DOI:
10.1039/D0RA09314A
(Paper)
RSC Adv., 2021,
11, 1743-1749
Microencapsulation of lime (Citrus aurantifolia) oil for antibacterial finishing of cotton fabric
Received
2nd November 2020
, Accepted 10th December 2020
First published on 6th January 2021
Abstract
Functional cotton fabric incorporated with antibacterial microcapsules of lime (C. aurantifolia) essential oil (LO) was prepared. The coacervation method, employing two biopolymers of alginate and gelatin as the shells, was preferentially selected to produce the LO microcapsules, whereas immobilization of the LO microcapsules onto the fabric was done using the pad–dry–cure method using various concentrations of citric acid binder. The antibacterial inhibition zone of the functional fabric was subsequently analysed using the Kirby Bauer method. The LO microcapsules were produced with a yield, encapsulation efficiency (EE), and oil content (OC) of 47 ± 4%, 84 ± 11%, and 58 ± 4%, respectively. The homogenous spherical and soft microcapsules (1.554 μm) bonded effectively by 4% citric acid onto the surface of the fabric and detached back by only 3% after 15 cycles of washing. Overall, the optimized functional fabric exhibited the highest antibacterial activities among others against typical skin bacteria, such as S. aureus, E. coli, K. pneumoniae, and S. epidermidis, and thus it can be potentially applied to obtain antibacterial functional textile.
Introduction
Numerous industries harvest and process the primary part of Citrus aurantifolia, including the pulp and juice, into food and beverages but usually discard the lime peels even though they contain essential oil (LO) that can be utilized as antimicrobials, disinfectants, anti-inflammatory, relaxing, stimulating, antioxidant, anticarcinogenic, antidepressant, and antifungal agents.1–4 The extensive activities possessed by LO are because of their substantial amounts of beneficial compounds like D-limonene, γ-terpinene, linalool, linalyl acetate, α-terpineol, geranyl acetate, terpinolene, and β-pinene.4 However, the direct application of LO is hampered by the properties of these components like high volatility and susceptibility to oxidative deterioration upon exposure to oxygen in the air and changes in temperature and light.5 Thereupon, many alternative methods have been progressively developed, including microencapsulation, which utilizes shells to protect the active core from outside interferences.6 The technology is widely applied in health, cosmetics, pharmacy, textiles, and agriculture.7 In particular, the microencapsulation technology in textiles requires further incorporation of the capsules onto the fabric, employing a binder to prevent the loss upon washing.8
Cotton fabrics are susceptible to microbial colonization due to the hydrophilic composition of carbohydrates. Moreover, the pores tend to retain moisture and also serve as a source of oxygen, nutrients, and energy for the growth of microorganisms.9,10 The outcome will not only increase the spread risk of diseases but also degrade the quality of the textile products including loss in strength, color damage, and unpleasant odours.11 To the best of our knowledge, the first study related to the manufacture of functional fabrics immobilized with microcapsule of C. aurantifolia essential oil (LO) was reported by Wijesirigunawardana & Perera in 2018.12 Large-sized of LO microcapsules coated with biopolymers of chitosan and Arabic gum were developed and subsequently incorporated onto cotton fabrics using succinic acid binder. Overall, the modified cotton fabric exhibited medium antibacterial inhibition against S. aureus, E. coli, Bacillus cereus, and Salmonella typhimurium.
In this study, we developed antimicrobial functional fabrics immobilized with LO microcapsules. To improve the performance, the modification of previous findings was applied, such as employing alginate and gelatin as the shells and optimizing citric acid as the binder of microcapsules with fabrics. In addition, antibacterial activities of the products were evaluated against common skin bacteria like S. aureus, E. coli, K. pneumoniae, and S. epidermidis.
Results and discussion
LO microencapsulation and characterization
The microencapsulation of LO employing the complex coacervation method with two biopolymers of alginate and gelatin 2% (w/v) as shells produced 47 ± 4% yield with an oil content (OC) and encapsulation efficiency (EE) of 58 ± 4 and 84 ± 11%, respectively. As anticipated, the combined biopolymer shells bearing opposite charges could improve the properties of the microcapsules. Strong intermolecular hydrogen bonds and ionic interactions of the shells presumably existed13 along with a tendency to cause electrostatic attraction.14 As a result, the forces tend to decrease the aggregation and precipitation upon encapsulation15 and finally increase the yield, oil content (OC), and encapsulation efficiency (EE) of the microcapsules.
