DOI:
10.1039/D0QO01437K
(Research Article)
Org. Chem. Front., 2021,
8, 908-914
Copper-catalyzed three-component oxycyanation of alkenes†
Received
18th November 2020
, Accepted 22nd December 2020
First published on 25th December 2020
Abstract
A copper-catalyzed, three-component reaction for the direct oxycyanation of various alkenes with aryl diacyl peroxides and trimethylsilyl cyanide has been developed. Both unactivated alkenes and styrenes are reliable substrates and produce β-cyanohydrin derivatives. An asymmetric version of this reaction has been conducted and proceeds well.
Nitriles are important molecules that are structural motifs in natural products and pharmaceuticals.1 They are also one of the most versatile types of compounds in organic synthesis because the cyano group can be easily transformed into other different functional groups to afford valuable organic compounds.2 In view of the abundant supply of alkenes, direct vicinal cyanofunctionalization of C
C double bonds is a straightforward strategy for the construction of nitriles. The cyanofunctionalization of the C
C double bond has drawn much attention and significant advances have been achieved in the hydrocyanation3 and carbocyanation4 of alkenes through either an intramolecular or an intermolecular process.5
The direct vicinal oxycyanation of alkenes is a remarkable strategy which simultaneously incorporates a cyano group and an oxygen-containing group into the C
C double bond, forming versatile β-cyanohydrins or their derivatives. A common and versatile approach to β-cyanohydrins is the nucleophilic ring-opening of strained triangular epoxides with a cyanide anion source (Scheme 1a).6 The cyanide anion prefers to regioselectively attack the less substituted carbon center in the epoxide. In recent years, successful oxycyanation of alkenes has also been developed. Nakao et al.7 and Shi's group8 independently disclosed a palladium-catalyzed intramolecular oxycyanation of C
C double bonds (Scheme 1b). Both the methods are proposed to involve the cleavage of the O-CN bond by an electron-rich Pd(0) catalyst, as depicted in their mechanisms. On the other hand, two-component oxycyanation of alkenes with a linked oxygen source has been reported by Alexanian,9 Han,5g and Zhu10 (Scheme 1b). However, these oxycyanation reactions are limited by their tendency to generate β-cyanohydrin derivatives with the cyano group attached to the less substituted carbon center.
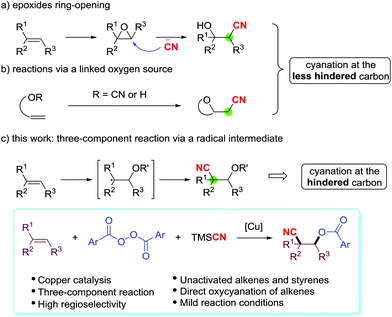 |
| Scheme 1 Oxycyanation of alkenes. | |
An attraction of three-component oxycyanation of alkenes is that it uses a one-step process to assemble multifunctional structures from various compounds, and this can greatly broaden the utility of the method. Recently, Liu et al. described an electron donor–acceptor (EDA) complex enabled three-component oxycyanation of C
C double bonds.11 Electron-rich vinyl ethers are the key substrates in this reaction, but the direct oxycyanation of alkenes in a three-component model with generic unactivated alkenes is a valuable process. Herein, we report the three-component, copper-catalyzed oxycyanation of unactivated alkenes and styrenes with aryl diacyl peroxides as the oxygen source and trimethylsilyl cyanide (TMSCN) as the cyano source (Scheme 1c). Mechanistic studies suggest that the O-radical attacks the double bond first and the reaction produces the β-cyanohydrin derivatives with the cyano group at the more substituted carbon center.
We began by examining the scope of copper catalysts, ligands, solvents and temperature, using the model reaction of styrene with benzoyl peroxide12 (BPO, 2a) and TMSCN (3). The details of the optimization of the conditions are provided in Table 1. Copper catalysts were screened with 1,10-phenanthroline (L1) as the ligand and CH3CN as the solvent at 50 °C. Cu(CH3CN)4PF6 was the most effective catalyst tested with which the reaction provided the desired oxycyanation product (4a) in 47% yield (entries 1–3). Solvents such as HFIP (hexafluoroisopropanol) and 1,4-dioxane were less efficient than CH3CN, but TFEA (2,2,2-trifluoroethanol) provided the highest yield (entries 4–6). The yield of 4a was increased to 77% by using higher concentrations of the substrates (entries 7–9). Other ligands were evaluated, and it was found that 1,10-phenanthroline is the most effective ligand (entries 10–14 vs. entry 9). The yield of the desired product (4a) was further improved to 83% by using two equivalents of TMSCN (entry 15). In the absence of any ligand, the catalytic efficiency drops dramatically (entry 16).
Table 1 Optimization of the reaction conditionsa
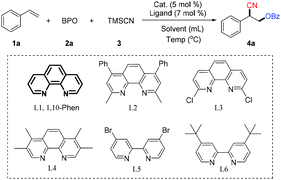
|
Entry |
Cat. |
Ligand |
Solvent (mL) |
Yieldb (%) |
Reaction conditions: 1a (0.50 mmol, 1 equiv.), 2a (1.5 equiv.), 3 (1.5 equiv.), cat., ligand, and solvent, at 50 °C for 24 h.
