DOI:
10.1039/D0QO01171A
(Research Article)
Org. Chem. Front., 2021,
8, 120-126
Ruthenium catalyzed α-methylation of sulfones with methanol as a sustainable C1 source†
Received
24th September 2020
, Accepted 16th November 2020
First published on 17th November 2020
Abstract
Methylation of sulfones, which have an α-CH bond, can be easily achieved via a one-step, Ru(II) catalyzed redox neutral reaction using methanol as a sustainable C1 building block. The reaction requires a stoichiometric amount of base and generates only water as a byproduct. A series of value-added methylated sulfones with various functional groups are produced under the reaction conditions from readily available substrates. Mechanism studies shows that a sulfone carbanion addition to an in situ generated aldehyde formed via catalytic dehydrogenation and subsequent catalyst mediated reduction of the alkene by hydrogen may be involved.
Introduction
The methyl group, as a very important chemical motif, is widely utilized in the discovery and development of new drugs, which can significantly increase the binding affinity,1 improve the binding selectivity2 and half-life.3 Njarđarson's poster entitled “Top 200 Brand Name Drugs by Prescription in 2016” reveals that over 77% of small-molecule drugs have at least one methyl group, emphasizing the great importance of this scaffold.4 Typically, α-methylated sulfones are often encountered in various biologically active molecules, pharmaceuticals, and natural products, as shown in Fig. 1.5 For example, ceritinib6 is a tyrosine kinase inhibitor for cure of non-small-cell lung cancer, while dorzolamide7 is used to treat elevated intraocular pressure. In addition, benzothiophenes A8 can be employed as insecticide. Furthermore, compound B9 exhibits highly potent inhibition of inflammatory cytokines production and compound C10 was regarded as a γ-secretase inhibitor.
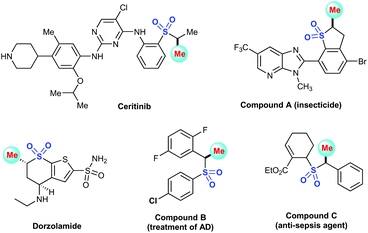 |
| Fig. 1 α-Methylated sulfones in various biologically active molecules, pharmaceuticals, and natural products. | |
Due to its widespread occurrence in nature as well as their remarkable biological activities, various synthetic methods have been developed for the construction of methyl-containing compounds via direct α-methylation. Conventionally, toxic and/or hazardous agents such as iodomethane, diazomethane, dimethyl sulfate, and dimethyl carbonate have been used for the formation of methylated products.11 In order to develop more sustainable strategies, the borrowing hydrogen (BH) strategy or hydrogen autotransfer processes using primary alcohol as green and sustainable starting materials has emerged as a powerful tool for alkylation over the past decades.12 In comparison with benzyl and long-chain n-alkyl alcohols, it is challenging to use methanol as the alkylating agent in BH processes, due partly to the increased energy of dehydrogenation to form the required transient reactive formaldehyde intermediate.13 Recently, exciting results have been achieved for the direct α-methylation of ketones through the borrowing hydrogen methodology employing methanol as a methyl source. Contributions from the groups of Donohoe,14 Obora,15 Andersson,16 Beller,17 Cai,18 Shimizu,19 Morrill,20 and others,21 have illustrated that varieties of transition metals (Ru, Ir, Pd, Mn,…) were able to intrigue such methylation reactions, leading to versatile α-methylated ketones (Scheme 1A). In addition, the methylation of esters has also been developed by Obora,22 then Sortais23 using iridium or manganese-based catalysts, respectively. In the meanwhile, nitriles were proved to be successful substrates in the borrowing hydrogen process to produce α-methyl arylacetonitriles (Scheme 1B).24 However, the methylation of sulfones via BH strategy remains an unsolved task, owing to the high reactivity of the Julia-like intermediate.25 Under previous reported conditions, the cyclopropanation26 (path a) or sulfone elimination to form the olefin27 (path b) was preferred to occur as a major reaction, whereas the direct reduction of Julia-like intermediate (path c) and the catalytic olefin hydrogenation of sulfonylated olefin (path d) to generate the methylated sulfones was suppressed. Very recently, Khaskin group achieved a α-C–H alkylation of sulfones and sulfonamides with long-chain n-alkyl alcohols via a commercial available MACHO ruthenium catalyst.28 Nevertheless, methanol was not compatible in Khashkin's reaction. Encouraged by our previous work,29 we reported here an efficient and straightforward methylation of sulfones using methanol as a sustainable C1 building block via a simple ruthenium catalysis (Scheme 1C).
