DOI:
10.1039/D0RA10253A
(Paper)
RSC Adv., 2021,
11, 9067-9075
Supercritical CO2 extraction of Marrubium vulgare: intensification of marrubiin†
Received
4th December 2020
, Accepted 22nd February 2021
First published on 1st March 2021
Abstract
Supercritical carbon dioxide and Soxhlet extraction were employed for delivering Marrubium vulgare extracts. By varying process temperature and pressure, the impact of different densities of carbon dioxide on the extraction yield and chemical profile of the extracts was investigated. The highest extraction yields of M. vulgare were obtained by supercritical carbon dioxide extraction (3.51%) at 300 bar and 60 °C, while the yield obtained with the Soxhlet extraction was 3.23%. The chemical profiles of these two extracts were significantly different and marrubiin, the most dominant component of supercritical extracts was not present in extracts obtained by this conventional method. This labdane diterpene was the most abundant in extracts obtained at 200 bar and temperatures of 40, 50, and 60 °C (62.60, 68.41, and 71.96%, respectively). For the intensification of marrubiin, supercritical fractions were collected in successive time intervals over the course of the extraction (300 bar/60 °C). It was determined that after 1 h of extraction the highest content of marrubiin (75.14%) can be achieved. The similarities between the obtained extracts were estimated and the correlations to the content of identified lipophilic compounds were established using multivariate data analysis tools.
Introduction
Marrubium vulgare L., also known as white horehound, is a perennial plant that belongs to the Lamiaceae family and genus Marrubium and is commonly applied in traditional medicine. Due to its bitter taste, it is used to treat numerous disorders of the digestive tract. It is also consumed as a remedy for respiratory tract diseases.1 The therapeutic properties of M. vulgare which include anti-inflammatory, hypoglycemic, analgesic, antispasmodic, vasorelaxant, anticoagulant, and antidiabetic effects are partly attributed to marrubiin (Fig. 1). Marrubiin was isolated for the first time from its leaves and is considered a chemotaxonomic marker for the genus Marrubium.2 Furthermore, this important secondary metabolite is conventionally extracted with acetone in yields of up to 1%, but different samples of the herb provide greatly differing amounts. This can be a consequence of the harvesting season, geographical origin, plant development stage, the way and time of drying, and storage.3 Additionally, M. vulgare belongs to the species that are not characterized as rich in content of essential oil (0.05–0.06%).4,5 Despite the low yield of essential oil, different conventional extraction techniques including hydrodistillation, steam distillation, and solvent extraction have been employed for its attainment due to the essential oil's high pharmacological potential and its most significant constituent marrubiin.6 In addition, several attempts were made to develop an extraction methodology suitable for an exhaustive extraction of marrubiin from this species. Furthermore, efforts were made to intensify the essential oil extraction yield by applying the pulsed electric field as a pretreatment.7 Additionally, optimization of process variables of hydrodistillation and Soxhlet extraction was conducted including temperature, drying and grinding the plant, the solvent to solid mass ratio, the contact-time, and the nature of the solvent.8
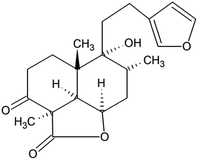 |
| Fig. 1 Chemical structure of marrubiin. | |
In addition to generating large amounts of liquid waste, the disadvantages of conventional processes such as the Soxhlet extraction are insufficient selectivity, time-consuming procedure, and potential emission of toxic vapors during the extraction.9 In order to overcome these shortcomings and isolate components from complex matrices, green technologies have been developed. With regard to contemporary methods for the extraction of M. vulgare, microwave-assisted extraction with 50% ethanol was applied. Moreover, extraction parameters including microwave power, irradiation time, and solvent to drug ratio were optimized to intensify the concentration of marrubiin.10
Supercritical fluid extraction which is a green method superior to the processes of hydrodistillation and Soxhlet has attracted considerable attention in recent decades as a promising alternative to conventional extraction technologies and mechanical compression for obtaining lipophilic extracts. The most commonly used fluid in supercritical fluid extraction is carbon dioxide (CO2). Moreover, this fact is attributed to its properties which include chemical inertness, non-flammability, mild supercritical condition, and availability.11 On the other hand, the drawback of CO2 is that it is adequate for non-polar components. However, this can be overcome by adding a polar modifier. Numerous studies demonstrated that the extraction with CO2 in supercritical state is efficient for extracting bioactive compounds from various natural materials such as medicinal plants, algae, food waste and by-products.12 In addition, supercritical CO2 (SC) extraction offers benefits which include complete removal of solvents residues in products, and more efficient and selective isolation of compounds.12–15 Although SC has been investigated extensively for the recovery of bioactive compounds from numerous medicinal plants, there are no unique SC parameters for the optimal recovery of bioactive constituents from different types of natural materials due to the significant differences in material type, way of cultivation, and other factors.
SC has not been applied for the extraction of white horehound. Therefore, the main aim of this study was to investigate for the first time the SC extraction of M. vulgare lipophilic compounds. Additionally, the study aimed to establish the optimal parameters for marrubiin isolation. To determine the efficacy of this alternative technique for the extraction of M. vulgare, conventional Soxhlet extraction was conducted and used as a reference method. The differences in the distribution of marrubiin and other detected lipophilic compounds in white horehound extracts were investigated by applying the multivariate data analysis.
