies to the Cryptococcus neoformans Capsule have Innate Glycosidase Activity, ChemRxiv, 2020 DOI:10.26434/chemrxiv.12144699.v2.
- S. K. Chow and A. Casadevall, Evaluation of Cryptococcus neoformans galactoxylomannan-protein conjugate as vaccine candidate against murine cryptococcosis, Vaccine, 2011, 29, 1891–1898 CrossRef CAS PubMed.
- A. Casadevall, J. Mukherjee, S. J. N. Devi, R. Schneerson, J. B. Robbins and M. D. Scharff, Antibodies elicited by a cryptococcus neoformans-tetanus toxoid conjugate vaccine have the same specificity as those elicited in infection, J. Infect. Dis., 1992, 165, 1086–1093 CrossRef CAS PubMed.
- C. J. Crawford, R. J. B. Cordero, L. Guazzelli, M. P. Wear, A. Bowen, S. Oscarson and A. Casadevall, Exploring Cryptococcus neoformans capsule structure and assembly with a hydroxylamine-armed fluorescent probe, J. Biol. Chem., 2020, 295, 4327–4340 CrossRef CAS PubMed.
- S. Oscarson, M. Alpe, P. Svahnberg, A. Nakouzi and A. Casadevall, Synthesis and immunological studies of glycoconjugates of Cryptococcus neoformans capsular glucuronoxylomannan oligosaccharide structures, Vaccine, 2005, 23, 3961–3972 CrossRef CAS PubMed.
- A. Nakouzi, T. Zhang, S. Oscarson and A. Casadevall, The common Cryptococcus neoformans glucuronoxylomannan M2 motif elicits non-protective antibodies, Vaccine, 2009, 27, 3513–3518 CrossRef CAS PubMed.
- K. J. Kwon-Chung, J. A. Fraser, T. Á. L. Doering, Z. A. Wang, G. Janbon, A. Idnurm and Y. S. Bahn, Cryptococcus neoformans and Cryptococcus gattii, the etiologic agents of cryptococcosis, Cold Spring Harbor Perspect. Med., 2015, 4, 7 Search PubMed.
- L. Guazzelli, R. Ulc and S. Oscarson, Synthesis of benzyl protected β-d-GlcA-(1 → 2)-α-d-Man thioglycoside building blocks for construction of Cryptococcus neoformans capsular polysaccharide structures, Carbohydr. Res., 2014, 389, 57–65 CrossRef CAS PubMed.
- J. Vesely, L. Rydner and S. Oscarson, Variant synthetic pathway to glucuronic acid-containing di- and trisaccharide thioglycoside building blocks for continued synthesis of Cryptococcus neoformans capsular polysaccharide structures, Carbohydr. Res., 2008, 343, 2200–2208 CrossRef CAS PubMed.
- L. Guazzelli, R. Ulc, L. Rydner and S. Oscarson, A synthetic strategy to xylose-containing thioglycoside tri- and tetrasaccharide building blocks corresponding to Cryptococcus neoformans capsular polysaccharide structures, Org. Biomol. Chem., 2015, 13, 6598–6610 RSC.
- L. Guazzelli, O. McCabe and S. Oscarson, Synthesis of part structures of Cryptococcus neoformans serotype C capsular polysaccharide, Carbohydr. Res., 2016, 433, 5–13 CrossRef CAS PubMed.
- P. J. Garegg, L. Olsson and S. Oscarson, Synthesis of oligosaccharides corresponding to structures found in capsular polysaccharides of crytococcus neoformans. Part 1, J. Carbohydr. Chem., 1993, 12, 955–967 CrossRef CAS.
- P. J. Garegg, L. Olsson and S. Oscarson, Synthesis of Oligosaccharides Corresponding to Structures Found in Capsular Polysaccharides of Cryptococcus Neoformans Part 3. Two Regioselectively Acetylated Tetrasaccharides, J. Carbohydr. Chem., 1997, 16, 973–981 CrossRef CAS.
- D. Crich and O. Vinogradova, Facile oxidative cleavage of 4-O-benzyl ethers with dichlorodicyanoquinone in rhamno- and mannopyranosides, J. Org. Chem., 2007, 72, 3581–3584 CrossRef CAS PubMed.
- P. Fügedi and P. J. Garegg, A novel promoter for the efficient construction of 1,2-trans linkages in glycoside synthesis, using thioglycosides as glycosyl donors, Carbohydr. Res., 1986, 149, 9–12 CrossRef.
- C. Crawford and S. Oscarson, Optimized Conditions for the Palladium-Catalyzed Hydrogenolysis of Benzyl and Naphthylmethyl Ethers: Preventing Saturation of Aromatic Protecting Groups, Eur. J. Org. Chem., 2020, 2020, 3332–3337 CrossRef CAS.
