DOI:
10.1039/D0RA10605D
(Paper)
RSC Adv., 2021,
11, 6407-6414
Meso-functionalization of calix[4]arene with 1,3,7-triazapyrene in the design of novel fluorophores with the dual target detection of Al3+ and Fe3+ cations†
Received
17th December 2020
, Accepted 23rd January 2021
First published on 4th February 2021
Abstract
A meso-functionalization strategy has successfully been applied to the synthesis of novel 1,3,7-triazapyrene derivatives of calixarenes. The key synthetic step in these transformations providing the direct C–C bond formation is nucleophilic substitution of hydrogen (SNH) in 1,3,7-triazapyrene. General photophysical characteristics for these macrocyclic compounds, as well as features in emission properties upon addition of various metal cations have been elaborated. Studies using NMR spectroscopy have also shown a mutual effect of both calix[4]arene and 1,3,7-triazapyrene moieties on the coordination process. The complex stoichiometry and binding constants for Al3+ and Fe3+ guests have been explored with titration experiments.
Introduction
Over the last decade, the design of organic molecules for selective detection of biologically and environmentally important analytes, such as small molecules and metal ions attracts a considerable attention from the broad scientific community.1 Among a variety of metal ions, Al3+ and Fe3+ are known to be of great importance due to a wide range of practical applications in machinery, aviation, electronic devices, and daily life.2 Aluminum-containing substances are well-spread in food additives and water treatment systems.3 It is worth noting that accumulation of aluminum in living system turns out to cause anemia and pathology of the nervous system, e.g., Alzheimer disease.4 Besides, iron is an important microelement that plays a key role in the metabolism, synthesis of DNA and RNA,5 also it provides the ability of haem to transfer oxygen.6 On the one hand, the lack of Fe3+ ions results in hemochromatosis, liver injury, Parkinson's disease, and cancer. On the other hand, its abundance is accompanied by certain types of cancer, dysfunction of the heart or pancreas.7 In this regard, the elaboration of efficient chemosensors affording prompt monitoring and the quantitative assessment of ions in biological and environmental systems seems to be a challenging task for modern organic chemistry and materials science.
Calixarenes and their functional derivatives are known to be of practical interest as efficient macrocyclic ionophore receptors. Due to their unique three-dimensional characteristics and also diverse opportunities for the target functionalization, these molecules are widely used as catalysts, liquid crystals, and fluorescent materials.8 Also, macrocyclic ring of calixarene is considered to be a promising scaffold in the design of active pharmaceutical ingredients, drug delivery systems, and modern chemosensors.9 Moreover, calixarene-based compounds are known to be used as highly efficient sensory systems for selective detection of various metal ions (Al3+, Fe3+, Sn2+, Hg2+, Cd2+, etc.).10
Notably, there have been various approaches for the calixarene scaffold functionalization both on upper and low rims, whereas modification of the methylene bridges has remained relatively unexplored for a long time.11 Incorporation of functional blocks into this position enables one to alter solubility and flexibility, control the conformational preferences of the macrocyclic ring, and also provides the point for connection to surfaces or other molecules. Bridge-functionalized calixarenes can be obtained through either cyclocondensation of substituted phenols or via direct modification of the methylene groups.12 One of these approaches is lithiation of the methylene carbon followed by direct coupling with electrophiles13 to give the corresponding meso-substituted calixarenes. In addition, synthetic opportunities for the C–Li/C–H coupling reactions of lithiated calix[4]arene with π-deficient azaaromatics are able to be considered as fruitful and challenging ones. These green chemistry-oriented transformations based on nucleophilic substitution of hydrogen (SNH) in azines have recently been shown to be efficient methods for the synthesis of mono-, di- and triazinyl-substituted tetramethoxycalixarenes.14
This paper deals with a convenient synthetic methodology to afford novel calix[4]arenes modified at the bridge position with azaaromatic scaffold, namely 1,3,7-triazapyrene. An increased interest in this type of macroheterocyclic structures is due to wide opportunities for their practical applications in molecular electronics, e.g., organic photovoltaics (OPVs), organic light-emitting diodes (OLEDs), and organic field-effect transistors (OFETs) as well.15 Besides, some intriguing results on photophysical properties of novel 1,3,7-triazapyrene-modified calixarenes are reported herein, as well as their possibilities for practical use as effective macrocyclic sensory molecules for metal ions, in particular for the dual detection of Al3+ and Fe3+ cations.