Results and discussion
Extraction yield
In order to isolate the lipophilic components of M. vulgare, SC was applied in addition to the conventional Soxhlet extraction. The influence of different pressure and temperature on the extraction yield (g of obtained extract per 100 g of dry herbal material) and chemical composition of white horehound extracts was examined. Furthermore, the yield of Soxhlet extraction of M. vulgare (3.23%) was comparable to the yield of SC (3.51%) achieved at 300 bar and 60 °C. By observing the Soxhlet extraction as a reference method, the obtained results suggested that SC achieved complete and more efficient exhaustion of the lipophilic fraction of M. vulgare. The lower yield of the Soxhlet extraction can be caused by extended exposure to high temperature. Fig. 2a–c (ESI-Table 1†) demonstrate that with increasing pressure, the total extraction yield increases at temperatures 40 and 60 °C. Moreover, this is in line with the statement that increasing the CO2 density also increases its dissolving power.16 At 50 °C temperature, no significant was recorded in the total extraction yield obtained at 200 and 300 bar. Also, at pressures of 200 and 300 bar, two extraction periods can be observed: (1) the period of fast extraction during which easily accessible solutes in the supercritical fluid are extracted, and (2) the period of slow extraction during which components of higher boiling point and higher molecular weight such as fatty oils, pigments, and waxes are extracted.17 By observing the isobaric process at the lowest pressure of 100 bar, the highest yield was achieved at the lowest temperature of 40 °C which is also in accordance with the fact that applying CO2 of higher density positively affects the yield. On the other hand, a positive correlation between the extraction yield and temperatures was noted at higher pressures, that is, a negative correlation with the density of the CO2. Hence, 60 °C temperature was the most adequate for achieving the highest yield at pressures 200 and 300 bar. At constant pressure and with temperature increase, the density of CO2 decreased which also lowers its solvatation ability. However, as a consequence of the lower viscosity of CO2, diffusion is improved which further enhances the mass transfer.18 Westerman et al.19 explained this influence of temperature with the enhanced solubility effect. More specifically, the rise in the solute's vapour pressure outweighs the solvent power reduction as a result of reduced density. Ivanovic et al.20 observed that the model parameter related to solvent diffusion in the solid phase increases with supercritical CO2 density from 290 to 630 kg m−3, while above 630 kg m−3 it decreases. The authors stated that a possible reason behind this lies in the fact that a higher amount of solute in supercritical CO2 defined by the increase of extract's solubility at densities higher than 630 kg m−3 also requires more time for diffusion of extract from solid matrix to supercritical phase. Furthermore, extraction kinetics are affected negatively as the residence time of CO2 may be extended when CO2 density is increased above 630 kg m−3.20
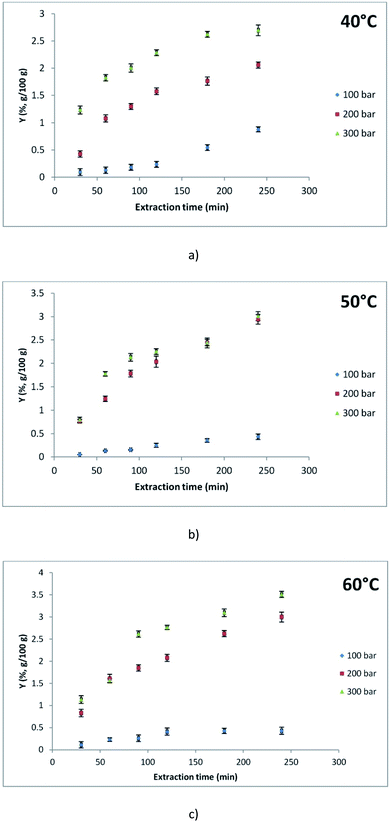 |
| Fig. 2 Extraction kinetics at different pressures (100, 200, and 300 bar) at (a) 40 °C, (b) 50 °C, and (c) 60 °C. | |
Chemical composition of lipophilic extracts
To determine the chemical profile of M. vulgare extracts obtained by SC and Soxhlet extractions, the GC-MS analysis was applied (Tables 1–4), and the peak area was used to determine the relative content of compounds in samples. The presence of 20 components was confirmed in extracts obtained at different temperatures and 100 bar pressure, while at 200 and 300 bar pressure, 10 and 20 components were identified, respectively (Tables 2–4). In the Soxhlet extract in which the presence of 10 components was confirmed, the most dominant compounds where sesquiterpene hydrocarbons (34.31%) followed by the saturated fatty acid and palmitic acid with 31.18%, while oxidized monoterpenes, which include citric oxide and carvone, were present in 7.84% (Table 1). Among the sesquiterpenes, trans-caryophyllene had the highest content (8.37%). This compound might be considered as an alternative to synthetic drugs used for the treatment of inflammatory diseases as its anti-inflammatory effect on treated animals was