The average particle size of the capsule was 1.554 μm with a homogenous distribution (mean/median = 0.951), and thus it was categorized as a microcapsule (Fig. 1). Based on the SEM of 1000× magnification, the shape of the microcapsules was observed to be spherical with a smooth surface (Fig. 2).
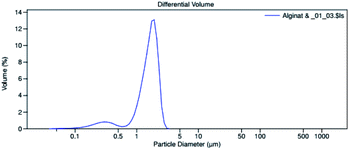 |
| Fig. 1 PSA spectrum of the LO microcapsule. | |
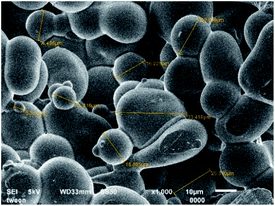 |
| Fig. 2 SEM micrograph of LO microcapsule at 1000× magnification. | |
FTIR studies revealed the functional group bands and their relationship between all materials and LO after the microencapsulation process (Fig. 3). During the coacervation process, the gelatin moieties such as amines at 3321 cm−1, carboxylate at 1652 cm−1 (Fig. 3a), and the hydroxyl group of alginate at 3450 cm−1 (Fig. 3b) seemingly remained the same after the encapsulation (Fig. 3c). A non-covalent interaction apparently occurred between the two biopolymers. According to Saravanan and Rao (2010),16 polyelectrolyte complexation between the two ionic shells was reported to take place due to ionic interaction between the amino group of gelatin and the carboxylate moiety of sodium alginate at an applied acidic pH. Correspondingly, a signal similar to that of pure LO (Fig. 3d) appeared and overlapped with the coating signal, thereby indicating no significant interaction between the LO and their coatings.
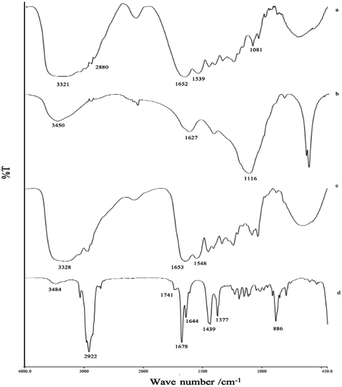 |
| Fig. 3 FTIR spectra of (a) gelatin, (b) sodium alginate, (c) LO microcapsules, (d) LO. | |
The thermograph of the LO microcapsule (Fig. 4) showed that LO microcapsules experienced a mass loss of 1.5% in the first stage of heating (<100 °C). The loss was presumably due to the evaporation of water/moisture and a small fraction of LO, with boiling point <100 °C. As more heat was applied to the microcapsule in the second stage (100–300 °C), more mass loss occurred, which might be due to the release of more heavy compounds of LO. Finally, the microcapsules started to lose more than 60% of their mass in the third stage (>300 °C). At this stage, the microcapsules initially experienced dehydration and depolymerization before finally suffering from decomposition.17 The mass loss of the blank microcapsule and LO also occurred upon heating them in the temperature range of 100–750 °C. In particular, the drastic change in the mass of LO below 150 °C was anticipated to be due to the release of the volatile compounds (Fig. 4). The achievement of good thermal stability of the microcapsules would delay the direct evaporation of LO inside the core and slow down the polymer degradation process upon heating.18
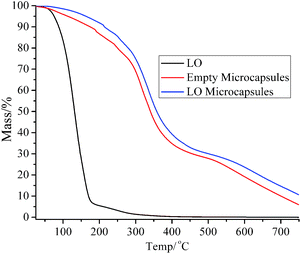 |
| Fig. 4 TGA thermographs of ( ) LO, ( ) empty microcapsules, ( ) LO microcapsules. | |
The performance of LO microcapsules against chemical and mechanical interferences was further investigated. Upon chemical extraction at a steady stirring force, the active LO inside the core was freed gradually (Fig. 5). Within 2 h, 79% of LO was found to be released from the microcapsules. Moreover, upon employing Avrami's kinetic model (eqn (3)), the mechanism and rate constant of LO release were obtained (Fig. 5). The acquired n value of 1.3 ± 0.1 suggested the release mechanism preferentially fits the first order kinetics, while the R2 value of 0.9423 indicated that the LO release from the microcapsules was better fitted to Avrami's equation. Referring to the computed rate constant of (2.2 ± 0.4) × 10−4 s−1, the release of LO over two shells of alginate and gelatin delayed successfully. LO was implied to be overlaid strong enough inside the microcapsules commonly because of the density of the microcapsule shell material.19 The aforementioned results implicitly suggested that the retention rate of LO inclusion microcapsules might be even slower at normal conditions, where there were no chemical and exaggerated mechanical interferences. Nevertheless, further investigation is necessary, particularly to understand the stability and rate constant of the release of the LO inclusion complex at various temperatures.