Results and discussion
In order to synthesize the desired 1,3,7-triazapyren-6-yl derivatives of calix[4]arenes, a meso-functionalization strategy including a series of subsequent transformations, such as protection of the hydroxy group, bridge-lithiation, followed by nucleophilic substitution of hydrogen (SNH) in 1,3,7-triazapyrene, oxidation of the σH-adducts, and final deprotection has successfully been applied in this study (Scheme 1). It should be emphasized that the exploited reactions of nucleophilic substitution of hydrogen (SNH) are now considered as environmentally benign PASE (Pot, Atom, Step Economy) methodology,16 enabling the direct C–H functionalization of aromatics,17 as well as non-aromatic azaheterocyclic substrates.18 At the same time, a limited number of methods for the synthesis of substituted 1,3,7-triazapyrene derivatives are now available.19 Taking into account this issue, the SNH methodology has herein been chosen as a basic synthetic approach towards to meso-functionalized calix[4]arenes.
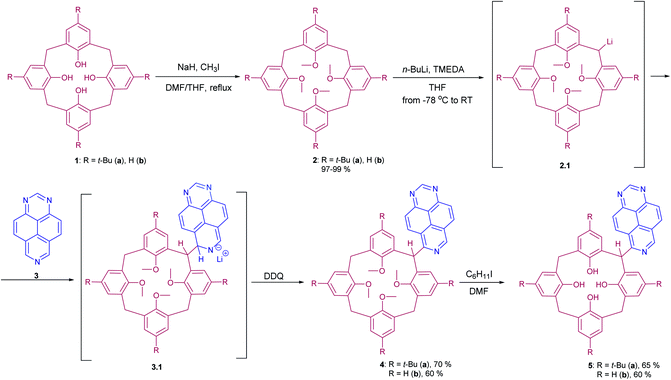 |
| Scheme 1 Meso-heteroarylation strategy in the synthesis of bridge-modified calix[4]arenes. | |
Initially, to protect the hydroxy group in calix[4]arene, we exploited the known approach.20 The further direct C–Li/C–H coupling reaction of meso-Li-calix[4]arene with 1,3,7-triazapyrene was carried out according to the modified literature procedure.14 The methylene group of calix[4]arene 2 was first lithiated with n-BuLi and TMEDA, the lithium compound 2.1 reacted subsequently with N
C–H fragment of 1,3,7-triazapyrene 3, thus leading to unstable σ-adduct 3.1. These intermediates could be transformed into the corresponding products 4 in 60–70% yields by action of DDQ as oxidizing agent. Notably, the typical procedure21 for demethylation using BBr3 in DCM was experimentally found to result in either the partial deprotection (formed in the complex mixtures of various isomers) or decomposition of starting materials as a result of breaking C–C bond between bridge calixarene and azaheterocyclic moieties. The latter was the reason to apply another demethylation procedure using by iodocyclohexane in DMF.22 In this case, tetrahydroxycalix[4]arenes 5 modified with the 1,3,7-triazapyrene scaffold at the meso-position have finally been synthesized in 60–65% yields.
Structures of the bridge-heteroaryl-substituted tetramethoxy and tetrahydroxy derivatives of calix[4]arenes were confirmed by 1H, 13C NMR, and IR spectroscopy including two-dimension NMR experiments, such as 1H–13C HSQC, 1H–13C HMBC, 1H–1H COSY, as well as by the data of mass spectrometry and elemental analysis.
