DOI:
10.1039/D1QO00611H
(Research Article)
Org. Chem. Front., 2021,
8, 4095-4100
Visible-light-induced radical cascade cyclization of 1-(allyloxy)-2-(1-arylvinyl)benzenes with sulfonyl chlorides for the synthesis of sulfonated benzoxepines†
Received
20th April 2021
, Accepted 27th May 2021
First published on 2nd June 2021
Abstract
A visible light photocatalyzed cascade sulfonylation/cyclization of 1-(allyloxy)-2-(1-arylvinyl)benzenes with sulfonyl chlorides for the construction of sulfonated benzoxepines is developed. In the presence of Eosin Y (2.0 mol%) as a photocatalyst and Na2CO3 as a base, the reactions proceed smoothly to afford seven-membered rings in good yields. This transformation features wide substrate scope, the use of easily accessible materials and excellent functional group tolerance.
Benzoxepines are ubiquitous oxygen-containing heterocycles widely found in a variety of natural alkaloids and biologically active compounds, such as pterulone, IV ptaeroxylin and bauhinoxepin A (Scheme 1a).1 Therefore, the development of efficient and straightforward synthetic strategies to easily assemble benzoxepine scaffolds has gained substantial attention. In the past decade, various synthetic methodologies have been established for the construction of the benzoxepine skeleton.2 Among the elegant strategies for the synthesis of benzoxepines are transition-metal-catalyzed intramolecular cyclization reactions3 and transition-metal-catalyzed intermolecular [m + n]-cyclization reactions.4 For example, Saá and co-workers reported an osmium-catalyzed regioselective 7-endo heterocyclization of aromatic alkynols to form benzoxepines in 2010.3a Very recently, the Ruijter group employed a Pd-catalyzed intermolecular cascade [4 + 3] cyclocondensation of salicylaldehydes with vinylcyclopropanes for the synthesis of benzoxepines.4b It should be noted that many organic chemists, for example Heo's,5a Reddy's,5b Jiang's,5c and other groups,5d,e have reported the synthesis of these seven-membered heterocyclic compounds under transition-metal-free conditions. In contrast, radical methodologies for the construction of benzoxepines have been much less well-developed. For instance, in 2018, Yang and co-workers developed a visible light induced cascade cyclization of (E)-1-(2-(allyloxy)phenyl)-3-(dimethylamino)-prop-2-en-1-ones with 2-bromo-2,2-difluoro-acetic acid ethyl ester (BrCF2COOEt), generating functionalized CF2-containing benzoxepine derivatives.6 Despite these significant advances, the exploration of an efficient, convenient and ecofriendly approach to diverse functionalized benzoxepines is still of importance.
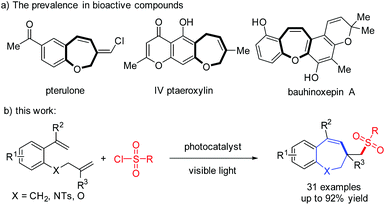 |
| Scheme 1 (a) Examples of biologically active compounds containing the benzoxepine scaffold; (b) Our work concept. | |
Organosulfone compounds have found extensive use in organic and medicinal chemistry.7 Indeed, heterocyclic compounds containing a sulfone group exhibit unique chemical activities and biological properties.8 Therefore, developing a new, sustainable and straightforward protocol for the construction of sulfone-containing compounds is considered highly desirable.9 Sulphonyl chlorides are commercially available reagents and relatively stable, and have been widely used as sulfonylation agents to synthesize organosulfones.10 To date, there has been a lack of methods for the construction of sulfonated benzoxepines via sulfonylation and cyclization of 1-(allyloxy)-2-(1-arylvinyl)benzenes with sulfonyl radicals, which are formed from sulphonyl chlorides.