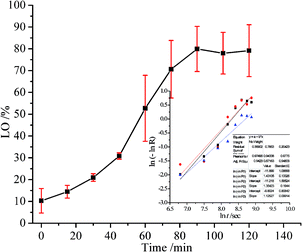 |
| Fig. 5 Oil release of LO microcapsules (mean ± SD/%; n = 3) in n-hexane when stirred at 100 rpm, inset graph; proposed Avrami's kinetic model of the LO release (n = 1.3 ± 0.1; k = (2.2 ± 0.4) × 10−4 s−1). | |
Microcapsule immobilization
The pad–dry–cure method for the immobilization of the LO microcapsules on cotton fabric was optimized using various concentrations of citric acid as a natural binder (Table 1). In general, the weight of the fabric was affected by the concentration of the binder. The optimum add-on of the fabric was achieved upon addition of 4% binder. The process suggested that such interaction between the carboxylate moieties of citric acid with the –OH group of cotton fibers existed through an esterification reaction (Fig. 6); hence, an increase in total mass was acceptable. Nevertheless, using an adhesive of more than 4% (citric acid) did not increase the add-on, which plausibly means that the reaction reached a saturation point. The curing process became essential as the binding process was induced by heating. Certainly, the appropriate temperature used in the curing process tends to form a stable and continuous film on the fabric surface.20 Thus, perhaps more microcapsules bonded firmly on the fabric and were resistant against the washing cycle.
Table 1 The add-on percentage of fabric upon application of various concentrations of binder
Code of fabric |
Binder of citric acid (%) |
Add on (%) |
A |
2 |
4.6 |
B |
4 |
6.5 |
C |
6 |
6.0 |
 |
| Fig. 6 The reaction scheme of citric acid binder with the fabric and microcapsules. | |
The successful accomplishment of immobilization was further confirmed by SEM micrographs (Fig. 7). More microcapsules were observed on the surface of the fabric treated with 4% binder (Fig. 7c). The morphology of the microcapsules before (Fig. 2) and after incorporation into cotton fabrics (Fig. 7c) was seemingly different. However, their particle size was confirmed to remain in the range of 14–20 μm, and thus the immobilization process did not considerably alter either the size or the morphology of the microcapsules. On the other hand, application of more binder (6%) in the immobilization process caused the microcapsules to be covered by a thin film layer on the surface of the fabric (Fig. 7d). At a certain point, the more citric acid applied in immobilization presumably increased more active carboxylic acid moieties to react with the cellulose of the fabric,21 and consequently, more binder covered the LO microcapsules. In such a case, excessive binder was not recommended by commercial brands because it changed the fabric hand.22 Overall, the resultant fabric was found to remain flexible, and natural binders, including citric acid, are known to help control air circulation and elasticity without altering the tensile strength, elasticity, flexibility, air permeability, and fabric softness.9
 |
| Fig. 7 SEM micrographs of fabric immobilized with LO microcapsules at 1000× magnification: (a) blank fabric, (b) 2% binder, (c) 4% binder, and (d) 6% binder. | |
To test the stability of the LO microcapsules immobilized on the fabric, the washing strength test was conducted using a Launder O-meter. The fabrics were tested for 1, 5, 10 and 15 times of standard household washing (Fig. 8). The mass loss of fabric B and C upon 1-time washing was more than that of 3-time washing of fabric A. It was supposed that large particles of microcapsules tend to detach from the fabric in the first cycle of washing, yet the small ones resisted detachment from the fabric.22 Furthermore, the mass loss tendency of fabric A and C was even more after 10 times of washing. However, after 15 times of washing, fabric B remained firm, and the mass loss was around 3%. This was plausibly due to the 4% binder, which held the LO microcapsules effectively on the surface of the fabric. In general, the results were in agreement with major limitations found in the modified fabric, including the fabric immobilized with LO microcapsules.20
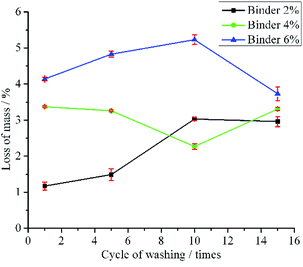 |
| Fig. 8 Mass loss (mean ± SD/%; n = 3) of fabric immobilized with LO microcapsules in the washing strength test. | |