The synthesized methoxy- (4a, b) or hydroxycalixarenes (5a, b), being species that are devoid of sterically bulky substituents at the oxygen atom, are known to be characterized by a dynamic equilibrium between conformers in solutions. In order to fix a more preferable conformation for these species, the complexation strategy with Na+ was used. According to the 1H NMR spectra recorded for 4a after addition of NaI, specific changes were found with regard to the signals for aryl and methylene bridge protons. In particular, several peaks are observed in the regions, where the methylene (3.0–4.5 ppm) and aryl (6.4–7.8 ppm) proton signals are registered in the absence of sodium ions. After addition of Na+ ions, the methylene protons resonated as two signals (4H) at 3.4 ppm, one signal (2H) overlapped with ones of methyl groups at 4.27 ppm, and one signal (1H) at 7.72 ppm. It is worth noting that these features observed correlate well with the literature data for the structurally similar meso-functionalized calix[4]arenes reported.23 Besides, there is one doublet (6H) and one singlet (2H) found in the aryl region of the NMR spectra. Thus, we suppose that partial cone (paco) is the most favourable conformation for the synthesized calixarenes in the presence of Na+ ions. This suggestion is also supported by the data for the bridge-substituted calixarenes with bulky fragments at the meso-position.24
The photophysical properties, such as absorbance and emission spectra of azapyrene-modified calix[4]arenes 4 and 5 have comprehensively been studied to evaluate opportunities for their practical applications in the design of prospective fluorescent sensors (Table 1). As far as the absorbance spectra are concerned, the obtained calix[4]arenes 4 and 5 have proven to possess a similar pattern, exhibiting two broadened bands with maxima at 275 and 350 nm in a THF solution (Fig. 1).
Table 1 Photophysical properties of meso-heteroarylated calix[4]arenes 4 and 5
Entry |
Compounds |
Absorbance λabsa (nm) |
Emission λemb (nm) |
Absorbance maxima in THF. Emission maxima in THF. |
1 |
4a |
270, 340, 350 |
392, 575 (br) |
2 |
4b |
270, 340, 350 |
391, 467, 498, 550 (br) |
3 |
5a |
277, 281, 284, 340 (br) |
378, 452, 475 |
4 |
5b |
275, 345 (br) |
390, 451, 476 |
 |
| Fig. 1 Normalized absorbance spectra of calixarenes 4 and 5. Sample preparation: C = 1.0 × 10−5 mol L−1 in THF at room temperature. | |
In the emission spectra of tetramethoxy-substituted calix[4]arenes 4, the intramolecular charge transfer (ICT) effects are observed as bands with maxima at 575 nm and 550 nm for 4a and 4b, respectively (Fig. 2).25 Notably, the calix[4]arene scaffold is likely to act here as an electron-donating group, whereas 1,3,7-triazapyrene plays a role of electron-withdrawing functional block. Moreover, compound 4a is characterized by the enhanced ICT effect due to a strong inductive electronic effect (+I) of tert-butyl substituent in the para-positions of the benzene rings. In case of tetrahydroxy substituted calixarenes 5, a complicated structure of peaks is presumably attributed to 1,3,7-triazapyrene contribution in the range from 450 to 550 nm, observed in the emission spectra.
