Acetaminophen and caffeine removal by MnOx(s) and GAC media in column experiments†
Received
24th July 2020
, Accepted 10th November 2020
First published on 10th November 2020
Abstract
The objective of this study was to investigate the application of manganese oxide [MnOx(s)] and granular activated carbon (GAC) media for the removal of caffeine and acetaminophen from water. Organic contaminants of emerging concern represent a developing issue due to their effects on human health and the environment. Manganese oxides are effective for water treatment because of their ability to mediate adsorption and oxidation–reduction reactions for many organic and inorganic constituents. Laboratory scale column experiments were performed using different combinations of commercial MnOx(s) and GAC for assessing the removal of caffeine and acetaminophen, and the subsequent release of soluble Mn due to the reductive dissolution of MnOx(s). The removal of acetaminophen was detected for all media combinations investigated. However, the removal of caffeine by adsorption only occurred in columns containing GAC media. There was no removal of caffeine in columns containing only MnOx(s) media. Manganese release occurred in columns containing MnOx(s) media, but concentrations were below the secondary drinking water standard of 50 μg L−1 set by the US Environmental Protection Agency. Soluble Mn released from a first process by MnOx(s) media column was removed through adsorption into the GAC media used in a second process. The results of this investigation are relevant for implementation of MnOx(s) and GAC media combinations as an effective treatment process to remove organic contaminants from water.
Water impact
Columns with MnOx(s) and GAC media operated in series can be used for the removal of organic compounds such as acetaminophen and caffeine in waters with low organic matter content. The MnOx(s) media can selectively remove acetaminophen, which would retard the exhaustion of available adsorption sites on the non-selective GAC media.
|
1. Introduction
The presence of organic contaminants of emerging concern (CECs) in natural waters is relevant to human health and the environment due to their persistence, accumulation and bioactivity.1–4 These CECs include pharmaceuticals and personal care products (PPCPs), synthetic organic compounds (SOCs), endocrine disrupting compounds (EDCs) and industrial chemicals that can occur in water supplies at very low concentrations ranging from nanograms per liter (ng L−1) to micrograms per liter (μg L−1).5–8 Endocrine disrupting compounds may cause hormonal imbalances in aquatic life, including feminization and decreased reproductive success of organisms. These ecological impacts present the need for the development of water treatment technologies that can target the selective and specific removal of CECs. Many of these compounds may pose a threat to human health, however these risks are not well established.9,10
A variety of treatment processes have been studied for the removal of CECs applicable for drinking water and wastewater. Successful removal depends on the chemical properties of each compound, as well as the characteristics of the solution in which they are found. The most common removal processes are activated carbon adsorption, membrane filtration, and oxidation processes such as chlorination, ozonation, and ultra violet (UV) light.2 Activated carbon adsorption has been thoroughly studied because of its ability to remove a wide range of organic compounds. Activated carbon removal of micropollutants can decrease if the water has a high concentration of dissolved organic matter which competes for sorption sites on the carbon.6,11–13 Similarly, high dissolved organic carbon concentrations can consume oxidants, which decreases removal effectiveness.2,6,14,15 Advanced oxidation processes (AOPs), involving combinations of UV light and oxidants such as hydrogen peroxide (H2O2) or ozone (O3),14–16 or silica-gel enhanced oxidation,17 have been shown to remove acetaminophen, caffeine, and other organic contaminants. However, these technologies have high capital and operating costs and are difficult to operate and maintain. Thus, there remains a need for simpler yet effective technologies.
Acetaminophen and caffeine are common organic contaminants often detected in water effluents which can cause adverse toxic effects on freshwater species and can be considered surrogates for other CECs.16–20 The chemical properties of acetaminophen and caffeine are presented in Table 1. Acetaminophen (N-acetyl-para-aminophenol), also known as paracetamol, is used as an active ingredient in pain relieving drugs such as Tylenol®. Acetaminophen has a phenolic structure that can be oxidized by MnOx(s) (ref. 21–23) into 1,4-benzoquinone.23,24 Caffeine is a naturally occurring central nervous stimulant that is the most consumed psychoactive drug by humans. Caffeine is a purine alkaloid from the methylxanthine compound.16,25,26 Caffeine scavenges highly reactive free radicals and has been shown to have antioxidant activity.25,27 A challenge for degrading caffeine is attacking the C4
C5 double bond in its ring structure.25–28
Table 1 Relevant chemical properties and structure for caffeine and acetaminophen
Chemical |
Structure |
Molecular mass (g mol−1) |
Acid dissociation constant (pKa) |
Obtained from A. C. Moffat et al.52
Obtained from National Center for Biotechnology Information.53
|
Caffeine |
|
194.19 |
14a |
0.7b |
Acetaminophen |
|
151.16 |
9.7 |
Manganese oxides [MnOx(s)] have been commercialized for use in water treatment systems as an adsorbent and oxidation catalyst for removal of inorganic and organic contaminants.22,29–31 Various organic compounds including amines, anilines, phenols, antibacterial agents, atrazine, aromatic N-oxides, and fluoroquinolonics can react with MnOx(s) through adsorption and oxidation.21,32–39 Additionally, oxidation of organic compounds such as aromatic amines and phenols using MnOx(s) has been researched,1,22,36 but fewer studies have focused on the investigation of MnOx(s) reactions with alkaloids such as caffeine. Full scale application of MnOx(s) for adsorption and oxidation of organic compounds is limited and is an area that will benefit from additional research. The use of MnOx(s) and GAC media in combination may provide an alternative technology for treatment to remove organic compounds from drinking water. Addressing these gaps will aid in providing a better understanding of the use and application of MnOx(s) media alone or in combination with GAC as a treatment technology for CECs.