Yield was determined by 1H NMR analysis.
2 equiv. of 3 were used; an isolated yield in parentheses.
|
1 |
Cu(CH3CN)4PF6 |
L1
|
CH3CN (2) |
47 |
2 |
CuTc |
L1
|
CH3CN (2) |
17 |
3 |
CuOAc |
L1
|
CH3CN (2) |
2 |
4 |
Cu(CH3CN)4PF6 |
L1
|
HFIP (2) |
33 |
5 |
Cu(CH3CN)4PF6 |
L1
|
Dioxane (2) |
23 |
6 |
Cu(CH3CN)4PF6 |
L1
|
TFEA (2) |
54 |
7 |
Cu(CH3CN)4PF6 |
L1
|
TFEA (1.5) |
60 |
8 |
Cu(CH3CN)4PF6 |
L1
|
TFEA (1) |
67 |
9 |
Cu(CH3CN)4PF6 |
L1
|
TFEA (0.5) |
77 |
10 |
Cu(CH3CN)4PF6 |
L2
|
TFEA (0.5) |
38 |
11 |
Cu(CH3CN)4PF6 |
L3
|
TFEA (0.5) |
35 |
12 |
Cu(CH3CN)4PF6 |
L4
|
TFEA (0.5) |
66 |
13 |
Cu(CH3CN)4PF6 |
L5
|
TFEA (0.5) |
69 |
14 |
Cu(CH3CN)4PF6 |
L6
|
TFEA (0.5) |
71 |
15c |
Cu(CH3CN)4PF6 |
L1
|
TFEA (0.5) |
83(81) |
16 |
Cu(CH3CN)4PF6 |
— |
TFEA (0.5) |
13 |
With the optimized reaction conditions in hand, the substrate scope of the reaction with respect to vinylarenes was investigated (Table 2). The reactions of o-, m- or p-alkyl-substituted vinylarenes with BPO afforded the corresponding oxycyanation products (4b–4e) in moderate yields. Vinylarenes bearing an ester substituent also underwent this reaction, affording the product (4f) in 66% yield. When a halogen, such as fluorine, chlorine, or bromine, was attached to the phenyl ring, the reaction afforded the desired products (4g–4k) in moderate yields. A 1,1-disubstituted vinylarene was also examined and it was found to be a suitable substrate in the reaction, producing the product (4l) in 53% yield. Acyclic or cyclic 1,2-disubstituted vinylarenes were also compatible under the reaction conditions, giving the corresponding oxycyanation products (4m–4p) in moderate yields. This reaction can proceed with more complex substrates. For example, the oxycyanation product (4q) can be produced in 46% yield with the substrate that is a derivative from the natural product estrone.13
Table 2 Scope for oxycyanation of styrenesa
Reaction conditions: 1 (0.50 mmol, 1 equiv.), 2a (1.5 equiv.), and 3 (2 equiv.) in TFEA (0.5 mL) at 50 °C for 24 h.
Addition of 5 mol% of Hantzsch ester.
|
|
We next examined unactivated alkenes, a class of challenging but useful substrates (Table 3). In general, the reactions of a variety of unactivated alkenes afford the corresponding products with less efficiency than the reactions of styrenes. Unactivated alkenes with an alkyl chain could afford the desired oxycyanation in moderate yield (6a–6c). 1,1-Disubstituted unactivated alkenes were suitable substrates for this reaction, producing the oxycyanation products (6f–6j) in yields of 39–61%. Notably, this reaction can use not only terminal alkenes as the substrates, but also internal alkenes. For example, cyclohexene is a good substrate for the reaction, affording the desired product (6h) in 61% yield. Substrates with a phenyl group (6d), a trimethylsilyl group (6e) or a free hydroxyl group (6l) are compatible with the reaction, making it a powerful method for the difunctionalization of alkenes.
Table 3 Scope of the oxycyanation reaction with unactivated alkenesa
Reaction conditions: 5 (0.50 mmol, 1 equiv.), 2a (1.5 equiv.), and 3 (2 equiv.) in TFEA (0.5 mL) at 50 °C for 24 h.
|
|
The scope of diacyl peroxides in this oxycyanation reaction was also examined (Table 4). A variety of diacyl peroxides can afford the corresponding oxycyanation products (7a–7f) in moderate yields.12a
Table 4 Scope of the oxycyanation reaction with diacyl peroxidesa
Reaction conditions: 1a (0.5 mmol), 2 (0.75 mmol), and 3 (1.0 mmol) in TFEA (0.5 mL) at 50 °C for 24 h.
|
|
The copper-catalyzed asymmetric oxycyanation of alkenes was also evaluated.12c,14 After considerable evaluation (for details, see the ESI†), a combination of a Cu(II) species and a bisoxazoline ligand (*L13) was found to be the most efficient catalyst system, and the results are summarized in Table 5. The copper-catalyzed asymmetric oxycyanation of alkenes could afford the desired products with an er value of 91
:
9. However, under the asymmetric conditions, almost no enantiomeric excess was obtained with an aliphatic alkene (5d). The absolute configuration of the products (R-form of 4e, CCDC 2040065†) was confirmed by X-ray single crystal diffraction.