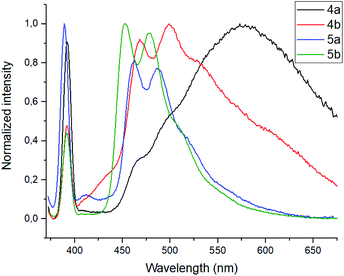 |
| Fig. 2 Normalized emission spectra for calixarenes 4 and 5. Conditions: 4a: λex = 352 nm, 4b: λex = 351 nm, 5a: λex = 341 nm, 5b: λex = 350 nm. Sample preparation: C = 1.0 × 10−5 mol L−1 in THF at room temperature. | |
To evaluate ionophore ability and selectivity of the obtained fluorophores, the emission spectra taken upon the addition of various metal cations, such as Al3+, Ba2+, Be2+, Ca2+, Co2+, Cu2+, Fe3+, K+, Mg2+, Ni2+, Sr2+, Zn2+, Na+, Bi3+, Cd2+, Hg2+, Pb2+, Sn2+ have also been investigated. It is worth mentioning that tetramethoxy-substituted calix[4]arenes 4a, b showed no selectivity to metal cations (see ESI S39 and S40†). At the same time, additional peaks in the emission spectra at 560 nm and 570 nm, respectively, have been observed in case of tetrahydroxycalix[4]arene 5a and 5b in the presence of Al3+ and Fe3+ cations (Fig. 3). In this regard, the chelation of iron(III) ions might induce both appearance of additional peaks, but also decrease in the emission intensity. The latter phenomenon could be accounted for the oxidation of phenolic fragments of calix[4]arenes into the corresponding quinones by action of Fe3+ ions.26
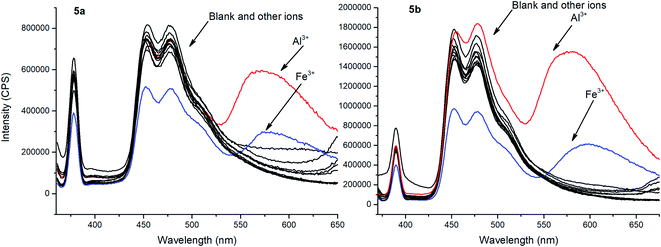 |
| Fig. 3 Emission spectra of 5a and 5b with the presence of various metal ions (10 equiv.). Sample preparation: C = 1.0 × 10−5 mol L−1 in THF at room temperature. | |
To evaluate the changes in emission intensities for the obtained compounds in the presence of various metals cations quantitatively, the fluorescence enhancement factors (FEF = I/Io) have been calculated according to the typical procedure (Fig. 4).27 In case of compound 5a, the emission intensity (λem = 560 nm) appears to be four times higher in the presence of Al3+ and 1.5 times higher with Fe3+ than in the blank experiment performed. Moreover, the emission intensity (λem = 570 nm) for 5b with Al3+ has been found to be 9.5 times higher and 3 times higher in the presence of Fe3+. Thus, one can suggest that the tert-butyl radical in the para-position of the phenol moiety affects the sensory properties of the triazapyrene-modified calixarenes.
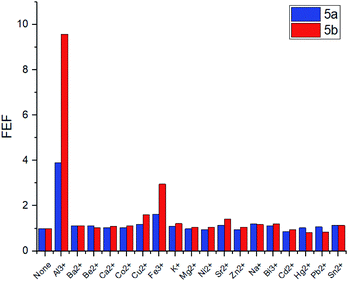 |
| Fig. 4 Fluorescence enhancement factors (FEF = I/Io) of 5a and 5b upon addition of various metal cations. Io = fluorescence emission intensity of free 5a or 5b, I = fluorescence emission intensity of 5a or 5b with metal ion respectively. Conditions: 5a: C5a = 10−5 mol L−1 in THF, 10 equiv. of metal ion in H2O, λex = 341 nm, λem = 560 nm; 5b: C5b = 10−5 mol L−1 in THF, 10 equiv. of metal ion in H2O, λex = 350 nm, λem = 570 nm. | |
The selectivity of the meso-triazapyrenyl-substituted calix[4]arenes for Al3+ ion has also been confirmed by the competition experiment with a number of other ions (Fig. 5). Herein, we have found that emission intensity at the corresponding wavelength in most cases is retained in the presence of other ions in the system. However, emission intensity decreases upon addition of Cu2+ ions, probably because of the formation a non-fluorescent copper(II) complex or dynamic quenching.28
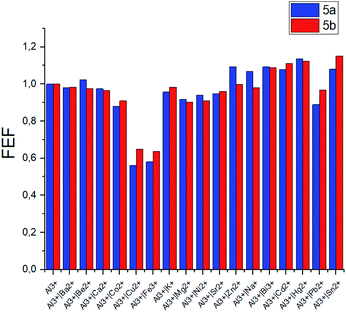 |
| Fig. 5 Fluorescence enhancement factors (FEF = I/Io) of 5a and 5b upon addition of various metal cations in competition with Al3+. Io = fluorescence emission intensity of 5a or 5b with Al3+, I = fluorescence emission intensity of 5a or 5b with Al3+ and metal ion respectively. Experimental conditions: 5a: C5a = 10−5 mol L−1 in THF, 10 equiv. of Al3+ and metal ion in H2O, λex = 341 nm, λem = 560 nm; 5b: C5b = 10−5 mol L−1 in THF, 10 equiv. of Al3+ and metal ion in H2O, λex = 350 nm, λem = 570 nm. | |
The same competition experiments have been carried out for complexing with Fe3+ ions (Fig. 6). Emission intensity at the corresponding wavelength for 5a and 5b has proven to be nearly the same in the presence of other competing ions. It should be noted that the emission intensity decreases slightly, when Cu2+ ions are present in the system compared to the previous experiments with Al3+. This observation is able to be associated with the enhanced relative stability of calixarene complexes with Fe3+ in comparison with the results obtained for non-fluorescent Cu2+ complexes.