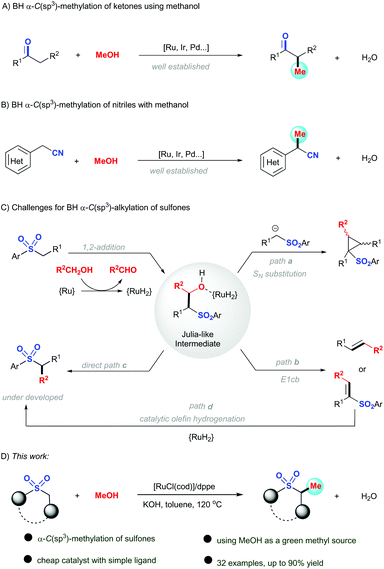 |
| Scheme 1 Transition metal catalyzed borrowing hydrogen (BH) reactions using MeOH as a C1 source. | |
Results and discussion
Initially, the methylation of (benzylsulfonyl)benzene (1a) with methanol was selected as a model reaction to optimize the reaction conditions. As shown in Table 1, the reaction of 1a (0.5 mmol) with methanol (1.5 mL) was performed in the presence of [Rh(cod)Cl]2 (0.05 mmol, 5 mol%) and tBuOK (0.5 mmol, 100 mol%) at 120 °C for 24 h, giving the α-methylated product 2a in 25% isolated yield albeit in low efficiency (Table 1, entry 1). In order to improve the reaction yield, Ir and Ru complexes were used as catalysts, and the yield of the targeting product 2a was significantly increased to 88% (entries 2 and 3). The phosphine ligand played a significant role to enhance catalytic activity, switching dppe to other biphosphine ligand like dppb, dppp and dppf lead to a lower yield (entries 4–6). It was noteworthy that the enantioselective methylation was not observed when using chiral biphosphines (entries 7–9). Next, a set of bases were investigated, and KOH was most suitable for this transformation to offer the best yield of 91% (entries 11–13). In addition, a dramatically decreasement in yield was obtained when lowing the reaction temperature or reducing the amount of KOH (entries 14 and 15). Delightfully, no obvious influence was found when the reaction performed with 2.5 mol% of [Ru(cod)Cl2] (entry 16). Further decreasing the catalyst loading to 1 mol% delivered a relatively lower yield (entry 17). In contrast, 13% yield of ((1-phenylethyl)sulfonyl)benzene (2a) was formed when a blank experiment was performed without the use of ligand, while the reaction failed to yield 2a in the absence of Ru catalyst (entries 18 and 19).
Table 1 Ru-Catalyzed α-methylated sulfones (1a) with MeOHa
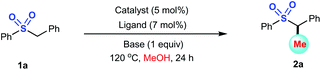
|
Entry |
Catalyst |
Ligand |
Base |
Yieldb (%) |
Reaction conditions: 1a (0.5 mmol), catalyst (5.0 mol%), ligand (7.0 mol%), base (0.5 mmol), MeOH (2.0 mL), oil bath 120 °C, 24 h.
All yields are isolated yields.
100 °C.
KOH (0.25 mmol).
2.5 mol% of [Ru(cod)Cl2] and 3.5 mol% of dppe were used.
1 mol% of [Ru(cod)Cl2] and 1.4 mol% of dppe were used.
|
1 |
[Rh(cod)Cl]2 |
dppe |
t
BuOK |
25 |
2 |
[Ir(cod)Cl]2 |
dppe |
t
BuOK |
80 |
3 |
[Ru(cod)Cl2] |
dppe |
t
BuOK |
88 |
4 |
[Ru(cod)Cl2] |
dppb |
t
BuOK |
71 |
5 |
[Ru(cod)Cl2] |
dppp |
t
BuOK |
80 |
6 |
[Ru(cod)Cl2] |
dppf |
t
BuOK |
41 |
7 |
[Ru(cod)Cl2] |
(S)-BINAP |
t
BuOK |
50 |
8 |
[Ru(cod)Cl2] |
(S,S)-CHIRAPHOS |
t
BuOK |
20 |
9 |
[Ru(cod)Cl2] |
(R,R)-NORPHOS |
t
BuOK |
52 |
10 |
[Ru(cod)Cl2] |
dppe |
t
BuOLi |
43 |
11 |
[Ru(cod)Cl2] |
dppe |
K2CO3 |
54 |
12 |
[Ru(cod)Cl2] |
dppe |
CS2CO3 |
70 |
13 |
[Ru(cod)Cl2] |
dppe |
KOH |
91 |
14c |
[Ru(cod)Cl2] |
dppe |
KOH |
20 |
15d |
[Ru(cod)Cl2] |
dppe |
KOH |
67 |
16e |
[Ru(cod)Cl2] |
dppe |
KOH |
90 |
17f |
[Ru(cod)Cl2] |
dppe |
KOH |
75 |
18 |
[Ru(cod)Cl2] |
|
KOH |
13 |
19 |
|
dppe |
KOH |
0 |