Visible light induced photoredox catalysis has been a powerful tool for synthetic conversions in organic synthesis due to its safety, high efficiency, operational simplicity and environmental friendliness.11 Very recently, our group reported a visible-light-induced synthesis of 4-sulfonated cyclopenta[gh]phenanthridines via radical cyclization of 3-(arylethynyl)-[1,1′-biphenyl]-2-carbonitriles.11k In continuation of our interest in the construction of sulfone-containing compounds,12 we reported herein the visible-light-induced cascade sulfonylation/cyclization of 1-(allyloxy)-2-(1-arylvinyl)benzenes with sulfonyl chlorides for the construction of sulfonated benzoxepines (Scheme 1b).
Initially, we chose 1-(allyloxy)-2-(1-phenylvinyl)benzene 1a and p-tosyl chloride 2a as model substrates to identify the optimal reaction conditions. As shown in Table 1, the reaction employing fac-Ir(ppy)3 as a photocatalyst, K2CO3 as a base, MeCN as a solvent and irradiation with a 12 W blue LED light source at 100 °C under an argon atmosphere for 18 h can afford the product 3aa in 58% yield. Several photoredox catalysts such as Ru(bpy)3(PF6)2 and Eosin Y were evaluated, and Eosin Y could afford a higher yield than fac-Ir(ppy)3 (entries 2 and 3). Then, a series of bases such as NaHCO3, NaOAc, Na2HPO4, Na2CO3, Et3N and 2,6-lutidine were examined (entries 4–9), and Na2CO3 was determined to be the best choice (entry 7). Several other solvents such as DCM, THF, DMF and DMSO were screened, and none of them could afford higher yields than MeCN (entries 10–13). When the reaction was conducted at room temperature, product 3aa was obtained (entry 14). Raising the reaction temperature or decreasing the reaction temperature did not give a better result (entries 15 and 16). When a 33 W fluorescent light bulb was used as a light source, 3aa was obtained in 83% yield (entry 17). Using 5 W blue LED light instead of 12 W blue LED light also could not improve the reaction yield (entry 18). Finally, the desired product 3aa was not obtained when the reaction was conducted in the absence of Eosin Y or visible light (entries 19 and 20), and only 16% yield of 3aa was detected without a base (entry 21).
Table 1 Optimization of reaction conditionsa

|
Entry |
Photocatalyst |
Base |
Solvent |
Yieldb (%) |
Reaction conditions: 1a (0.1 mmol), 2a (0.2 mmol), base (0.25 mmol), photocatalyst (2 mol%), solvent (1 mL), a 12 W blue LED under Ar at 100 °C for 18 h.
Isolated yield.
Reaction temperature: 25 °C.
Reaction temperature: 80 °C.
Reaction temperature: 120 °C.
Using a 33 W fluorescent light bulb.
Using a 5 W blue LED light.
In the dark.
|
1 |
fac-Ir(ppy)3 |
K2CO3 |
MeCN |
58 |
2 |
Ru(bpy)3(PF6)2 |
K2CO3 |
MeCN |
10 |
3 |
Eosin Y |
K2CO3 |
MeCN |
67 |
4 |
Eosin Y |
NaHCO3 |
MeCN |
66 |
5 |
Eosin Y |
NaOAc |
MeCN |
57 |
6 |
Eosin Y |
Na2HPO4 |
MeCN |
70 |
7 |
Eosin Y |
Na2CO3 |
MeCN |
88 |
8 |
Eosin Y |
Et3N |
MeCN |
35 |
9 |
Eosin Y |
2,6-Lutidine |
MeCN |
58 |
10 |
Eosin Y |
Na2CO3 |
DCM |
53 |
11 |
Eosin Y |
Na2CO3 |
THF |
41 |
12 |
Eosin Y |
Na2CO3 |
DMF |
12 |
13 |
Eosin Y |
Na2CO3 |
DMSO |
15 |
14c |
Eosin Y |
Na2CO3 |
MeCN |
0 |
15d |
Eosin Y |
Na2CO3 |
MeCN |
75 |
16e |
Eosin Y |
Na2CO3 |
MeCN |
84 |
17f |
Eosin Y |
Na2CO3 |
MeCN |
83 |
18g |
Eosin Y |
Na2CO3 |
MeCN |
78 |
19h |
Eosin Y |
Na2CO3 |
MeCN |
0 |
20 |
— |
Na2CO3 |
MeCN |
0 |
21 |
Eosin Y |
— |
MeCN |
16 |
