DOI:
10.1039/D1QO00290B
(Research Article)
Org. Chem. Front., 2021,
8, 2429-2433
Palladium-catalyzed 1,2-amino carbonylation of 1,3-dienes with (N-SO2Py)-2-iodoanilines: 2,3-dihydroquinolin-4(1H)-ones synthesis†
Received
18th February 2021
, Accepted 12th March 2021
First published on 16th March 2021
Abstract
A palladium-catalyzed 1,2-amino carbonylation of 1,3-dienes with (N-SO2Py)-2-iodoanilines has been developed for the construction of 2,3-dihydroquinolin-4(1H)-one scaffolds. Using benzene-1,3,5-triyl triformate (TFBen) as the CO source under the assistance of the N-SO2Py directing group, the reaction proceeded well to afford various 2,3-dihydroquinolin-4(1H)-ones in good yields (up to 88%). The effect of the directing groups was investigated and control experiments were performed to have a better understanding of the reaction pathway.
Introduction
1,3-Dienes represent a class of important building blocks in organic synthesis and are prevalent structural motifs in natural products.1 Difunctionalization of 1,3-dienes provides an efficient and straightforward method to rapidly assemble complexed molecules.2 Among them, pioneered by Dieck and Larock,3,4 metal-catalyzed 1,2-carboamination of 1,3-dienes have been extensively studied for the synthesis of diverse N-containing heterocyclic compounds.5–8 For instance, Yang and Xing developed a palladium-catalyzed intramolecular 1,2-aminoalkylation of β-ketoamide-tethered 1,3-dienes for the synthesis of pyrrolizidine derivatives.5 In 2016, Han's group disclosed an enantioselective annulation of 1,3-dienes by 2-iodoanilines in the presence of the palladium catalyst to give a series of chiral indolines.6 Very recently, Gevorgyan and co-workers realized the photoinduced palladium-catalyzed intermolecular 1,2-aminoalkylation of 1,3-dienes, furnishing a variety of allylic amines.7 However, to the best of our knowledge, there have been no reports on 1,2-amino carbonylation of 1,3-dienes for the expeditious construction of 2,3-dihydroquinolin-4(1H)-one scaffolds, which are frequently found in various molecules with biological activities such as anti-tumor9 and analgesic effects.10
Transition-metal-catalyzed carbonylative reactions have become one of the most powerful tools to access valuable carbonyl-containing compounds.11 TFBen, a benign CO surrogate developed by our group, has been widely employed in carbonylative processes.12 As a part of our continued interest in carbonylation, we now report a palladium-catalyzed carbonylation of (N-SO2Py)-2-iodoanilines and 1,3-dienes. With the assistance of the N-SO2Py directing group, 1,3-dienes could undergo 1,2-amino carbonylation with (N-SO2Py)-2-iodoanilines to give various 2,3-dihydroquinolin-4(1H)-ones in good yields by using TFBen as the CO source.
Results and discussion
Initially, (N-SO2Py)-2-iodoaniline 1a and diene 2a were treated with TFBen in the presence of Pd(OAc)2 as the catalyst and various phosphine ligands at 110 °C for 24 h (Table 1, entries 1–5). To our delight, the reaction with DPPP gave the desired product 3aa in 39% yield (Table 1, entry 4). Then, a set of palladium catalysts was screened (Table 1, entries 6–10). It was shown that the reaction yield was significantly improved to 60% when Pd(TFA)2 was employed (Table 1, entry 7). Furthermore, much lower yields of 3aa were obtained when other solvents such as toluene, dioxane, MeCN, and DMSO were tested (Table 1, entries 11–14). It was noted that extending the reaction time enhanced the yield to 79% (Table 1, entry 15). Finally, a few bases were investigated and the reaction with K3PO4 gave 84% yield of 3aa (Table 1, entry 17). Additionally, reducing the catalyst loading hampered the reaction remarkably (Table 1, entry 19). Notably, 36% yield of the target product can be obtained with a CO balloon instead of TFBen. Here, the requisite amount of TFBen is for maintaining a certain pressure of CO in the reaction tube, as carbonylation and decarbonylation are reversible. The formation of CO gas was confirmed using a CO detector.