Table 5 Asymmetric oxycyanation of alkenesa
1 (0.5 mmol), 2 (0.75 mmol), and 3 (1.0 mmol) in TFEA (0.5 mL) at rt for 24 h.
|
|
In order to demonstrate the potential synthetic value of the oxycyanation reactions, we investigated the subsequent transformations of compound 4a. As shown in Scheme 2, the oxycyanation product (4a) efficiently engaged in selective olefination, deprotection or cyano functionalization to afford an α,β-unsaturated primary amide (8), a β-cyanohydrin (9), or a tetrazole (10).15 These results demonstrate the potential of this method to provide valuable synthetic compounds.
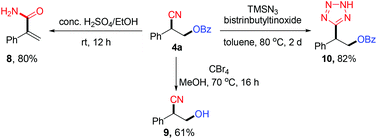 |
| Scheme 2 Synthetic applications of the oxycyanation products. | |
Preliminary experiments were performed to probe the mechanism of the oxycyanation reaction (Scheme 3). First, radical trapping experiments were conducted and found that TEMPO (2,2,6,6-tetramethyl-1-piperidinyloxy) and BHT (2,6-di-tert-butyl-4-methylphenol) inhibit the formation of the oxycyanation product16 (Scheme 3a). The results are consistent with the involvement of radical species. To further support this hypothesis, compound 11 bearing a cyclopropylmethyl moiety was tested as a radical clock (Scheme 3b).17 The reaction of compound 11 with BPO and TMSCN afforded the ring-opening product (12) in 33% yield, further supporting the involvement of radical species in the reaction.
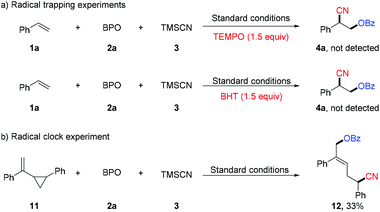 |
| Scheme 3 Preliminary mechanistic studies. | |
Based on these mechanistic studies, a catalytic mechanism† for the oxycyanation reaction is proposed (Scheme 4). Initially, a copper(I) species (A) undergoes single-electron transfer with BPO to afford the copper(II) species (B) and a benzoyl radical,12a,18 which can be trapped by an alkene to produce a more stable carbon-centered radical (C). Upon ligand exchange with TMSCN, the copper(II) species (B) is converted into a copper(II) species (D),12c,19 which then undergoes cyano group transfer with the stabilized carbon-centered radical (C) through an intermediate (E) to afford the oxycyanation product, and regenerate the copper catalyst. The oxycyanation product can also be formed through the reductive elimination of the copper(III) species (F).20
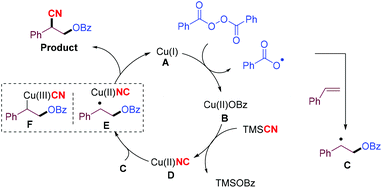 |
| Scheme 4 Plausible catalytic cycle for the oxycyanation of alkenes. | |
Conclusions
In conclusion, we have developed a copper-catalyzed three-component reaction which leads to direct oxycyanation of various alkenes with aryl diacyl peroxides and TMSCN. Both unactivated alkenes and styrenes are reliable substrates under the mild reaction conditions, affording β-cyanohydrin derivatives in moderate to good yields. The utility of these direct oxycyanation products has been demonstrated with further synthetic applications. The copper-catalyzed asymmetric oxycyanation of alkenes has been conducted and proceeds well.
Conflicts of interest
There are no conflicts to declare.
Acknowledgements
Supported by the NSFC (Grant No. 21672213, 21871258, 21922112 and 22001251), the Haixi Institute of CAS (Grant No. CXZX-2017-P01), the Scientific Research Foundation of Fujian Normal University in China and Innovative Research Teams Program II of Fujian Normal University in China (IRTL1703).
Notes and references
-
(a) F. F. Fleming, Nitrile-containing natural products, Nat. Prod. Rep., 1999, 16, 597 RSC;
(b) F. F. Fleming, L. Yao, P. C. Ravikumar, L. Funk and B. C. Shook, Nitrile-containing pharmaceuticals: efficacious roles of the nitrile pharmacophore, J. Med. Chem., 2010, 53, 7902 CrossRef CAS.
-
(a)
Z. Rappoport and S. Patai, The Chemistry of the Cyano Group, Wiley, London, 1970 Search PubMed;
(b) G. Yan, Y. Zhang and J. Wang, Recent Advances in the Synthesis of Aryl Nitrile Compounds, Adv. Synth. Catal., 2017, 359, 4068 CrossRef CAS.
