DOI:
10.1039/D0QI01085E
(Research Article)
Inorg. Chem. Front., 2021,
8, 72-78
Sponge-like NiCo2S4 nanosheets supported on nickel foam as high-performance electrode materials for asymmetric supercapacitors
Received
8th September 2020
, Accepted 17th October 2020
First published on 19th October 2020
Abstract
Herein, binder-free sponge-like NiCo2S4 nanosheets supported on Ni foam with ultra-high mass loading were synthesized via a facile one-step hydrothermal route. The unique 3D sponge-like network structure of the obtained NiCo2S4 nanosheets is feasible for the fast transport of ions and electrons. The NiCo2S4 nanosheet-based electrode could achieve a high area capacity of 11.97 F cm−2 at 15 mA cm−2, demonstrating its superior electrochemical performance. Besides, the corresponding asymmetric supercapacitor possesses a maximum area energy density of 0.47 mW h cm−2 at a power density of 6.59 mW cm−2 and a great cycle life with 80.1% capacitance retention even after 5000 cycles. The outstanding performances confirm that the obtained sponge-like NiCo2S4 nanosheets grown on Ni foam can be a prospective candidate for electrochemical energy storage.
Introduction
With the rapid consumption of fossil fuels in the world, it is urgent to develop new energy storage devices such as supercapacitors (SC).1,2 Since pseudocapacitive materials exhibit higher specific capacitance and energy density compared with carbonaceous materials, they have attracted great interest among researchers for supercapacitors.3,4 Until now, a large number of pseudocapacitive materials, such as transition metal oxides5/hydroxides6/sulfides,7 conductive polymers8 and metal–organic frameworks,9 have been investigated and developed for enhanced pseudocapacitance. Among the various materials above, transition metal sulfides, especially ternary transition metal sulfides, have become a research hotspot in the field of SC due to their high theoretical capacitance and excellent electrical conductivity.10
As a typical ternary transition metal sulfide, NiCo2S4 has gained great attention because of its low cost and rich redox reactions.11 Nowadays, NiCo2S4 with various nanostructures and morphologies, such as nanosheets,12 nanoneedles,13 nanorods,14 nano-petals15 and core–shell structures,7 has been widely reported. It is worth noting that NiCo2S4 nanosheets exhibit excellent electrochemical performance. The large specific surface and ultra-thin thickness of NiCo2S4 nanosheets could provide abundant active sites as well as a short transport channel for ions and electrons.16 For example, Lin et al. prepared NiCo2S4 nanosheet arrays with self-decorated nanoneedles on nickel foam by a facile and efficient two-step hydrothermal approach.17 It was demonstrated that the morphology of NiCo2S4 can be transformed from pony-sized and curly nanosheet arrays to large but still thin nanosheet arrays with the increase of reaction time. After that, Liu and co-workers synthesized NiCo2S4 nanosheets arrays on carbon cloth via an in situ growth and subsequent hydrothermal sulfidation, which delivered a high capacity of 1653 F g−1 at a current density of 1 A g−1, superior rate capability and extraordinary cycling stability.18 However, the mass loading of active materials on carbon cloth was only 0.6 mg cm−2, far less than the requirement for commercial equipment (8–10 mg cm−2).5 Although the NiCo2S4 nanosheets mentioned above demonstrate outstanding electrochemical characteristics, the complex fabrication process and low mass loading limit their further application.19
It is well known that the rational design of electrode materials with a well-defined morphology and microstructure is an effective strategy to further enhance their electrochemical properties.20 Recently, electrode materials with a hierarchical structure have been reported widely due to their exceptional properties and remarkable potential in the field of SC.21 Taking 3D sponge-like electrode materials as an example, the presence of a rich open framework and large contact-specific surface area were proved to achieve extraordinary electrochemical performance for SC significantly, especially high-rate performance and long-term cycling stability.22 For instance, Feng reported that 3D sponge-like porous Mn2O3 tiny nanosheets grown on a Ni(OH)2/Mn2O3 nanosheet array-based electrode delivered an outstanding specific capacitance of 2274.4 F g−1 at a current density of 1 A g−1.23 Meanwhile, the corresponding specific capacitance and rate performance are obviously superior to those of pristine Ni(OH)2/Mn2O3 nanosheet arrays. In addition, Li demonstrated a series of 3D sponge-like mesoporous Mn3O4 electrodes, which exhibited superior rate and cycling performance to that of non-sponge-like porous Mn3O4 microspheres.24 It was found that the synthesis of electrode materials with a sponge-like structure is an effective strategy to achieve excellent electrochemical properties. Nevertheless, to the best of our knowledge, there are no reports focused on the synthesis of sponge-like NiCo2S4-based electrodes and their applications for supercapacitors.