- L. Wang, R. D. Cummings, D. F. Smith, M. Huflejt, C. T. Campbell, J. C. Gildersleeve, J. Q. Gerlach, M. Kilcoyne, L. Joshi, S. Serna, N.-C. Reichardt, N. Parera Pera, R. J. Pieters, W. Eng and L. K. Mahal, Cross-platform comparison of glycan microarray formats, Glycobiology, 2014, 24, 507–517 CrossRef CAS PubMed.
- M. Kilcoyne, J. Q. Gerlach, R. Gough, M. E. Gallagher, M. Kane, S. D. Carrington and L. Joshi, Construction of a Natural Mucin Microarray and Interrogation for Biologically Relevant Glyco-Epitopes, Anal. Chem., 2012, 84, 2020 CrossRef PubMed.
- J. Mukherjee, M. D. Scharff and A. Casadevall, Protective murine monoclonal antibodies to Cryptococcus neoformans, Infect. Immun., 1992, 60, 4534–4541 CrossRef CAS PubMed.
- R. Yuan, A. Casadevall, G. Spira and M. D. Scharff, Isotype switching from IgG3 to IgG1 converts a nonprotective murine antibody to Cryptococcus neoformans into a protective antibody, J. Immunol., 1995, 154, 1810–1816 CAS.
- A. Casadevall, M. DeShaw, M. Fan, F. Dromer, T. R. Kozel and L. A. Pirofski, Molecular and idiotypic analysis of antibodies to Cryptococcus neoformans glucuronoxylomannan, Infect. Immun., 1994, 62, 3864–3872 CrossRef CAS PubMed.
- J. Mukherjee, G. Nussbaum, M. D. Scharff and A. Casadevall, Protective and nonprotective monoclonal antibodies to Cryptococcus neoformans originating from one B cell, J. Exp. Med., 1995, 181, 405–409 CrossRef CAS PubMed.
- D. C. McFadden and A. Casadevall, Unexpected diversity in the fine specificity of monoclonal antibodies that use the same V region gene to glucuronoxylomannan of Cryptococcus neoformans, J. Immunol., 2004, 172, 3670–3677 CrossRef CAS PubMed.
- A. Janda, E. Eryilmaz, A. Nakouzi, M. A. Pohl, A. Bowen and A. Casadevall, Variable region identical IgA and IgE to Cryptococcus neoformans capsular polysaccharide manifest specificity differences, J. Biol. Chem., 2015, 290, 12090–12100 CrossRef CAS PubMed.
- A. Casadevall and A. Janda, Proc. Natl. Acad. Sci. U. S. A., 2012, 109, 12272–12273 CrossRef CAS PubMed.
- A. Janda, A. Bowen, N. S. Greenspan and A. Casadevall, Ig Constant Region Effects on Variable Region Structure and Function, Frontiers in Microbiology, 2016, 7, 22 CrossRef PubMed.
- J. Mukherjee, L. S. Zuckier, M. D. Scharff and A. Casadevall, Therapeutic efficacy of monoclonal antibodies to Cryptococcus neoformans glucuronoxylomannan alone and in combination with amphotericin B, Antimicrob. Agents Chemother., 1994, 38, 580–587 CrossRef CAS PubMed.
- R. A. Larsen, P. G. Pappas, J. Perfect, J. A. Aberg, A. Casadevall, G. A. Cloud, R. James, S. Filler and W. E. Dismukes, Phase I evaluation of the safety and pharmacokinetics of murine-derived anticryptococcal antibody 18B7 in subjects with treated cryptococcal meningitis, Antimicrob. Agents Chemother., 2005, 49, 952–958 CrossRef CAS PubMed.
- A. Varki, R. D. Cummings, M. Aebi, N. H. Packer, P. H. Seeberger, J. D. Esko, P. Stanley, G. Hart, A. Darvill, T. Kinoshita, J. J. Prestegard, R. L. Schnaar, H. H. Freeze, J. D. Marth, C. R. Bertozzi, M. E. Etzler, M. Frank, J. F. G. Vliegenthart, T. Lütteke, S. Perez, E. Bolton, P. Rudd, J. Paulson, M. Kanehisa, P. Toukach, K. F. Aoki-Kinoshita, A. Dell, H. Narimatsu, W. York, N. Taniguchi and S. Kornfeld, Symbol nomenclature for graphical representations of glycans, Glycobiology, 2015, 25, 1323–1324 CrossRef CAS PubMed.
Footnotes |
† Electronic supplementary information (ESI) available. See DOI: 10.1039/d0sc01249a |
‡ Current address: Department of Pharmacy, Università di Pisa, Via Bonanno 6, 56126, Pisa, Italy. |
§ SO and AC share senior authorship. |
|
This journal is © The Royal Society of Chemistry 2020 |
Click here to see how this site uses Cookies. View our privacy policy here.