-
(a) A. Falk, A. L. Goderz and H. G. Schmalz, Enantioselective nickel-catalyzed hydrocyanation of vinylarenes using chiral phosphine-phosphite ligands and TMS-CN as a source of HCN, Angew. Chem., Int. Ed., 2013, 52, 1576 CrossRef CAS;
(b) Y. Liu, S. Shirakawa and K. Maruoka, Phase-transfer-catalyzed asymmetric conjugate cyanation of alkylidenemalonates with KCN in the presence of a Bronsted acid additive, Org. Lett., 2013, 15, 1230 CrossRef CAS;
(c) C. Lu, X. Su and P. E. Floreancig, Stereocontrolled cyanohydrin ether synthesis through chiral Bronsted acid-mediated vinyl ether hydrocyanation, J. Org. Chem., 2013, 78, 9366 CrossRef CAS;
(d) Y. Sakaguchi, N. Kurono, K. Yamauchi and T. Ohkuma, Asymmetric conjugate hydrocyanation of alpha,beta-unsaturated N-acylpyrroles with the Ru(phgly)2(binap)-CH3OLi catalyst system, Org. Lett., 2014, 16, 808 CrossRef CAS;
(e) J. Zhang, X. Liu and R. Wang, Magnesium complexes as highly effective catalysts for conjugate cyanation of alpha,beta-unsaturated amides and ketones, Chem. – Eur. J., 2014, 20, 4911 CrossRef CAS;
(f) Y.-J. Zhang, Q.-L. Hou, H.-J. Wang and W.-W. Liao, Lewis base-promoted rearrangement of allylic cyanohydrins: construction of functionalized nitriles bearing 1,3-diketone moieties, J. Org. Chem., 2014, 79, 10890 CrossRef CAS;
(g) S. Arai, H. Hori, Y. Amako and A. Nishida, A new protocol for nickel-catalysed regio- and stereoselective hydrocyanation of allenes, Chem. Commun., 2015, 51, 7493 RSC;
(h) A. Falk, A. Cavalieri, G. S. Nichol, D. Vogt and H.-G. Schmalz, Enantioselective Nickel-Catalyzed Hydrocyanation using Chiral Phosphine-Phosphite Ligands: Recent Improvements and Insights, Adv. Synth. Catal., 2015, 357, 3317 CrossRef CAS;
(i) K. Kiyokawa, T. Nagata, J. Hayakawa and S. Minakata, Straightforward synthesis of 1,2-dicyanoalkanes from nitroalkenes and silyl cyanide mediated by tetrabutylammonium fluoride, Chem. – Eur. J., 2015, 21, 1280 CrossRef CAS;
(j) X. Fang, P. Yu and B. Morandi, Catalytic reversible alkene-nitrile interconversion through controllable transfer hydrocyanation, Science, 2016, 351, 832 CrossRef CAS;
(k) A. Jakhar, P. Kumari, M. Nazish, N.-u. H. Khan, R. I. Kureshy, S. H. R. Abdi and E. Suresh, Catalytic asymmetric synthesis of enantioenriched β-nitronitrile bearing a C-CF3 stereogenic center, RSC Adv., 2016, 6, 29977 RSC;
(l) K. Kiyokawa, T. Nagata and S. Minakata, Electrophilic Cyanation of Boron Enolates: Efficient Access to Various beta-Ketonitrile Derivatives, Angew. Chem., Int. Ed., 2016, 55, 10458 CrossRef CAS;
(m) K. Nemoto, T. Nagafuchi, K.-i. Tominaga and K. Sato, Efficient nickel-catalyzed hydrocyanation of alkenes using acetone cyanohydrin as a safer cyano source, Tetrahedron Lett., 2016, 57, 3199 CrossRef CAS;
(n) G. Strappaveccia, T. Angelini, L. Bianchi, S. Santoro, O. Piermatti, D. Lanari and L. Vaccaro, Synthesis of β-Cyano Ketones Promoted by a Heterogeneous Fluoride Catalyst, Adv. Synth. Catal., 2016, 358, 2134 CrossRef CAS;
(o) Y. Amako, S. Arai and A. Nishida, Transfer of axial chirality through the nickel-catalysed hydrocyanation of chiral allenes, Org. Biomol. Chem., 2017, 15, 1612 RSC;
(p) M. Hatano, K. Yamakawa and K. Ishihara, Enantioselective Conjugate Hydrocyanation of α,β-Unsaturated N-Acylpyrroles Catalyzed by Chiral Lithium(I) Phosphoryl Phenoxide, ACS Catal., 2017, 7, 6686 CrossRef CAS;