Taking the optimal reaction conditions in hand, the methylation of C(sp3)–H bonds in a broad range of aryl sulfones derivatives was investigated (Scheme 2). The variation of Ar1 adjacent to the sulfonyl group was first examined. Sulfones with electron-donating groups (CH3–, CH3O– and tBu–) gave relatively lower yield (2b, 83%; 2c, 80%; 2d, 78%) than that with an electron-withdrawing group (CF3–, 2e, 88%). The substituted position on Ar1 had a strong influence on the reaction yield, and the ortho-substituted aryl sulfones produced 2i in the lowest yield of 41%. It was noteworthy that when substrates bearing a halogen atom like Cl or Br at the para-position of Ar1 lead to the desired products 2f and 2g successfully under the modified reaction conditions using weak base K2CO3, while meta- or ortho-halo-substituted sufones provided the targeted products in moderate to good yields. In addition, disubstituted sulfones reacted with methanol smoothly to generate the targeted products 2j and 2k in 75% and 83% yields, respectively. Thienyl and naphthyl group were aslo tolerated in this transformation, producing 2l and 2m in excellent yields. Delightfully, alkyl sufones like cyclohexylsulfone 1n still perfomed well to deliver the corresponding product 2n in 52% yield via heating up to 150 °C. Surprisingly, other alcohols such as propanol and butanol reacted with sulfone 1a successfully to offer the expected products 2o and 2p in moderate yields. Next, the variation of Ar2 was screened. Both electron-donating groups and electron-withdrawing groups underwent this reaction efficiently to give the desired products (2q–2y) in 77–88% yields, in which no obvious electronic and steric effects were observed. Pleasingly, the para-halogenated substrates 1s and 1t were compatible with this process, therefore offering a changce for further manupilation. Notably, multiple substituents of aryl moieties were also allowed in this reaction (2z and 2aa). Interestingly, sulfones with fused (hetero)-aromatic rings were found to be promising substrates to provide the corresponding α-methylated products 2ab–2ad in up to 86% isolated yield. Unfortunately, no product was observed while introducing alkyl substituted sulfones as starting materials.
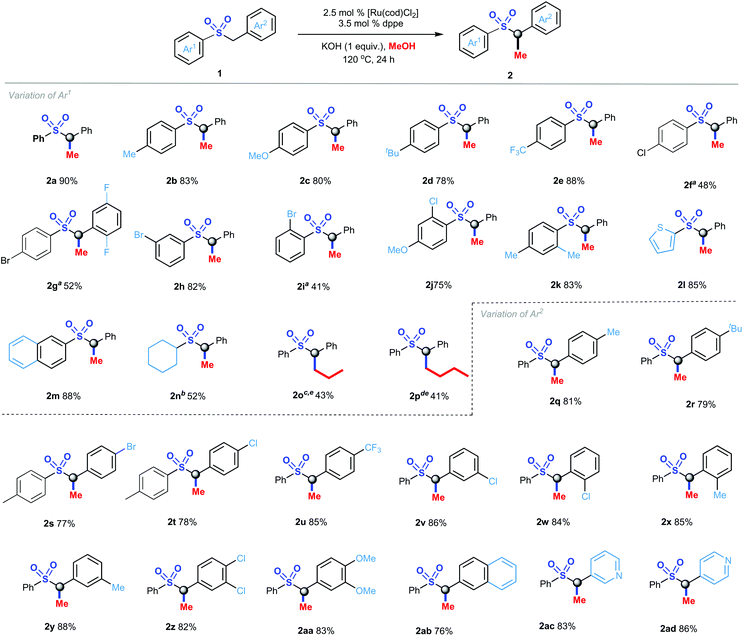 |
| Scheme 2 Substrate scope of sulfones. Reaction conditions: 1 (0.5 mmol), [Ru(cod)Cl2] (2.5 mol%), dppe (3.5 mol%), KOH (0.5 mmol), 2.0 mL of MeOH, 120 °C, 24 h. Isolated yields. aK2CO3 was used instead of KOH. b150 °C. cPropanol was used instead of MeOH. dButanol was used instead of MeOH. eBy-product was formed via cyclopropanation. | |
Dibenzo[b,e]thiepin-11(6H)-one 5,5-dioxide constitutes the fundamental structure of many products with biological activities including antidepressant, antipsychotic, antibacterial, anti-inflammatory and antifungal, and antidepressant activity.30 The substrate scope of this reaction was finally extended to the catalytic α-methylation of dibenzo[b,e]thiepin-11(6H)-one 5,5-dioxide (Scheme 3), providing the methylated dibenzo[b,e]thiepin-11(6H)-one 5,5-dioxide derivatives in 58–65% yields (4a–4d).