Table 1 Screening of the reaction conditionsa
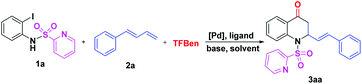
|
Entry |
[Pd] |
Ligand |
Base |
Solvent |
Yield (%) |
Reaction conditions: 1a (0.5 mmol, 1.0 equiv.), 2a (1.5 mmol, 3.0 equiv.), Pd catalyst (20 mol%), ligand (20 mol%), TFBen (2.0 mmol, 4.0 equiv.), base (1.0 mmol, 2.0 equiv.), solvent (3.0 mL), 110 °C, 24 h, isolated yield.
30 h.
Pd catalyst (10 mol%).
|
1 |
Pd(OAc)2 |
PPh3 |
Et3N |
THF |
n.r. |
2 |
Pd(OAc)2 |
PCy3 |
Et3N |
THF |
n.r. |
3 |
Pd(OAc)2 |
Xphos |
Et3N |
THF |
n.r. |
4 |
Pd(OAc)2 |
DPPP |
Et3N |
THF |
39 |
5 |
Pd(OAc)2 |
DPPB |
Et3N |
THF |
Trace |
6 |
Pd(acac)2 |
DPPP |
Et3N |
THF |
10 |
7 |
Pd(TFA)2 |
DPPP |
Et3N |
THF |
60 |
8 |
Pd(PPh3)4 |
DPPP |
Et3N |
THF |
31 |
9 |
Pd2(dba)3 |
DPPP |
Et3N |
THF |
23 |
10 |
PdCl2 |
DPPP |
Et3N |
THF |
20 |
11 |
Pd(TFA)2 |
DPPP |
Et3N |
Toluene |
Trace |
12 |
Pd(TFA)2 |
DPPP |
Et3N |
Dioxane |
Trace |
13 |
Pd(TFA)2 |
DPPP |
Et3N |
MeCN |
40 |
14 |
Pd(TFA)2 |
DPPP |
Et3N |
DMSO |
n.r. |
15b |
Pd(TFA)2 |
DPPP |
Et3N |
THF |
79 |
16b |
Pd(TFA)2 |
DPPP |
DBU |
THF |
n.r. |
17
|
Pd(TFA)
2
|
DPPP
|
K
3
PO
4
|
THF
|
84
|
18b |
Pd(TFA)2 |
DPPP |
Cs2CO3 |
THF |
n.r. |
19b,c |
Pd(TFA)2 |
DPPP |
K3PO4 |
THF |
18 |
With the optimal reaction conditions in hand (Table 1, entry 17), the scope of (N-SO2Py)-2-iodoanilines 1 was examined (Scheme 1). For compounds bearing electron-donating or withdrawing substituents, the reaction gave the corresponding products 3ba–ia in good yields (75–86%). It was found that the reaction of the substrate with a phenyl unit gave product 3ja in 77% yield. Moreover, 86% yield of product 3ka was achieved when 4,5-dimethyl-(N-SO2Py)-2-iodoaniline 1k was subjected to the reaction system.
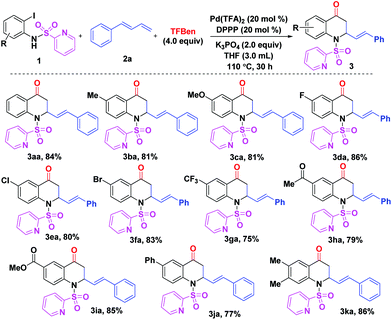 |
| Scheme 1 Scope of (N-SO2Py)-2-iodoanilines. Reaction condition: 1a (0.5 mmol, 1.0 equiv.), 2 (1.5 mmol, 3.0 equiv.), Pd(TFA)2 (20 mol%), DPPP (20 mol%), TFBen (2.0 mmol, 4.0 equiv.), K3PO4 (1.0 mmol, 2.0 equiv.), THF (3.0 mL), 110 °C, 30 h, isolated yield. | |
Subsequently, the scope of 1,3-dienes 2 was investigated as shown in Scheme 2. The reaction of the phenyl-substituted 1,3-dienes having para-electron-donating or withdrawing groups proceeded well to give the expected products 3ab–ai in high yields (70–86%). For the meta-substituted compounds 2j–l, the reaction led to the desired products 3aj–al in 70–84% yields. In addition, it was shown that ortho-substituted 1,3-dienes can also be successfully converted to product 3am and 3an in high yields. In addition, 88% yield of product 3ap was obtained in the reaction of the naphthyl-substituted 1,3-diene 2p. Notably, compound 2q containing a thiophene ring was smoothly transformed into the target product 3aq in 80% yield. Unfortunately, 1,1-disubsituted, 1,2-disubsituted, and alkyl-substituted 1,3-dienes 2r–t failed to undergo the designed reactions.