Herein, novel 3D sponge-like NiCo2S4 nanosheets with an ultra-high mass loading (18.7 mg cm−2) were obtained via a one-step hydrothermal process. The synthesized sample demonstrates an impressive electrochemical performance with an outstanding area capacitance of 11.97 F cm−2 at a current density of 15 mA cm−2 and superior rate performance. Besides, the corresponding asymmetric supercapacitor presents a high area energy density of 0.47 mW h cm−2, a superior power density of 65.88 mW cm−2 at an energy density of 0.18 mW h cm−2 as well as a great cycle life with 80.1% capacitance retention even after 5000 cycles.
Experimental section
Materials
Ni(NO3)2·6H2O (AR), Co(NO3)2·6H2O (AR), CH3COONa (AR), polyethylene glycol 200 (GD) and (NH2)2CS (AR) were supplied by Sinopharm Chemical Reagent Co., Ltd. Active carbon (AC) was provided by Nanjing XFNANO Materials Tech Co., Ltd. All of the reagents were used without further purification. Ni foam (NF) was cut to 3 × 1 cm2, immersed in 3 M HCl solution, ultrasonicated for 30 min and carefully washed with deionized water and absolute ethanol three times separately.
Growth of NiCo2S4 on NF
The growth of NiCo2S4 on nickel foam was realized through a facile one-step hydrothermal method. In a typical procedure, 0.291 g of Ni(NO3)2·6H2O, 0.582 g of Co(NO3)2·6H2O, 0.492 g of CH3COONa and 0.36 g of (NH2)2CS were added in a mixed solution of 36 mL of deionized water and 4 mL of polyethylene glycol 200 with magnetic stirring for 30 min to form a clear and transparent pink solution. Then, the above solution and two pieces of NFs were transferred to a 100 mL Teflon-lined stainless-steel autoclave and heated at 180 °C for 12 h. After the autoclave was cooled to room temperature naturally, the NFs were taken out and rinsed with deionized water and absolute ethanol three times separately and dried for 12 h at 80 °C. The obtained materials were labeled as NiCo2S4/NF.
Characterization
The crystal structure of the as-synthesized products was characterized using X-ray diffraction (XRD, Rigaku Ultima IV) with Cu-Kα radiation at 35 kV. The morphologies and microstructures of the products were studied by scanning electron microscopy (SEM, Thermo VEG 3 TESCAN) with energy-dispersive X-ray (EDS) and elemental mapping equipment. Transmission electron microscopy (TEM) was performed by using a FEI tecnai G2 F20 S-Twin system operated at 200 kV. The mass loading of NiCo2S4 on NF was studied with an atomic absorption spectrometer (AAS, PERSEE TAS-990).
Electrochemical measurements
Electrochemical measurements were conducted in a three-electrode cell with 2 M KOH as the electrolyte. The as-prepared NiCo2S4/NF was used as the working electrode directly, while a platinum wire was used as the counter electrode and an Ag/AgCl electrode acted as the reference electrode. The electrochemical properties of the as-synthesized sample including cyclic voltammetry (CV), galvanostatic charge/discharge (GCD) and electrochemical impedance spectroscopy (EIS) were measured on a CHI 660E (Chenhua) electrochemical workstation. The nominal area of the sample immersed in the KOH electrolyte was around 1 cm2. The areal and specific capacitance of the electrode was calculated from GCD and based on the following equations:
where Ca (F cm−2) is the areal capacitance, Cs (F g−1) is the specific capacitance, I (A) is the discharge current, t (s) is the discharge time, S (cm2) is the geometrical area of the electrode, m (g) is the mass of the active material on the NF, and ΔV is the potential window. For assembling the asymmetric supercapacitor (ASC), the NiCo2S4/NF electrode and active carbon (AC) were utilized as the positive electrode and negative electrode respectively. 2 M KOH solution was used as the electrolyte. The mass ratio between the positive and negative electrodes is determined by balancing the charge stored in each electrode according to the following equation:
where m+ and m− are the mass of NiCo2S4 and AC on the NF separately, Cs− and Cs+ are the specific capacitance of the positive and negative electrodes respectively, and ΔV− and ΔV+ are the potential window of the positive and negative electrodes, respectively. The corresponding energy density (E, mW h cm−2) and power density (P, mW cm−2) of the ASC were calculated according to the following equations:
where C (F cm−2) is the specific capacitance of the ASC calculated from the GCD curve, V (V) is the potential window of the ASC, and Δt (S) is the discharge time of the ASC obtained from the GCD curve.