With the optimized conditions in hand, we then investigated the scope of sulfonyl chlorides 2 with 1-(allyloxy)-2-(1-phenylvinyl)benzene 1a for the synthesis of sulfonated benzoxepines. As shown in Table 2, arylsulfonyl chlorides bearing both electron-donating (MeO and tBu) and electron-withdrawing (F, Cl and Br) groups at the para-position of the aromatic ring underwent this transformation smoothly, and the desired products 3ab–3af were obtained in 78–85% yields. The meta- and ortho-substituted arylsulfonyl chlorides (2g and 2h) afforded the corresponding products (3ag and 3ah) in 84% and 76% yield, respectively. 4-Nitrobenzenesulfonyl chloride (2i) was compatible with the standard reaction conditions, providing the corresponding product 3ai in 77% yield. In addition, benzenesulfonyl chloride (2j) was also suitable for this conversion to give the desired product 3aj in good yield. Moreover, naphthalene-1-sulfonyl chloride (2k), naphthalene-2-sulfonyl chloride (2l) and thiophene-2-sulfonyl chloride (2m) were good sulfonating agents, delivering the corresponding benzoxepines 3ak–3am in 63% to 75% yields. Furthermore, aliphatic sulfonyl chlorides, such as methanesulfonyl chloride and cyclopropanesulfonyl chloride, were also proved to be viable substrates for this conversion, providing the desired products 3an and 3ao in 36% and 70% yields, respectively.
Table 2 Scope of sulfonyl chloridesa
Reaction conditions: 1a (0.1 mmol), 2 (0.2 mmol), Na2CO3 (0.25 mmol), Eosin Y (2 mol%), MeCN (1 mL), 12 W blue LEDs under Ar at 100 °C for 18 h; isolated yields.
5 mmol scale.
|
|
Next, the scope of the reaction with various 1-(allyloxy)-2-(1-arylvinyl)benzenes 1 was examined, as shown in Table 3. First, we investigated the effect of the substitution pattern on a benzene ring tethered alkene. The substrates bearing both electron-donating (Me) and electron-withdrawing (Br and CF3) groups at the 2 or 4 positions of the phenyl ring could be engaged in this reaction and gave the desired 3ba–3ea in 73–86% yields. Subsequently, we investigated the effect of the substitution pattern on the aromatic ring of the phenol moiety. This cascade reaction was tolerant of electron-donating (Me and MeO) and electron-withdrawing (F, Cl and Br) groups at the 4 or 5 positions of the phenol moiety, and the desired sulfonated benzoxepines 3fa–3ka were obtained in moderate to good yields, suggesting that the position of the substituent on the aryl ring has limited effects on the reaction outcome. The structure of 3ka was confirmed by single-crystal X-ray analysis (see the ESI† for details). Furthermore, substrates bearing disubstituted groups, such as 4,6-ditert-butyl and 4,6-dichloro groups, were also suitable for this cascade cyclization, producing 3la and 3ma in 68% and 65% yields, respectively. Gratifyingly, 2-(allyloxy)-1-(1-phenylvinyl)naphthalene (1n) was also compatible with the optimized conditions, and the desired product 3na was obtained in 56% yield. It should be noted that 1-((2-methylallyl)oxy)-2-(1-phenylvinyl)benzene (1o) was used to react with 2a, affording the product 3oa in good yield. Unfortunately, when R2 is a methyl group, no desired product 3pa was detected probably due to the lower stability of the benzyl radical intermediate. To our delight, N-allyl-4-methyl-N-(2-(1-phenylvinyl)phenyl)benzenesulfonamide 1q and 1-(but-3-en-1-yl)-2-(1-phenylvinyl)benzene 1r were also amenable to this transformation, giving the products 3qa and 3ra in 89% and 65% yields, respectively.