(q) Z. Li and J. Yin, Conjugate Hydrocyanation of Chalcone Derivatives Using Ethyl Cyanoacetate as an Organic Cyanide Source, Chin. J. Chem., 2017, 35, 1179 CrossRef CAS;
(r) A. Jakhar, M. Nazish, N. Gupta, N.-u. H. Khan and R. I. Kureshy, Enantioselective Addition of Cyanide to CF3-Substituted Alkylidenemalonates: Construction of Trifluoromethylated All-Carbon Quaternary Stereocenters, ChemistrySelect, 2018, 3, 4838 CrossRef CAS;
(s) A. Bhunia, K. Bergander and A. Studer, Cooperative Palladium/Lewis Acid-Catalyzed Transfer Hydrocyanation of Alkenes and Alkynes Using 1-Methylcyclohexa-2,5-diene-1-carbonitrile, J. Am. Chem. Soc., 2018, 140, 16353 CrossRef CAS;
(t) X. Li, C. You, J. Yang, S. Li, D. Zhang, H. Lv and X. Zhang, Asymmetric Hydrocyanation of Alkenes without HCN, Angew. Chem., Int. Ed., 2019, 58, 10928 CrossRef CAS;
(u) A. W. Schuppe, G. M. Borrajo-Calleja and S. L. Buchwald, Enantioselective Olefin Hydrocyanation without Cyanide, J. Am. Chem. Soc., 2019, 141, 18668 CrossRef CAS;
(v) L. Song, N. Fu, B. G. Ernst, W. H. Lee, M. O. Frederick, R. A. DiStasio Jr. and S. Lin, Dual electrocatalysis enables enantioselective hydrocyanation of conjugated alkenes, Nat. Chem., 2020, 12, 747 CrossRef;
(w) R. Yu, S. Rajasekar and X. Fang, Enantioselective Nickel-Catalyzed Migratory Hydrocyanation of Nonconjugated Dienes, Angew. Chem., Int. Ed., 2020, 59, 21436 CrossRef CAS.
-
(a) A. Pinto, Y. Jia, L. Neuville and J. Zhu, Palladium-catalyzed enantioselective domino heck-cyanation sequence: development and application to the total synthesis of esermethole and physostigmine, Chem. – Eur. J., 2007, 13, 961 CrossRef CAS;
(b) M. P. Watson and E. N. Jacobsen, Asymmetric intramolecular arylcyanation of unactivated olefins via C-CN bond activation, J. Am. Chem. Soc., 2008, 130, 12594 CrossRef CAS;
(c) Y. Nakao, S. Ebata, A. Yada, T. Hiyama, M. Ikawa and S. Ogoshi, Intramolecular arylcyanation of alkenes catalyzed by nickel/AlMe2Cl, J. Am. Chem. Soc., 2008, 130, 12874 CrossRef CAS;
(d) J. Chen, G. Zou and W. Liao, Metal-free intramolecular carbocyanation of activated alkenes: functionalized nitriles bearing beta-quaternary carbon centers, Angew. Chem., Int. Ed., 2013, 52, 9296 CrossRef CAS;
(e) N. O. Ilchenko, P. G. Janson and K. J. Szabo, Copper-mediated cyanotrifluoromethylation of styrenes using the Togni reagent, J. Org. Chem., 2013, 78, 11087 CrossRef CAS;
(f) Y.-T. He, L.-H. Li, Y.-F. Yang, Z.-Z. Zhou, H.-L. Hua, X.-Y. Liu and Y.-M. Liang, Copper-catalyzed intermolecular cyanotrifluoromethylation of alkenes, Org. Lett., 2014, 16, 270 CrossRef CAS;
(g) N. R. Rondla, J. M. Ogilvie, Z. Pan and C. J. Douglas, Palladium catalyzed intramolecular acylcyanation of alkenes using alpha-iminonitriles, Chem. Commun., 2014, 50, 8974 RSC;
(h) D. A. Petrone, A. Yen, N. Zeidan and M. Lautens, Dearomative Indole Bisfunctionalization via a Diastereoselective Palladium-Catalyzed Arylcyanation, Org. Lett., 2015, 17, 4838 CrossRef CAS;
(i) N. S. Dange, F. Robert and Y. Landais, Free-Radical Carbocyanation of Cyclopropenes: Stereocontrolled Access to All-Carbon Quaternary Stereocenters in Acyclic Systems, Org. Lett., 2016, 18, 6156 CrossRef CAS;