Results and discussion
The synthesis procedure of sponge-like 3D interconnected NiCo2S4 nanosheets on Ni foam is depicted in Scheme 1. The NiCo2S4/NF material is directly obtained via a simple hydrothermal process.
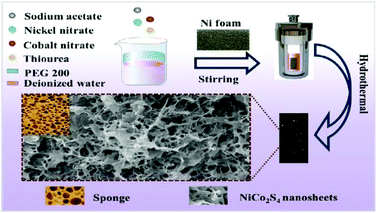 |
| Scheme 1 Preparation process of 3D interconnected NiCo2S4 nanosheets with ultra-high mass loading supported on Ni foam. | |
The structure and morphology of NiCo2S4/NF were studied using a scanning electron microscope as shown in Fig. 1a and b. As depicted in Fig. 1a, a sponge-like 3D interconnected network structure of NiCo2S4 nanosheets on Ni foam can be observed. The presence of high-density nanosheets with a hierarchical and porous structure could provide abundant contact surface area and active sites for redox reactions. More importantly, as shown in Fig. 1b, the 3D sponge-like configuration was made up of interconnected nanosheets with a thickness of around 30 nm, which was beneficial for the materials to be fully utilized. Based on the test using an atomic absorption spectrometer, the mass loading of NiCo2S4 on NF was about 18.7 mg cm−2. As revealed in Fig. 1c, an ultra-thin and porous NiCo2S4 nanosheet-based 3D hierarchical structure directly growing on the Ni foam substrate could not only improve the conductivity of the as-obtained materials, but also supply fast ion diffusion channels and efficient transport pathways, which were feasible for the electrochemical reactions.25
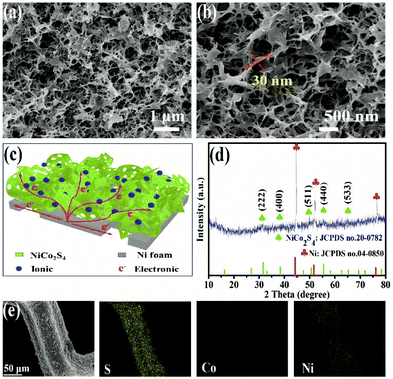 |
| Fig. 1 (a) and (b) SEM images of sponge-like 3D interconnected NiCo2S4 nanosheets supported on Ni foam at different magnifications, (c) possible transportation paths of ions and electrons in NiCo2S4/NF, (d) XRD pattern and (e) SEM image and the corresponding elemental mappings of NiCo2S4/NF. | |
In addition, the crystallographic structure of the NiCo2S4 nanosheets was examined by XRD as shown in Fig. 1d. There were five characteristic diffraction peaks at 31.6°, 38.3°, 50.5°, 55.3° and 65.1°, which could be well indexed to the (222), (400), (511), (440), and (533) planes of the spinel NiCo2S4 crystalline structure (JCPDS card no. 20-0782), separately.26 Excep for three characteristic diffraction peaks derived from Ni foam, there were no additional diffraction peaks, indicating the successful synthesis of NiCo2S4 on NF. Furthermore, elemental mapping of the sample was carried out as shown in Fig. 1e. Ni, Co and S were uniformly distributed on the surface of the materials and no other elements were detected. The above results further proved that NiCo2S4/NF with an interconnected network structure was successfully synthesized by a facile one-step hydrothermal method.
What's more, the structure of NiCo2S4 nanosheets was clarified by TEM and HRTEM as shown in Fig. 2a and b. As depicted in Fig. 2a, the interconnected network structure was consistent with the SEM images of NiCo2S4/NF. In addition, as shown in Fig. 2b, the HRTEM image showed clear lattice fringes with the lattice spacing of 0.166 nm and 0.235 nm, corresponding to the (440) and (400) planes of the spinel NiCo2S4 crystalline structure, which was also in accordance with the XRD pattern.27 Meanwhile, the corresponding selected area electron diffraction (SAED) pattern, as shown in the inset of Fig. 2b, exhibits obvious diffraction rings.