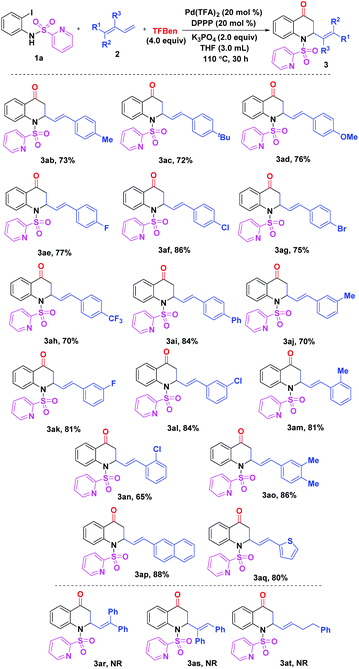 |
| Scheme 2 Scope of 1,3-dienes. Reaction condition: 1a (0.5 mmol, 1.0 equiv.), 2 (1.5 mmol, 3.0 equiv.), Pd(TFA)2 (20 mol%), DPPP (20 mol%), TFBen (2.0 mmol, 4.0 equiv.), K3PO4 (1.0 mmol, 2.0 equiv.), THF (3.0 mL), 110 °C, 30 h, isolated yield. | |
Gratifyingly, treatment of product 3aa with excess zinc powder in a mixed solvent (THF/NH4Cl(aq) = 1
:
1) at 60 °C for 12 h could smoothly remove the N-SO2Py directing group to give the corresponding free amino compound 4 in 88% yield. Therefore, further derivatizations on the N-position of 2,3-dihydroquinolin-4(1H)-one skeletons could be realized easily (Scheme 3, eqn (1)). In order to demonstrate the utility of this protocol, a few transformations of product 3aa were completed as well. When 3aa was reacted with hydroxylamine hydrochloride in the presence of pyridine at 80 °C for 10 h, 87% yield of the oxime 5 was obtained (Scheme 3, eqn (2)).14 Reduction of 3aa with NaBH4 afforded the alcohol 6 in 90% yield with 2.5
:
1 diastereoselectivity (Scheme 3, eqn (3)).15
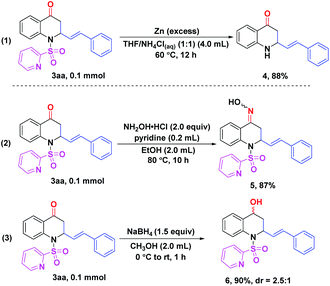 |
| Scheme 3 Removal of the N-SO2Py directing group and transformations of product 3aa. | |
Then, we examined the effect of the directing group on 2-iodoanilines and performed some control experiments as well to gain more insights into the reaction mechanism (Scheme 4). The reaction with the unprotected 2-iodoaniline 7 led to complicated mixtures with no observation of the expected product (Scheme 4, eqn (1)). It was shown that the reaction did not occur when N-Me-2-iodoaniline 8 or N-Boc-2-iodoaniline 9 was subjected to the standard conditions, respectively (Scheme 4, eqn (2) and (3)). Interestingly, N-picolinyl-2-iodoaniline 10 was converted to the corresponding Heck coupling product 11 in 47% yield and the target product 12 was not obtained (Scheme 4, eqn (4)). It suggested that the sulfuryl unit on the directing group was crucial in promoting the carbonylation. Notably, when the N-benzenesulfonyl-2-iodoaniline 13 was tested, complicated mixtures were obtained, which indicated that the N atom on N-SO2Py of 2-iodoanilines played an important role in this reaction (Scheme 4, eqn (5)). Furthermore, when 1a was treated with Pd(TFA)2 (1.0 equiv.) and PPh3 (2.0 equiv.) under the standard conditions, the acylpalladium intermediate 14 was obtained in 98% yield, whose structure was unambiguously confirmed by X-ray crystallography (CCDC 2058728, see the ESI†) (Scheme 4, eqn (6)).13 An attempt to obtain a similar complex with DPPP failed, perhaps due to its high reactivity. Finally, the reaction of 14 with the diene 2a in the presence of DPPP (1.0 equiv.) furnished the desired product 3aa in 27% yield with 56% of 14 recovered (Scheme 4, eqn (7)). These results revealed that a similar intermediate like 14 could be formed in this reaction through the coordination of the palladium catalyst with the N atom on N-SO2Py of 2-iodoanilines.