(j) F. Wang, D. Wang, X. Wan, L. Wu, P. Chen and G. Liu, Enantioselective Copper-Catalyzed Intermolecular Cyanotrifluoromethylation of Alkenes via Radical Process, J. Am. Chem. Soc., 2016, 138, 15547 CrossRef CAS;
(k) N. Wang, L. Li, Z.-L. Li, N.-Y. Yang, Z. Guo, H.-X. Zhang and X.-Y. Liu, Catalytic
Diverse Radical-Mediated 1,2-Cyanofunctionalization of Unactivated Alkenes via Synergistic Remote Cyano Migration and Protected Strategies, Org. Lett., 2016, 18, 6026 CrossRef CAS;
(l) Q. Guo, M. Wang, Y. Wang, Z. Xu and R. Wang, Photoinduced, copper-catalyzed three components cyanofluoroalkylation of alkenes with fluoroalkyl iodides as fluoroalkylation reagents, Chem. Commun., 2017, 53, 12317 RSC;
(m) H. Hassan, V. Pirenne, M. Wissing, C. Khiar, A. Hussain, F. Robert and Y. Landais, Free-Radical Carbocyanation of Olefins, Chem. – Eur. J., 2017, 23, 4651 CrossRef CAS;
(n) R. Ren, Z. Wu, L. Huan and C. Zhu, Synergistic Strategies of Cyano Migration and Photocatalysis for Difunctionalization of Unactivated Alkenes: Synthesis of Di- and Mono-Fluorinated Alkyl Nitriles, Adv. Synth. Catal., 2017, 359, 3052 CrossRef CAS;
(o) W. Sha, L. Deng, S. Ni, H. Mei, J. Han and Y. Pan, Merging Photoredox and Copper Catalysis: Enantioselective Radical Cyanoalkylation of Styrenes, ACS Catal., 2018, 8, 7489 CrossRef CAS;
(p) F. Wang, D. Wang, Y. Zhou, L. Liang, R. Lu, P. Chen, Z. Lin and G. Liu, Divergent Synthesis of CF3 -Substituted Allenyl Nitriles by Ligand-Controlled Radical 1,2- and 1,4-Addition to 1,3-Enynes, Angew. Chem., Int. Ed., 2018, 57, 7140 CrossRef CAS;
(q) Q. Guo, M. Wang, Q. Peng, Y. Huo, Q. Liu, R. Wang and Z. Xu, Dual-Functional Chiral Cu-Catalyst-Induced Photoredox Asymmetric Cyanofluoroalkylation of Alkenes, ACS Catal., 2019, 9, 4470 CrossRef CAS;
(r) M. Ji, Z. Wu and C. Zhu, Visible-light-induced consecutive C-C bond fragmentation and formation for the synthesis of elusive unsymmetric 1,8-dicarbonyl compounds, Chem. Commun., 2019, 55, 2368 RSC;
(s) Y. Jiao, M.-F. Chiou, Y. Li and H. Bao, Copper-Catalyzed Radical Acyl-Cyanation of Alkenes with Mechanistic Studies on the tert-Butoxy Radical, ACS Catal., 2019, 9, 5191 CrossRef CAS;
(t) C. Wang, Q. Shang, R. Qi, H. Chai, H. Wang, M. Guo and Z. Xu, Cu-Catalyzed cyanoalkylation of electron-deficient alkenes with unactivated alkyl bromides, Chem. Commun., 2019, 55, 9991 RSC;
(u) W. Bao, Z.-P. Gao, D.-P. Jin, C.-G. Xue, H. Liang, L.-S. Lei, X.-T. Xu, K. Zhang and S.-H. Wang, Direct synthesis of 2-substituted benzonitriles via alkylcyanation of arynes with N,N-disubstituted aminomalononitriles, Chem. Commun., 2020, 56, 7641 RSC;
(v) X.-J. Chen, Q.-W. Gui, R. Yi, X. Yu, Z.-L. Wu, Y. Huang, Z. Cao and W.-M. He, Copper(I)-catalyzed intermolecular cyanoarylation of alkenes: convenient access to α-alkylated arylacetonitriles, Org. Biomol. Chem., 2020, 18, 5234 RSC;
(w) M. Israr, H. Xiong, Y. Li and H. Bao, Copper-Catalyzed Enantioselective Cyano(Fluoro)Alkylation of Alkenes, Adv. Synth. Catal., 2020, 362, 2211 CrossRef CAS;
(x) T. Zhang, Y.-X. Luan, S.-J. Zheng, Q. Peng and M. Ye, Chiral Aluminum Complex Controls Enantioselective Nickel-Catalyzed Synthesis of Indenes: C-CN Bond Activation, Angew. Chem., 2020, 59, 7439 CrossRef CAS.