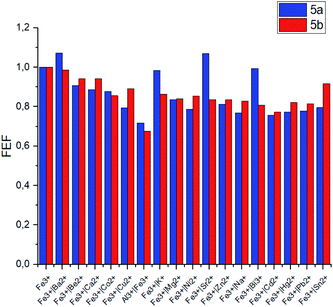 |
| Fig. 6 Fluorescence enhancement factors (FEF = I/Io) of 5a and 5b upon addition of various metal cations in competition with Fe3+. Io = fluorescence emission intensity of 5a or 5b with Fe3+, I = fluorescence emission intensity of 5a or 5b with Fe3+ and metal ion respectively. Experimental conditions: 5a: C5a = 10−5 mol L−1 in THF, 10 equiv. of Fe3+ and metal ion in H2O, λex = 341 nm, λem = 560 nm; 5b: C5b = 10−5 mol L−1 in THF, 10 equiv. of Fe3+ and metal ion in H2O, λex = 350 nm, λem = 570 nm. | |
To gain insight into the mechanism for interaction of the bridge-1,3,7-triazapyrenyl calix[4]arene ligands with metal cations Al3+ and Fe3+, a series of NMR experiments have been carried out. We here report that the addition of 10 equivalents of AlCl3 in D2O to a solution of 5b in DMSO-d6 leads to some changes in both chemical shifts and also multiplicities for the signals of phenyl substituents linked directly to C(sp3)-functionalized center and 1,3,7-triazapyrene fragments (Fig. 7). Remarkably, it has been found that some 1,3,7 triazapyrene proton signals undergo upfield shifts, while the signals of hydrogen in the para-position Hp1-4 are being split into two multipletes. Based on these changes in the spectra, one can propose that both oxygen atoms of calix[4]arene and nitrogen atoms of 1,3,7-triazapyrene fragment are involved in the organometallic complex formation.
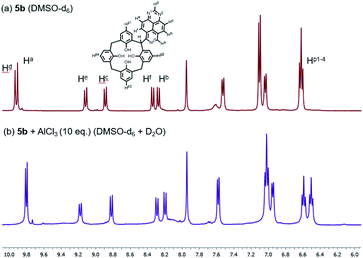 |
| Fig. 7 1H NMR spectra of 5b in DMSO-d6 at 298 K (a) free (b) upon addition of AlCl3 (10 equiv.) in D2O. | |
In this study, the Job's method29 has been applied to determine the stoichiometry of 5b
:
Al3+ and 5b
:
Fe3+ and shown 1
:
1 host–guest complex formation. Binding interactions of 5b with Al3+ and Fe3+ cations in THF with addition of various concertation of guests in H2O have been estimated using the modified Benesi–Hildebrand equation30 (see ESI S27–S30†). We found that K (Al3+) = 2.27 × 103 M−1; K (Fe3+) = 1.63 × 105 M−1 corresponding to the literature data for similar fluorescent chemosensors.8c
Conclusions
In summary, the meso-functionalization strategy, based on the sequence of reactions including protection of the hydroxy group, bridge-lithiation of tetramethoxy-substituted calix[4]arenes followed by C–Li/C–H coupling with 1,3,7-triazapyrene, and further deprotection, has successfully been applied to the synthesis of novel heterocyclic derivatives of calix[4]arenes. The key step in these transformations, which provides a macrocyclic ionophore scaffold to be directly incorporated into the photoactive azaaromatics, is nucleophilic substitution of hydrogen (SNH) in 1,3,7-triazapyrene. This synthetic approach has been found to result in the formation of new bifunctional fluorophore systems based on meso-1,3,7-triazapyrene-substituted calix[4]arene ensembles in good yields under mild reaction conditions. The elucidation of photophysical properties enabled us to reveal the intramolecular charge transfer (ICT) in tetramethoxy meso-heteroaryl-substituted calix[4]arenes, which can be considered as challenging molecules for materials science and molecular electronics. The fluorescent sensory properties with respect to metal cations, including applications of meso-heteroaryl-substituted calixarenes for the dual target detection of Al3+ and Fe3+ cations, and also mechanistic features for the complex formation have been studied by NMR spectroscopy. Remarkably, tetrahydroxycalix[4]arenes have experimentally been shown to be more promising compounds in the design of fluorescent chemosensors versus the corresponding tetramethoxy analogues. Thus, the properties of azaheterocyclic calix[4]arene fluorophores illustrated in this research indicate new opportunities for practical applications of these macrocyclic ensembles for selective detection of metal ions (Al3+, Fe3+) in biological, environmental, and technogenic systems.