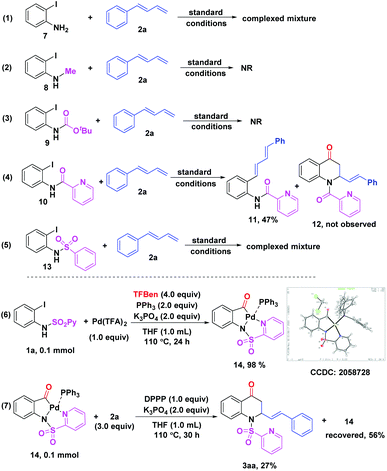 |
| Scheme 4 Effect of directing groups and control experiments. | |
On the basis of the previous reports3–5,8 and our experimental results, a plausible mechanism for this palladium-catalyzed 1,2-amino carbonylation of 1,3-dienes with (N-SO2Py)-2-iodoanilines is proposed (Scheme 5). Initially, the active Pd(0) species, generated from Pd(TFA)2 and DPPP, undergoes oxidative addition with (N-SO2Py)-2-iodoaniline 1a to afford Pd(II) complex A. Then, the insertion of CO which was released from TFBen to A with the aid of a base gives the acyl Pd(II) intermediate B. Subsequently, the π-allyl Pd(II) intermediate C is formed via the insertion of diene 2a to B and which is further converted into Pd(II) complex D. Finally, reductive elimination of D leads to the desired product 3aa and regenerates the active Pd(0) species for the next catalytic cycle.
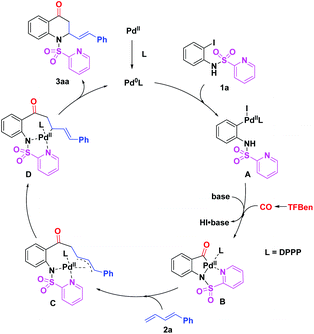 |
| Scheme 5 Plausible mechanism. | |
Conclusions
In conclusion, we have developed a facile and straightforward method to synthesize important 2,3-dihydroquinolin-4(1H)-one scaffolds via a palladium-catalyzed 1,2-amino carbonylation of 1,3-dienes with (N-SO2Py)-2-iodoanilines. This protocol can be realized to give various 2,3-dihydroquinolin-4(1H)-ones by utilizing TFBen as the CO source and N-SO2Py as the directing group.
Experimental
The (N-SO2Py)-2-iodoanilines 1 (0.5 mmol, 1.0 equiv.), Pd(TFA)2 (33.2 mg, 0.1 mmol, 20 mol%), dppp (41.2 mg, 0.1 mmol, 20 mol%), K3PO4 (212.3 mg, 1.0 mmol, 2.0 equiv.) and a 2.5 mL vial containing TFBen (420.3 mg, 2.0 mmol, 4.0 equiv.) were added to an oven-dried tube (15.0 mL) which was then placed under vacuum and refilled with nitrogen three times.16 The 1,3-dienes 2 (1.5 mmol, 3.0 equiv.) and THF (3.0 mL) were added into the tube via a syringe. The tube was sealed and stirred at 110 °C for 30 h. When the reaction was completed, the resulting mixture was concentrated under vacuum and purified by silica gel column using chromatography (petroleum ether/dichloromethane = 2
:
1) to obtain the products 3.
Conflicts of interest
There are no conflicts to declare.
Acknowledgements
We acknowledge the financial support from the Joint Funds of Zhejiang Natural Science Foundation and Taizhou (LTY21B020001).
Notes and references
- For selected reviews on 1,3-dienes, see:
(a) J.-E. Bäckvall, R. Chinchilla, C. Nájera and M. Yus, The use of sulfonyl 1,3-dienes in organic synthesis, Chem. Rev., 1998, 98, 2291–2312 CrossRef PubMed;
(b) M. Holmes, L. A. Schwartz and M. J. Krische, The intermolecular metal-catalyzed reductive coupling of dienes, allenes, and enynes with carbonyl compounds and imines, Chem. Rev., 2018, 118, 6026–6052 CrossRef CAS PubMed;
(c) Z. Xi, 1,4-Dilithio-1,3-dienes: reaction and synthetic applications, Acc. Chem. Res., 2010, 43, 1342–1351 CrossRef CAS PubMed;
(d) P. Hubert, E. Seibel, C. Beemelmanns, J.-M. Campagne and R. M. deFigueiredo, Stereoselective construction of (E,Z,)-1,3-dienes and its application in natural product synthesis, Adv. Synth. Catal., 2020, 362, 5532–5575 CrossRef CAS.