-
(a) H. Zhang, W. Pu, T. Xiong, Y. Li, X. Zhou, K. Sun, Q. Liu and Q. Zhang, Copper-catalyzed intermolecular aminocyanation and diamination of alkenes, Angew. Chem., Int. Ed., 2013, 52, 2529 CrossRef CAS;
(b) H. Gao, B. Liu, W. Wu and H. Jiang, Palladium-catalyzed selective aminoamidation and aminocyanation of alkenes using isonitrile as amide and cyanide sources, Chem. Commun., 2014, 50, 15348 RSC;
(c) Y. Miyazaki, N. Ohta, K. Semba and Y. Nakao, Intramolecular aminocyanation of alkenes by cooperative palladium/boron catalysis, J. Am. Chem. Soc., 2014, 136, 3732 CrossRef CAS;
(d) Z. Pan, S. M. Pound, N. R. Rondla and C. J. Douglas, Intramolecular aminocyanation of alkenes by N-CN bond cleavage, Angew. Chem., Int. Ed., 2014, 53, 5170 CAS;
(e) L. Xu, X.-Q. Mou, Z.-M. Chen and S.-H. Wang, Copper-catalyzed intermolecular azidocyanation of aryl alkenes, Chem. Commun., 2014, 50, 10676 RSC;
(f) Z. Wu, R. Ren and C. Zhu, Combination of a Cyano Migration Strategy and Alkene Difunctionalization: The Elusive Selective Azidocyanation of Unactivated Olefins, Angew. Chem., Int. Ed., 2016, 55, 10821 CrossRef CAS;
(g) F. Chen, F.-F. Zhu, M. Zhang, R.-H. Liu, W. Yu and B. Han, Iminoxyl Radical-Promoted Oxycyanation and Aminocyanation of Unactivated Alkenes: Synthesis of Cyano-Featured Isoxazolines and Cyclic Nitrones, Org. Lett., 2017, 19, 3255 CrossRef CAS;
(h) H. Shen, Q. Deng, R. Liu, Y. Feng, C. Zheng and Y. Xiong, Intramolecular aminocyanation of alkenes promoted by hypervalent iodine, Org. Chem. Front., 2017, 4, 1806 RSC;
(i) D. Wang, F. Wang, P. Chen, Z. Lin and G. Liu, Enantioselective Copper-Catalyzed Intermolecular Amino- and Azidocyanation of Alkenes in a Radical Process, Angew. Chem., Int. Ed., 2017, 56, 2054 CrossRef CAS;
(j) Z. Pan, S. Wang, J. T. Brethorst and C. J. Douglas, Palladium and Lewis-Acid-Catalyzed Intramolecular Aminocyanation of Alkenes: Scope, Mechanism, and Stereoselective Alkene Difunctionalizations, J. Am. Chem. Soc., 2018, 140, 3331 CrossRef CAS.
- S. Yamasaki, M. Kanai and M. Shibasaki, Novel Multiaction of Zr Catalyst: One-Pot Synthesis of β-Cyanohydrins from Olefins, J. Am. Chem. Soc., 2001, 123, 1256 CrossRef CAS.
- D. C. Koester, M. Kobayashi, D. B. Werz and Y. Nakao, Intramolecular oxycyanation of alkenes by cooperative Pd/BPh3 catalysis, J. Am. Chem. Soc., 2012, 134, 6544 CrossRef CAS.
- Y. C. Yuan, H. B. Yang, X. Y. Tang, Y. Wei and M. Shi, Unprecedented Oxycyanation of Methylenecyclopropanes for the Facile Synthesis of Benzoxazine Compounds Containing a Cyano Group, Chem. – Eur. J., 2016, 22, 5146 CrossRef CAS.
- R. K. Quinn, V. A. Schmidt and E. J. Alexanian, Radical carbooxygenations of alkenes using hydroxamic acids, Chem. Sci., 2013, 4, 4030 RSC.
- F. Meng, H. Zhang, K. Guo, J. Dong, A. M. Lu and Y. Zhu, Access to Cyano-Containing Isoxazolines via Copper-Catalyzed Domino Cyclization/Cyanation of Alkenyl Oximes, J. Org. Chem., 2017, 82, 10742 CrossRef CAS.
- J.-L. Liu, Z.-F. Zhu and F. Liu, Oxycyanation of Vinyl Ethers with 2,2,6,6-Tetramethyl-N-oxopiperidinium Enabled by Electron Donor–Acceptor Complex, Org. Lett., 2018, 20, 720 CrossRef CAS.
-
(a) N. Zhu, B. Qian, H. Xiong and H. Bao, Copper-catalyzed regioselective allylic oxidation of olefins via C-H activation, Tetrahedron Lett., 2017, 58, 4125 CrossRef CAS;
(b) B. Qian, H. Xiong, N. Zhu, C. Ye, W. Jian and H. Bao, Copper-catalyzed diesterification of 1,3-diene for the synthesis of allylic diester compounds, Tetrahedron Lett., 2016, 57, 3400 CrossRef CAS;
(c) Y. Zeng, M. F. Chiou, X. Zhu, J. Cao, D. Lv, W. Jian, Y. Li, X. Zhang and H. Bao, Copper-Catalyzed Enantioselective Radical 1,4-Difunctionalization of 1,3-Enynes, J. Am. Chem. Soc., 2020, 142, 18014 CrossRef CAS.
-
(a) F. Wang, D. Wang, X. Mu, P. Chen and G. Liu, Copper-catalyzed intermolecular trifluoromethylarylation of alkenes: Mutual activation of arylboronic acid and CF3+ reagent, J. Am. Chem. Soc., 2014, 136, 10202 CrossRef CAS;
(b) X. Sun, X. Li, S. Song, Y. Zhu, Y.-F. Liang and N. Jiao, Mn-Catalyzed Highly Efficient Aerobic Oxidative Hydroxyazidation of Olefins: A Direct Approach to beta-Azido Alcohols, J. Am. Chem. Soc., 2015, 137, 6059 CrossRef CAS.