The objective of this study was to investigate the performance of MnOx(s) and GAC media for removal of acetaminophen and caffeine for water treatment in de-ionized (DI) water and a real water system using laboratory column experiments. The compounds acetaminophen and caffeine were selected because they are: 1) common in water and have different chemical behavior in solution; 2) relatively recalcitrant for biological oxidation; and 3) easy to detect and measure. The experimental approach involved the use of column studies to contact contaminated water with MnOx(s) and GAC media separately and in combination. The integration of aqueous chemistry, microscopy, and spectroscopy analyses facilitated the investigation of processes for the removal of acetaminophen and caffeine. The findings from this study provide insights into the combined use of MnOx(s) and GAC for removal of organic contaminants from water.
2. Materials and methods
2.1 Materials
Acetaminophen (≥99% purity) was purchased from Sigma Aldrich. Caffeine (99% purity) was purchased from Alfa Aesar (Ward Hill, MA). Acetaminophen was selected because of its phenolic structure since it is known to be adsorbed by GAC and can be oxidized and adsorbed by MnOx(s). Caffeine was chosen because it has a purine alkaloid structure and limited studies have focused on its removal by MnOx(s). Commercial manganese oxide (LayneOx) was purchased from Layne (Woodlands, TX). The commercial manganese oxide has a Mn content of 70–80% by weight, as reported in other studies that used the same media.37,40 Granular activated carbon (Norit® PK 3-5) was purchased from Sigma Aldrich. Manganese oxides and granular activated carbon media were crushed, washed with 18 mΩ ultrapure water, dried and sieved to particle sizes of 0.250–0.840 mm (US sieve 20 × 60 mesh) for use in the columns.
2.2 Analytical methods
A PerkinElmer Flexar 400 HPLC was used to measure acetaminophen and caffeine. Calibration of the HPLC-UV-VIS was done using stock solutions of acetaminophen and caffeine measured at a wavelength of 254 nm. The HPLC column used was TSKgel ODS-80Tm C-18 (4.6 × 250 mm, 5 μm) with a mobile phase of 40% methanol, 60% 10 mM phosphate buffer at a flow rate of 0.8 mL min−1. The peak for acetaminophen occurred at 2.5 minutes retention time, and the peak for caffeine occurred at 3 minutes retention time. Anion analyses were conducted using ion chromatography (IC, ThermoFisher ICS-1100). Analyses of metals in solution were conducted using a PerkinElmer Optima 5300DV inductively coupled plasma-optical emission spectrometer (ICP-OES) for major elements, and a Perkin Elmer NexIon 300D inductively coupled plasma-mass spectrometer (ICP-MS) for trace elements. Alkalinity was determined by the standardized titration method.41 X-ray photoelectron spectroscopy (XPS) was used to characterize the surface of unreacted and reacted MnOx media. Spectra were collected from three different areas on each sample using a Kratos Axis DLD Ultra XPS with a non-monochromatic Mg Kα source. XPS was done for the experiments with samples taken from both the top and bottom of the columns. A Tescan Vega3 XMU scanning electron microscope/energy dispersive X-ray spectroscope SEM/EDX was used to determine the morphology and chemical composition of both the GAC and MnOx(s) media.
2.3 Experimental set up
2.3.1 Preliminary experiments for process control.
A batch experiment was performed using tap water from the University of New Mexico (UNM) water system to determine whether the water is buffered well enough to maintain a stable pH and measure alkalinity. This tap water comes from a well that supplies the university campus. Chlorination is the only treatment provided before distribution to the campus. For the batch experiment, 50 mL of tap water were placed in an open beaker and stored for 7 days prior to use to allow residual chlorine to decay below the detection limit of 0.1 mg L−1. Then the pH was monitored for 72 hours. Samples of tap water were taken to measure alkalinity using the standardized titration method.41 A second batch experiment was performed to investigate whether the acetaminophen or caffeine decay naturally in tap water. This experiment was performed in beakers using 50 mL of tap water spiked with 50 mg L−1 of acetaminophen and caffeine, along with an additional beaker of un-spiked tap water as a negative control. A calibrated portable meter (Yellow Springs Instrument, Co., Model 63) was used to measure solution pH. Water samples and pH measurements were taken at time intervals 0, 1, 4, 24, and 72 hours. The concentration of caffeine and acetaminophen were determined using HPLC-UV-VIS. Samples were filtered through 0.45 μm filters prior to ICP analyses. Cations (Ca, Mg, Al, Si) in the tap water were analyzed using ICP-OES. Anions (SO42−, NO3−, Cl−, PO43−) were analyzed using IC. All experiments were performed in triplicate.
2.3.2 Removal from spiked DI water.
The single-stage column experiments were conducted as an initial assessment of the performance of MnOx(s) and GAC single-media compared to 50% MnOx(s)/50% GAC dual-media in a spiked DI water solution. This experiment consisted of a setup of single packed columns. Acrylic columns 15.2 cm long by 1.3 cm in diameter were packed with 13 cm of media. 1 cm of glass wool was placed at the entrance and exit of each column to support the media and to distribute flow across the column cross section. A volumetric flow rate of 2.0 ml min−1 was used. Each column was fully packed with the following media combinations: 100% GAC, 100% MnOx(s), and 50% MnOx(s)/50% GAC, and one control column which contained no media (empty column). The 100% MnOx(s) columns were packed full with MnOx(s) media, the 100% GAC columns were packed full with GAC media, and the 50%/50% combination was packed with MnOx(s) media on the bottom and GAC media on top. A spiked DI water containing 50 mg L−1 of acetaminophen and caffeine was prepared by dissolving each constituent in 18 megaohm (MΩ) water with 10 mM HEPES buffer which maintained the pH of the solution at 7.5. This concentration was selected to ensure effluent concentrations would be within the detection limit of the HPLC-UV-VIS. The empty bed contact time (EBCT) was 8.04 minutes, corresponding to 12.7 cm of media considering that the bottom and top of the column were packed with wool. A Masterflex L/S cartridge peristaltic pump, model 7519-25 pumped water into the columns, which were operated in up-flow configuration. Each column experiment was performed in triplicate, except for the control. Effluent samples were collected at times 0.5, 1, 2, 4, 24, 48, 72, 96, 120, 144, 168, and 192 hours and reported as the number of bed volumes (BVs) of water treated. Samples were analyzed with HPLC-UV-VIS for acetaminophen and caffeine, and filtered through 0.45 μm filters prior to Mn analyses using ICP-MS.