- For selected reviews on difunctionalization of 1,3-dienes, see:
(a) E. McNeill and T. Ritter, 1,4-Functionalization of 1,3-dienes with low-valent iron catalysts, Acc. Chem. Res., 2015, 48, 2330–2343 CrossRef CAS PubMed;
(b) B. N. Hemric, Beyond osmium: progress in 1,2-amino oxygenation of alkenes, 1,3-dienes, alkynes, and allenes, Org. Biomol. Chem., 2021, 19, 46–81 RSC;
(c) X. Wu and L.-Z. Gong, Palladium(0)-catalyzed difunctionalization of 1,3-dienes: from racemic to enantioselective, Synthesis, 2019, 51, 122–134 CrossRef CAS.
- J. M. O'Connor, B. J. Stallman, W. G. Clark, A. Y. L. Shu, R. E. Spada, T. M. Stevenson and H. A. Dieck, Some aspects of palladium-catalyzed reactions of aryl and vinylic halides with conjugated dienes in the presence of mild nucleophiles, J. Org. Chem., 1983, 48, 807–809 CrossRef.
-
(a) R. C. Larock and C. A. Fried, Palladium-catalyzed carboannulation of 1,3-dienes by aryl halides, J. Am. Chem. Soc., 1990, 112, 5882–5884 CrossRef CAS;
(b) R. C. Larock, N. Berrios-Peña and K. Narayanan, Palladium-catalyzed heteroannulation of 1,3-dienes by functionally substituted aryl halides, J. Org. Chem., 1990, 55, 3447–3450 CrossRef CAS.
- D. Xing and D. Yang, Pd(II)-catalyzed intramolecular 1,2-aminoalkylation of conjugated 1,3-dienes for the synthesis of pyrrolizidines, Org. Lett., 2013, 15, 4370–4373 CrossRef CAS PubMed.
- S.-S. Chen, J. Meng, Y.-H. Li and Z.-Y. Han, Palladium-catalyzed enantioselective heteroannulation of 1,3-dienes by functionally substituted aryl iodides, J. Org. Chem., 2016, 81, 9402–9408 CrossRef CAS PubMed.
- K. P. S. Cheung, D. Kurandina, T. Yata and V. Gevorgyan, Photoinduced palladium-catalyzed carbofunctionalization of conjugated dienes proceeding via radical-polar crossover scenario: 1,2-aminoalkylation and beyond, J. Am. Chem. Soc., 2020, 142, 9932–9937 CrossRef PubMed.
-
(a) C. E. Houlden, C. D. Bailey, J. G. Ford, M. R. Gagné, G. C. Lloyd-Jones and K. I. Booker-Milburn, Distinct reactivity of Pd(OTs)2: the intermolecular Pd(II)-catalyzed 1,2-carboamination of dienes, J. Am. Chem. Soc., 2008, 130, 10066–10067 CrossRef CAS PubMed;
(b) J. Yang, H. Mo, X. Jin, D. Cao, H. Wu, D. Chen and Z. Wang, Vinylogous elimination/Heck coupling/allylation domino reactions: access to 2–substituted 2,3-dihydrobenzofurans and indolines, J. Org. Chem., 2018, 83, 2592–2600 CrossRef CAS PubMed.
-
(a) Y. Xia, Z.-Y. Yang, P. Xia, K. F. Bastow, Y. Tachibana, S.-C. Kuo, E. Hamel, T. Hackl and K.-H. Lee, Synthesis and biological evaluation of 6,7,2′,3′,4′-substituted-1,2,3,4-tetrahydro-2-phenyl-4-quinolones as a new class of antimitotic antitumor agents, J. Med. Chem., 1998, 41, 1155–1162 CrossRef CAS PubMed;
(b) S.-X. Zhang, J. Feng, S.-C. Kuo, A. Brossi, E. Hamel, A. Tropsha and K.-H. Lee, Three-dimensional quantitative structure-activity relationship study of the colchicine binding site ligands using comparative molecular field analysis, J. Med. Chem., 2000, 43, 167–176 CrossRef CAS PubMed.
- M. S. Atwal, L. Bauer, S. N. Dixit, J. E. Gearien and R. W. Morris, Some substituted 2,3-dihydro-4-quinolones, J. Med. Chem., 1965, 8, 566–571 CrossRef CAS.