comparable to that of dexamethasone.21 A similar content of trans-caryophyllene was determined in a supercritical extract obtained at 100 bar and 40 °C (9.16%). In the Soxhlet extract, the presence of marrubiin was not recorded. Considering that the marrubiin is soluble in methylene chloride and that it is a highly oxidized compound,3 the potential cause of the Soxhlet method inefficiency for the extraction of marrubiin can be the degradation due to exposure to high temperature during 6 h of extraction. Methylene chloride has boiling point approximately 40 °C, however, there is still possibility of compound decomposition due to the long period of extraction.22
Table 1 GC-MS analyses of Soxhlet horehound extract (area percentage (%) and retention time (TR))
Compound |
TR (min) |
Area percentage (%) |
Oxygenated monoterpenes |
Limonene oxide |
50.63 |
4.88 |
Carvone |
34.08 |
2.96 |
![[thin space (1/6-em)]](https://www.rsc.org/images/entities/char_2009.gif) |
Sesquiterpenes hydrocarbons |
trans-Caryophyllene |
28.12 |
8.37 |
Caryophylla-4(12),8(13)-dien-5β-ol |
30.18 |
4.42 |
Ledene oxide |
32.02 |
7.22 |
Salvialan |
56.29 |
7.34 |
Longipinen-12,15-diol |
27.95 |
6.96 |
![[thin space (1/6-em)]](https://www.rsc.org/images/entities/char_2009.gif) |
Fatty acid |
Palmitic acid |
41.63 |
31.18 |
![[thin space (1/6-em)]](https://www.rsc.org/images/entities/char_2009.gif) |
Aliphatic hydrocarbons |
Tricyclo[6.3.0.0(3,7)]undec-1(8)-an-3-ol, 2,2,5,5-tetramethyl |
48.59 |
18.08 |
2-Tridecanone |
37.67 |
2.92 |
![[thin space (1/6-em)]](https://www.rsc.org/images/entities/char_2009.gif) |
Total |
|
94.33 |
Unknown |
|
5.67 |
Table 2 GC-MS analysis of SC horehound extracts obtained at 100 bar (area percentage; %) at different temperatures (40 and 50 °C) and extraction time 4 h
Compound |
Temperature (°C) |
40 |
50 |
Oxygenated monoterpenes |
Carvacrol |
1.04 |
0.46 |
![[thin space (1/6-em)]](https://www.rsc.org/images/entities/char_2009.gif) |
Sesquiterpene hydrocarbons |
trans-Caryophyllene |
9.16 |
5.39 |
α-Humulene |
0.69 |
0.45 |
Germacrene-D |
11.71 |
7.38 |
Bicyclogermacrene |
9.43 |
4.81 |
α-Cedrol |
0.34 |
— |
Spathulenol |
4.77 |
3.30 |
Caryophyllene oxide |
2.69 |
1.34 |
Viridiflorol |
1.50 |
0.73 |
![[thin space (1/6-em)]](https://www.rsc.org/images/entities/char_2009.gif) |
Aliphatic hydrocarbons |
(E,Z)-3,8-Dimethyldeca-4,6-dien |
0.54 |
— |
6,10,14-Trimethyl-2-pentadecanone |
1.36 |
1.22 |
Nonadecane |
1.87 |
— |
Tricosane |
— |
1.62 |
![[thin space (1/6-em)]](https://www.rsc.org/images/entities/char_2009.gif) |
Fatty acids |
Palmitic acid |
15.81 |
11.24 |
Linoleic acid |
2.09 |
2.01 |
9,12,15-Octadecatrienoic acid, methyl ester |
3.48 |
3.90 |
![[thin space (1/6-em)]](https://www.rsc.org/images/entities/char_2009.gif) |
Diterpenes |
Phytol |
2.05 |
2.20 |
Labda-8(17), 13E-dien-15-al |
2.20 |
1.77 |
Marrubiin |
4.32 |
30.18 |
7,8-Epoxy-α-dihydroionone |
— |
11.41 |
![[thin space (1/6-em)]](https://www.rsc.org/images/entities/char_2009.gif) |
Total |
65.66 |
89.41 |
Unknown |
34.34 |
10.59 |
In the study by Mittal and Nanda,10 Soxhlet extraction of M. vulgare aerial parts with ethanol was applied and the obtained yield of 11.27% had a low marrubiin yield (0.69%). Additionally, the time-consuming Soxhlet extraction (18 h) and the drug/solvent ratio 1
:
50 (m/v) which suggests a high solvent consumption point to the inferiority of this conventional technique compared to the microwave-assisted extraction. The authors stated that the yield of marrubiin doubled with the application of optimal conditions of the microwave extraction compared to Soxhlet.10
The area percentages of compounds identified in supercritical extracts of M. vulgare obtained at 100 bar and temperatures of 40 and 50 °C are shown in Table 2. The chemical profile of the extract at 60 °C is not presented due to an extremely low extraction yield achieved under these conditions, insufficient for chemical analysis. The most likely reason for such a large difference in the extraction yields achieved at 100 bar and temperatures of 40 and 60 °C is a large difference in the density of CO2 which is 628.7 kg m−3 at 40 °C and 290 kg m−3 at 60 °C.
The percentages of total identified components in supercritical extracts varied depending on the applied pressure and temperature and ranged from 65.66–89.41% at 100 bar, 76.39–80.66% at 200 bar, and 61.98–92.26% at 300 bar.