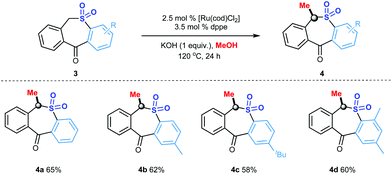 |
| Scheme 3 Substrate scope of dibenzo[b,e]thiepin-11(6H)-one 5,5-dioxides 3. Reaction conditions: 3 (0.5 mmol), [Ru(cod)Cl2] (2.5 mol% mmol), dppe (3.5 mol% mmol), KOH (0.5 mmol), 2.0 mL of MeOH, 120 °C, 24 h. Isolated yields. | |
To demonstrate the scalability and utility of this method, we applied it in a gram-scale experiment and functional group transformations (Scheme 4). Initially, a 5 mmol scale of sulfone 1 reacted with MeOH smoothly to offer the desired product 2a in 1.04 g, 85% yield after 36 h (Scheme 4-1). Next, two desulfonative coupling reactions were conducted, as shown in Scheme 4-2. Using 4-anthpy as a catalyst, the desulfonative borylation of benzyl sulfone 2a procceded successfully to give benzyl boronic ester 5a in 65% yield.31 Alkene 6a was generated from a nickel catalyzed Kumada reaction of 2a with Grignard reagents in excellent yield.32 Finally, sulfone 2i could underwent a Pd-catalyzed intramolecular cyclization to deliver a versatile 6-methyl-6H-benzo[c]thiochromene 5,5-dioxide 7i in 35% yield (Scheme 4-3). Furthermore, to broaden the application of our approach, we were also devoted to synthesis of compound 2ac, a potent inhibitor of inflammatory cytokines that mentioned above. Terminal sulfone B was obtained in 52% yield after 24 h (Scheme 4-4).
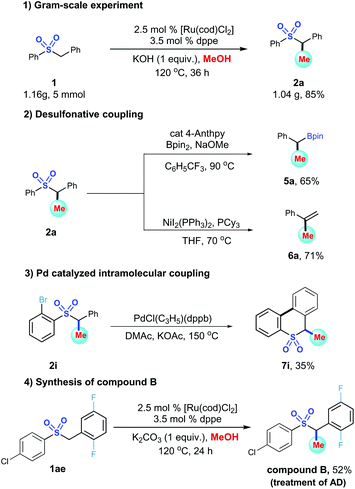 |
| Scheme 4 Gram-scale reaction and transformations. | |
In order to get a preliminary understanding of the reaction mechanism, we carried out several control experiments. Initially, we confirmed that unsaturated aryl sulfone 8a was produced in 80% yield using paraformaldehyde instead of methanol in absence of Ru catalyst (Scheme 5, eqn (1)). Next, the unsaturated aryl ester 8a that was observed in the time-course experiment was reacted in the presence of the Ru catalyst to give α-methylated sulfone 2a in 92% (Scheme 5, eqn (2)). Moreover, a deuterium labeling reaction using methanol-d4 was attempted, which gave the deuterated product 2a′ in 91% yield with 94% deuteration (Scheme 5, eqn (3)). Based on these observations and previous literature,33 we believed that a sulfone carbanion addition to an in situ generated aldehyde formed via catalytic dehydrogenation and subsequent catalyst mediated reduction of the alkene by hydrogen may be involved.
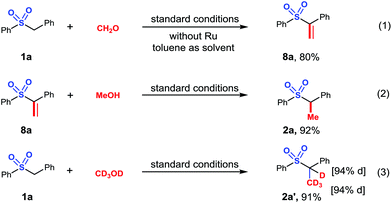 |
| Scheme 5 | |
Conclusions
In conclusion, we have developed a Ru-catalyzed methylation of sulfones using methanol as a sustainable C1 building block. The reaction requires a stoichiometric amount of base and generates only water as a byproduct. A series of value-added methylated sulfones with various functional groups are produced under the reaction conditions from readily available substrates. It is anticipated that this methylation reaction will inspire more new findings and find various applications in future.
Experimental
General procedure for the synthesis of compound 2 and 4
A mixture of [Ru(cod)Cl2] (2.5 mol%), dppe (3.5 mol% mmol), KOH (0.5 mmol), aryl sulfone 1 or 3 (0.5 mmol) and methanol (2.0 mL) was stirred at 120 °C for 24 h under Ar in a pressure tube (ACE pressure tube, 15 mL). After cooling to room temperature, the reaction was diluted with ethyl acetate (10 mL) and water (10 mL). The organic layer was separated, and the aqueous layer was extracted with ethyl acetate (10 mL) for three times. The combined organic layer was washed by brine and dried over magnesium sulfate and the volatiles were removed under reduced pressure. The residue was purified by column chromatography on silica gel (petroleum ether/ethyl acetate = 30
:
1–10
:
1) to give the methylated products 2 and 4.
Conflicts of interest
There are no conflicts to declare.
Acknowledgements
We thank the National Natural Science Foundation of China (No. 22078298, 21978271, 21706234, and 21676253) and the Natural Science Foundation of Zhejiang Province of China (No. LY19B060011) for financial support.