2.3.3 Removal from spiked tap water.
Another set of experiments was conducted operating MnOx(s) and GAC columns in series which has important implications for the application of a two-stage water treatment process to remove organic compounds in tap water. These experiments consisted of two columns connected in series with the first column containing MnOx(s) media and the second column containing GAC media. Columns of the same dimensions of those for single column experiments were used. Columns were operated in an up-flow configuration to minimize the presence of trapped bubbles in the packed bed. These experiments were done to determine if the use of GAC media as the second column would remove constituents not completely removed by the MnOx(s) media. Two control columns with no media (empty columns) were operated for the column experiments in series. One column (control 1) was operated in parallel with the MnOx(s) media column in a first process, and another column (control 2) was operated in parallel with the GAC media in a second process.
The concentration of acetaminophen and caffeine in solution was 50 mg L−1 prepared using tap water. The alkalinity of the tap water was sufficient so that an additional buffer was unnecessary. Each of the series was performed in triplicate. Effluent samples were collected at the times 0, 0.5, 1, 2, 3, 4, 5, 24, 48, 72, 96, 120, 144, 192, 216, and 240 hours and reported as the number of BVs of water treated. Samples were analyzed by HPLC-UV-VIS for acetaminophen and caffeine, and filtered through 0.45 μm filters prior to Mn analyses using ICP-MS.
3. Results and discussion
3.1 Removal of acetaminophen and caffeine by MnOx(s) and GAC from spiked DI water
Removal of both acetaminophen and caffeine from spiked DI water was observed in columns reacted with 100% GAC and 50% MnOx(s)/50% GAC media combination (Fig. 1a and b). However, only acetaminophen was removed in columns containing 100% MnOx(s) (Fig. 1a). Minimal caffeine removal was observed in columns containing only MnOx(s) (Fig. 1b). Each column combination reached exhaustion after treating 1254 and 1433 bed volumes (BV) of water after 168–192 hours of reaction. Limited change in concentrations was observed in columns containing no media (control), indicating that the HEPES buffer (at pH 7.5) did not react with acetaminophen or caffeine.
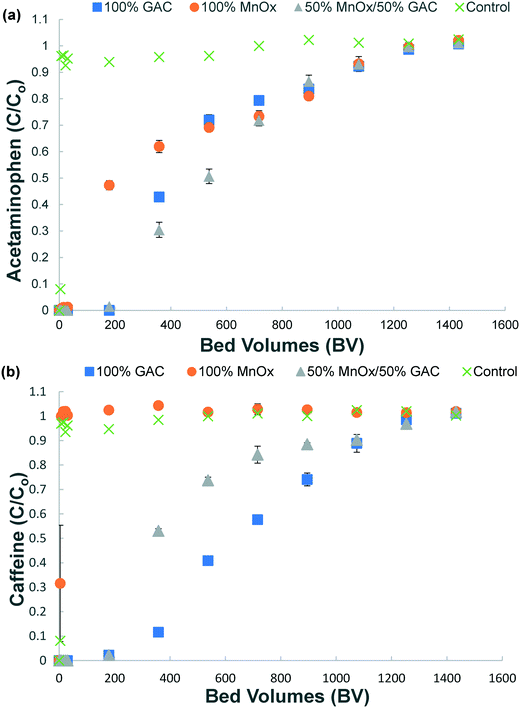 |
| Fig. 1 Normalized effluent concentrations of acetaminophen (a) and caffeine (b) for bench-scale packed columns operated as single columns and fed with a spiked DI water. Each point was obtained by dividing the measured effluent concentration at each point in time by the initial concentration (C0) of 50 mg L−1 for acetaminophen and caffeine. Error bars represent standard deviation of triplicate data. | |
Acetaminophen was removed from solution by all combinations of media. The removal of acetaminophen accomplished with the 50% MnOx(s)/50% GAC media column combination motivated the column experiments with MnOx(s) and GAC columns in series presented in the next sub-section. Acetaminophen has been shown to be removed by GAC.10,32 Other studies have shown that the removal of phenolic compounds, such as acetaminophen, by MnOx(s) occurs by adsorption followed by rapid oxidation.21,22,39 Oxidation of phenolic compounds such as acetaminophen is triggered by reactions that take place on the surface of the MnOx(s) media and is generally considered to occur in two steps: (i) surface complex formation and (ii) electron transfer. These two steps occur at the MnOx(s) surface with subsequent desorption of by-products.23
Caffeine was removed from solution by columns containing 100% GAC and 50% MnOx(s)/50% GAC. The use of GAC alone or in combination with MnOx(s) provided the best removal for both organic compounds. Granular activated carbon can adsorb a large variety of compounds, including caffeine6,12,13,42 due to its high specific surface area.11,43 There was negligible removal of caffeine by the 100% MnOx(s) column. It is possible that, due to the free radical scavenging properties of caffeine,25,44,45 MnOx(s) is not capable of cleaving the C4
C5 double bond,25 thus limiting removal of caffeine by reaction with MnOx(s).
