DOI:
10.1039/D0RA07991J
(Paper)
RSC Adv., 2021,
11, 883-890
The yield and quality of Pleurotus abieticola grown on nematode-infected Pinus massoniana chips
Received
18th September 2020
, Accepted 28th November 2020
First published on 4th January 2021
Abstract
In this study, we investigated the use of nematode-infected Pinus massoniana chips (NPC) as the main ingredient in Pleurotus abieticola substrate. The effects of different substrate formulas on nutritional parameters, including total sugars, polysaccharides, proteins, lipids, amino acids, and fatty acids were assessed. The results indicated that NPC was suitable for P. abieticola cultivation. However, the addition of certain amounts of corncobs (CC) and cottonseed hulks (CH) improved the yield. Substrate T5 (45% NPC, 6% CC, and 27% CH) had the greatest yield (121.38 g per bag), 34.56% greater than the yield of the control (78% poplar chips), which was 79.43 g per bag. Across the 11 substrate formulas tested, the total sugar, polysaccharide, crude protein, and crude lipid contents were 16.60–28.90%, 2.71–3.73%, 36.49–45.42%, and 1.03–4.34%, respectively. On all substrates, the fruiting bodies contained 17 amino acids, primarily glutamine (2.42–4.11%), followed by proline (2.56–3.73%), leucine (2.09–3.19%), phenylalanine (1.56–2.61%), and glycine (1.76–2.55%). The fruiting bodies contained 12 fatty acids, of which linoleic acid was the most abundant (82.36%–84.03%), followed by palmitic acid (6.42%–6.89%) and oleic acid (5.50%–7.34%). The fatty acid content was closely associated with the NPC content, which might indicate that NPC promoted fatty acid accumulation. Thus, NPC represents a new substrate suitable for P. abieticola cultivation.
1 Introduction
Pine trees, one of the most common coniferous groups worldwide, have considerable economic and ecological value.1 Pine wilt, a destructive disease affecting pine trees, is caused by the pinewood nematode Bursaphelenchus xylophilus.2 Pine wilt originated in North America and then spread to Asia and Europe.3 In China, pine wilt has spread rapidly since the discovery of the pinewood nematode in Nanjing in 1982.4 At present, this disease threatens nearly 60 million hectares of pine trees in China. Outbreaks of the invasive pinewood nematode have led to huge ecological and economic losses in China.5 The areas affected by pine wilt disease caused by pinewood nematode, as well as the number of dead trees, have increased significantly in recent years.6 Pinus massoniana is one of the dominant tree species in various southern Chinese provinces, including Chongqing, Sichuan, and Yunnan. Pinewood nematode infections result in large numbers of nematode-infected Pinus massoniana trees.6 However, at present, the spread of pinewood nematode infection is primarily inhibited by cutting down and burning infected trees.5 Thus, the infected wood is wasted.
Traditionally, mushrooms are primarily cultivated on woodchips derived from broad-leaved trees such as poplar trees (poplar chips, PC) and fruit trees.7,8 Sawdust from coniferous trees is not commonly utilized, because, due to the rosin present in pine trees, most fungi are unable to colonize coniferous wood directly.1 However, it has been shown that edible fungi can be cultivated on coniferous wood substrates after an initial inoculation with fungi, such as Aureobasidium spp., Ceratocystis spp., and Ophiostoma piliferum, that decompose pine rosin. Indeed, fungal species in the genus Pleurotus, including P. euosmus, P. eryngii, P. ostreatus, P. populinus, and P. pulmonarius, grow normally and form fruiting bodies on fungus-treated coniferous wood.1 The content of pine rosin in dead nematode-infected P. massoniana wood is lower than that in healthy Pinus trees.9 We thus hypothesized that it might be possible to cultivate mushrooms directly on the dead wood. However, to date no studies have investigated the use of nematode-infected P. massoniana chips (NPC) as a substrate for edible mushroom cultivation. The average price of oak chips ranges from 600 to 1200 RMB per ton in China. However, the NPC is usually burned or abandoned in the forest, which is underutilized. The main cost is the freight, which is about 400 to 800 RMB per ton according to the distance. If we can use the NPC as a mushroom substrate, the cost will decrease relative to oak wood chips, PC, or other sawdust sources.
Pleurotus species, commonly known as oyster mushrooms, are the third largest cultivated mushrooms in the world. Oyster mushroom consumption continues to increase rapidly due to their delicious taste, medicinal properties, and nutritional value.10 Pleurotus species are easy to cultivate and can degrade various agricultural and industrial wastes.11 The species P. abieticola is morphologically and phylogenetically similar to other species in the P. ostreatus–P. pulmonarius complex.12 P. abieticola not only is delicious but also has strong anti-tumor and anti-inflammatory functions.13 The specific epithet “abieticola” may indicate that this species has a preference for substrates in the genus Abies. In addition, P. abieticola has been observed to grow on rotten wood from several species in the genera Picea, Alnus, and Salix.13 We predicted that P. abieticola might grow on NPC directly.
However, it is unclear how the use of NPC-based substrates might affect the yield and primary nutritional content of P. abieticola. Here, we evaluated the ability of P. abieticola to grow on an NPC-based substrate and analyzed the effects of different media on the main nutrients in the P. abieticola fruiting bodies. Our results will provide technical evidence for possible uses for otherwise-wasted NPC.