-
(a) W. Zhang, F. Wang, S. D. McCann, D. Wang, P. Chen, S. S. Stahl and G. Liu, Enantioselective cyanation of benzylic C-H bonds via copper-catalyzed radical relay, Science, 2016, 353, 1014 CrossRef CAS;
(b) F. Wang, P. Chen and G. Liu, Copper-Catalyzed Radical Relay for Asymmetric Radical Transformations, Acc. Chem. Res., 2018, 51, 2036 CrossRef CAS;
(c) F. D. Lu, D. Liu, L. Zhu, L. Q. Lu, Q. Yang, Q. Q. Zhou, Y. Wei, Y. Lan and W. J. Xiao, Asymmetric Propargylic Radical Cyanation Enabled by Dual Organophotoredox and Copper Catalysis, J. Am. Chem. Soc., 2019, 141, 6167 CrossRef CAS;
(d) M. Israr, H. Xiong, Y. Li and H. Bao, Copper–Catalyzed Enantioselective Cyano(Fluoro)Alkylation of Alkenes, Adv. Synth. Catal., 2020, 362, 2211 CrossRef CAS.
- A. L. Fuentes de Arriba, E. Lenci, M. Sonawane, O. Formery and D. J. Dixon, Iridium-Catalyzed Reductive Strecker Reaction for Late-Stage Amide and Lactam Cyanation, Angew. Chem., Int. Ed., 2017, 56, 3655 CrossRef CAS.
-
(a) G. Moad, E. Rizzardo and D. H. Solomon, On the regioselectivity of free radical processes; reactions of benzoyloxy, phenyl and t-butoxy radicals with some α,β-unsaturated esters, Aust. J. Chem., 1983, 36, 1573 CrossRef CAS;
(b) X. Zhu, C. Ye, Y. Li and H. Bao, Iron-Catalyzed Radical Decarboxylative Oxyalkylation of Terminal Alkynes with Alkyl Peroxides, Chem. – Eur. J., 2017, 23, 10254 CrossRef CAS;
(c) W. A. Pryor, J. T. Gu and D. F. Church, Trapping free radicals formed in the reaction of ozone with simple olefins using 2,6-di-tert-butyl-4-cresol (BHT), J. Org. Chem., 1985, 50, 185 CrossRef CAS;
(d) H. Zhou, W. Jian, B. Qian, C. Ye, D. Li, J. Zhou and H. Bao, Copper-Catalyzed Ligand-Free Diazidation of Olefins with TMSN3 in CH3CN or in H2O, Org. Lett., 2017, 19, 6120 CrossRef CAS.
-
(a) D. Griller and K. U. Ingold, Free-radical clocks, Acc. Chem. Res., 2002, 13, 317 CrossRef;
(b) W. Deng, W. Feng, Y. Li and H. Bao, Merging Visible-Light Photocatalysis and Transition-Metal Catalysis in Three-Component Alkyl-Fluorination of Olefins with a Fluoride Ion, Org. Lett., 2018, 20, 4245 CrossRef CAS.
-
(a) E. Vismara, A. Donna, F. Minisci, A. Naggi, N. Pastori and G. Torri, Reactivity of carbohydrate radicals derived from iodo sugars and dibenzoyl peroxide. Homolytic heteroaromatic and aromatic substitution, reduction, and oxidation, J. Org. Chem., 1993, 58, 959 CrossRef CAS;
(b) C. Qian, D. Lin, Y. Deng, X. Q. Zhang, H. Jiang, G. Miao, X. Tang and W. Zeng, Palladium-catalyzed ortho-functionalization of azoarenes with aryl acylperoxides, Org. Biomol. Chem., 2014, 12, 5866 RSC.
- X. Zhu, W. Deng, M. F. Chiou, C. Ye, W. Jian, Y. Zeng, Y. Jiao, L. Ge, Y. Li, X. Zhang and H. Bao, Copper-Catalyzed Radical 1,4-Difunctionalization of 1,3-Enynes with Alkyl Diacyl Peroxides and N-Fluorobenzenesulfonimide, J. Am. Chem. Soc., 2019, 141, 548 CrossRef CAS.
-
(a) Q. Zhang, Y. Liu, T. Wang, X. Zhang, C. Long, Y. D. Wu and M. X. Wang, Mechanistic Study on Cu(II)-Catalyzed Oxidative Cross-Coupling Reaction between Arenes and Boronic Acids under Aerobic Conditions, J. Am. Chem. Soc., 2018, 140, 5579 CrossRef CAS;
(b) S. D. McCann and S. S. Stahl, Copper-Catalyzed Aerobic Oxidations of Organic Molecules: Pathways for Two-Electron Oxidation with a Four-Electron Oxidant and a One-Electron Redox-Active Catalyst, Acc. Chem. Res., 2015, 48, 1756 CrossRef CAS.
Footnote |
† Electronic supplementary information (ESI) available. CCDC 2040065. For ESI and crystallographic data in CIF or other electronic format see DOI: 10.1039/d0qo01437k |
|
This journal is © the Partner Organisations 2021 |
Click here to see how this site uses Cookies. View our privacy policy here.