Soluble Mn was found in effluent from the 50% MnOx(s)/50% GAC and 100% MnOx(s) columns at concentrations ranging from 3 to 5 μg L−1, following an initial spike ranging from 20 to 35 μg L−1 for the time zero samples (ESI† – Fig. S1 and S2). The reductive dissolution of MnOx(s) has been observed after reaction with organic compounds; MnOx(s) is reduced from a combined +3/+4 oxidation state to an oxidation state of +2.37,39,46 Dissolved Mn(II) can be adsorbed onto the surface of both the GAC and the MnOx(s). Previous research has identified the re-adsorption of Mn after reductive dissolution of MnOx(s).40,47 All dissolved Mn concentrations detected in the spiked DI water were below the secondary drinking water standard of 50 μg L−1. Reductive dissolution of MnOx(s) after reaction with acetaminophen, aniline, triclosan, BPA and other organic compounds has also been reported in other studies.21,39,46
3.2 Removal of caffeine and acetaminophen by MnOx(s) and GAC from spiked tap water
3.2.1 Preliminary tests.
The pH of the tap water ranged between 7.6 and 7.9 throughout the batch control test (ESI† – Fig. S4). The circumneutral pH range observed throughout the 72 hours of the experiment indicates that the tap water is well buffered with bicarbonate as indicated by the measured alkalinity range of 105–160 mg L−1 as CaCO3. The chemistry of tap water is shown in Table 2. Total organic carbon of the tap water is low, as evidenced by low DBP formation (maximum trihalomethanes are 9 μg L−1, maximum haloacetic acids is 2 μg L−1).48 A batch control experiment found no degradation of acetaminophen or caffeine in tap water over 72 hours (ESI† – Fig. S3). No acetaminophen or caffeine were detected in unspiked UNM tap water. Negligible changes in acetaminophen or caffeine concentrations were detected in the spiked tap water indicating that the background ions have limited effect on the degradation of these organic contaminants.
Table 2 Physicochemical characteristics of tap water
Analyte |
Detected concentration (mg L−1) |
B.D.L. = below detection limit. Note that the lowest standard used for calibration of the ICP-OES (for Ca, Mg, K, Na, Si, Fe, and Mn) and IC (SO42−, NO3−, Cl−, and PO43−) for these analyses is 0.1 mg L−1.
|
Calcium (Ca) |
43.50 |
Magnesium (Mg) |
8.01 |
Potassium (K) |
5.22 |
Sodium (Na) |
33.53 |
Silicon (Si) |
27.9 |
Iron (Fe) |
B.D.L.a |
Manganese (Mn) |
B.D.L.a |
Sulfate (SO42−) |
58.50 |
Nitrate (NO3−) |
2.42 |
Chloride (Cl−) |
30.50 |
Phosphate (PO43−) |
B.D.L.a |
Alkalinity (mg L−1 as CaCO3) |
105–160 |
pH |
7.6–7.9 |
3.2.2 Columns in-series.
The results from the column-in-series experiments indicate that MnOx(s) media will selectively react with phenolic compounds like acetaminophen, but not with caffeine. Removal of acetaminophen was observed by MnOx(s) from spiked tap water in the column experiments (Fig. 2a). The pH of the tap water stayed at a comparable range to that reported in the batch experiments in Fig. S4.† The outcomes with spiked tap water were similar to those obtained in the single column experiments done with the spiked DI water, indicating negligible effect of solution chemistry on adsorption/oxidation reactions of acetaminophen and caffeine. The MnOx(s) media removed acetaminophen throughout the experiment, reaching a maximum C/C0 in the range of 0.8. As observed in the single column spiked DI water experiments, limited removal of caffeine occurred after reaction with MnOx(s) media in the experiments conducted in series (Fig. 2b).
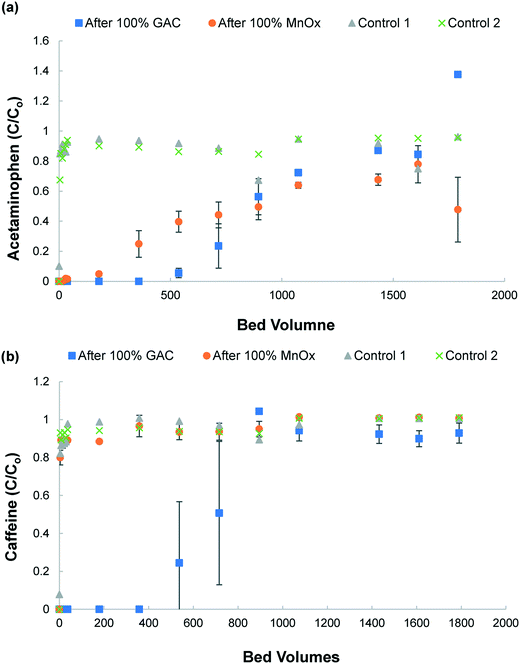 |
| Fig. 2 Normalized effluent concentrations of acetaminophen (a) and caffeine (b) of bench-scale packed columns completed columns in series, first MnOx(s) followed by GAC, and fed with the spiked tap water solution. Each point was obtained by dividing the measured effluent concentration at each point in time by the initial concentration (C0) of acetaminophen and caffeine which was 50 mg L−1. Error bars represent standard deviation of triplicate data. Control 1 represents an empty column with no media operated in parallel with the MnOx(s) media column, and control 2 represents another empty column with no media operated in parallel with the GAC media. | |
The occupation of surface adsorption sites by acetaminophen and reduced Mn can cause gradual passivation of MnOx(s) (Fig. 1 and 2). The results confirm that there is no adsorption or oxidation of caffeine provided by the MnOx(s) media. Acetaminophen and caffeine were both removed by GAC (Fig. 2b). However, since the columns were operated in series with MnOx(s) followed by GAC media, the minimal removal of caffeine by MnOx(s) in the first stage caused exhaustion of the GAC media within 1074 BV. In contrast, the contribution of acetaminophen removal by the MnOx(s) media column in the first stage prior to reaction with the GAC column contributed to the retardation of GAC exhaustion, obtaining a higher BV (after 1430 BV) for acetaminophen compared to caffeine (after 1074 BV). Note that, due to analytical and experimental error, some values for C/C0 are higher than 1. The removal mechanism for GAC media is by adsorption, as it is a non-selective adsorptive media.49 Previous studies have described measurements of Freundlich isotherm parameters for adsorption of acetaminophen and caffeine by GAC.50 Values of K = 1.84 and n = 0.746 have been reported for acetaminophen, and K = 10.8 and n = 0.551 have been reported for caffeine.43 Using these values and a concentration of 50 mg L−1, the adsorption capacity for acetaminophen based on the Polanyi equation and Freundlich parameters was 34.06 mg gGAC−1 for acetaminophen and 93.23 mg gGAC−1 for caffeine, which are consistent with those reported in another study.50 In full scale operating treatment plants, the initial concentrations of these CECs would be in the μg L−1 range but the influence of competing water constituents such as natural organic matter (NOM) and other ions should also be considered.