Table 3 Scope of 1-(allyloxy)-2-(1-arylvinyl)benzenesa
Reaction conditions: 1 (0.1 mmol), 2a (0.2 mmol), base (0.25 mmol), Eosin Y (2 mol%), MeCN (1 mL), 12 W blue LEDs under Ar at 100 °C for 18 h; isolated yields.
|
|
To understand the reaction mechanism, several radical trapping experiments were conducted, as shown in Scheme 2; when a radical scavenger such as TEMPO (2.5 equiv.) was added to the reaction, the reaction was completely inhibited and the TEMPO-Ts adduct 4 was detected by HRMS. When 1,4-dinitrobenzene was added to this reaction system, the desired product 3aa was not detected. The experimental results suggested that a radical process might be involved in this transformation (see the ESI† for details).
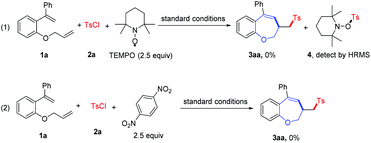 |
| Scheme 2 Control experiment. | |
According to the presented results and literature,10 a plausible reaction mechanism was proposed, as shown in Scheme 3. Initially, the excited state Eosin Y* was formed under visible-light irradiation, and it was further oxidized by p-tosyl chloride 2a to generate Eosin Y+˙ and radical anion I. The radical anion I undergoes decomposition to generate the corresponding sulfonyl radical II, which causes an addition to the C
C bond of 1a to afford the alkyl radical III. Then, an intramolecular addition of radical III to the other C
C bond leads to benzyl radical intermediate IV. Subsequently, the radical IV is oxidized by Eosin Y+˙ to form intermediate V with the concurrent regeneration of Eosin Y. Finally, a deprotonation step occurred to provide product 3aa.
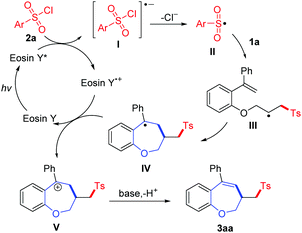 |
| Scheme 3 Proposed mechanism. | |
In summary, we have successfully developed a facile and efficient protocol for the synthesis of sulfonated benzoxepine derivatives via a visible light induced cascade sulfonylation/cyclization process involving the reaction of the easily prepared 1-(allyloxy)-2-(1-arylvinyl)benzenes with sulfonyl chlorides. This protocol featured wide substrate scope, excellent functional group tolerance and good yields of products, providing a general approach toward sulfonated benzoxepines. Further reaction mechanism studies and applications of 1-(allyloxy)-2-(1-arylvinyl)benzenes are underway in our laboratory.
Conflicts of interest
There are no conflicts to declare.
Acknowledgements
We gratefully acknowledge the National Natural Science Foundation of China (21901006) and the Anhui Provincial Natural Science Foundation (1908085QB80). We thank Dr Yun Wei (AHNU) for her help with the X-ray crystallographic analyses.
Notes and references
-
(a) M. Engler, T. Anke and O. Sterner, Pterulinic acid and pterulone, two novel inhibitors of NADH: ubiquinone oxidoreductase (complex I) produced by a pterula species, J. Antibiot., 1997, 50, 330–333 Search PubMed
;
(b) S. Kim, B.-N. Su, S. Riswan, L. B. S. Kardono, J. J. Afriastini, J. C. Galluci, H. Chai, N. R. Farnswerth, G. A. Cordell, S. M. Swanson and A. D. Kinghorn, Edulisones A and B, two epimeric benzo[b]oxepine derivatives from the bark of Aglaia edulis, Tetrahedron Lett., 2005, 46, 9021–9024 Search PubMed
;
(c) I. Barrett, M. J. Meegan, R. B. Hughes, M. Carr, A. J. Knox, N. Artemenko, G. Golfis, D. M. Zisterer and D. G. Lloyd, Synthesis, biological evaluation, structural–activity relationship, and docking study for a series of benzoxepin-derived estrogen receptor modulators, Bioorg. Med. Chem., 2008, 16, 9554–9573 Search PubMed
;
(d) J. R. Vyvyan and R. E. Looper, Total synthesis of (±)-heliannuol D, an allelochemical from Helianthus annuus, Tetrahedron Lett., 2000, 41, 1151–1154 Search PubMed
.