Experimental
General experimental methods
Nuclear Magnetic Resonance (NMR) spectra were recorded on Bruker Avance II (400 MHz) spectrometers. All 1H experiments were reported in δ units, parts per million (ppm), and were measured relative to residual chloroform DCCl3 (7.26 ppm) or DMSO-d6 (2.50 ppm) signals in the deuterated solvent. All 13C NMR spectra were reported in ppm relative to CDCl3 (77.16 ppm) or DMSO-d6 (39.52 ppm) and all spectra were obtained with 1H decoupling. All coupling constants J were reported in hertz (Hz). The following abbreviations were used to describe peak splitting patterns (s = singlet, d = doublet, t = triplet, dd = doublet of doublet, m = multiplet, and br s = broad singlet). The mass spectra were recorded on a mass spectrometer SHIMADZU GCMS-QP2010 Ultra with sample ionization by electron impact (EI) equipped with a quadrupole mass analyzer. The IR spectra were recorded using a Fourier-transform infrared spectrometer equipped with a diffuse reflection attachment. The elemental analysis was carried out on a CHNS/O analyzer. The course of the reactions was monitored by TCL on 0.25 mm silica gel plates (60F 254).
4-tert-Butylcalix[4]arene (1a), calix[4]arene (1b), tetramethylethylenediamine (TMEDA), n-buthyllithium (2.5 M solution in hexane), 2,3-dichloro-5,6-dicyano-1,4-benzoquinone (DDQ), iodocyclohexane were purchased and used as received. 5,11,17,23-Tetra-tertbutyl-25,26,27,28-tetramethoxycalix[4]arene (2a), 25,26,27,28-tetramethoxycalix[4]arene (2b),20 1,3,7-triazapyrene (3)31 were prepared according to the literature procedures.
General procedure for synthesis of azaheterocycles derivatives of 25,26,27,28-tetramethoxycalix[4]arene 4a, b
A Schlenk flask (100 mL) equipped with a magnetic stirrer was flame-dried under vacuum, and cooled to room temperature under an argon flow. Then TMEDA (2.25 mL, 0.00454 mol, 5.8 equiv.), THF (5 mL, dry) and n-BuLi (2.4 mL 2.5 M solution in hexane, 7.66 equiv.) was added, and stirred 15 minutes in acetone-liquid nitrogen bath at −20 °C. Subsequently, 2a (0.551 g, 0.000783 mol, 1 equiv.) or 2b (0.376 g, 0.000783 mol, 1 equiv.) was dissolved in THF (15 mL, dry), and the solution was cooled to −78 °C. The mixture was allowed to warm up to ambient temperature, and stirred for additional 1 h. Then 1,3,7-triazapyrene 3 (0.162 g, 0.000783 mol, 1 equiv.) was dissolved in THF (35 mL, dry) and added to the mixture, and the solution was cooled to −78 °C. The mixture again was allowed to warm up to ambient temperature and stirred for additional 1 h. After that, DDQ (0.277 g, 0.001566 mol, 2 equiv.) in THF (5 mL, dry) was added, and the reaction mixture was refluxed in oil bath for 4 h. The solvents were removed under reduced pressure, CHCl3 (30 mL) was added to the residual, and filtered through a short column with Al2O3 (neutral) with CHCl3 as eluent (100 mL). Then a solvent was removed in vacuo, and the desired product was purified by column chromatography on SiO2 with a mixture of hexane/EtOAc as an eluent.