2 Materials and methods
2.1. Strain and spawn preparation
P. abieticola (strain number: CCMJ1805) was obtained from the Engineering Research Centre of Edible and Medicinal Fungi, Ministry of Education, Jilin Agricultural University, Changchun, China. Mycelia were activated on a potato dextrose agar (PDA) slant for 10 days at 25 °C in darkness.
Liquid spawn were prepared by culturing mycelial plugs (2 mm in diameter) in 250 mL flasks containing 100 mL of liquid media (4 plugs/flask) in a rotatory incubator at 25 °C with shaking at 150 rpm min−1 for 9 days in darkness. Next, 1 L flasks containing 500 mL of liquid media were inoculated with liquid spawn to a final concentration of 10% and were incubated for 9 days under identical conditions. The liquid media (pH 6.0) contained soluble starch (40 g L−1), sucrose (10 g L−1), KH2PO4 (3 g L−1), MgSO4 (1.5 g L−1), and yeast extract (10 g L−1).14
2.2. Substrate preparation
Nematode-infected Pinus massoniana wood was collected from Yinglong Gorge Scenic Area, Banan District, Chongqing, China. The wood was chopped, air dried, and ground into fine chips. Corn cobs (CC), PC, wheat bran, and light CaCO3 were purchased from the mushroom department at Jilin Agricultural University, Jilin Province, China. Cottonseed hulls (CH) were purchased in Xinjiang Province, China.
2.3. Substrate formulation
We designed 11 different substrate mixtures based on a simplex-lattice design, with the main ingredient of each mixture accounting for 78.0% of the total (Table 1). The main ingredient of the control substrate mixture (CK) was PC. In addition to the main ingredient, all formulations contained 20.0% wheat bran, 1.0% sugarcane, and 1.0% lime; the water content of each mixture was adjusted to 65%. All mixtures had a natural pH.
Table 1 Pleurotus abieticola cultivation formulas (wt%, except for C/N)a
Formula |
NPC |
CC |
CH |
PC |
C |
N |
C/N ratio |
Different letters (a–f) in the same column and rank indicate significant differences (P < 0.05). NPC, nematode-infected Pinus massoniana chips; CC, corn cobs; CH, cottonseed hulls. |
CK |
0 |
0 |
0 |
78 |
56.57 ± 0.77c |
1.30 ± 0.01a |
43.40 ± 0.85e |
T1 |
38 |
0 |
40 |
0 |
57.38 ± 2.58b |
1.05 ± 0.04g |
54.68 ± 0.68a |
T2 |
38 |
20 |
20 |
0 |
57.92 ± 1.77b |
1.11 ± 0.01f |
52.06 ± 2.18b |
T3 |
38 |
40 |
0 |
0 |
54.14 ± 0.62c |
1.16 ± 0.04e |
46.68 ± 1.38d |
T4 |
45 |
27 |
6 |
0 |
43.81 ± 0.60d |
1.32 ± 0.02b |
33.61 ± 0.73f |
T5 |
45 |
6 |
27 |
0 |
56.50 ± 0.74c |
1.32 ± 0.02b |
42.88 ± 0.77e |
T6 |
52 |
13 |
13 |
0 |
57.74 ± 1.19b |
1.32 ± 0.01c |
41.45 ± 1.34e |
T7 |
58 |
20 |
0 |
0 |
63.37 ± 3.54a |
1.35 ± 0.00c |
47.03 ± 2.68d |
T8 |
58 |
0 |
20 |
0 |
60.10 ± 1.59b |
1.32 ± 0.01d |
45.48 ± 1.23d |
T9 |
65 |
7 |
6 |
0 |
56.97 ± 0.89c |
1.15 ± 0.02e |
49.43 ± 0.23c |
T10 |
78 |
0 |
0 |
0 |
60.84 ± 1.11a |
1.13 ± 0.00e |
53.75 ± 0.85a |
The chemical compositions of all substrates were analyzed before the experiment began. Substrate samples were dried in an oven at 60 °C to a constant weight, and then ground into powder. The total carbon (C) content was measured following a previous study,15 and the total nitrogen (N) content was determined using the Kjeldahl method.16 Crude protein was calculated by multiplying the nitrogen content by a factor of 6.25.9 The assays used to determine the C and N contents were performed by Suzhou Keming Biotechnology Co., Ltd (Suzhou Province, China). Then, the C/N ratio of each substrate was calculated based on the contents of C and N.
We added 800.0 g of each substrate (dry weight 280.0 g) to a separate polypropylene bag (33 cm × 17 cm × 0.05 cm); each bag had a 2.5 cm collar at the mouth. A polyvinylchloride (PVC) tube (2.5 cm diameter × 12 cm long) was inserted into the collar of each bag to ensure the even distribution and colonization of the mycelia. The filled bags were autoclaved at 121 °C for 2 h. After cooling to room temperature, each bag was inoculated with 10 mL of P. abieticola liquid spawn under aseptic conditions. Each formula was represented by 15 bags.