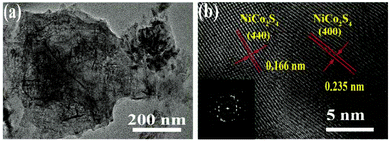 |
| Fig. 2 (a) TEM image of the as-obtained NiCo2S4/NF, (b) HRTEM image with the inset showing the corresponding SAED pattern. | |
In order to study the electrochemical performance of NiCo2S4/NF, the CV curves, galvanostatic charge–discharge curves (GCD) and EIS Nyquist plot were obtained in a three-electrode system with 2 M KOH as the electrolyte. As depicted in Fig. 3a, the CV curves were surveyed in a potential window between −0.3 V and 0.65 V at different scanning rates ranging from 5 mV s−1 to 25 mV s−1. In particular, a part of redox peaks can be observed, indicating that NiCo2S4 is a typical psudocapacitance material with reversible redox based on the following reaction equations:6
NiCo2S4 + 2OH− + H2O ↔ NiSOH + 2CoSOH + 2e− |
NiSOH + OH− ↔ NiSO + H2O + e− |
CoSOH + OH− ↔ CoSO + H2O + e− |
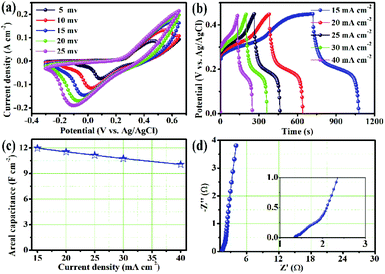 |
| Fig. 3 Three-electrode test of NiCo2S4/NF: (a) CV curves at different sweep rates from 5 mV s−1 to 25 mV s−1, (b) galvanostatic charge–discharge (GCD) curves at different current densities from 15 mA cm−2 to 40 mA cm−2, (c) area capacitance of NiCo2S4/NF at various current densities calculated by GCD, and (d) the corresponding EIS Nyquist plot; the inset shows the magnified image at a high frequency. | |
It is noted that the shape of CV curves does not twist significantly with the increase in sweep rates, demonstrating the excellent stability and high rate performance of the electrode.28 Moreover, the area capacitance of the NiCo2S4/NF electrode was estimated by the GCD test from 0 V to 0.45 V. As shown in Fig. 3b, there are a part of obvious potential voltage plateaus between 0.15 V and 0.35 V, which are in agreement with the CV curves and previous reports.29 As revealed in Fig. 3c, the NiCo2S4/NF electrode shows a series of ultra-high area capacitances of 11.97, 11.54, 11.14, 10.75 and 10.06 F cm−2 at current densities of 15, 20, 25, 30 and 40 mA cm−2, respectively. Impressively, even when the current density was increased to 40 mA cm−2, the area capacitance still retained 84% of the initial value, demonstrating outstanding rate performance and superior psudocapacitance. In addition, the Nyquist plot in Fig. 3d shows the low charge-transfer resistance of the sample. The high slope of the Warburg straight line reflects the low diffusion resistance of electrolyte ions in sponge-like NiCo2S4/NF, which suggests that the synthesized NiCo2S4/NF electrode has good electrical conductivity.30
To further explore the practical use of the NiCo2S4/NF electrode, an asymmetric supercapacitor was packaged. The CV curves of the device were measured at different potential windows as shown in Fig. 4a, and the optimum operation window can be up to 1.65 V. When the potential window was further extended to 1.75 V, the oxygen evolution reaction occurred on the surface of the NiCo2S4/NF electrode.31Fig. 4b shows a series of CV curves of the ASC with different scan rates in the voltage range of 0 V–1.65 V. The change of the redox peak is almost negligible, which illustrates the excellent charge/discharge performance of the ASC.32 Furthermore, a series of symmetrical GCD curves of the NiCo2S4/NF//AC device were measured with the potential range of 0 V–1.65 V as shown in Fig. 4c. The observed voltage plateaus from 1.0 V to 1.65 V are consistent with the CV curves of the device, exhibiting the apparent pseudocapacitance characteristic of the electrode material.33 Furthermore, the area capacitances of the NiCo2S4/NF//AC device were calculated from the GCD curves. As shown in Fig. 4d, the highest area capacitance of the device could reach up to 1.23 F cm−2 at 8 mA cm−2. Even when the current density increased to 80 mA cm−2, the device delivered a capacitance retention of 40% and still maintained the area capacitance of 0.49 F cm−2, indicating the superior rate performance of the assembled ASC.