3.3 MnOx(s) and GAC media solid analyses
Analyses were conducted to investigate the changes in chemical composition of unreacted and reacted MnOx(s) and GAC media, and oxidation state of Mn for MnOx(s) to further assess adsorption and oxidation removal processes. Scanning electron microscope (SEM) and energy dispersive X-ray spectroscopy (EDX) analyses found that both unreacted and reacted MnOx(s) from the column experiments operated in series have a similar morphology and chemical composition. For example, both unreacted and reacted MnOx(s) media (ESI† – Fig. S5a and S6a) have an average grain size ranging from 460–775 μm characterized by a porous surface. The EDX results (ESI† – Fig. S5b and S6b) found that the chemical composition of the virgin and reacted MnOx(s) media consisted primarily of Mn and O (ESI† – Table S1). Impurities included Al (5.69%), and Si (3.94%) (ESI† – Table S1). These results are similar to those reported in a previous study in which commercial MnOx(s) contained 29.1% impurities, including 10% Al, 9% Fe, and 7% Si.37 The chemical composition detected by EDX analyses for unreacted and reacted MnOx(s) media is similar which is why XPS analyses are presented below to further investigate changes in Mn oxidation state after the reaction. The SEM/EDX analyses for unreacted GAC media (ESI† – Fig. S7a) showed a porous surface with grain sizes ranging from 600–800 μm. The EDX results found that the chemical composition of the unreacted GAC was mostly C (94.17%) with a small amount of O (5.22%) (ESI† – Table S1). This is consistent with previous reports.51 As expected, the SEM images for reacted GAC analysis looked similar to those obtained for unreacted GAC (ESI† – Fig. S7a and S8a) with various pore and an average grain size raining from 370–730 μm. Although imaging looked similar, EDX spectra (ESI† – Fig. S7b and S8b) indicated that there were other constituents adsorbed on the surface of the GAC including Si (0.65%), Mg (0.43%), Al (0.35%), and Ca (0.26%), but Mn was below detection (ESI† – Table S1). The presence of these constituents on the reacted GAC is likely due to the adsorption of ions from the tap water.
The detection of soluble Mn in the column effluent indicates that reductive dissolution of the MnOx(s) media occurred in these experiments (Fig. 3), suggesting that the main mechanism for acetaminophen removal was oxidized by MnOx(s). The higher concentration of Mn in columns in series compared to single columns could be due to background concentrations from tap water and constituents in this tap water that undergo redox reactions with the MnOx(s) media which cause Mn dissolution. The Mn reduction in MnOx(s) media was confirmed by XPS analyses of the Mn3p high resolution scans (Fig. 4). The fits of the XPS Mn3p high resolution spectra indicate an increase of 28.4% Mn(III) and 8.8% Mn(II) for the MnOx(s) media from the 50% MnOx(s)/50% GAC media combination column and increase of 33.3% Mn(III) and 8.5% Mn(II) for the 100% MnOx(s) media column compared to what was initially present in the in unreacted media. These results are consistent with previous research which reports the occupation of MnOx(s) surface by reduced Mn on MnOx(s) media during reaction with phenolic organic compounds.37,39,46 It should be noted that the effluent Mn concentration in the effluent of the GAC media is lower than that of the MnOx(s) media (Fig. 3). This result indicates that soluble Mn is released from the MnOx(s) media column caused by acetaminophen removal oxidized by MnOx(s). However, some of this soluble Mn is adsorbed to the GAC media in the second process, resulting in the decrease on soluble Mn in the effluent of the GAC media column compared to the effluent concentration detected in the effluent of the MnOx(s) media column (Fig. 3).