2.4. Cultivation
The inoculated bags were randomly arranged on shelves in a growth room. All bags were incubated at 25 °C in the dark, at a carbon dioxide (CO2) concentration less than 2500 ppm. After the mycelia covered each cultivation bag, “V”-shaped holes were cut through the surfaces of the bags using a sterilized knife. Cut bags were then transferred to fruiting chambers in a cultivation room. The fruiting chamber conditions were maintained as follows to induce basidiome formation: temperature at 19–21 °C, humidity at 93–97%, CO2 concentration at 400–700 ppm, and illumination at 200 Lux × 12 h. Fruiting bodies were harvested before the caps had unfolded.14
2.5. Assessment of agronomic traits
After harvest, agronomic traits were recorded for the first flush of each bag; 15 bags per mixture were included in the statistical analyses. Spawning time was calculated as the period from inoculation to the point at which mycelia filled the bag. The first harvest period was defined as the time from inoculation to the first harvest of the fruiting body. Growth parameters pertaining to a single fruiting body, including pileus width, stipe length, and stipe width, were measured using Vernier calipers. The weight of the fruiting bodies was measured using an electronic balance with an accuracy of 0.01 g. Biological efficiency (BE) was calculated as the fresh weight of the mushrooms divided by the dry weight of the substrate × 100%.17
2.6. Analysis of the main nutrients
Mushroom samples were dried in an oven at 60 °C to a constant weight to calculate the moisture content. Dried samples were then ground into powder for further analysis. The dried mushroom powder was hydrolyzed, and the total soluble sugar content was measured with an ultraviolet-visible spectrophotometer using the 3,5-dinitrosalicylic acid (DNS) method.18 The polysaccharide content (i.e., the content of alcohol-insoluble carbohydrates) was measured using the Rajarathnam method by extracting dry mushroom power in 70% alcohol and hydrolyzing the alcohol-insoluble residue using 72% sulfuric acid. Then, the polysaccharide content was estimated using the phenol–sulfuric acid method.19,20 The protein content of each sample was estimated using the Kjeldahl method.16 Crude protein contents in the mushroom fruiting bodies were calculated by multiplying the nitrogen content by a factor of 6.25.9 Lipid levels were determined by extracting a known weight of powdered sample with ethyl ether, using a Soxhlet apparatus. After crude lipid extraction, fatty acids were measured using a TSQ 8000 EVO (Thermo Scientific) gas chromatograph equipped with an FID detector and a DB-1 fused silica capillary non-polar column (30 m × 0.25 mm i.d.; film thickness 0.25 μm), following the manufacturer's instructions. The hydrogen flow rate was 1.4 mL min−1. Split injection was carried out at 250 °C (1
:
40). The relative percentage of each fatty acid was expressed with respect to fatty acid methyl ester (FAME) standards as described previously.21 Amino acid levels in fruiting bodies were determined using high-performance liquid chromatography (HPLC) as described previously.22,23 The above assays, including those used to determine the soluble sugars, polysaccharides, proteins, lipids, amino acids, and fatty acids, were performed by Suzhou Keming Biotechnology Co., Ltd (Suzhou Province, China).
2.7. Statistical analyses
ANOVAs (analyses of variance) analyses were performed to estimate statistical parameters using SPSS for Windows (version 25.0). Differences among means were identified using the LSD test in SPSS. The relationships between the substrate content and fatty acid content were analyzed using Pearson correlations. We considered P < 0.05 to be statistically significant.
3 Results and discussion
3.1. Effects of the substrate formulas on the agronomic traits of the mushrooms
C/N ratios ranged from 33.61–54.68% across the 11 substrate formulas (Table 1), consistent with previous studies on P. ostreatus substrates.11,24 Across all substrates, the average spawning time duration was 22–28 days. Spawning time was shortest for T8 (22.18 days) and longest for T3 (28.0 days). The lengths of the first harvest period differed significantly among the 11 formulas (P < 0.05). T9 had the fewest days until first harvest (29 days), while T4 had the most (34.67 days). The length of the first flush period was 6–8 days from primordia initiation to fruit-body maturation. The average cap diameter was 42.56 mm (range 35.95–49.16 mm), the average stipe thickness was 9.39 mm (range 35.95–49.16 mm), and the average stipe length was 21.12 mm (range 15.65–26.59 mm). The single branch weight ranged from 2.69 g to 4.40 g. As NPC increased, yield first increased and then decreased. T5 had the greatest yield (121.32 g) and BE (43.7%). The yield and BE of T10 (75.74 g and 27.1%, respectively), which was grown on 78% NPC, did not differ significantly from the yield and BE of CK (79.43 g and 28.3%, respectively), which was grown on 78% PC (Table 2).