-
(a) J. O. Hoberg, Synthesis of seven-membered oxacycles, Tetrahedron, 1998, 54, 12631–12670 Search PubMed
;
(b) N. L. Snyder, H. M. Haines and M. W. Peczuh, Recent developments in the synthesis of oxepines, Tetrahedron, 2006, 62, 9301–9320 Search PubMed
;
(c) P. W. H. Chan, W. T. Teo, S. W. Y. Koh, B. R. Lee, B. J. Ayers, D.-L. Ma and C.-H. Leung, Silver triflate catalyzed cyclopropyl carbinol rearrangement for benzo[b]oxepine and 2H-chromene synthesis, Eur. J. Org. Chem., 2015, 4447–4456 Search PubMed
.
-
(a) A. Varela-Fernández, C. García-Yebra, J. A. Varela, M. A. Esteruelas and C. Saá, Osmium-catalyzed 7-endo heterocyclization of aromatic alkynols into benzoxepines, Angew. Chem., Int. Ed., 2010, 49, 4278–4281 Search PubMed
;
(b) M. M. Coulter, P. K. Dornan and V. M. Dong, Rhcatalyzed intramolecular olefin hydroacylation: enantioselective synthesis of seven- and eight-membered heterocycles, J. Am. Chem. Soc., 2009, 131, 6932–6933 Search PubMed
;
(c) E. D. D. Calder, S. A. I. Sharif, F. I. McGonagle and A. Sutherland, One-pot synthesis of 5-amino-2,5-dihydro-1-benzoxepines: access to pharm-acologically active heterocyclic scaffolds, J. Org. Chem., 2015, 80, 4683–4696 Search PubMed
;
(d) J. Liu and Y. Liu, Gold-catalyzed cyclizations of (o-alkynyl)phenoxyacrylates with external nucleophiles: regio- and stereoselective synthesis of functionalized benzo[b]oxepines, Org. Lett., 2012, 14, 4742–4745 Search PubMed
.
-
(a) M. Lautens, J.-F. Paquin and S. Piguel, Palladium-catalyzed sequential alkylation-alkenylation reactions. application to the synthesis of 2-substituted-4-benzoxepines and 2,5-disubstituted-4- benzoxepines, J. Org. Chem., 2002, 67, 3972–3974 Search PubMed
;
(b) M. Faltracco, K. N. A. Vrande, M. Dijkstra, J. M. Saya, T. A. Hamlin and E. Ruijter, Pd-catalyzed cascade to benzoxepins by using vinyl-substituted donoracceptor cyclopropanes, Angew. Chem., Int. Ed., 2021 DOI:10.1002/anie.202102862
;
(c) X. Yu and X. Lu, Cationic palladiumcatalyzed [5 + 2] annulation of 2-acylmethoxyaryl-boronic acids and allenoates: synthesis of 1-benzoxepine derivatives, J. Org. Chem., 2011, 76, 6350–6355 Search PubMed
;
(d) A. Seoane, N. Casanova, N. Quiñones, J. L. Mascareñas and M. Gulías, Straightforward assembly of benzoxepines by means of a rhodium(III)-catalyzed C–H functionalization of o-vinylphenols, J. Am. Chem. Soc., 2014, 136, 834–837 Search PubMed
.