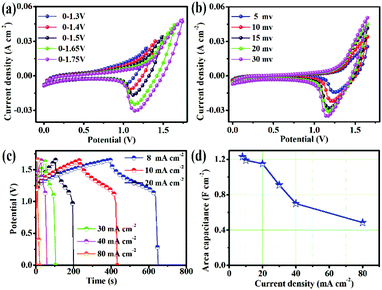 |
| Fig. 4 Electrochemical performance of the NiCo2S4/NF//AC asymmetric supercapacitor: (a) CV curves of ASC at different potential windows with the scanning rate of 20 mV s−1, (b) CV curves of the ASC at different scanning rates with the potential window from 0 V to 1.65 V, (c) GCD curves of the ASC at different current densities, (d) area capacitances of the ASC at various current densities. | |
Meanwhile, Fig. 5a shows the cycling stability and coulombic efficiency of the device. After cycling at 40 mA cm−2 for 5000 cycles, the capacitance retention and coulombic efficiency of the NiCo2S4/NF//AC device were 80.1 and 91.5%, respectively. Furthermore, the capacitance retention of the ASC surpassed 100% after 1500 cycles, which can be attributed to the activation of the electrode material.27 What's more, the energy density and power density of the ASC were calculated according to the GCD curves. As shown in Fig. 5b, the highest area energy density was up to 0.47 mW h cm−2 at an area power density of 6.59 mW cm−2, and an energy density up to 0.18 mW h cm−2 at a maximum power density of 65.88 mW cm−2, demonstrating the excellent application potential. Obviously, the assembled NiCo2S4/NF//AC ASC device in this work is superior to the results reported for similar supercapacitors as depicted in Table 1.34–38
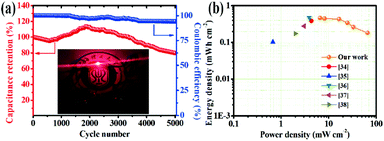 |
| Fig. 5 (a) Cycling performance and coulombic efficiency of the ASC at 40 mA cm−2 after 5000 cycles, with the inset presenting the two ASC configurations connected in series to illuminate a red LED, (b) Ragone plots of the NiCo2S4/NF//AC ASC device in comparison with other reported SC devices. | |
Table 1 Comparison of the electrochemical performance of the asymmetric supercapacitor based on NiCo2S4/NF in this work with other materials as the substrate
Asymmetric supercapacitor |
Energy density (mW h cm−2) |
Power density (mW cm−2) |
Ref. |
Porous carbonized cotton/ZnS/CuS//AC |
0.39 |
4.32 |
34
|
Cu–W–S/Ni//graphene |
0.10 |
0.68 |
35
|
NiS nanotrees//PAC |
0.47 |
3.92 |
36
|
NiCo2S4@NiCo2S4//AC |
0.28 |
3.05 |
37
|
NiCo2S4@CNT//Ti3C2Tx@CCT |
0.18 |
2 |
38
|
NiCo2S4/NF//AC |
0.47 |
6.59 |
Our work |
The excellent electrochemical performance of the NiCo2S4/NF-based electrode can be explained as follows: firstly, the Ni foam underneath can act as a current collector for the NiCo2S4/NF electrode, which is feasible for the rapid transport of electrons and ions as well as decreasing the transfer resistance of the NiCo2S4/NF electrode material significantly. Secondly, ultra-high loading of NiCo2S4 nanosheets on Ni foam could provide plenty of active supporters for energy storage in a unit area. Thirdly, the 3D sponge-like structure consisting of ultra-thin porous nanosheets could offer a larger effective specific surface area, more active sites, and a shorter transport channel for ions and electrons, which plays a vital role in the redox reaction to realize exceptional function. As a result, NiCo2S4/NF with ultra-high mass loading exhibits excellent electrochemical performance.