Table 2 Effects of different substrate formulas on the agronomic parameters of the fruiting bodiesa
Substrate formula |
Spawn running time (day) |
First harvest period (day) |
Pileus diameter (mm) |
Stipe thickness (mm) |
Stipe length (mm) |
Single branch weight (g) |
Yield per bag (g) |
BE (%) |
Different letters (a–c) in the same column and rank indicate significant differences (P < 0.05). |
CK |
24.50 ± 0.84 ab |
31.86 ± 2.96bc |
38.49 ± 10.94b |
9.45 ± 3.81b |
25.36 ± 6.54a |
4.60 ± 1.39b |
79.43 ± 11.44b |
28.36 ± 1.95b |
T1 |
23.67 ± 1.58bc |
31.71 ± 0.95bc |
49.16 ± 28.40a |
9.16 ± 3.46bc |
15.65 ± 6.59c |
4.40 ± 2.51a |
82.71 ± 20.55b |
29.54 ± 4.25b |
T2 |
25.67 ± 2.12 ab |
32.42 ± 1.39b |
43.72 ± 11.87a |
9.59 ± 2.38b |
20.94 ± 5.52b |
4.56 ± 2.51 ab |
81.03 ± 23.56b |
28.94 ± 4.16b |
T3 |
28.00 ± 2.08a |
32.50 ± 2.03b |
37.57 ± 12.58b |
9.03 ± 2.24bc |
26.59 ± 5.01a |
2.69 ± 1.24b |
83.46 ± 14.50b |
29.81 ± 2.37b |
T4 |
26.64 ± 2.29a |
34.67 ± 0.82a |
40.18 ± 15.24 ab |
9.78 ± 2.62b |
23.32 ± 7.49a |
3.78 ± 1.87b |
95.32 ± 29.61 ab |
34.04 ± 8.84 ab |
T5 |
23.92 ± 2.47b |
30.33 ± 3.01bc |
42.03 ± 15.13a |
10.51 ± 3.30a |
25.48 ± 7.84a |
4.87 ± 2.50 ab |
121.38 ± 29.09a |
43.35 ± 8.63a |
T6 |
24.62 ± 1.47b |
32.33 ± 2.06abc |
44.12 ± 16.01a |
9.35 ± 2.42b |
26.15 ± 7.83a |
4.31 ± 2.36b |
108.8 ± 21.99 ab |
38.86 ± 5.79 ab |
T7 |
26.00 ± 2.78a |
33.43 ± 3.64 ab |
40.18 ± 12.28b |
9.25 ± 3.06b |
24.49 ± 7.70a |
3.59 ± 1.80b |
93.49 ± 16.93 ab |
33.39 ± 3.77 ab |
T8 |
22.18 ± 0.35c |
29.00 ± 2.64c |
38.93 ± 11.64b |
8.89 ± 2.43bc |
26.28 ± 7.46a |
3.42 ± 1.81b |
92.12 ± 11.99b |
32.90 ± 1.22b |
T9 |
24.00 ± 1.67b |
30.14 ± 3.07c |
39.37 ± 13.07b |
8.7 ± 2.20bc |
22.24 ± 5.72 ab |
3.58 ± 1.19b |
78.27 ± 14.50b |
27.95 ± 2.80b |
T10 |
24.37 ± 1.72b |
30.73 ± 2.28c |
35.96 ± 10.92b |
8.27 ± 2.15c |
24.61 ± 6.03a |
2.84 ± 0.98b |
75.74 ± 12.01b |
27.05 ± 2.80b |
There were no significant differences in spawning time or yield between CK (grown on PC) and T10 (grown on NPC), suggesting that NPC was a suitable substrate for the growth of P. abieticola and might serve as a replacement for chips from broad-leaved trees. The yield of mushrooms grown on T5 was 1.6-fold greater than that of mushrooms grown on T10, suggesting that an optimal blend of CC and CH would increase yield. Similarly, supplementing rice straw growth substrate with CH and CC increased the yield of P. florida.25 This might be because mixed substrates contain more types of nutrients that promote growth. The first flush BEs for P. ostreatus, P. geesteranus, P. abalones, P. eryngii, and P. nebrodensi on a substrate of old asparagus stalks were 77.1%, 74.8%, 42.8%, 30.9%, and 25.4%, respectively.26 Here, the highest BE was 43.7% and the lowest BE was 27.1% in the first flush, which was lower than that of P. ostreatus and higher than that of P. nebrodensi.
Fruiting bodies formed normally on substrate formulas CK through T10 (Fig. 1). The colors of the pileus surfaces were grey to brownish grey. When wet, the surfaces were slightly viscid. The average cap diameter was less than 50 mm (range 35.96–49.16 mm), and we harvested approminately 14–30 branches per bag (Table 2). The morphological characters of the mushrooms grown in this study were consistent with those described by Liu et al.12 The cap diameter, stipe thickness, and stipe length of P. abieticola were smaller than those of P. ostreatus and P. cystidiosus.27 This might suggest that P. abieticola can potentially be cultivated as a small mushroom (cap diameter < 5 cm), with a good taste and a high protein content.
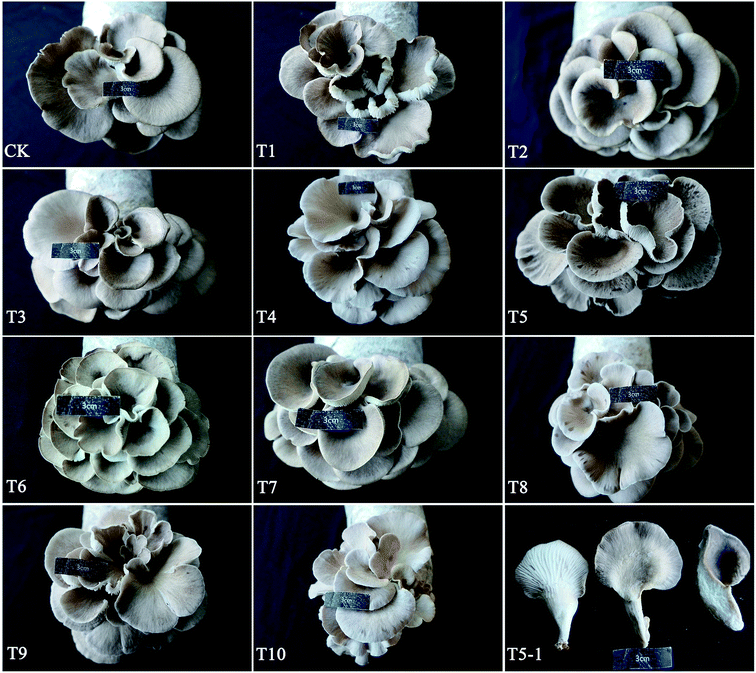 |
| Fig. 1 Fruiting bodies of P. abieticola grown on different substrate formulas. (CK) compost consisted of 78% poplar chips; (T1) compost consisted of 38% nematode-infected Pinus massoniana chips and 40% cottonseed hulls; (T2) compost consisted of 38% nematode-infected Pinus massoniana chips, 20% corn cobs and 20% cottonseed hulls; (T3) compost consisted of 38% nematode-infected Pinus massoniana chips and 40% corn cobs; (T4) compost consisted of 45% nematode-infected Pinus massoniana chips, 27% corn cobs and 6% cottonseed hulls; (T5) compost consisted of 45% nematode-infected Pinus massoniana chips, 6% corn cobs and 27% cottonseed hulls; (T6) compost consisted of 52% nematode-infected Pinus massoniana chips, 13% corn cobs and 13% cottonseed hulls; (T7) compost consisted of 58% nematode-infected Pinus massoniana chips and 20% corn cobs; (T8) compost consisted of 58% nematode-infected Pinus massoniana chips and 20% cottonseed hulls; (T9) compost consisted of 65% nematode-infected Pinus massoniana chips, 7% corn cobs and 6% cottonseed hulls; (T10) compost consisted of 78% nematode-infected Pinus massoniana chips; (T5-1) the back, front, and profile views of fruiting bodies of P. abieticola. | |
In recent years, many types of agricultural and forestry wastes, including CC, corn straw, old and asparagus stalks, as well as landfill items, such as used diapers and food, have been used as mushroom substrates8,10,26,28 Our results demonstrated that NPC could be used as the main substrate for P. abieticola cultivation.