- For selected recent reviews on carbonylation reactions, see:
(a) J.-B. Peng, F.-P. Wu and X.-F. Wu, First-row transition-metal catalyzed carbonylative transformations of carbon electrophiles, Chem. Rev., 2019, 119, 2090–2127 CrossRef CAS PubMed;
(b) S. Zhao and N. P. Mankad, Metal-catalysed radical carbonylation reactions, Catal. Sci. Technol., 2019, 9, 3603–3613 RSC;
(c) J. Cao, Z.-J. Zheng, Z. Xu and L.-W. Xu, Transition-metal-catalyzed transfer carbonylation with HCOOH or HCHO as non-gaseous C1 source, Coord. Chem. Rev., 2017, 336, 43–53 CrossRef CAS;
(d) S. D. Friis, A. T. Lindhardt and T. Skrydstrup, The development and application of two-chamber reactors and carbon monoxide precursors for safe carbonylation reactions, Acc. Chem. Res., 2016, 49, 594–605 CrossRef CAS PubMed;
(e) P. Gautam and B. M. Bhanage, Recent advances in the transition metal catalyzed carbonylation of alkynes, arenes and aryl halides using CO surrogates, Catal. Sci. Technol., 2015, 5, 4663–4702 RSC;
(f) S. Sumino, A. Fusano, T. Fukuyama and I. Ryu, Carbonylation reactions of alkyl iodides through the interplay of carbon radicals and Pd catalysts, Acc. Chem. Res., 2014, 47, 1563–1574 CrossRef CAS PubMed.
-
(a) Q. Gao, J.-M. Lu, L. Yao, S. Wang, J. Ying and X.-F. Wu, Cobalt-catalyzed direct C-H carbonylative synthesis of free (NH)–indolo[1,2–a]quinoxalin-6(5H)–ones, Org. Lett., 2021, 23, 178–182 CrossRef CAS PubMed;
(b) J. Ying, Z. Le and X.-F. Wu, Benzene-1,3,5-triyl triformate (TFBen)-promoted palladium-catalyzed carbonylative synthesis of 2–oxo-2,5-dihydropyrroles from propargyl amines, Org. Lett., 2020, 22, 194–198 CrossRef CAS PubMed;
(c) J. Ying, L.-Y. Fu, G. Zhong and X.-F. Wu, Cobalt-catalyzed direct carbonylative synthesis of free (NH)–benzo[cd]indol-2(1H)–ones from naphthylamides, Org. Lett., 2019, 21, 5694–5698 CrossRef CAS PubMed;
(d) J. Ying, Q. Gao and X.-F. Wu, Site-selective carbonylative synthesis of structurally diverse lactams from heterocyclic amines with TFBen as the CO source, J. Org. Chem., 2019, 84, 14297–14305 CrossRef CAS PubMed;
(e) L.-Y. Fu, J. Ying and X.-F. Wu, Cobalt-catalyzed carbonylative synthesis of phthalimides from N–(pyridin-2-ylmethyl)benzamides with TFBen as the CO source, J. Org. Chem., 2019, 84, 1421–1429 CrossRef CAS PubMed;
(f) L.-Y. Fu, J. Ying, X. Qi, J.-B. Peng and X.-F. Wu, Palladium-catalyzed carbonylative synthesis of isoindolinones from benzylamines with TFBen as the CO source, J. Org. Chem., 2019, 84, 1421–1429 CrossRef CAS PubMed.
- CCDC 2058728† contains the supplementary crystallographic data for this paper.
- X. Peng, B. M. K. Tong, H. Hirao and S. Chiba, Inorganic-base-mediated hydroamination of alkenyl oximes for the synthesis of cyclic nitrones, Angew. Chem., Int. Ed., 2014, 53, 1959–1962 CrossRef CAS PubMed.
- J. Liang, G. Huang, P. Peng, T. Zhang, J. Wu and F. Wu, Palladium-catalyzed benzodifluoroalkylation of alkynes: a route to fluorine-containing 1,1-diarylethylenes, Adv. Synth. Catal., 2018, 360, 2221–2227 CrossRef CAS.
- Z. Yin and X. F. Wu, The Design of an In-Ex Tube for Gas Related Organic Reactions, Org. Process Res. Dev., 2017, 21, 1869–1871 CrossRef CAS.
Footnote |
† Electronic supplementary information (ESI) available: General comments, general procedure, analytic data, and NMR spectra. CCDC 2058728. For ESI and crystallographic data in CIF or other electronic format see DOI: 10.1039/d1qo00290b |
|
This journal is © the Partner Organisations 2021 |