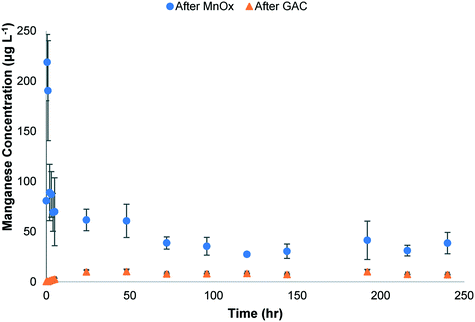 |
| Fig. 3 Manganese concentration from effluents of MnOx and GAC media columns operated in series with spiked tap water. | |
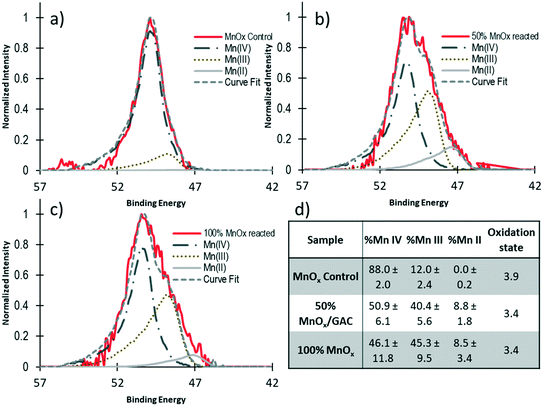 |
| Fig. 4 Fitting of high resolution XPS Mn3p spectra for the single column experiments: (a) unreacted commercial MnOx media before experiments were conducted. (b) Reacted MnOx sample from 50% MnOx(s)/50% GAC media combination column after experiment completion. (c) Reacted MnOx media sample from 100% MnOx media column after experiment completion. (d) Percent composition of the fits for each media (uncertainty shown is standard deviation for triplicate data). | |
3.4 Implications for water treatment
The results from this study suggest that the application of MnOx(s) and GAC in series can remove organic compounds from water with minimal release of soluble Mn. Acetaminophen and caffeine were effectively adsorbed by GAC confirming that the process could be used for water treatment. The price by weight of the commercially available MnOx(s) was ten times less compared to that of the GAC at the time this study was conducted. The use of MnOx(s) and GAC media may be more affordable and applicable for treating a broader range of compounds than by either process alone. Additionally, the performance of the columns in series with MnOx(s) and GAC was better compared to that obtained for each media operated separately. This approach can facilitate the selective removal of phenols, anilines, and other compounds that are removed by MnOx(s),21,34,35,39 slowing the exhaustion of available adsorption sites on GAC. Comparison of results from spiked DI water and spiked tap water solutions suggest that co-occurring ions did not affect the removal of acetaminophen and caffeine. This suggests that a treatment system using MnOx(s) and GAC media for the removal of organic compounds is technically feasible in waters with low organic matter content such as the tap water used in this study. Experiments with high concentrations of NOM and other constituents are necessary to evaluate the removal of organic contaminants from different water chemistry and media combinations. Also, identification of the oxidative byproducts from MnOx(s) treatment and their presence after GAC treatment needs to be further studied due to increased toxicity of some byproducts compared to the parent organic compounds. Addressing these research gaps could provide further opportunities for the application of these media in treatment systems and determine the removal of emerging organic contaminants under these conditions.
4. Conclusions
• The removal of acetaminophen was observed by packed bed columns containing MnOx(s). However, MnOx(s) did not remove caffeine. Columns packed with GAC provided removal of both acetaminophen and caffeine.
• The results indicate that the combination of MnOx(s) and GAC resulted in the removal of acetaminophen and caffeine in the spiked DI water and spiked tap water. The MnOx(s) media can selectively remove acetaminophen, which would retard the exhaustion of available adsorption sites on the non-selective GAC media.
• Reaction of MnOx(s) with acetaminophen resulted in the reductive dissolution of MnOx(s), causing the release of soluble Mn. However, soluble Mn can be adsorbed by GAC media after operation in series with MnOx(s).
Conflicts of interest
There are no conflicts to declare.
Acknowledgements
Special acknowledgement to Cassy McClintock, Andrea Gomez, and Anyssa Romero for supporting the column experiments. This work was supported by the National Science Foundation through the University of New Mexico Center for Water and the Environment (CREST Grant Numbers 1345169 and 1914490). Any opinion, findings, and conclusions or recommendations expressed in this material are those of the authors(s) and do not necessarily reflect the views of the National Science Foundation.
References
- S. Laha and R. G. Luthy, Oxidation of aniline and other primary aromatic amines by manganese dioxide, Environ. Sci. Technol., 1990, 24, 363–373 CrossRef CAS.
- S. A. Snyder, P. Westerhoff, Y. Yoon and D. L. Sedlak, Pharmaceuticals, personal care products, and endocrine disruptors in water: implications for the water industry, Environ. Eng. Sci., 2003, 20, 449–469 CrossRef CAS.
- J. De Rudder, T. Van De Wiele, W. Dhooge, F. Comhaire and W. Verstraete, Advanced water treatment with manganese oxide for the removal of 17α-ethynylestradiol (EE2), Water Res., 2004, 38, 184–192 CrossRef CAS.
- K. Fent, A. A. Weston and D. Caminada, Ecotoxicology of human pharmaceuticals, Aquat. Toxicol., 2006, 76, 122–159 CrossRef CAS.
- C. Pablos, J. Marugán, R. van Grieken and E. Serrano, Emerging micropollutant oxidation during disinfection processes using UV-C, UV-C/H2O2, UV-A/TiO2 and UV-A/TiO2/H2O2, Water Res., 2013, 47, 1237–1245 CrossRef CAS.
- Y. Luo, W. Guo, H. H. Ngo, L. D. Nghiem, F. I. Hai, J. Zhang, S. Liang and X. C. Wang, A review on the occurrence of micropollutants in the aquatic environment and their fate and removal during wastewater treatment, Sci. Total Environ., 2014, 473–474, 619–641 CrossRef CAS.
- T. L. Zearley and R. S. Summers, Removal of trace organic micropollutants by drinking water biological filters, Environ. Sci. Technol., 2012, 46, 9412–9419 CrossRef CAS.
- S. Bernard, P. Chazal and M. Mazet, Removal of organic compounds by adsorption on pyrolusite (β-MnO2), Water Res., 1997, 31, 1216–1222 CrossRef CAS.
- S. T. Glassmeyer, E. T. Furlong, D. W. Kolpin, A. L. Batt, R. Benson, J. S. Boone, O. Conerly, M. J. Donohue, D. N. King, M. S. Kostich, H. E. Mash, S. L. Pfaller, K. M. Schenck, J. E. Simmons, E. A. Varughese, S. J. Vesper, E. N. Villegas and V. S. Wilson, Nationwide reconnaissance of contaminants of emerging concern in source and treated drinking waters of the United States, Sci. Total Environ., 2017, 581–582, 909–922 CrossRef CAS.