Conclusion
In summary, sponge-like NiCo2S4 nanosheets supported on NF with ultra-high mass loading were successfully obtained through a facile method. Since the unique 3D porous sponge-like network structure could provide more accessible surface areas and active sites, the NiCo2S4/NF-based electrode exhibited superior electrochemical performance. Noticeably, NiCo2S4/NF delivered a high area capacitance of 11.97 F cm−2 at a current density of 15 mA cm−2. Meanwhile, the corresponding ASC was assembled and achieved the maximum area energy density of 0.47 mW h cm−2 with a power density of 6.59 mW cm−2 and 80.1% capacitance retention even after 5000 cycles. The above results demonstrated that the 3D sponge-like NiCo2S4 nanosheets supported by NF based electrodes with high mass loading could have great potential for energy storage applications.
Conflicts of interest
There are no conflicts to declare.
Acknowledgements
This work was financially supported by the National Natural Science Foundation of China (21405105), the Shanghai Natural Science Foundation (14ZR1429300), and the State Key Laboratory of Green Catalysis of Sichuan Institutes of Higher Education (LZJ1703).
Notes and references
- S. Chen, C. Lu, L. Liu, M. Xu, J. Wang, Q. Deng, Z. Zeng and S. Deng, A hierarchical glucose-intercalated NiMn-G-LDH@NiCo2S4 core-shell structure as a binder-free electrode for flexible all-solid-state asymmetric supercapacitors, Nanoscale, 2020, 12, 1852–1863 RSC.
- B. Li, P. Gu, Y. Feng, G. Zhang, K. Huang, H. Xue and H. Pang, Ultrathin nickel-cobalt phosphate 2D nanosheets for electrochemical energy storage under aqueous/solid-state electrolyte, Adv. Funct. Mater., 2017, 27, 1605784 CrossRef.
- W. Chen, X. Zhang, L. E. Mo, Y. Zhang, S. Chen, X. Zhang and L. Hu, NiCo2S4 quantum dots with high redox reactivity for hybrid supercapacitors, Chem. Eng. J., 2020, 388, 124109 CrossRef CAS.
- Y. Yan, B. Li, W. Guo, H. Pang and H. Xue, Vanadium based materials as electrode materials for high performance supercapacitors, J. Power Sources, 2016, 329, 148–169 CrossRef CAS.
- Z. X. Tong, Y. J. Ji, Q. Z. Tian and W. M. Ouyang, High mass loading and high-density flower-like NiCo2O4 nanosheets on Ni foam for superior capacitance, Chem. Commun., 2019, 55, 9128–9131 RSC.
- J. Zhao, C. Ge, Z. Zhao, Q. Wu, M. Liu, M. Yan, L. Yang, X. Wang and Z. Hu, Sub-nanometer-scale fine regulation of interlayer distance in Ni-Co layered double hydroxides leading to high-rate supercapacitors, Nano Energy, 2020, 76, 105026 CrossRef CAS.
- A. Singh, S. K. Ojha, M. Singh and A. K. Ojha, Controlled synthesis of NiCo2S4@NiCo2O4 core@Shell nanostructured arrays decorated over the rGO sheets for high-performance asymmetric supercapacitor, Electrochim. Acta, 2020, 349, 136349 CrossRef CAS.
- X. Huang and L. Gou, High performance asymmetric supercapacitor based on hierarchical flower-like NiCo2S4@polyaniline, Appl. Surf. Sci., 2019, 487, 68–76 CrossRef CAS.
- C. Ye, Q. Qin, J. Liu, W. Mao, J. Yan, Y. Wang, J. Cui, Q. Zhang, L. Yang and Y. Wu, Coordination derived stable Ni-Co MOFs for foldable all-solid-state supercapacitors with high specific energy, J. Mater. Chem. A, 2019, 7, 4998–5008 RSC.
- Y. Liu, Y. Wen, Y. Zhang, X. Wu, H. Li, H. Chen, J. Huang, G. Liu and S. Peng, Reduced CoNi2S4 nanosheets decorated by sulfur vacancies with enhanced electrochemical performance for asymmetric supercapacitors, Sci. China Mater., 2020, 63, 1216–1226 CrossRef CAS.
- M. Liang, M. Zhao, H. Wang, J. Shen and X. Song, Enhanced cycling stability of hierarchical NiCo2S4@Ni(OH)2@PPy core-shell nanotube arrays for aqueous asymmetric supercapacitors, J. Mater. Chem. A, 2018, 6, 2482–2493 RSC.
- Y. Liu, Z. Li, L. Yao, S. Chen, P. Zhang and L. Deng, Confined growth of NiCo2S4 nanosheets on carbon flakes derived from eggplant with enhanced performance for asymmetric supercapacitors, Chem. Eng. J., 2019, 366, 550–559 CrossRef CAS.