The components identified in the extracts can be classified into the following groups: oxidized monoterpenes, sesquiterpene hydrocarbons, aliphatic hydrocarbons, fatty acids, and diterpenes. Based on the obtained results, extract of M. vulgare obtained at 100 bar and 40 °C had the most sesquiterpene hydrocarbons (40.29%) which include trans-caryophyllene, α-humulene, germacrene D, bicyclogermacrene, α-cedrol, spathulenol, caryophyllene oxide, and viridiflorol. Other authors also detected trans-caryophyllene, α-humulene, germacrene D, caryophyllene oxide, and viridiflorol in M. vulgare essential oil.23,24 Furthermore, the importance of detected sesquiterpenes has also been established. Germacrene D, which is a constituent of juniper species essential oil, exerts antimicrobial activity against Staphylococcus aureus and Bacillus cereus.25 Natural bicyclic sesquiterpenes, β-caryophyllene, and β-caryophyllene oxide possess significant anticancer activities, affecting the growth and proliferation of numerous cancer cells. Caryophyllene oxide was found to exhibit anti-inflammatory, antioxidant, antiviral, and analgesic properties.26–28 In addition, carvacrol represents a highly important monoterpene which is used as a food flavoring ingredient and preservative in the food industry and a fragrance ingredient in the cosmetic industry. Carvacrol possesses wide specter of pharmacological activities such as antimicrobial, antioxidant, and anticancer activities.29 The extract obtained at 40 °C and 100 bar contained 4.32% marrubiin. However, by increasing the temperature to 50 °C while maintaining the same 100 bar pressure, the content of marrubiin was increased approximately sevenfold (30.18%) making it the most dominant component of the extract.
By applying a higher pressure of 200 bar, the content of marrubiin was significantly increased by 2.5 times. M. vulgare extracts obtained at 200 bar and 40–60 °C temperatures had the most labdan diterpene marrubiin (62.60, 68.41, and 71.96%, respectively), followed by sesquiterpene hydrocarbons (7.26, 5.77, and 4.2%, respectively) which include trans-caryophyllene, germacrene D, bicyclogermacrene, spathulenol, and caryophyllene oxide. Oxidized monoterpenes were not identified (Table 3). As the temperature was increased, the relative percentages of all sesquiterpenes decreased because the density of CO2 also decreased from 839.90 to 723.80 kg m−3 and thus its dissolution power. Saturated fatty acid – palmitic acid, was present in the composition of all three extracts and its content also decreased with increasing the temperature (5.02, 3.77, and 2.18%). Unsaturated ω-6 fatty acid – linoleic acid, was present in all three extracts but in a significantly smaller proportion than palmitic acid (0.79, 0.56, and 0.74%).
Table 3 GC-MS analysis of SC horehound extracts obtained at 200 bar (area percentage; %) at different temperatures (40–60 °C) and extraction time 4 h
Compound |
Temperature (°C) |
40 |
50 |
60 |
Sesquiterpene hydrocarbons |
trans-Caryophyllene |
1.79 |
1.44 |
1.09 |
Germacrene-D |
2.32 |
1.77 |
1.27 |
Bicyclogermacrene |
1.62 |
1.23 |
0.82 |
Spathulenol |
1.04 |
0.91 |
0.69 |
Caryophyllene oxide |
0.49 |
0.42 |
0.33 |
![[thin space (1/6-em)]](https://www.rsc.org/images/entities/char_2009.gif) |
Aliphatic hydrocarbons |
Hexadecane |
— |
— |
1.20 |
![[thin space (1/6-em)]](https://www.rsc.org/images/entities/char_2009.gif) |
Fatty acids |
Palmitic acid |
5.02 |
3.77 |
2.18 |
Linoleic acid |
0.79 |
0.56 |
0.74 |
![[thin space (1/6-em)]](https://www.rsc.org/images/entities/char_2009.gif) |
Diterpenes |
Phytol |
0.72 |
0.60 |
0.38 |
Marrubiin |
62.60 |
68.41 |
71.96 |
![[thin space (1/6-em)]](https://www.rsc.org/images/entities/char_2009.gif) |
Total |
76.39 |
79.11 |
80.66 |
Unknown |
23.61 |
20.89 |
19.34 |
When the highest pressure of 300 bar was applied, the lower content of marrubiin was obtained compared to 200 bar, but it was higher compared to 100 bar (30.50, 23.74, and 58.39% for extracts obtained at temperatures 40, 50, and 60 °C, respectively) (Table 4).