- K. A. Baken, R. M. A. Sjerps, M. Schriks and A. P. van Wezel, Toxicological risk assessment and prioritization of drinking water relevant contaminants of emerging concern, Environ. Int., 2018, 118, 293–303 CrossRef CAS.
- A. Katsigiannis, C. Noutsopoulos, J. Mantziaras and M. Gioldasi, Removal of emerging pollutants through Granular Activated Carbon, Chem. Eng. J., 2015, 280, 49–57 CrossRef CAS.
- J. L. Sotelo, G. Ovejero, A. Rodríguez, S. Álvarez, J. Galán and J. García, Competitive adsorption studies of caffeine and diclofenac aqueous solutions by activated carbon, Chem. Eng. J., 2014, 240, 443–453 CrossRef CAS.
- A. M. Kennedy, A. M. Reinert, D. R. U. Knappe, I. Ferrer and R. S. Summers, Full- and pilot-scale GAC adsorption of organic micropollutants, Water Res., 2015, 68, 238–248 CrossRef CAS.
- L. Bo, Z. Shengen and C.-C. Chang, Emerging pollutants - part II: treatment, Water Environ. Res., 2016, 88, 1876–1904 CrossRef.
- M. M. Huber, S. Canonica, G. Y. Park and U. Von Gunten, Oxidation of pharmaceuticals during ozonation and advanced oxidation processes, Environ. Sci. Technol., 2003, 37, 1016–1024 CrossRef CAS.
- I. J. Buerge, T. Poiger, M. D. Müller and H.-R. Buser, Caffeine, an anthropogenic marker for wastewater contamination of surface waters, Environ. Sci. Technol., 2003, 37, 691–700 CrossRef CAS.
- D. W. Kolpin, E. T. Furlong, M. T. Meyer, E. M. Thurman, S. D. Zaugg, L. B. Barber and H. T. Buxton, Pharmaceuticals, hormones, and other organic wastewater contaminants in U.S. streams, 1999−2000: A national reconnaissance, Environ. Sci. Technol., 2002, 36, 1202–1211 CrossRef CAS.
- C. V. T. Rigueto, M. T. Nazari, C. F. De Souza, J. S. Cadore, V. B. Brião and J. S. Piccin, Alternative techniques for caffeine removal from wastewater: An overview of opportunities and challenges, J. Water Process. Eng., 2020, 35, 101231 CrossRef.
- M. T. Moore, S. L. Greenway, J. L. Farris and B. Guerra, Assessing caffeine as an emerging environmental concern using conventional approaches, Arch. Environ. Contam. Toxicol., 2008, 54, 31–35 CrossRef CAS.
- T. G. Santos-Silva, C. C. Montagner and C. B. R. Martinez, Evaluation of caffeine effects on biochemical and genotoxic biomarkers in the neotropical freshwater teleost Prochilodus lineatus, Environ. Toxicol. Pharmacol., 2018, 58, 237–242 CrossRef CAS.
- H. Xiao, H. Song, H. Xie, W. Huang, J. Tan and J. Wu, Transformation of acetaminophen using manganese dioxide − mediated oxidative processes: Reaction rates and pathways, J. Hazard. Mater., 2013, 250–251, 138–146 CrossRef CAS.
- C. K. Remucal and M. Ginder-Vogel, A critical review of the reactivity of manganese oxides with organic contaminants, Environ. Sci.: Processes Impacts, 2014, 16, 1247 RSC.
- M. Huguet, V. Simon and H. Gallard, Transformation of paracetamol into 1,4-benzoquinone by a manganese oxide bed filter, J. Hazard. Mater., 2014, 271, 245–251 CrossRef CAS.
- M. Bedner and W. A. MacCrehan, Transformation of acetaminophen by chlorination produces the toxicants 1,4-benzoquinone and N-acetyl-p-benzoquinone imine, Environ. Sci. Technol., 2006, 40, 516–522 CrossRef CAS.
- I. Dalmázio, L. S. Santos, R. P. Lopes, M. N. Eberlin and R. Augusti, Advanced oxidation of caffeine in water: On-line and real-time monitoring by electrospray ionization mass spectrometry, Environ. Sci. Technol., 2005, 39, 5982–5988 CrossRef.
- J. Sotelo, A. Rodríguez, S. Álvarez and J. García, Removal of caffeine and diclofenac on activated carbon in fixed bed column, Chem. Eng. Res. Des., 2012, 90, 967–974 CrossRef CAS.
- J. R. León-Carmona and A. Galano, Is Caffeine a Good Scavenger of Oxygenated Free Radicals?, J. Phys. Chem. B, 2011, 115, 4538–4546 CrossRef.
- K. Manoli, G. Nakhla, A. K. Ray and V. K. Sharma, Enhanced oxidative transformation of organic contaminants by activation of ferrate(VI): Possible involvement of FeV/FeIV species, Chem. Eng. J., 2017, 307, 513–517 CrossRef CAS.
- M. Hua, S. Zhang, B. Pan, W. Zhang, L. Lv and Q. Zhang, Heavy metal removal from water/wastewater by nanosized metal oxides: A review, J. Hazard. Mater., 2011, 211–212, 317–331 Search PubMed.
- K. A. Bierlein, W. R. Knocke, J. E. Tobiason, A. Subramaniam, M. Pham and J. C. Little, Modeling manganese removal in a pilot-scale postfiltration contactor, J. – Am. Water Works Assoc., 2015, 107, E109–E119 CrossRef.
- M. A. Kessick and B. M. Thomson, Reactions between manganese dioxide and aqueous sulfide, Environ. Lett., 1974, 7, 163–173 CrossRef CAS.