- M. Dong, Z. Wang, G. Yan, J. Wang, H. Guo and X. Li, Confine growth of NiCo2S4 nanoneedles in graphene framework toward high-performance asymmetric capacitor, J. Alloys Compd., 2020, 822, 153645 CrossRef CAS.
- M. Zhang, H. Liu, Z. Song, T. Ma and J. Xie, Self-assembling NiCo2S4 nanorods arrays and T-Nb2O5 nanosheets/three-dimensional nitrogen-doped garphene hybrid nanoarchitectures for advanced asymmetric supercapacitor, Chem. Eng. J., 2020, 392, 123669 CrossRef CAS.
- Y. Wen, S. Peng, Z. Wang, J. Hao, T. Qin, S. Lu, J. Zhang, D. He, X. Fan and G. Cao, Facile synthesis of ultrathin NiCo2S4 nano-petals inspired by blooming buds for high-performance supercapacitors, J. Mater. Chem. A, 2017, 5, 7144–7152 RSC.
- T. Chen, S. Wei and Z. Wang, NiCo2S4-Based Composite Materials for Supercapacitors, ChemPlusChem, 2019, 85, 43–56 CrossRef.
- L. Lin, J. Liu, T. Liu, J. Hao, K. Ji, R. Sun, W. Zeng and Z. Wang, Growth-controlled NiCo2S4 nanosheet arrays with self-decorated nanoneedles for high-performance pseudocapacitors, J. Mater. Chem. A, 2015, 3, 17652–17658 RSC.
- T. Liu, J. Liu, L. Zhang, B. Cheng and J. Yu, Construction of nickel cobalt sulfide nanosheet arrays on carbon cloth for performance-enhanced supercapacitor, J. Mater. Sci. Technol., 2020, 47, 113–121 CrossRef.
- W. Kong, C. Lu, W. Zhang, J. Pu and Z. Wang, Homogeneous core-shell NiCo2S4 nanostructures supported on nickel foam for supercapacitors, J. Mater. Chem. A, 2015, 3, 12452–12460 RSC.
- G. Qu, C. Li, P. Hou, G. Zhao, X. Wang, X. Zhang and X. Xu, Hierarchically hollow structured NiCo2S4@NiS for high-performance flexible hybrid supercapacitors, Nanoscale, 2020, 12, 4686–4694 RSC.
- Y. K. Wang, M. C. Liu, J. Cao, H. J. Zhang, L. B. Kong, D. P. Trudgeon, X. Li and F. C. Walsh, 3D hierarchically structured CoS nanosheets: Li+ storage mechanism and application of the high-performance lithium-ion capacitors, ACS Appl. Mater. Interfaces, 2020, 12, 3709–3718 CrossRef CAS.
- N. Kumar, D. Mishra, S. Y. Kim, T. Na and S. H. Jin, Two dimensional, sponge-like In2S3 nanoflakes aligned on nickel foam via one-pot solvothermal growth and their application toward high performance supercapacitors, Mater. Lett., 2020, 279, 128467 CrossRef CAS.
- L. Feng, J. Sun, Y. Liu, X. Li, L. Ye and L. Zhao, 3D sponge-like porous structure of Mn2O3 tiny nanosheets coated on Ni(OH)2/Mn2O3 nanosheet arrays for quasi-solid-state asymmetric supercapacitors with high performance, Chem. Eng. J., 2018, 339, 61–70 CrossRef CAS.
- S. Li, L. L. Yu, R. B. Li, J. Fan and J. T. Zhao, Template-free and room-temperature synthesis of 3D sponge-like mesoporous Mn3O4 with high capacitive performance, Energy Storage Mater., 2018, 11, 176–183 CrossRef.
- X. Xu, L. Liang, Q. Liu, X. Zhang, Y. Zhao and S. Qiao, In situ induced sponge-like NiMoS4 nanosheets on self-supported nickel foam skeleton for electrochemical capacitor electrode, Colloids Surf., A, 2020, 602, 125099 CrossRef CAS.
- Z. Sun, C. Zhao, X. Cao, K. Zeng, Z. Ma, Y. Hu, J. H. Tia and R. Yang, Insights into the phase transformation of NiCo2S4@rGO for sodium-ion battery electrode, Electrochim. Acta, 2020, 338, 135900 CrossRef CAS.