-
(a) Y. L. Choi, H. S. Lim, H. J. Lim and J.-N. Heo, One-pot transition-metal-free synthesis of dibenzo[b,f]oxepins from 2-halobenzaldehydes, Org. Lett., 2012, 14, 5102–5105 Search PubMed
;
(b) S. J. Gharpure and S. R. B. Reddy, Stereoselective synthesis of benzoxepines through tandem alkylation/michael addition to vinylogous carbonates, Eur. J. Org. Chem., 2013, 2981–2984 Search PubMed
;
(c) L. Ouyang, C. Qi, H. He, Y. Peng, W. Xiong, Y. Ren and H. Jiang, Base-promoted formal [4 + 3] annulation between 2-fluorophenylacetylenes and ketones: a route to benzoxepines, J. Org. Chem., 2016, 81, 912–919 Search PubMed
;
(d) J. Xu, S. Yuan, J. Peng, M. Miao, Z. Chen and H. Ren, Base-mediated diastereoselective [4 + 3] annulation of in situ generated ortho-quinone methides with C,N-cyclic azomethine imines, Org. Biomol. Chem., 2017, 15, 7513–7517 Search PubMed
;
(e) Y. Zhang, A. Yu, J. Jia, S. Ma, K. Li, Y. Wei and X. Meng, NaH promoted [4 + 3] annulation of crotonate-derived sulfur ylides with thioaurones: synthesis of 2,5-dihydrobenzo[4,5]thieno[3,2-b]oxepines, Chem. Commun., 2017, 53, 10672–10675 Search PubMed
.
- H. Xiang, Q.-L. Zhao, P.-J. Xia, J.-A. Xiao, Z.-P. Ye, X. Xie, H. Sheng, X.-Q. Chen and H. Yang, Visible-light-induced external radical-triggered annulation to access CF2-containing benzoxepine derivatives, Org. Lett., 2018, 20, 1363–1366 Search PubMed
.
-
(a) A. EI-Awa, M. N. Noshi, X. M. Jourdin and P. L. Fuchs, Evolving organic synthesis fostered by the pluripotent phenylsulfone moiety, Chem. Rev., 2009, 109, 2315–2349 Search PubMed
;
(b) B. A. Frankel, M. Bentley, R. G. Kruger and D. G. McCafferty, Vinyl sulfones: inhibitors of SrtA, a transpeptidase required for cell wall protein anchoring and virulence in staphylococcus aureus, J. Am. Chem. Soc., 2004, 126, 3404–3405 Search PubMed
;
(c) J. N. Desrosiers and A. B. Charette, Catalytic enantioselective reduction of β,β-disubstituted vinyl phenyl sulfones by using bisphosphine monoxide ligands, Angew. Chem., Int. Ed., 2007, 46, 5955–5957 Search PubMed
.
-
(a) C.-Q. Lai and B. J. Backes, Efficient preparation of S-aryl thioacetates from aryl halides and potassium thioacetate, Tetrahedron Lett., 2007, 48, 3033–3037 Search PubMed
;
(b) R. Ettari, E. Nizi, M. E. Di Francesco, M.-A. Dude, G. R. Pradel, T. Schirmeister, N. Micale, S. Grasso and M. Zappala, Development of peptidomimetics with a vinyl sulfone warhead as irreversible falcipain-2 inhibitors, J. Med. Chem., 2008, 51, 988–996 Search PubMed
;
(c) V. P. Sandanayaka, A. S. Prashad, Y.-J. Yang, R. T. Williamson, Y.-I. Lin and T. S. Mansour, Spirocyclopropyl βlactams as mechanism-based inhibitors of serine β-lactamases. synthesis by rhodium-catalyzed cyclopropanation of 6- diazopenicillanate sulfone, J. Med. Chem., 2003, 46, 2569–2571 Search PubMed
.