3.2. Nutritional component analysis
Several fundamental nutritional characteristics of P. abieticola, including the total sugar, polysaccharide, protein, and crude oil contents, differed significantly with respect to the dry-weight abundance among the substrate formulas. The total sugar content ranged from 16.60% ± 0.65% (T8) to 28.90% ± 0.92% (T9), while the total polysaccharide content ranged from 2.71% ± 0.21% (CK) to 3.73% ± 0.22% (T7). The protein content ranged from 36.49% ± 0.55% (T6) to 45.42% ± 0.69% (T10). The protein content of CK, which was grown on 78% PC, was 43.60% ± 0.46%. The crude oil content ranged from 1.03% ± 0.07% to 4.34% ± 0.17%; T6 had the lowest crude oil contents (1.03%), and T1 had the highest (4.34%). T6 had the lowest protein and crude oil content. The protein and crude oil contents of mushrooms grown on NPC were significantly higher than those grown on PC (Table 3).
Table 3 Nutritional contents of P. abieticola fruiting bodies harvested from different substrate formulas (g per 100 g dry weight)a
Treatments |
Total sugar |
Polysaccharide |
Protein |
Lipid |
Different letters (a–f) in the same column and rank indicate significant differences (P < 0.05). |
CK |
17.34 ± 1.10c |
2.71 ± 0.21d |
43.60 ± 0.46b |
2.22 ± 0.32d |
T1 |
18.32 ± 0.60c |
2.85 ± 0.17d |
42.92 ± 0.29b |
4.34 ± 0.17a |
T2 |
24.01 ± 0.81b |
2.85 ± 0.15d |
39.48 ± 0.38c |
2.58 ± 0.31d |
T3 |
22.06 ± 0.83c |
2.94 ± 0.23 cd |
36.91 ± 0.40ef |
3.84 ± 0.12b |
T4 |
21.81 ± 1.53c |
2.92 ± 0.09 cd |
36.89 ± 0.31ef |
3.02 ± 0.23c |
T5 |
28.55 ± 0.96a |
3.34 ± 0.03c |
37.36 ± 0.42e |
1.78 ± 0.19e |
T6 |
24.20 ± 0.70b |
3.46 ± 0.11b |
36.49 ± 0.55f |
1.03 ± 0.07f |
T7 |
21.88 ± 0.76c |
3.73 ± 0.22a |
37.17 ± 0.66ef |
2.31 ± 0.19d |
T8 |
16.60 ± 0.65c |
2.95 ± 0.08 cd |
38.70 ± 0.41d |
2.26 ± 0.15d |
T9 |
28.90 ± 0.92a |
3.26 ± 0.10c |
38.13 ± 0.24d |
2.32 ± 0.22d |
T10 |
20.54 ± 0.18c |
2.85 ± 0.18d |
45.42 ± 0.69a |
2.64 ± 0.32c |
Substrate had a significant effect on nutrient composition. The contents of total sugar, protein, and fat in P. florida grown on rice straw supplemented with cotton seed were 28.1%, 37.2%, and 5.4%, respectively; however, total sugar, protein, and fat contents of P. florida grown on rice straw alone were 52.8%, 19.6%, and 4.0%, respectively.25 Across all 11 substrate formulas, the total sugar content ranged from 30% to 56%, lower than the total sugar contents of P. ostreatus, P. cystidiosus, P. flabellatus, and P. sajor-caju.16,27 Zhao et al. found that soluble polysaccharide contents in 19 P. ostreatus strains ranged from 0.555% to 2.806%.29 The polysaccharide contents of P. abieticola grown on NPC (T1–T10) were higher than those of P. ostreatus. The protein values in oyster mushroom dry matter we measured were consistent with previously reported values (20–56%).24,27 Our results indicated that the fruiting bodies of P. abieticola grown on all substrate formulas were rich in polysaccharides and proteins but low in fat, making these mushrooms excellent sources of nutrients for low-calorie diets (Table 3).