Table 4 GC-MS analysis of SC horehound extracts obtained at 300 bar (area percentage; %) at different temperatures (40–60 °C) and extraction time 4 h
Compound |
Temperature (°C) |
40 |
50 |
60 |
Oxygenated monoterpenes |
Carvacrol |
0.30 |
0.29 |
0.32 |
![[thin space (1/6-em)]](https://www.rsc.org/images/entities/char_2009.gif) |
Sesquiterpene hydrocarbons |
trans-Caryophyllene |
3.55 |
1.04 |
2.27 |
Germacrene-D |
4.07 |
1.32 |
2.61 |
Bicyclogermacrene |
2.34 |
0.86 |
1.79 |
Spathulenol |
1.85 |
0.81 |
1.35 |
Caryophyllene oxide |
0.77 |
0.37 |
0.66 |
![[thin space (1/6-em)]](https://www.rsc.org/images/entities/char_2009.gif) |
Aliphatic hydrocarbons |
Octadecane |
13.54 |
— |
4.23 |
Tricosane |
— |
— |
1.22 |
Nonacosane |
— |
— |
3.90 |
Docosane |
9.64 |
22.03 |
1.33 |
Nonadecane |
4.10 |
5.58 |
2.96 |
Tetracosane |
14.98 |
— |
— |
![[thin space (1/6-em)]](https://www.rsc.org/images/entities/char_2009.gif) |
Fatty acids |
Palmitic acid |
5.24 |
4.61 |
6.56 |
![[thin space (1/6-em)]](https://www.rsc.org/images/entities/char_2009.gif) |
Diterpenes |
Phytol |
1.06 |
0.99 |
0.79 |
Labda-8(17),13E-dien-15-al |
0.32 |
— |
0.37 |
Marrubiin |
30.50 |
23.74 |
58.39 |
7,8-Epoxy-α-dihydroionone |
— |
0.34 |
1.85 |
![[thin space (1/6-em)]](https://www.rsc.org/images/entities/char_2009.gif) |
Total |
92.26 |
61.98 |
90.60 |
Unknown |
7.74 |
38.02 |
9.4 |
The largest proportions of sesquiterpene hydrocarbons were obtained at a temperature of 40 °C, while the smallest were obtained at 50 °C temperature under isobaric extraction conditions. According to Pourmortazavi and Hajimirsadeghi,30 SC at higher temperatures reduces the yield of non-volatile compounds, as well as the competition between solubility and volatility of compounds. Therefore, the relatively high extraction yield achieved at 40 °C (92.26%) decreased at 50 °C (61.98%) and increased again with an increase in operating temperature to 60 °C (90.60%). Palmitic acid was present in all three extracts (5.24, 4.61, and 6.56%). With regard to oxidized monoterpenes, the presence of carvacrol was confirmed in all three extracts at 300 bar with similar relative proportions (0.30, 0.29, and 0.32%).
The most dominant component in all obtained supercritical extracts was the chemotaxonomic marker marrubiin, except for the extract obtained at 100 bar and 40 °C. The relative content of this diterpene in the extracts obtained at a pressure of 200 bar was 62.60–71.96% and in the extracts obtained at 300 bar, it was 23.74–58.39%. Therefore, the most favorable parameters for extraction of marrubiin are 200 bar pressure and 60 °C temperature (Fig. 3). Moreover, Soxhlet demonstrated to be an inadequate technique for the recovery of marrubiin. In addition, compared to the yield of marrubiin (1.35%) obtained by microwave-assisted extraction with ethanol in the Mittal and Nanda10 study, it can be concluded that the SC extraction is a more adequate and efficient method for the recovery of marrubiin compared to the traditional Soxhlet extraction with organic solvents and the microwave-assisted extraction with ethanol.
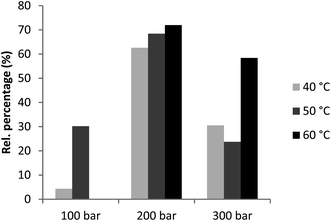 |
| Fig. 3 Area percentages (%) of marrubiin in supercritical horehound extracts obtained at different pressures and temperatures. | |
At selected pressure and temperature (300 bar, 60 °C) which provided the highest extraction yield, supercritical fractions were collected in successive time intervals (0.5, 1, 1.5, 2, 3, and 4 h) over the course of the extraction and analyzed by GC-MS in reference to marrubiin content (Fig. 4). The procedure aimed to investigate the most favorable conditions for the extraction of marrubiin and report the quantitative differences among fractions collected during successive time intervals. The highest content of marrubiin (75.14%) was obtained after 1 h of extraction which is of high importance from the economic perspective. Additionally, it facilitates the scale-up of the process. Subsequently, the content of marrubiin gradually decreased to 44.39% after 4 h of extraction when SC was completed.
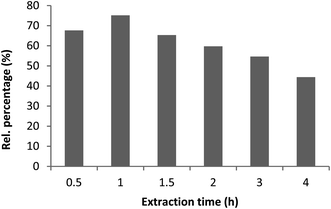 |
| Fig. 4 Extraction kinetics at 300 bar and 60 °C in relation to the area percentage of marrubiin. | |
Correspondence and canonical correspondence analysis
Correspondence analysis (CA) analysis was employed in order to visually explore the similarities between the white horehound extracts obtained using Soxhlet and SC extraction under various extraction conditions (pressure and temperature). The score plot obtained using all detected lipophilic compounds as variables is shown in Fig. 5.
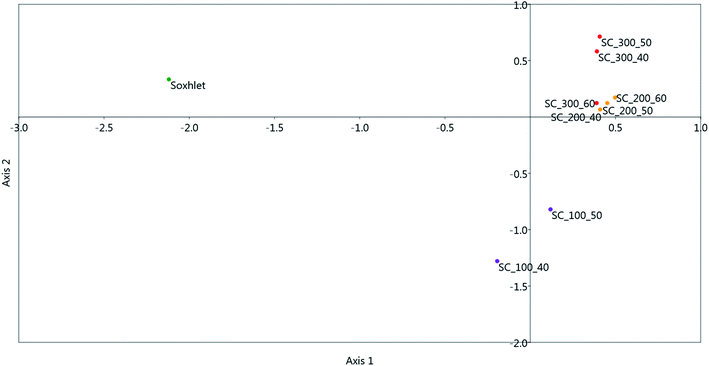 |
| Fig. 5 Correspondence analysis (CA) score plot of extracts obtained using Soxhlet and SC under various conditions (pressure and temperature). | |
Fig. 5 demonstrates that the white horehound extract obtained using Soxhlet extraction exhibits significant differences in qualitative and quantitative content of the compounds identified using the GC-MS system, whereas extracts obtained using SC at higher pressures (200 and 300 bar) exhibit more similarities, regardless of the applied extraction temperature.