Since the fabric immobilized with LO microcapsules using 4% binder showed relatively better resistance against the washing cycle than other fabrics, further SEM analysis was performed to ascertain the morphology of the washed fabric (Fig. 9). The micrographs emphasized that mass loss was observed in the washing strength test. The more the washing cycles applied to the modified fabric, the more LO microcapsules cracked into small (2–10 μm) and irregular shapes. Nevertheless, after 15 times of washing, the microcapsules were still firmly bound on the surface of cotton fabric, yet lost their core partially. Beneficially, the organoleptic test on the modified fabric (15 times of washing) confirmed the availability of the LO core, even after 4 months of exposure at ambient temperature. The binder played important roles to prevent the microcapsules from being washed out from the fabric. The decreasing amount of microcapsules on the surface of the fabric suggested that more investigations about stronger binders are necessary. Hitherto, the poor washing resistance of the modified functional fabric is still the main issue.20
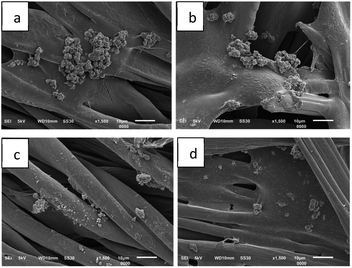 |
| Fig. 9 SEM micrographs of fabric immobilized with LO microcapsules with 4% binder against cycle of washing (a) 1×, (b) 5×, (c) 10×, and (d) 15× (1500× magnification). | |
Antibacterial activity
The performance of LO microcapsules immobilized on the fabrics as an antibacterial agent was examined using the Kirby Bauer method (Table 2). The assay was also compared to the activity of LO of C. aurantifolia (Table 3). In general, the microcapsules coated on the fabrics with various binders exhibited medium ZoI against all representative bacteria; furthermore, the modified fabrics with 4% binder showed the highest activity among others, especially against S. epidermidis. However, antibacterial activities of all modified fabrics were at least 3 times lower than that of LO alone or even limonene, which is the major constituent of LO. The distinctive antibacterial inhibition between LO and encapsulated LO was reasonable because the LO concentration applied was different. According to the oil release data, LO inside the shells were excreted slowly upon mechanical and chemical force. Hence, the concentration of LO on the fabric surface to inhibit the bacteria plausibly was not homogeneous. The SEM micrograph also emphasized the finding, where the microcapsules were not distributed well all over the fabric surface. After the washing test, the antibacterial activity of deflated and small microcapsules found on the 15 time washed fabrics should expose higher inhibition than before the washing test. This was because of the stronger LO odor released from the cracked microcapsules, which still remained after 4 months. However, more studies should be carried out.
Table 2 The inhibition zone of bacterial growth by LO microcapsules immobilized on fabrics at various binder concentrationsa
Conc. binder (%) |
Zone of inhibition (mm)* against bacteria |
S. aureus |
S. epidermidis |
E. coli |
K. pneumoniae |
N.a = not active, *mean ± SD; n = 2. |
2 |
6.1 ± 0.1 |
9.0 ± 1.0 |
6.3 ± 0.2 |
7.0 ± 1.0 |
4 |
7.0 ± 0.2 |
12.0 ± 0.0 |
7.1 ± 0.1 |
8.4 ± 0.3 |
6 |
6.1 ± 0.1 |
8.0 ± 0.0 |
7.0 ± 0.1 |
7.2 ± 0.1 |
Table 3 The inhibition zone of bacterial growth by LO of C. aurantifoliaa
Bacteria |
Zone of inhibition (mm)* |
Essential oil |
Limonene |
Ampicillin positive control (1000 ppm) |
n-Hexane negative control |
N.a = not active, *mean ± SD; n = 2. |
Gram positive bacteria |
S. aureus |
34.3 ± 0.3 |
24.4 ± 0.1 |
36.0 ± 1.0 |
N.a |
S. epidermidis |
38.0 ± 0.1 |
32.0 ± 0.4 |
15.0 ± 0.4 |
N.a |
![[thin space (1/6-em)]](https://www.rsc.org/images/entities/char_2009.gif) |
Gram negative bacteria |
E. coli |
12.0 ± 0.1 |
28.0 ± 0.4 |
11.0 ± 1.0 |
N.a |
K. pneumoniae |
37.0 ± 2.3 |
36.0 ± 0.4 |
11.0 ± 0.4 |
N.a |
In comparison, the immobilized LO microcapsules on fabrics prepared in this study exhibited different properties than the previous findings reported by Wijesirigunawardana & Perera in 2018.12 Since the biopolymer shells used in this study were alginate and gelatin, the particle size of the LO microcapsules was smaller than the ones reported previously.12 In combination with citric acid as the binder, smaller LO microcapsules could be incorporated more tightly onto the surface of the fabric compared to the larger one.22 In addition, the modified antibacterial fabrics exhibited strong activity, especially against S. epidermidis bacteria.