- S. Balgooyen, G. Campagnola, C. K. Remucal and M. Ginder-Vogel, Impact of bisphenol A influent concentration and reaction time on MnO2 transformation in a stirred flow reactor, Environ. Sci.: Processes Impacts, 2019, 21, 19–27 RSC.
- E. L. Trainer, M. Ginder-Vogel and C. K. Remucal, Organic structure and solid characteristics determine reactivity of phenolic compounds with synthetic and reclaimed manganese oxides, Environ. Sci.: Water Res. Technol., 2020, 6, 540–553 RSC.
- H. Zhang, W.-R. Chen and C.-H. Huang, Kinetic modeling of oxidation of antibacterial agents by manganese oxide, Environ. Sci. Technol., 2008, 42, 5548–5554 CrossRef CAS.
- H. Zhang and C.-H. Huang, Oxidative transformation of triclosan and chlorophene by manganese
oxides, Environ. Sci. Technol., 2003, 37, 2421–2430 CrossRef CAS.
- H. Zhang and C.-H. Huang, Oxidative transformation of fluoroquinolone antibacterial agents and structurally related amines by manganese oxide, Environ. Sci. Technol., 2005, 39, 4474–4483 CrossRef CAS.
- N. Shaikh, H. Zhang, K. Rasamani, K. Artyushkova, A.-M. S. Ali and J. M. Cerrato, Reaction of bisphenol A with synthetic and commercial MnOx(s): spectroscopic and kinetic study, Environ. Sci.: Processes Impacts, 2018, 20, 1046–1055 RSC.
- J. Z. Huang, S. F. Zhong, Y. F. Dai, C. C. Liu and H. C. Zhang, Effect of MnO2 phase structure on the oxidative reactivity toward bisphenol A degradation, Environ. Sci. Technol., 2018, 52, 11309–11318 CrossRef CAS.
- N. Shaikh, S. Taujale, H. Zhang, K. Artyushkova, A. M. S. Ali and J. M. Cerrato, Spectroscopic investigation of interfacial interaction of manganese oxide with triclosan, aniline, and phenol, Environ. Sci. Technol., 2016, 50, 10978–10987 CrossRef CAS.
- J. M. Cerrato, W. R. Knocke, M. F. Hochella, A. M. Dietrich, A. Jones and T. F. Cromer, Application of XPS and solution chemistry analyses to investigate soluble manganese removal by MnOx(s)-coated media, Environ. Sci. Technol., 2011, 45, 10068–10074 CrossRef CAS.
-
R. B. Baird, A. D. Eaton, E. W. Rice and L. Bridgewater, Standard methods for the examination of water and wastewater, American Public Health Association Washington, DC, 2017 Search PubMed.
- S. W. Nam, D. J. Choi, S. K. Kim, N. Her and K. D. Zoh, Adsorption characteristics of selected hydrophilic and hydrophobic micropollutants in water using activated carbon, J. Hazard. Mater., 2014, 270, 144–152 CrossRef CAS.
-
K. J. Howe, D. W. Hand, J. C. Crittenden, R. R. Trussell and G. Tchobanoglous, Principles of Water Treatment, John Wiley & Sons, Incorporated, 1st edn, 2012 Search PubMed.
- T. P. A. Devasagayam and P. C. Kesavan, Radioprotective and antioxidant action of caffeine: mechanistic considerations, Indian J. Exp. Biol., 1996, 34, 291–297 CAS.
- T. P. A. Devasagayam, J. P. Kamat, H. Mohan and P. C. Kesavan, Caffeine as an antioxidant: inhibition of lipid peroxidation induced by reactive oxygen species, Biochim. Biophys. Acta, Biomembr., 1996, 1282, 63–70 CrossRef.
- S. Balgooyen, P. J. Alaimo, C. K. Remucal and M. Ginder-Vogel, Structural transformation of MnO2 during the oxidation of bisphenol A, Environ. Sci. Technol., 2017, 51, 6053–6062 CrossRef CAS.
- W. R. Knocke, S. C. Occiano and R. Hungate, Removal of Soluble Manganese by Oxide-coated Filter Media: Sorption Rate and Removal Mechanism Issues, J. – Am. Water Works Assoc., 1991, 83, 64–69 CrossRef CAS.
-
University of New Mexico, 2019 Water Quality Report, Albuquerque, New Mexico, 2019 Search PubMed.
- J. M. Dias, M. C. M. Alvim-Ferraz, M. F. Almeida, J. Rivera-Utrilla and M. Sánchez-Polo, Waste materials for activated carbon preparation and its use in aqueous-phase treatment: A review, J. Environ. Manage., 2007, 85, 833–846 CrossRef CAS.
-
S. Snyder, E. Wert, H. Lei, P. Westerhoff and Y. Yoon, Removal of EDCs and pharmaceuticals in drinking and reuse treatment processes, American Water Works Association Research Foundation (AWWARF), Denver, CO, USA, 2007 Search PubMed.
-
F. Çeçen and Ö. Aktaş, Activated Carbon for Water and Wastewater Treatment, Wiley-VCH, Weinheim, 1st edn, 2011 Search PubMed.
-
A. Moffat, Clarke's Isolation and Identification of Drugs, Pharmaceutical Press, London, U.K, 2nd edn, 1986, pp. 421–423 Search PubMed.
- National Center for Biotechnology Information (2020). PubChem Compound Summary for CID 2519, Caffeine. Retrieved from https://pubchem.ncbi.nlm.nih.gov/compound/Caffeine.
Footnote |
† Electronic supplementary information (ESI) available. See DOI: 10.1039/d0ew00689k |
|
This journal is © The Royal Society of Chemistry 2021 |
Click here to see how this site uses Cookies. View our privacy policy here.