- H. Wang, M. Liang, C. Ma, W. Shi, D. Duan, G. He and Z. Sun, Novel dealloying-fabricated NiCo2S4 nanoparticles with excellent cycling performance for supercapacitors, Nanotechnology, 2019, 30, 235402 CrossRef CAS.
- F. Yu, Z. Chang, X. Yuan, F. Wang, Y. Zhu, L. Fu, Y. Chen, H. Wang, Y. Wu and W. Li, Ultrathin NiCo2S4@graphene with a core-shell structure as a high performance positive electrode for hybrid supercapacitors, J. Mater. Chem. A, 2018, 6, 5856–5861 RSC.
- Y. Gao, B. Wu, J. Hei, D. Gao, X. Xu, Z. Wei and H. Wu, Self-assembled synthesis of waxberry-like open hollow NiCo2S4 with enhanced capacitance for high-performance hybrid asymmetric supercapacitors, Electrochim. Acta, 2020, 347, 136314 CrossRef CAS.
- Y. J. Ji, Y. L. Deng, F. Chen, Z. Q. Wang, Y. Z. Lin and Z. H. Guan, Ultrathin Co3O4 nanosheets anchored on multi-heteroatom doped porous carbon derived from biowaste for high performance solid-state supercapacitors, Carbon, 2020, 156, 359–369 CrossRef CAS.
- T. Xing, Y. Ouyang, Y. Chen, L. Zheng, H. Shu, C. Wu, B. Chang and X. Wang, Preparation and performances of 3D hierarchical core-shell structural NiCo2S4@NiMoO4·xH2O nanoneedles for electrochemical energy storage, Electrochim. Acta, 2020, 351, 136447 CrossRef CAS.
- B. S. Soram, I. S. Thangjam, J. Y. Dai, T. Kshetri, N. H. Kim and J. H. Lee, Flexible transparent supercapacitor with core-shell Cu@Ni@NiCoS nanofibers network electrode, Chem. Eng. J., 2020, 395, 125019 CrossRef CAS.
- W. Zhao, G. Yan, Y. Zheng, B. Liu, D. Jia, T. Liu, L. Cui, R. Zheng, D. Wei and J. Liu, Bimetal-organic framework derived Cu(NiCo)2S4/Ni3S4 electrode material with hierarchical hollow heterostructure for high performance energy storage, J. Colloid Interface Sci., 2020, 565, 295–304 CrossRef CAS.
- S. Zhai, Z. Fan, K. Jin, M. Zhou, H. Zhao, Y. Zhao, F. Ge, X. Li and Z. Cai, Synthesis of zinc sulfide/copper sulfide/porous carbonized cotton nanocomposites for flexible supercapacitor and recyclable photocatalysis with high performance, J. Colloid Interface Sci., 2020, 575, 306–316 CrossRef CAS.
- P. Pazhamalai, K. Krishnamoorthy, S. Sahoo, V. K. Mariappan and S. J. Kim, Copper tungsten sulfide anchored on Ni-foam as a high-performance binder free negative electrode for asymmetric supercapacitor, Chem. Eng. J., 2019, 359, 409–418 CrossRef CAS.
- G. S. R. Raju, E. Pavitra, G. Nagaraju, S. C. Sekhar, S. M. Ghoreishian, C. H. Kwak, J. S. Yu, Y. S. Huh and Y. K. Han, Rational design of forest-like nickel sulfide hierarchical architectures with ultrahigh areal capacity as a binder-free cathode material for hybrid supercapacitors, J. Mater. Chem. A, 2018, 6, 13178–13190 RSC.
- J. Xie, Y. Yang, G. Li, H. Xia, P. Wang, P. Sun, X. Li, H. Cai and J. Xiong, One-step sulfuration synthesis of hierarchical NiCo2S4@NiCo2S4 nanotube/nanosheet arrays on carbon cloth as advanced electrodes for high-performance flexible solid-state hybrid supercapacitors, RSC Adv., 2019, 9, 3041–3049 RSC.
- J. Yu, J. Zhou, P. Yao, J. Huang, W. Sun, C. Zhu and J. Xu, A stretchable high performance all-in-one fiber supercapacitor, J. Power Sources, 2019, 440, 227150 CrossRef CAS.
|
This journal is © the Partner Organisations 2021 |
Click here to see how this site uses Cookies. View our privacy policy here.