In principle, the antibacterial activity of the microcapsule was due to the lipophilic LO released gradually from the microcapsule. When these cores interacted with the cell membrane of bacteria, it changed the permeability of the membrane and destroyed its integrity. Finally, the cell experienced lysis and died.23 As Gram positive and Gram negative bacteria have distinctive membrane layer properties,24 the LO microcapsules should exhibit antibacterial activity differently.
Experimental
Materials
Lime (C. aurantifolia) peel samples were obtained from Main Market, Bandung, Indonesia. Cotton fabric (100%) with a weight of 117 g m−2 was obtained from the Center for Textile in Bandung. Pro analysis grade chemicals like acetone, anhydrous sodium sulfate, citric acid, glacial acetic acid, glutaraldehyde, n-hexane, and sodium dihydrogen phosphate dihydrate were obtained from Merck. In addition, some technical grade chemicals such as gelatin, sodium alginate, and Tween-80 were acquired from PT. Subur Jaya Kimia in Bandung, Indonesia.
LO microencapsulation
The preparation of LO followed the procedure reported by Julaeha et al. (2018),7 while their encapsulation was performed according to the method reported by Devi et al. (2012)25 with modification. Initially, 2% gelatin solution in distilled water (140 mL) was poured into a beaker and stirred at 600 rpm, and maintained up to a temperature of 60 ± 1 °C. Then, Tween 80 (0.8 g) and LO (2.0 g) were added dropwise. Afterward, 2% sodium alginate solution in distilled water (40 mL) was added slowly and stirred for 15 minutes. Later on, the pH of the solution was adjusted to 3.75, using 2.5% (v/v) glacial acetic acid solution. The mixture was further cooled down to 5–10 °C using an ice-bath, and subsequently, glutaraldehyde (0.2 g) was gradually added. The mixture was then reheated up to 35 °C and further stirred for 3–4 hours. In the next step, the microcapsules were filtered from the solution at 25 °C, washed with n-hexane, and finally dried and frozen in the refrigerator.
Microcapsule characterization
Oil content measurement. The oil content of microcapsules was determined from the standard curve of LO in n-hexane by employing UV-Vis spectrophotometry at 230 nm. The microcapsules were ground in a mortar, and then n-hexane (5 mL) was added to extract the LO excreted from the microcapsules. Afterward, the absorbance of the solution was measured and plotted to obtain the standard curve. The data was used to calculate the oil content (OC) and encapsulation efficiency (EE), using eqn (1) and (2). |
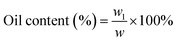 | (1) |
|
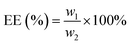 | (2) |
where w = weight of microcapsules; w1 = the actual amount of coated oil, w2 = amount of added oil.
Particle size measurement. Particle size measurements were performed using PSA Beckman Coulter LS 13 320, equipped with optical Fraunhofer and water-carrying liquid.
Morphological measurement. Morphological measurements were conducted through a JEOL JSM-6510 SEM apparatus with magnifications of 500×, 1000×, and 1500×, as well as 10 kV electricity and BEI (Backscatter Electron Image) detector.
Fourier transform infrared (FTIR). FTIR study was carried out on a PerkinElmer spectrum-100 brand in the range of 450–4000 cm−1.
Thermal stability. The thermal stability was studied using a thermogravimetric analyzer (TGA) instrument of TG 209 F1 Libra brand, NETZSCH Germany at a temperature range of 30–750 °C and 20 °C per minute heating rate in nitrogen atmosphere.