-
(a) Q.-Q. Lu, J. Zhang, G.-L. Zhao, Y. Qi, H.-M. Wang and A.-W. Lei, Dioxygen-triggered oxidative radical reaction: direct aerobic difunctionalization of terminal alkynes toward β-keto sulfones, J. Am. Chem. Soc., 2013, 135, 11481–11484 Search PubMed
;
(b) T.-Y. Shang, L.-H. Lu, Z. Cao, Y. Liu, W.-M. He and B. Yu, Recent advances of 1,2,3,5-tetrakis(carbazol-9-yl)-4,6-dicyanoben-zene (4CzIPN) in photocatalytic transformations, Chem. Commun., 2019, 55, 5408–5419 Search PubMed
;
(c) L. Zhang, S. Chen, Y. Gao, P. Zhang, Y. Wu, G. Tang and Y. Zhao,
tert-Butyl hydroperoxide mediated cascade synthesis of 3-arylsulfonylquinolines, Org. Lett., 2016, 18, 1286–1289 Search PubMed
;
(d) M. Y. Ansari, N. Kumar and A. Kumar, Regioselective intermolecular sulfur–oxygen difunctionalization (phenoxysulfonylation) of alkynes: one-pot construction of (Z)-β-phenoxy vinylsulfones, Org. Lett., 2019, 21, 3931–3936 Search PubMed
;
(e) Y.-Z. Zhang, Z.-Y. Mo, H.-S. Wang, X.-A. Wen, H.-T. Tang and Y.-M. Pan, Electrochemically enabled chemoselective sulfonylation and hydrazination of indoles, Green Chem., 2019, 21, 3807–3811 Search PubMed
;
(f) S. K. Pagire, A. Hossain and O. Reiser, Temperature controlled selective C–S or C–C bond formation: photocatalytic sulfonylation versus arylation of unactivated heterocycles utilizing aryl sulfonyl chlorides, Org. Lett., 2018, 20, 648–651 Search PubMed
;
(g) O. M. Mulina, A. I. Ilovaisky, V. D. Parshin and A. O. Terent'ev, Oxidative sulfonylation of multiple carbon-carbon bonds with sulfonyl hydrazides, sulfinic acids and their salts, Adv. Synth. Catal., 2020, 362, 4579–4654 Search PubMed
;
(h) Y.-Q. Jiang, J. Li, Z.-W. Feng, G.-Q. Xu, X. Shi, Q.-J. Ding, W. Li, C.-H. Ma and B. Yu, Ethylene glycol: A green solvent for visible light-promoted aerobic transition metal-free cascade sulfonation/cyclization reaction, Adv. Synth. Catal., 2020, 362, 2609–2614 Search PubMed
;
(i) Z.-W. Feng, J. Li, Y.-Q. Jiang, Y. Tian, G.-Q. Xu, X. Shi, Q.-J. Ding, W. Li, C.-H. Ma and B. Yu, Transition-metal-free sulfonylations of methylthiolated alkynones to synthesize 3-sulfonylated thioflavones, New J. Chem., 2020, 44, 14786–14790 Search PubMed
;
(j) K. Sun, X.-L. Chen, Y.-L. Zhang, K. Li, X.-Q. Huang, Y.-Y. Peng, L.-B. Qua and B. Yu, Metal-free sulfonyl radical-initiated cascade cyclization to access sulfonated indolo[1,2-a]quinolones, Chem. Commun., 2019, 55, 12615–12618 Search PubMed
.
-
(a) J. Yan, J. Xu, Y. Zhou, J. Chen and Q. Song, Photoredox-catalyzed cascade annulation of methyl(2- (phenylethynyl)phenyl)sulfanes and methyl(2-(phenylethyn-yl)phenyl)selanes with sulfonyl chlorides: synthesis of benzothiophenes and benzoselenophenes, Org. Chem. Front., 2018, 5, 1483–1487 Search PubMed
;
(b) Y. Liu, Q.-L. Wang, Z. Chen, Q. Zhou, H. Li, C.-S. Zhou, B.-Q. Xiong, P.-L. Zhang and K.-W. Tang, Visible-light-catalyzed C–C bond difunctionalization of methylenecyclopropanes with sulfonyl chlorides for the synthesis of 3-sulfonyl-1,2-dihydronaphthalenes, J. Org. Chem., 2019, 84, 2829–2839 Search PubMed
.