3.3. Amino acid analysis
The amino acid contents of the fruiting bodies grown on different substrate formulas were analyzed. The total amino acid content ranged from 21.58% (CK) to 35.07% (T6). The fruiting bodies contained 17 types of amino acids. The most abundant of these was glutamine (Glu; mean 3.17%; range 2.42–4.11%), followed by proline (Pro; mean 3.12%; range 2.56–3.73%), leucine (Leu; mean 2.60%; range 2.09–3.19%), phenylalanine (Phe; mean 2.10%; range 1.56–2.61%), and glycine (Gly; mean 2.01%; range 1.76–2.55%). The least abundant amino acid was cysteine (Cys; mean 0.24%; range 0.10–0.74%). The most abundant essential and inessential amino acids were Glu and Leu, respectively. The high Glu content of P. abieticola reflected its delicious taste (Fig. 2).
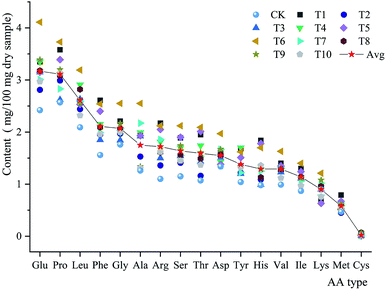 |
| Fig. 2 Amino acid analyses of different substrate formulas. Glu: glutamate; Pro: proline; Leu: leucine; Phe: phenylalanine; Gly: glycine; Ala: alanine; Arg: arginine; Ser: serine; Thr: threonine; Asp: aspirate; Tyr: tyrosine; His: histidine; Val: valine; Ile: isoleucine; Lys: lysine; Met: methionine; Cys: cysteine. Avg: average. | |
The total amino acid content of P. abieticola was 21.58–35.06%, while the total amino acid content of P. sajor-caju was 29.36–34.76% and that of P. florida was 8.92–20.2%.25,30 The most abundant amino acids in P. sajor-caju were similar to those of P. abieticola: Glu (4.14%), Ala (3.38%), Leu (2.5%), Pro (2.24%), and Phe (2.23%).30 P. florida grown on Moso bamboo sawdust primarily contained Ala, Glu, Arg, and Glu.25 The total amino acid content of P. abieticola did not differ significantly from that of other Pleurotus species. However, the high Pro content in P. abieticola might indicate that this mushroom could have a high nutritional value.31,32
3.4. Fatty acid analysis
On average, across all substrate formulas, the most abundant fatty acid was linoleic acid, followed by palmitic acid, oleic acid, stearic acid, and pentadecanoic acid. In addition to these abundant fatty acids, the fruiting bodies also contained other fatty acids at lower abundances. Of these, the most abundant was myristic acid, followed by methyl arachidonate acid, arachidonic acid, 11,14-eicosadienoic acid, palmitoleic acid, heptadecanoic acid, and arachidic acid. T10 had the highest fatty acid content (8265.35 μg g−1), while T3 had the lowest (5636.10 μg g−1). T10 also had the highest levels of linoleic acid (6889.81 μg g−1) and palmitic acid (555.35 μg g−1) (Table 4). NPC contains pine resin, which might be transferred by mycelia. Therefore, we investigated the relationship between the substrate content and fatty acid content using Pearson correlation analysis. We found that the NPC content was positively associated with the total fatty acid, linoleic acid, palmitic acid, and oleic acid contents. However, the CC and CH contents were not significantly associated with these parameters. Thus, our results indicated that NPC increased the accumulation of fatty acids (Table 5).
Table 4 Fatty acid contents of P. abieticola fruiting bodies grown on the different substrate formulas (μg per g dry weight)a
Fatty acid |
Linoleic acid |
Palmitic acid |
Oleic acid |
Stearic acid |
Pentadecanoic acid |
Myristic acid |
Methyl arachidonate acid |
Arachidonic acid |
11, 14-Eicosadienoic acid |
Palmitoleic acid |
Heptadecanoic acid |
Arachidic acid |
Sum |
Different letters (a–f) in the same column and rank indicate significant differences (P < 0.05). |
CK |
5607.23c |
445.76c |
489.89b |
82.67b |
62.61b |
15.95c |
17.18c |
9.01a |
8.02a |
10.58a |
4.49c |
3.61b |
6756.98e |
T1 |
5117.66d |
391.58d |
366.74d |
68.93c |
79.75b |
12.12d |
29.63a |
8.10b |
7.29b |
5.37c |
6.30a |
2.11d |
6095.57f |
T2 |
5405.97c |
433.29c |
444.50c |
86.47b |
80.37a |
14.16c |
17.16c |
7.92b |
7.26b |
6.43c |
5.33b |
3.12c |
6511.98e |
T3 |
4736.84e |
385.53d |
309.90d |
70.16c |
80.65a |
13.84c |
9.91d |
7.53c |
6.51c |
5.81c |
6.68a |
3.75b |
5637.10g |
T4 |
6698.79a |
547.05a |
460.64c |
123.74a |
101.50a |
18.63a |
22.03a |
10.68a |
10.15a |
8.05b |
8.98a |
4.87a |
8015.12b |
T5 |
6663.94a |
550.59a |
526.17b |
105.8a |
92.89a |
19.32a |
20.27 ab |
10.37a |
8.86a |
8.78b |
7.74a |
3.46b |
8017.88b |
T6 |
6093.63b |
509.97b |
524.92b |
111.32a |
91.12a |
17.37b |
16.49c |
8.65b |
7.90b |
7.54c |
5.54c |
4.26a |
7398.72c |
T7 |
6478.46a |
525.51a |
514.07b |
104.09a |
75.14b |
19.07a |
16.56c |
9.59a |
9.25a |
8.76b |
6.62a |
4.34a |
7771.48d |
T8 |
6155.36b |
503.11b |
507.03b |
103.70a |