Notes and references
- P. J. Coleman, J. D. Schreier, C. D. Cox, M. J. Breslin, D. B. Whitman, M. J. Bogusky, G. B. McGaughey, R. A. Bednar, W. Lemaire, S. M. Doran, S. V. Fox, S. L. Garson, A. L. Gotter, C. M. Harrell, D. R. Reiss, T. D. Cabalu, D. Cui, T. Prueksaritanont, J. Stevens, P. L. Tannenbaum, R. G. Ball, J. Stellabott, S. D. Young, G. D. Hartman, C. J. Winrow and J. J. Renger, Discovery of [(2R,5R)-5-{[(5-Fluoropyridin-2-yl)oxy]methyl}-2methylpiperidin-1-yl][5-methyl-2-(pyrimidin-2-yl)phenyl]methanone (MK-6096): A Dual Orexin Receptor Antagonist withPotent SleepPromoting Properties, ChemMedChem, 2012, 7, 415 CrossRef CAS; M. Node, X.-J. Hao, K. Nishide and K. Fuji, Chem. Pharm. Bull., 1996, 44, 715–719 CrossRef.
- L. Li, C. Beaulieu, M. C. Carriere, D. Denis, G. Greig, D. Guay, G. O'Neill, R. Zamboni and Z. Wang, Potent and highly selective DP1 antagonists with 2,3,4,9-tetrahydro-1H-carbazole as pharmacophore, Bioorg. Med. Chem. Lett., 2010, 20, 7462 CrossRef CAS.
- A. Gomtsyan, E. K. Bayburt, R. Keddy, S. C. Turner, T. K. Jinkerson, S. Didomenico, R. J. Perner, J. R. Koenig, I. Drizin, H. A. McDonald, C. S. Surowy, P. Honore, J. Mikusa, K. C. Marsh, J. M. Wetter, C. R. Faltynek and C.-H. Lee,
α-Methylation at benzylic fragment of N-aryl-N′-benzyl ureas provides TRPV1 antagonists with better pharmacokinetic properties and higher efficacy in inflammatory pain model, Bioorg. Med. Chem. Lett., 2007, 17, 3894 CrossRef CAS;
(a) D. J. Edmonds, D. Johnston and D. J. Procter, Chem. Rev., 2004, 104, 3371–3404 CrossRef CAS;
(b) C. N. Rao, C. Bentz and H.-U. Reissig, Chem. – Eur. J., 2015, 21, 15951–15954 CrossRef CAS.
- N. A. McGrath, M. Brichacek and J. T. Njarđarson, A Graphical Journey of Innovative Organic Architectures That Have Improved Our Lives, J. Chem. Educ., 2010, 87, 1348 CrossRef CAS.
-
(a) E. A. Ilardi, E. Vitaku and J. T. Njardarson, Data-Mining for Sulfur and Fluorine: An Evaluation of Pharmaceuticals to Reveal Opportunities for Drug Design and Discovery, J. Med. Chem., 2014, 57, 2832 CrossRef CAS;
(b) M. Feng, B. Tang, S.-H. Liang and X. Jiang, Sulfur Containing Scaffolds in Drugs: Synthesis and Application in Medicinal Chemistry, Curr. Top. Med. Chem., 2016, 16, 1200 CrossRef CAS.
- J.-C. Soria, D. S. W. Tan, R. Chiari, Y.-L. Wu, L. Paz-Ares, J. Wolf, S. L. Geater, S. Orlov, D. Cortinovis, C.-J. Yu, M. Hochmair, A. B. Cortot, C.-M. Tsai, D. Moro-Sibilot, R. G. Campelo, T. McCulloch, P. Sen, M. Dugan, S. Pantano, F. Branle, C. Massacesi and G. de Castro Jr., First-Line Ceritinib Versus Platinum-Based Chemotherapy in Advanced ALK-Rearranged Non-Small-Cell Lung Cancer (ASCEND-4): a Randomised, Open-Label, Phase 3 Study, Lancet, 2017, 389, 917 CrossRef CAS.
- A. Nocentini, M. Ceruso, S. Bua, C. L. Lomelino, J. T. Andring, R. McKenna, C. Lanzi, S. Sgambellone, R. Pecori, R. Matucci, L. Filippi, P. Gratteri, F. Carta, E. Masini, S. Selleri and C. T. Supuran, Discovery of β-adrenergic receptors blocker-carbonic anhydrase inhibitor hybrids for multitargeted antiglaucoma therapy, J. Med. Chem., 2018, 61, 5380 CrossRef CAS.
-
R. G. Hall, O. Loiseleur, J. Pabba, S. Pal, A. Jeanguenat, A. Edmunds and A. Stoller, WO2009/010260A2, 2009.
- M. Teall, P. Oakley, T. Harrison, D. Shaw, E. Kay, J. Elliott, U. Gerhard, J. L. Castro, M. Shearman, R. G. Ball and N. N. Tsou, Aryl sulfones: a new class of c-secretase inhibitors, Bioorg. Med. Chem. Lett., 2005, 15, 2685 CrossRef CAS.