In order to visually improve distributions between white horehound SC extracts, and to investigate the correlations between samples and identified chemical groups (diterpenes, aliphatic hydrocarbons, fatty acids, and sesquiterpenes), a sample obtained using Soxhlet extraction was removed as an outlier. Thus the obtained score plot of canonical correspondence analysis (CCA) is presented in Fig. 6a–d. Carvacrol was the only oxygenated monoterpene identified in SC extracts and its highest content was measured in an extract obtained under the mildest extraction conditions: pressure of 100 bar and temperature of 40 °C.
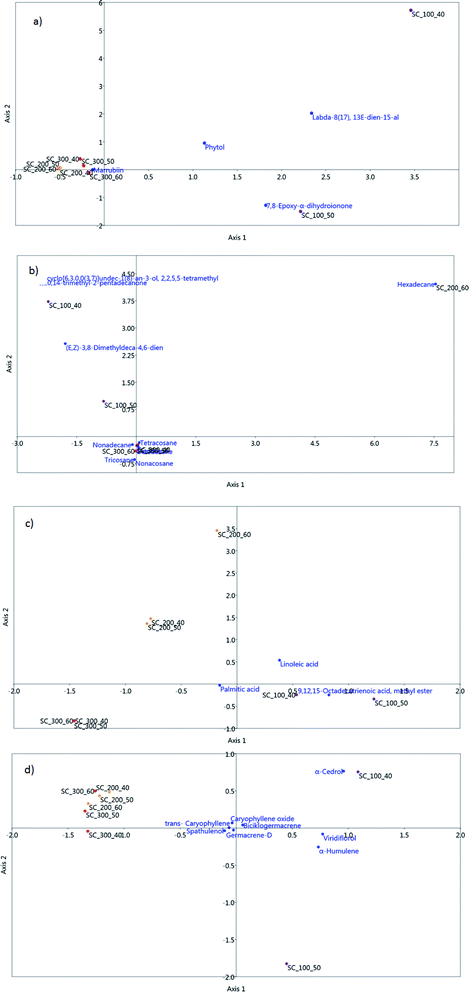 |
| Fig. 6 Cannonical correspondence analysis (CCA) bi-plot of SC extracts obtained under various pressure and temperature conditions showing the distribution of all compound classes used as variables: (a) – diterpenes; (b) – aliphatic hydrocarbons; (c) – fatty acids; (d) – sesquiterpenes. | |
Fig. 6a shows the distribution of diterpenes in the obtained white horehound SC extracts. The compound 7,8-epoxy-α-dihydroionone was the most abundant in the extract obtained at 100 bar and 50 °C, while marrubiin was most abundant in SC extracts obtained at higher pressures.
Compounds that belong to aliphatic hydrocarbons (tricyclo[6.3.0.0(3,7)]undec-1(8)-an-3-ol, 2,2,5,5-tetramethyl, (E,Z)-3,8-dimethyldeca-4,6-dien, and 6,10,14-trimethyl-2-pentadecanone) were present in samples obtained at the lowest extraction pressure of 100 bar, hexadecane was found in the 200 bar/60 °C extract, while longer chain aliphatic hydrocarbons (octadecane, nonadecane, docosane, tricosane, tetracosane, and nonacosane) were highly abundant in the SC extracts obtained at higher pressures of 200 and 300 bar (Fig. 6b). All detected fatty acids (palmitic, linoleic, and linolenic acid) had higher concentrations in SC extracts obtained at the lowest pressure of 100 bar (Fig. 6c).
Fig. 6d shows the distribution of sesquiterpenes in the obtained white horehound SC extracts. These chemical groups had higher concentrations in a sample obtained at the lowest pressure and temperature conditions of 100 bar and 40 °C, followed by a sample obtained at 100 bar and 50 °C.
Experimental
Plant material
M. vulgare aerial parts were purchased from the local supplier of cultivated plants Chamomilla (Banatski Karlovac, Serbia). The dried M. vulgare aerial parts were grounded in a domestic blender and the mean particle size (0.28 mm) of grounded material was determined using vibration sieve sets (CISA, Cedaceria, Spain).
Chemicals
The carbon dioxide (Messer, Novi Sad, Serbia) with purity >99.98% (w/w) was used for the lab-scale SC extraction. Methylene chloride was used as a solvent in the Soxhlet extraction.
Soxhlet extraction
Grounded material (8 g) was transferred to an extraction thimble which was placed in a Soxhlet apparatus with a connected reflux condenser. Methylene chloride (150 mL) was then added to the flask and used as a solvent. After 6 h of extraction and complete exhaustion of the white horehound herbal material, the solvent was removed by evaporation on a rotary vacuum evaporator, and the extraction yield and chemical composition were determined. The extraction was performed in duplicate.