-
(a) J. Zhu, W.-C. Yang, X.-D. Wang and L. Wu, Photoredox catalysis in C-S bond construction: recent progress in photo-catalyzed formation of sulfones and sulfoxides, Adv. Synth. Catal., 2018, 360, 386–400 Search PubMed
;
(b) Y. Zhao, J.-R. Chen and W.-J. Xiao, Visible-light photocatalytic decarboxylative alkyl radical addition cascade for synthesis of benzazepine derivatives, Org. Lett., 2018, 20, 224–227 Search PubMed
;
(c) X.-L. Yang, J.-D. Guo, H. Xiao, K. Feng, B. Chen, C.-H. Tung and L.-Z. Wu, Photoredox catalysis of aromatic β-ketoesters for in situ production of transient and persistent radicals for organic transformation, Angew. Chem., 2020, 59, 5365–5370 Search PubMed
;
(d) D. Staveness, I. C. Bosque and R. J. Stephenson, Free radical chemistry enabled by visible light-induced electron transfer, Acc. Chem. Res., 2016, 49, 2295–2306 Search PubMed
;
(e) X.-K. He, J. Lu, A.-J. Zhang, Q.-Q. Zhang, G.-Y. Xu and J. Xuan, BI-OAc-accelerated C3−H alkylation of quinoxalin-2(1H)-ones under visible-light irradiation, Org. Lett., 2020, 22, 5984–5989 Search PubMed
;
(f) T. Courant and G. Masson, Recent progress in visible light photoredox-catalyzed intermolecular 1,2-difunctionalization of double bonds via an ATRA-type mechanism, J. Org. Chem., 2016, 81, 6945–6952 Search PubMed
;
(g) D. C. Fabry and M. Rueping, Merging visible light photoredox catalysis with metal catalyzed C–H activations: on the role of oxygen and superoxide ions as oxidants, Acc. Chem. Res., 2016, 49, 1969–1979 Search PubMed
;
(h) J. Yan, H. Cheo, W.-K. Teo, X. Shi, H. Wu, S. B. Idres, L.-W. Deng and J. Wu, A radical smiles rearrangement promoted by neutral Eosin Y as a direct hydrogen atom transfer photocatalyst, J. Am. Chem. Soc., 2020, 142, 11357–11362 Search PubMed
;
(i) J. Jung, E. Kim, Y. You and E. J. Cho, Visible light-induced aromatic difluoroalkylation, Adv. Synth. Catal., 2014, 356, 2741–2748 Search PubMed
;
(j) N. Zhou, X.-A. Yuan, Y. Zhao, J. Xie and C.-J. Zhu, Synergistic photoredox catalysis and organocatalysis for inverse hydroboration of imines, Angew. Chem., Int. Ed., 2018, 57, 3990–3994 Search PubMed
;
(k) N. Zhou, M. Wu, K. Kuang, S. Wu and M. Zhang, Transition-metal-free photo-induced cascade sulfonylation/addition/cyclization of 3-arylethynyl-[1,1′-biphenyl]-2-carbonitriles with aryldiazonium tetrafluorobo-rates via the insertion of sulfur dioxide, Adv. Synth. Catal., 2020, 362, 5391–5397 Search PubMed
;
(l) J.-Y. Chen, W.-F. Wu, Q. Li and W.-T. Wei, Visible-light induced C(sp3)−H functionalization for the formation of C−N bonds under metal catalyst-free conditions, Adv. Synth. Catal., 2020, 362, 2770–2777 Search PubMed
.
- N. Zhou, M. Wu, M. Zhang, X. Zhou and W. Zhou, TBPB-initiated cascade cyclization of 3-arylethynyl-[1,1′-biphenyl]-2-carbonitriles with sulfinic acids: access to sulfone-containing cyclopenta[gh]phenanthridines, Org. Biomol. Chem., 2020, 18, 1733–1737 Search PubMed
.
Footnotes |
† Electronic supplementary information (ESI) available. CCDC 2074239. For ESI and crystallographic data in CIF or other electronic format see DOI: 10.1039/d1qo00611h |
‡ These authors contributed equally to this work. |
|
This journal is © the Partner Organisations 2021 |
Click here to see how this site uses Cookies. View our privacy policy here.