- M. Yamada, T. Ichikawa, M. Ii, K. Itoh, N. Tamura and T. Kitazaki, Novel cyclohexene derivatives as anti-sepsis agents: Synthetic studies and inhibition of NO and cytokine production, Bioorg. Med. Chem., 2008, 16, 3941 CrossRef CAS.
-
(a) G. Lamoureaux and C. Agüero, A comparison of several modern alkylating agents, ARKIVOC, 2009, 251 Search PubMed;
(b) G. Szekely, M. C. Amores de Sousa, M. Gil, F. Castelo Ferreira and W. Heggie, Genotoxic Impurities in Pharmaceutical Manufacturing: Sources, Regulations, and Mitigation, Chem. Rev., 2015, 115, 8182 CrossRef CAS.
- For selected recent reviews, see:
(a) A. Corma, J. Navas and M. J. Sabater, Advances in One-Pot Synthesis through Borrowing Hydrogen Catalysis, Chem. Rev., 2018, 118, 1410 CrossRef CAS;
(b) B. G. Reed-Berendt, K. Polidano and L. C. Morrill, Recent Advances in Homogeneous Borrowing Hydrogen Catalysis using Earth-Abundant First Row Transition Metals, Org. Biomol. Chem., 2019, 17, 1595 RSC;
(c) T. Irrgang and R. Kempe, 3d-Metal Catalyzed N- and CAlkylation Reactions via Hydrogen or Hydrogen Autotransfer, Chem. Rev., 2019, 119, 2524 CrossRef CAS.
-
(a) M. Qian, M. A. Liauw and G. Emig, Formaldehyde synthesis from methanol over silver catalysts, Appl. Catal., A, 2003, 238, 211 CrossRef CAS;
(b) W.-H. Lin and H.-F. Chang, A study of ethanol dehydrogenation reaction in a palladium membrane reactor, Catal. Today, 2004, 97, 181 CrossRef CAS.
-
(a) L. K. M. Chan, D. L. Poole, D. Shen, M. P. Healy and T. J. Donohoe, Rhodium-Catalyzed Ketone Methylation Using Methanol Under Mild Conditions: Formation of α-Branched Products, Angew. Chem., Int. Ed., 2014, 53, 761 CrossRef CAS;
(b) D. Shen, D. L. Poole, C. C. Shotton, A. F. Kornahrens, M. P. Healy and T. J. Donohoe, Hydrogen-Borrowing and Interrupted-Hydrogen Borrowing Reactions of Ketones and Methanol Catalyzed by Iridium, Angew. Chem., Int. Ed., 2015, 54, 1642 CrossRef CAS;
(c) X. Wu, F. Xie, Z. Ling, L. Tang and W. Zhang, Regio– and Enantioselective Copper–Catalyzed 1,4−Conjugate Addition of Trimethylaluminium to Linear α,β,γ,δ–Unsaturated Alkyl Ketones, Adv. Synth. Catal., 2016, 358, 2510 CrossRef CAS;
(d) G. Li, X. Huo, X. Jiang and W. Zhang, Asymmetric synthesis of allylic compounds via hydrofunctionalisation and difunctionalisation of dienes, allenes, and alkynes, Chem. Soc. Rev., 2020, 49, 2060 RSC;
(e) Z. Ma, F. Xie, H. Yu, Y. Zhang, X. Wu and W. Zhang, Copper-catalyzed asymmetric 1,4-conjugate addition of Grignard reagents to linear α,β,γ,δ-unsaturated ketones, Chem. Commun., 2013, 49, 5292 RSC.
-
(a) S. Ogawa and Y. Obora, Iridium-catalyzed selective α-methylation of ketones with methanol, Chem. Commun., 2014, 50, 2491 RSC;
(b) K. Oikawa, S. Itoh, H. Kawasaki and Y. Obora, DMF-stabilized iridium nanoclusters as methylation catalysts using methanol as the C1 source, Chem. Commun., 2017, 53, 1080 RSC.
- X. Quan, S. Kerdphon and P. G. Andersson, C-C Coupling of Ketones with Methanol Catalyzed by a N-Heterocyclic Carbene-Phosphine Iridium Complex, Chem. – Eur. J., 2015, 21, 3576 CrossRef CAS.
- Y. Li, H. Li, H. Junge and M. Beller, Selective ruthenium catalyzed methylation of 2-arylethanols using methanol as C1 feedstock, Chem. Commun., 2014, 50, 14991 RSC.
- S.-J. Chen, G.-P. Lu and C. Cai, Iridium-catalyzed methylation of indoles and pyrroles using methanol as feedstock, RSC Adv., 2015, 5, 70329 RSC.