Supercritical carbon dioxide extraction (SC)
The laboratory-scale high pressure extraction plant (HPEP, NOVA-Swiss, Effretikon, Switzerland) was used for SC. The main specifications of the SC unit are as follows: gas cylinder with carbon dioxide (CO2), diaphragm type compressor (with a pressure range up to 1000 bar), extractor vessel with a heating jacket for heating medium (with internal volume 200 mL, maximum operating pressure of 700 bar), separator with a cooling jacket for cooling medium (with internal volume 200 mL and maximum operating pressure of 250 bar), pressure control valve, temperature regulation system, and regulation valves. The dry and grounded M. vulgare material (40 g) was extracted at different pressures (100, 200, and 300 bar) and temperatures (40, 50, and 60 °C), while the CO2 flow (0.3 kg h−1) and extraction time (4 h) were constant in all experiments. At the selected pressure and temperature (300 bar, 60 °C) at which the highest extraction yield was achieved, supercritical fractions were collected in successive time intervals (0.5, 1, 1.5, 2, 3, and 4 h) over the course of the extraction and analyzed by GC-MS in reference to marrubiin content. The pressure and temperature in the separator were 15 bar and 23 °C. All extracts that were obtained were placed in glass bottles, sealed, and stored at 4 °C prior to further analysis. Each extraction was performed in duplicate.
Gas chromatography-mass spectrometry (GC-MS) analysis of lipophilic extracts
Agilent 7890B gas chromatography together with a mass spectrometer (Agilent, Series 5977A, USA) were used for the analysis. The components of M. vulgare lipophilic extracts were separated on an HP-5MS capillary column (30 m × 0.25 mm, 0.25 μm) (19091 S – 433 UI-INT, Agilent Technologies, USA). Injector temperature was set at 250 °C with an injected sample of 3 μL in split mode of 1
:
50. Helium of 99.99% purity was used as a carrier gas, in a constant flow regime of 1 mL min−1. The following temperature program was set: 70 °C temperature (2 min) which was increased by 3 °C min−1 to reach 200 °C and maintained constant for 18 min. The separated components were analyzed with a mass spectrometry (electron energy of 70 eV) with a scanning range in the m/z range of 30–450. Temperature of the ion source was 230 °C, while the temperature of the quadrupole was 150 °C. Qualitative identifications of present compounds were performed using Wiley9 and NIST09 databases, as well as the literature data of retention indices calculated with C9–C25 alkanes. The analysis of each sample was performed in three replicates.
Multivariate data analysis
Correspondence analysis (CA) and canonical correspondence analysis (CCA) were both performed using PAST software (Natural History Museum, University of Oslo, Norway).31,32 The correspondence analysis routine finds the eigenvalues and eigenvectors of a matrix containing the Chi-squared distances between all rows, i.e. investigated white horehound extracts. The scatter plot allows one to see all data points (rows) plotted in the coordinate system given by the CA. CCA is the correspondence analysis of a sample (extract) matrix where each sample has given values for one or more environmental variables – identified lipophilic compounds. The ordination axes are linear combinations of the environmental variables.33
Conclusions
Supercritical extraction methodology proved to be suitable for extracting marrubiin from white horehound efficiently. Optimal pressure and temperature values for its isolation are 200 bar and 60 °C, while more accurate quantitative analysis is needed to confirm the marrubiin content. These results were supported by the application of a multivariate statistical approach (CCA). Taking into account that during the first hour of the extraction more than 75% of marrubiin was extracted, this opens up new possibilities towards the scale-up of this green approach for efficient exploitation of M. vulgare as a source of marrubiin. Apart from marrubiin, the presence of other significant components that are applied in the food, cosmetic, and pharmaceutical industries was also confirmed.
Conflicts of interest
The authors confirm that they have no conflicts of interest with respect to the work described in this manuscript.
Acknowledgements
The authors would like to thank Ana Jovanoski for her technical and editorial support and Kristian Pastor, PhD for assistance with the statistical data treatment. This research was funded by the Ministry of Education, Science and Technological Development of Republic of Serbia; Project No. 451-03-68/2020-14/200134.
References
- S. A. Dar, A. Bhushan and P. Gupta, in Botanical Leads for Drug Discovery, Springer, Singapore, 2020, pp. 255–275 Search PubMed.
- O. K. Popoola, A. M. Elbagory, F. Ameer and A. A. Hussein, Molecules, 2013, 18(8), 9049–9060 CrossRef CAS.
- W. Cocker, B. E. Cross, S. R. Duff, J. T. Edward and T. F. Holley, J. Chem. Soc., 1953, 2540–2548 RSC.
- EMA/HMPC/604271/2012, Assessment report on Marrubium vulgare L., herba, Committee on Herbal Medicinal Products (HMPC) Search PubMed.
- ESCOP monographs: The Scientific Foundation for Herbal Medicinal Products, Marrubii herba, White horehound, ed. S. Mills and R. Hutchins, 2013 Search PubMed.
- S. Rezazi, S. Hanini, C. Si-Moussa and S. Abdelmalek, J. Essent. Oil-Bear. Plants, 2016, 19(4), 843–853 CrossRef.