Immobilization of microcapsules in cotton fabric. Microcapsule immobilization onto the fabric was initially performed by preparing a sample of 30 cm × 40 cm cotton fabric. Microcapsule, binder, and catalyst solutions were also formulated. The solution attained a 100 mL volume comprising 20% microcapsules, 2% citric acid binder, 1.5% sodium dihydrogen phosphate dihydrate catalyst, and Tween-80 (0.8 g). In addition, the solution was stirred to reach equilibrium, and then the cotton fabric was introduced into the solution for 5–10 minutes with stirring. Subsequently, the sample was squeezed using a padder (Werner Mathis AG) and dried on a HT steam curing machine (Werner Mathis AG) for 2 minutes at 100 °C, where the curing process also occurred at 130 °C for 2 minutes. The product sample was then characterized by SEM and further tested for washing resistance using a Launder-O-meter. Furthermore, the material was tested for its antibacterial activity based on the SNI ISO 105-C06: 2010 test standard with two repetitions. The weight gain by the fabric after treatment was calculated using eqn (4). |
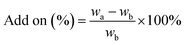 | (4) |
where wa = weight after treatment; wb = weight before treatment.Microcapsule immobilization onto the fabric was repeated by using a citric acid binder of 4% and 6%, independently.
Antibacterial activity. For the assay of antibacterial activity, we referred to the method reported by Sumathi et al. (2015),28 which employed the Kirby Bauer method (SNI ISO 105-C06: 2010 test standard). The assay was not only conducted on the modified fabric with LO microcapsules but also on LO and limonene for comparison. The microbial strains of commonly colonized skin bacteria, such as Gram-positive bacteria; Staphylococcus aureus (ATCC 6538), Staphylococcus epidermidis (ATCC 11228), and Gram-negative bacteria; Escherichia coli (ATCC 11229), and Klebsiella pneumoniae (ATCC 2357) were preferentially selected for the assay. Initially, each microbial strain was cultured overnight at 105 CFU mL−1 in disinfected saline solution. Then, 500 μL bacterial suspension was laid over the agar media containing MHA for Gram-positive bacteria and NA for Gram-negative bacteria. The sample of the modified fabric (6.0 ± 0.1 mm) or 20 μL of LO sample impregnated on a sterile blank disc was placed onto the media plate and incubated at 37 °C for 24 hours, and the antimicrobial activity was then measured based on the zone of inhibition (ZoI/mm). In addition, ampicillin of 1000 ppm per disc and n-hexane were used as positive and negative control, respectively.
Conclusions
The LO microcapsules were prepared with a yield, encapsulation efficiency (EE), and oil content (OC) of 47 ± 4%, 84 ± 11%, and 58 ± 4%, respectively. The morphology of the capsules was spherical and soft, with an average particle size of 1.554 μm. 79% LO was released in 2 hours upon applying mechanical and chemical force. The immobilization of the LO microcapsules onto the fabric was optimized at 4% citric acid binder with 3% loss of mass after 15 cycles of washing. Antibacterial activities of the optimized functional fabric (4% binder) exhibited the highest inhibition, among others, against S. aureus, E. coli, K. pneumoniae, and S. epidermidis.
Conflicts of interest
There are no conflicts to declare.
Acknowledgements
The authors are grateful to the Ministry of Research, Technology and Higher Education for providing PDUPT grant (No. 1827/UN6.3.1/LT/2020), and also Universitas Padjadjaran for ALG of Prof. Tati Herlina (No. 1397/UN6.3.1/PM/2020). The gratitude was also appreciated to the Head of Center for Textile for effective research collaboration and facilities.
References
- H. S. Elshafie and I. Camele, BioMed Res. Int., 2017, 1–14 Search PubMed.
- M. S. Al-Aamri, N. M. Al-Abousi, S. S. Al-Jabri, T. Alam and S. A. Khan, J. Taibah Univ. Medical Sci., 2018, 13, 108–112 Search PubMed.
- F. Firenzuoli, V. Jaitak, G. Horvath, I. H. N. Bassolé, W. N. Setzer and L. Gori, Evid.-Based Complementary Altern. Med., 2014, 1–2 Search PubMed.