- S. M. A. H. Siddiki, A. S. Touchy, M. A. R. Jamil, T. Toyao and K. Shimizu, C-Methylation of Alcohols, Ketones, and Indoles with Methanol Using Heterogeneous Platinum Catalysts, ACS Catal., 2018, 8, 3091 CrossRef CAS.
- K. Polidano, B. D. W. Allen, J. M. J. Williams and L. C. Morrill, Iron-Catalyzed Methylation Using the Borrowing Hydrogen Approach, ACS Catal., 2018, 8, 6440 CrossRef CAS.
- For selected recent examples, see:
(a) L. Jiang, F. Guo, Z. Shi, Y. Li and Z. Hou, Syndiotactic Poly(aminostyrene)-Supported Palladium Catalyst for Ketone Methylation with Methanol, ChemCatChem, 2017, 9, 3827 CrossRef CAS;
(b) Z. Liu, Z. Yang, X. Yu, H. Zhang, B. Yu, Y. Zhao and Z. Liu, Methylation of C(sp3)-H/C(sp2)-H Bonds with Methanol Catalyzed by Cobalt System, Org. Lett., 2017, 19, 5228 CrossRef CAS;
(c) J. Das, K. Singh, M. Vellakkaran and D. Banerjee, Nickel Catalyzed Hydrogen-Borrowing Strategy for α-Alkylation of Ketones with Alcohols: A New Route to Branched gem-Bis(alkyl) Ketones, Org. Lett., 2018, 20, 5587 CrossRef CAS;
(d) A. Charvieux, J. B. Giorgi, N. Doguet and E. Métay, Solvent-Free Direct α-Alkylation of Ketones by Alcohols Catalyzed by Nickel Supported on Silica-Alumina, Green Chem., 2018, 20, 4210 RSC;
(e) R. Mamidala, P. Biswal, M. S. Subramani, S. Samser and K. Venkatasubbaiah, Palladacycle-Phosphine Catalyzed Methylation of Amines and Ketones Using Methanol, J. Org. Chem., 2019, 84, 10472 CrossRef CAS.
- Y. Tsukamoto, S. Itoh, M. Kobayashi and Y. Obora, Iridium-catalyzed α-methylation of α-aryl esters using methanol as the C1 source, Org. Lett., 2019, 21, 3299 CrossRef CAS.
- A. Bruneau-Voisine, L. Pallova, S. Bastin, V. César and J.-B. Sortais, Manganese Catalyzed α-Methylation of Ketones with Methanol as a C1 Source, Chem. Commun., 2019, 55, 314 RSC.
- For selected recent examples, see:
(a) S. Thiyagarajan and C. Gunanathan, Facile Ruthenium(II)Catalyzed α-Alkylation of Arylmethyl Nitriles Using Alcohols Enabled by Metal−Ligand Cooperation, ACS Catal., 2017, 7, 5483 CrossRef CAS;
(b) J. Li, Y. Liu, W. Tang, D. Xue, C. Li, J. Xiao and C. Wang, Atmosphere-Controlled Chemoselectivity: Rhodium-Catalyzed Alkylation and Olefination of Alkylnitriles with Alcohols, Chem. – Eur. J., 2017, 23, 14445 CrossRef CAS;
(c) Z.-H. Zhu, Y. Li, Y.-B. Wang, Z.-G. Xinju, X. Zhu, X.-Q. Hao and M.-P. Song, α-Alkylation of Nitriles with Alcohols Catalyzed by NNN′Pincer Ru(II) Complexes Bearing Bipyridyl Imidazoline Ligands, Organometallics, 2019, 38, 2156 CrossRef CAS.
-
(a) J. B. Baudin, G. Hareau, S. A. Julia and O. Ruel, A direct synthesis of olefins by reaction of carbonyl compounds with lithio derivatives of 2-[alkyl- or (2′-alkenyl)- or benzyl-sulfonyl]-benzothiazoles, Tetrahedron Lett., 1991, 32, 1175 CrossRef CAS;
(b) P. R. Blakemore, The modified Julia olefination: alkene synthesis via the condensation of metallated heteroarylalkylsulfones with carbonyl compounds, J. Chem. Soc., Perkin Trans. 1, 2002, 2563 RSC.
- T. C. Jankins, R. R. Fayzullin and E. Khaskin, Three-Component [1+1+1] Cyclopropanation with Ruthenium(II), Organometallics, 2018, 37, 2609 CrossRef CAS.
-
(a) S. Waiba, M. K. Barman and B. Maji, Manganese-Catalyzed Acceptorless Dehydrogenative Coupling of
Alcohols with Sulfones: A Tool to Access Highly Substituted Vinyl Sulfones, J. Org. Chem., 2019, 84, 973 CrossRef CAS;
(b) S. Waiba, A. Das, M. K. Barman and B. Maji, Base Metal-Catalyzed Direct Olefinations of Alcohols with Sulfones, ACS Omega, 2019, 4, 7082 CrossRef CAS.