- K. Miloudi, A. Hamimed, Y. Benmimoun, Y. Bellebna, A. Taibi and A. Tilmatine, J. Essent. Oil-Bear. Plants, 2018, 21(3), 811–824 CrossRef CAS.
- S. Rezazi, S. Abdelmalek and S. Hanini, Energy Procedia, 2017, 139, 98–104 CrossRef CAS.
- S. Vidović, S. Simić, A. Gavarić, M. Aćimović and J. Vladić, J. Nat. Med., 2020, 20, 22–26 Search PubMed.
- V. Mittal and A. Nanda, Pharm. Biol., 2017, 55(1), 1337–1347 CrossRef CAS.
- V. Nadalin, Z. Lepojevic, M. Ristic, J. Vladic, B. Nikolovski and D. Adamovic, Chem. Ind. Chem. Eng. Q., 2014, 20(1), 71–86 CrossRef CAS.
- M. Herrero, A. Cifuentes and E. Ibañez, Food Chem., 2006, 98(1), 136–148 CrossRef CAS.
- N. N. Norulaini, A. Ahmad, F. M. Omar, A. A. S. Banana, I. M. Zaidul and M. O. A. Kadir, Sep. Purif. Technol., 2008, 60(3), 272–277 CrossRef CAS.
- J. Vladić, A. Gavarić, S. Jokić, N. Pavlović, T. Moslavac, L. Popović, A. Matias, A. Agostinho, M. Banožić and S. Vidović, Acta Chim. Slov., 2020, 67(3), 778–784 CrossRef.
- I. S. M. Zaidul, N. N. Norulaini, A. M. Omar and R. L. Smith, J. Food Eng., 2007, 79(3), 1007–1014 CrossRef CAS.
- I. Jerković, M. Molnar, S. Vidović, J. Vladić and S. Jokić, Phytochem. Anal., 2017, 28(6), 558–566 CrossRef.
- H. Sovová, Chem. Eng. Sci., 1994, 49(3), 409–414 CrossRef.
- O. Benito-Román, M. Rodríguez-Perrino, M. T. Sanz, R. Melgosa and S. Beltrán, J. Supercrit. Fluids, 2018, 139, 62–71 CrossRef.
- D. Westerman, R. C. D. Santos, J. A. Bosley, J. S. Rogers and B. Al-Duri, J. Supercrit. Fluids, 2006, 37(1), 38–52 CrossRef CAS.
- J. Ivanovic, M. Ristic and D. Skala, J. Supercrit. Fluids, 2011, 57(2), 129–136 CrossRef CAS.
- E. S. Fernandes, G. F. Passos, R. Medeiros, F. M. da Cunha, J. Ferreira, M. M. Campos, L. Pianowski and J. B. Calixto, Eur. J. Pharmacol., 2007, 569(3), 228–236 CrossRef CAS.
- M. A. López-Bascón and M. L. de Castro, in Liquid-Phase Extraction, Elsevier, 2020, pp. 327–354 Search PubMed.
- W. Elbali, A. Djouahri, Z. Djerrad, B. Saka, S. Aberrane, N. Sabaou, A. Baaliouamer and L. Boudarene, J. Essent. Oil Res., 2018, 30(6), 470–487 CrossRef CAS.
- A. R. Golparvar, A. Hadipanah, A. M. Mehrabi and A. Armin, J. Herb. Drug., 2015, 6(1), 1–5 Search PubMed.
- V. D. Zheljazkov, M. Kacaniova, I. Dincheva, T. Radoukova, I. B. Semerdjieva, T. Astatkie and V. Schlegel, Ind. Crops Prod., 2018, 124, 449–458 CrossRef CAS.
- T. P. Singh, R. K. Singh and P. Malik, Journal of Scientific and Innovative Research, 2014, 3, 60–64 Search PubMed.
- Y. T. Tung, M. T. Chua, S. Y. Wang and S. T. Chang, Bioresour. Technol., 2008, 99(9), 3908–3913 CrossRef CAS.
- S. Hammami, H. Jmii, R. E. Mokni, A. Khmiri, K. Faidi, H. Dhaouadi, M. Hédi El Aouni, M. Aouni and R. K. Joshi, Molecules, 2015, 20(11), 20426–20433 CrossRef CAS.
- Z. E. Suntres, J. Coccimiglio and M. Alipour, Crit. Rev. Food Sci. Nutr., 2015, 55(3), 304–318 CrossRef CAS.
- S. M. Pourmortazavi and S. S. Hajimirsadeghi, J. Chromatogr. A, 2007, 1163(1–2), 2–24 CrossRef CAS.
- M. Jakovljević, J. Vladić, S. Vidović, K. Pastor, S. Jokić, M. Molnar and I. Jerković, Plants, 2020, 9, 153 CrossRef.
- K. Pastor, M. Ilić, D. Vujić, D. Jovanović and M. Ačanski, Anal. Lett., 2019, 53(8), 1177–1189 CrossRef.
- O. Hammer, D. A. Harper and P. D. Ryan, Palaeontol. Electron., 2001, 4(1), 9 Search PubMed.
Footnote |
† Electronic supplementary information (ESI) available. See DOI: 10.1039/d0ra10253a |
|
This journal is © The Royal Society of Chemistry 2021 |
Click here to see how this site uses Cookies. View our privacy policy here.