75.67b |
18.38a |
9.89d |
9.04a |
9.19a |
8.07b |
4.81c |
3.87b |
7408.11c |
T9 |
6631.50a |
547.89a |
588.61a |
103.56a |
82.01a |
19.47a |
11.03d |
10.17a |
7.84b |
10.66a |
5.71b |
2.33d |
8020.79b |
T10 |
6889.81a |
555.35a |
573.86a |
91.98b |
84.93a |
17.20b |
13.58d |
8.74b |
10.01a |
10.70a |
6.26a |
2.93b |
8265.35a |
Average |
6043.56 |
490.51 |
482.39 |
95.65 |
82.42 |
16.86 |
16.70 |
9.07 |
8.39 |
8.25 |
6.22 |
3.51 |
7263.55 |
Table 5 Correlation coefficients between substrate contents and fatty acid contentsa
Substrate content |
Total fat acid |
Linoleic acid |
Palmitic acid |
Oleic acid |
Stearic acid |
Pentadecanoic acid |
**, P < 0.01, *, P < 0.05. NPC, nematode-infected Pinus massoniana chips; CC, corn cobs; CH, cottonseed hulls. |
NPC |
0.72* |
0.71* |
0.69* |
0.80** |
0.32 |
−0.14 |
CC |
−0.41 |
−0.33 |
−0.56 |
−0.62 |
0.17 |
−0.18 |
CH |
−0.31 |
−0.36 |
−0.24 |
−0.27 |
−0.04 |
−0.40 |
The main types of fatty acids identified in P. abieticola were similar to those identified in other mushrooms, which primarily included linoleic acid, palmitic acid, oleic acid, and stearic acid.25 Here, in addition to the most abundant fatty acids, the fruiting bodies also included several trace fatty acids, such as myristic acid, methyl arachidonate acid, arachidonic acid, 11,14-eicosadienoic acid, palmitoleic acid, heptadecanoic acid, and arachidic acid. These fatty acids have been rarely identified in the other mushrooms. Interestingly, the relative contents of linoleic acid, palmitic acid, oleic acid, and stearic acid with respect to total fatty acids were 82.36–84.03%, 6.42–6.89%, 5.50–7.34%, and 0.92–1.43%, respectively. Linoleic acid, which was the most abundant fatty acid, is an essential fatty acid that cannot be synthesized by human bodies.33 Thus, these fruiting bodies could potentially be further developed into linoleic acid-related products.
4. Conclusion
We found that P. abieticola successfully colonized substrate formulas containing NPC as the main ingredient and formed normal fruiting bodies. Therefore, NPC could be used as a substrate for P. abieticola cultivation. Formula T5 (45% NFC, 6% CC, and 27% CH) was more suitable for mushroom cultivation because it led to greater yield and BE. In addition, although fruiting bodies grown on CK (78% PC) did not differ significantly from those grown on T10 (78% NCF) with respect to colonization period and yield, the contents of total sugars, polysaccharides, crude proteins, crude oil, fatty acids, and amino acids in the T10 grown mushrooms were significantly higher. Thus, the use of NPC as a substrate might improve the nutrient accumulation of P. abieticola as compared to mushroom grown on broadleaf tree chips. The fruiting bodies contained 17 amino acids, primarily Glu, Pro, Leu, Phe, and Gly. The fruiting bodies also contained 12 fatty acids. Linoleic acid was the most abundant fatty acid, followed by palmitic acid, oleic acid, stearic acid, and pentadecanoic acid. The total fatty acid content was strongly positively associated with the NPC content, which might indicate that NPC promoted fatty acid accumulation. The results indicate it is profitable to develop and utilize NPC as a new potential substrate.
Author contribution
X Guo, CT Li, B Song and Y Li conceived and designed the project; X Guo and L Sun performed the experiments and analyzed the data; X Guo and CT Li wrote the manuscript. YP Fu and B Song revised the manuscript. All the authors read and approved the manuscript.
Ethical approval
This article does not contain any studies with animals or human participants performed by any of the authors.
Informed consent
Informed consent was obtained from all individual participants included in the study.
Conflicts of interest
The authors declare that they have no competing interests.
Acknowledgements
The National Key Research and Development Program of China (No. 2017YFD0601002), Science and Technology Research Program of Chongqing Municipal Education Commission (No. KJQN202000523), Program of Creation and Utilization of Germplasm of Mushroom Crop of the “111” Project (No. D17014), and Chongqing Normal University Foundation Program (11 × LB 015) supported this study.
References
- C. C. Suki, Forest Prod. J., 2004, 54(2), 68–76 Search PubMed.
- D. N. Proenca, G. Grass and P. V. Morais, Microbiology Open, 2017, 6(2), 415–435 CrossRef.
- A. Ryss, P. Vieira, M. Mota and O. A. Kulinich, Nematology, 2005, 7(3), 393–458 Search PubMed.
- Y. Mamiya, Annu. Rev. Phytopathol., 1983, 21, 201–220 CrossRef CAS.