- T. Vojkovsky, S. Deolka, S. Stepanova, M. C. Roy and E. Khaskin, Catalytic Sulfone Upgrading Reaction with Alcohols via Ru(II), ACS Catal., 2020, 10, 6810 CrossRef CAS.
-
(a) F. Ling, J. Chen, S. Nian, H. Hou, X. Yi, F. Wu, M. Xu and W. Zhong, Manganese-Catalyzed Enantioselective Hydrogenation of Simple Ketones Using an Imidazole-Based Chiral PNN Tridentate Ligand, Synlett, 2020, 31, 285 CrossRef CAS;
(b) F. Ling, H. Hou, J. Chen, S. Nian, X. Yi, Z. Wang, D. Song and W. Zhong, Highly Enantioselective Synthesis of Chiral Benzhydrols via Manganese Catalyzed Asymmetric Hydrogenation of Unsymmetrical Benzophenones Using an Imidazole-Based Chiral PNN Tridentate Ligand, Org. Lett., 2019, 21, 3937 CrossRef CAS;
(c) S. Nian, F. Ling, J. Chen, Z. Wang, H. Shen, X. Yi, Y.-F. Yang, Y. She and W. Zhong, Highly Enantioselective Hydrogenation of Non-ortho-Substituted 2-Pyridyl Aryl Ketones via Iridium-f-Diaphos Catalysis, Org. Lett., 2019, 21, 5392 CrossRef CAS;
(d) F. Ling, S. Nian, J. Chen, W. Luo, Z. Wang, Y. Lv and W. Zhong, Development of Ferrocene-Based Diamine-Phosphine-Sulfonamide Ligands for Iridium-Catalyzed Asymmetric Hydrogenation of Ketones, J. Org. Chem., 2018, 83, 10749 CrossRef CAS.
-
(a) T. Kumazawa, M. Yanase, H. Harakawa, H. Obase, S. Ohishi, E. Skirakura, S. Oda, K. Kubo and K. Yamada, Inhibitors of Acyl-CoA: Cholesterol Acyltransferase. Synthesis and Hypocholesterolemic Activity of Dibenz[b,e]oxepin-11-carboxanilides, J. Med. Chem., 1994, 37, 804 CrossRef CAS;
(b) E. Ohshima, S. Otaki, H. Sato, K. Toshiaki, H. Obase, A. Ishii, H. Ishii, K. Ohmori and N. Hirayama, Synthesis and antiallergic activity of 11-(aminoalkylidene)-6,11-dihydrodibenz[b,e]oxepin derivatives, J. Med. Chem., 1992, 35, 2074 CrossRef CAS;
(c) J. R. Mort, R. R. Aparasu and R. K. Baer, Interaction Between Selective Serotonin Reuptake Inhibitors and Nonsteroidal Antiinflammatory Drugs: Review of the Literature, Pharmacotherapy, 2006, 26, 1307 CrossRef CAS.
- Y. Maekawa, Z. T. Ariki, M. Nambo and C. M. Crudden, Pyridine-catalyzed desulfonative borylation of benzyl sulfones, Org. Biomol. Chem., 2019, 17, 7300 RSC.
- J.-C. Wu, L.-B. Gong, Y. Xia, R.-J. Song, Y.-X. Xie and J.-H. Li, Nickel-Catalyzed Kumada Reaction of Tosylalkanes with Grignard Reagents to Produce Alkenes and Modified Arylketones, Angew. Chem., Int. Ed., 2012, 51, 9909 CrossRef CAS.
-
(a) A. Corma, J. Navas and M. J. Sabater, Advances in One-Pot Synthesis through Borrowing Hydrogen Catalysis, Chem. Rev., 2018, 118, 1410 CrossRef CAS;
(b) A. Nandakumar, S. P. Midya, V. G. Landge and E. Balaraman, Transition-Metal-Catalyzed Hydrogen-Transfer Annulations: Access to Heterocyclic Scaffolds, Angew. Chem., Int. Ed., 2015, 54, 11022 CrossRef CAS;
(c) F. Huang, Z. Liu and Z. Yu, C-Alkylation of Ketones and Related Compounds by Alcohols: Transition-Metal Catalyzed Dehydrogenation, Angew. Chem., Int. Ed., 2016, 55, 862 CrossRef CAS;
(d) G. Chelucci, Ruthenium and osmium complexes in C-C bond-forming reactions by borrowing hydrogen catalysis, Coord. Chem. Rev., 2017, 331, 1 CrossRef CAS.
Footnote |
† Electronic supplementary information (ESI) available: Experimental procedures and characterization data of all the compounds. See DOI: 10.1039/d0qo01171a |
|
This journal is © the Partner Organisations 2021 |
Click here to see how this site uses Cookies. View our privacy policy here.