- L. Y. Lin, C. H. Chuang, H. C. Chen and K. M. Yang, Foods, 2019, 8, 398 CrossRef CAS.
- N. Mahato, K. Sharma, R. Koteswararao, M. Sinha, E. Baral and M. H. Cho, Crit. Rev. Food Sci. Nutr., 2017, 59, 611–625 CrossRef.
- P. Kumari, N. M. Rose and S. S. I. Singh, Ann. Agri Bio. Res., 2015, 20, 152–157 Search PubMed.
- E. Julaeha, R. Nugeraha, M. Nurzaman, D. Kurnia, T. Wahyudi and Y. Rosandi, J. Phys. Conf. Ser., 2018, 1080, 1–5 CrossRef.
- M. S. Stan, L. Chirila, A. Popescu, D. M. Radulescu, D. E. Radulescu and A. Dinischiotu, Materials, 2019, 12, 1–15 CrossRef.
- A. Sharkawy, I. P. Fernandes, M. F. Barreiro, A. E. Rodrigues and T. Shoeib, Ind. Eng. Chem. Res., 2017, 56, 5516–5526 CrossRef CAS.
- M. M. M. Khodary, H. M. El-Rafie, H. M. A. Salam and M. H. El-Rafie, Int. J. Des., 2017, 7, 131–140 Search PubMed.
- M. Naveed, Trends Text. Eng. Fashion Technol., 2018, 2, 226–235 Search PubMed.
- P. B. Wijesirigunawardana and B. G. K. Perera, Acta Chim. Slov., 2018, 65, 150–159 CrossRef CAS.
- S. Abbasiliasi, T. J. Shun, N. Ismail, A. B. Ariff and S. Mustafa, RSC Adv., 2019, 9, 16147–16157 RSC.
- L. F. Siow and C. S. Ong, J. Food Process. Technol., 2013, 4, 1–5 Search PubMed.
- L. Gentile, Curr. Opin. Colloid Interface Sci., 2020, 48, 18–27 CrossRef CAS.
- M. Saravanan and K. P. Rao, Carbohydr. Polym., 2010, 80(3), 808–816 CrossRef CAS.
- R. Stojanovic, A. Belscak-Cvitanovic, V. Manojlovic, D. Komes, V. Nedovic and B. Bugarski, J. Sci. Food Agric., 2012, 2, 685–696 CrossRef.
- P. H. Campelo-Felix, H. J. B. Souza, J. A. Figueiredo, R. V. B. Fernandes, D. A. Botrel, C. R. Oliveira, M. I. Yoshida and S. V. Borges, J. Agric. Food Chem., 2017, 65, 445–453 CAS.
- S. Petr, K. Pavel and S. Vladimir, J. Agric. Food Chem., 2012, 60(16), 4111–4119 CrossRef.
- T. Zhang, Y. Luo, M. Wang, F. Chen, J. Liu, K. Meng and H. Zhao, Polymers, 2020, 12, 1651 CrossRef CAS.
- M. M. G. Fouda and H. M. Fahmy, Carbohydr. Polym., 2011, 86, 625–629 CAS.
- P. Monllor, L. Capablanca, J. Gisbert, P. Díaz, I. Montava and Á. Bonet, Text. Res. J., 2010, 80, 631–635 CrossRef CAS.
- S. Jafari, S. Eshafani, M. R. Fazeli, H. Jamalifar, M. Samadi, N. Samadi, A. N. Toosi, M. R. S. Ardekani and M. Khanavi, Int. J. Biol. Chem., 2011, 5(4), 258 CrossRef CAS.
- Z. Breijyeh, B. Jubeh and R. Karaman, Molecules, 2020, 25(6), 1340 CAS.
- N. Devi, D. Hazarika, C. Deka and D. K. Kakati, J. Macromol. Sci., Part A: Pure Appl.Chem., 2012, 49, 936–945 CrossRef CAS.
- Y. H. Yang, X. Z. Li and S. Zhang, RSC Adv., 2018, 8, 29980–29987 RSC.
- F. Kayaci and T. Uyar, J. Agric. Food Chem., 2011, 59, 11772–11778 CrossRef CAS.
- S. Sumathi, T. Ancy and E. G. Wesely, Int. J. Biol. Pharm. Res., 2015, 6(4), 259–263 Search PubMed.
|
This journal is © The Royal Society of Chemistry 2021 |
Click here to see how this site uses Cookies. View our privacy policy here.