- X. X. Zhao and L. Y. Yang, Biological Disaster Sci, 2019, 42(3), 186–190 Search PubMed.
- Y. J. Guo, Q. N. Lin, L. Chen, C. L. Rebeca, A. H. Zhang, E. S. Shao, G. H. Liang, X. Hu, R. Wang, L. Xu, F. P. Zhang and S. Q. Wu, BMC Genomics, 2020, 21, 337 CrossRef CAS.
- K. M. Alananbeh, N. A. Bouqellah and N. S. Al Kaff, Saudi J. Biol. Sci., 2014, 21(6), 616–625 CrossRef.
- K. Mukaila and H. A. Auwalu, Bioresource. Technol., 2004, 94(1), 65–67 CrossRef.
- J. T. Wang, Q. Wang and J. R. Han, Sci. Hortic., 2013, 157, 84–89 CrossRef CAS.
- L. M. Nyuk, C. K. Shing, X. P. Wan, M. N. Chia, H. T. Chin, K. P. Young and S. L. Su, J. Clean Prod., 2020 DOI:10.1016/j.jclepro.2020.122272.
- F. R. Vieira and M. C. Andrade, World J. Microb. Biot., 2016, 32, 190–199 CrossRef.
- X. B. Liu, J. W. Liu and Z. L. Yang, Mycosystema, 2015, 34, 581–588 CAS.
- G. Sathyaprabha and R. Senthil, J. Pure Appl. Microbio., 2017, 11(2), 891–901 CrossRef.
- N. Wu, F. H. Tian, O. Moodley, B. Song, C. W. Jia, J. Q. Ye, R. N. Lv, Z. Qin and C. T. Li, AMB Express, 2019, 9, 184–193 CrossRef CAS.
- D. W. Nelson and L. E. Sommers, Soil Sci. Soc. Am. J., 1982, 539–579 Search PubMed.
- R. Gothwal, A. Gupta, A. Kumar, S. Sharma and B. J. Alappat, 3 Biotech., 2012, 2(3), 249–257 CrossRef.
- B. Song, J. Q. Ye, F. L. Sossah, C. T. Li, D. Li, L. S. Meng, S. Xu, Y. P. Fu and Y. Li, AMB Express, 2018, 8(1), 46–57 CrossRef.
- I. Kerepesi, M. Toth and L. Boross, J. Agric. Food Chem., 1996, 44, 3235–3239 CrossRef CAS.
- A. M. Alam, M. Kamruzzaman, T. D. Dang, S. H. Lee, Y. H. Kim and G. M. Kim, Anal. Bioanal. Chem., 2012, 404(10), 3165–3173 CrossRef CAS.
- S. Rajarathnam, D. B. Wankhede and M. V. Patwardhan, Eur. J. Appl. Microbiol. Biotechnol., 1979, 8, 125–134 CrossRef CAS.
- Ç. Fatih, T. Gülsen, E. D. Mehmet, Ö. Mehmet, T. Aziz and H. Mansur, Food Anal. Method, 2014, 7, 449–458 CrossRef.
- M. Y. Kim, I. M. Chung, S. J. Lee, J. K. Ahn, E. H. Kim, M. J. Kim, S. L. Kim, H. I. Moon, H. M. Ro, E. Y. Kang, S. H. Seo and H. K. Song, Food Chem., 2009, 113, 386–393 CrossRef CAS.
- H. Zhang, H. J. Jiang, X. J. Zhang and J. Z. Yan, Curr. Pharm. Anal., 2018, 14, 562–570 CrossRef CAS.
- Z. Q. Jin, Q. W. Hou and T. Z. Niu, J. Sci. Food Agric., 2020, 100, 4901–4910 CrossRef CAS.
- M. N. Shashirekha, S. Rajarathnam and B. Zakia, Food Chem., 2005, 92, 255–259 CrossRef CAS.
- F. H. Zhai and J. R. Han, Sci. Hortic., 2018, 231, 11–14 CrossRef CAS.
- T. H. Hoa, C. L. Wang and C. H. Wang, Mycobiol, 2015, 43(4), 423–434 CrossRef.
- S. Xu, F. Wang, Y. P. Fu, D. Li, X. Z. Sun, C. T. Li, B. Song and Y. Li, RSC Adv., 2020, 10, 9798–9807 RSC.
- S. Zhao, Y. Liu, B. B. Yin, S. X. Wang, F. Xu, X. L. Geng and L. L. Meng, J. Anhui Agri. Sci., 2011, 39(19), 11405–11406 CAS.
- G. Aditi, S. Satyawati, S. Supradip and W. Suresh, Food Chem., 2013, 141, 4231–4239 CrossRef.
- X. F. Xu, H. D. Yan, J. Chen and X. W. Zhang, Biotech. Adv., 2011, 29(6), 667–674 CrossRef CAS.
- A. González, M. Cruz, C. Losoya, C. Nobre, A. Loredo, R. Rordriguez, J. Contreras and R. Belmares, Food Funct., 2020, 11, 7400 RSC.
- P. Parikh, M. C. McDaniel, D. Ashen, J. I. Miller, M. Sorrentino, V. Chan, R. S. Blumenthal and L. S. Sperling, J. Am. Coll. Cardiol., 2005, 45, 1379–1387 CrossRef CAS.
Footnote |
† These authors contributed equally to this work. |
|
This journal is © The Royal Society of Chemistry 2021 |
Click here to see how this site uses Cookies. View our privacy policy here.