2-(1,3,7-Triazapyrene-6-yl)-5,11,17,23-tetra-tert-butyl-25,26,27,28-tetramethoxycalix[4]arene (4a). White solid. Yield: 497 mg (70%), mp = 175–177 °C. Rf 0.25 (hexane/EtOAc, 8
:
2). Note: NaI (1.5 equiv.) in CD3CN was added to lock the conformation of calix[4]arene 1H NMR (DCCl3 + NaI in CD3CN, 400 MHz): δ 9.79 (s, 1H); 9.76 (s, 1H); 9.39 (d, 1H, J = 9.7 Hz); 8.60–8.58 (m, 1H); 8.435 (d, 1H, J = 6.0 Hz); 8.185 (d, 1H, J = 6.0 Hz); 7.72 (s, 1H); 7.17–7.13 (m, 8H); 4.28–4.26 (m, 2H); 4.25 (s, 6H); 4.16 (s, 6H); 3.42–3.39 (m, 4H); 1.15–1.13 (m, 36H) ppm. 13C{1H} NMR (DCCl3 + NaI in CD3CN, 100 MHz): δ 157.8; 157.5; 154.8; 154.0; 150.8; 150.5; 148.9; 148.8; 147.1; 136.1; 134.6; 134.5; 134.2; 133.9; 131.6; 129.2; 128.2; 126.6; 126.5; 126.2; 126.1; 122.7; 121.9; 115.5; 65.9; 64.8; 34.5; 34.2; 31.1; 31.05; 30.3; 29.9 ppm. IR (DRA): ν 2954, 2866, 1628, 1555, 1478, 1388, 1359, 1244, 1201, 1117, 1019, 919, 870, 798, 727, 645, 578, 557 cm−1. MS (EI): m/z 907 [M]+. Anal. calcd for C61H69N3O4: C, 80.67; H, 7.66; N, 4.63; O, 7.05. Found: C, 80.81; H, 7.88; N, 4.56.
2-(1,3,7-Triazapyrene-6-yl)-25,26,27,28-tetramethoxycalix[4]arene (4b). White solid. Yield: 320 mg (60%), mp = 165–167 °C. Rf 0.15 (hexane/EtOAc, 8
:
2). Note: the addition of NaI in CD3CN to this compound does not lead to the changes in the 1H NMR spectrum (DCCl3, 400 MHz): δ 9.87 (s, 1H); 9.67 (s, 1H); 9.10–8.80 (m, 1H); 8.62–8.58 (m, 1H); 8.32–8.20 (m, 2H); 7.49–7.32 (m, 1H); 7.09–6.94 (m, 2H); 6.85–6.54 (m, 9H); 6.46–6.13 (m, 1H); 4.48–4.36 (m, 2H); 3.96–3.65 (m, 12H); 3.54–3.45 (m, 1H); 3.29–3.19 (m, 3H) ppm. 13C{1H} NMR (DCCl3, 100 MHz): δ 159.7; 159.4; 158.3; 158.1; 158.0; 157.4; 155.2; 154.3; 147.6; 136.9; 136.7; 135.4; 135.1; 134.8; 134.0; 133.3; 132.7; 132.3; 131.6; 130.5; 130.4; 129.8; 129.4; 128.8; 128.4; 128.3; 128.0; 126.8; 122.6; 122.3; 115.8; 100.0; 61.9; 61.7; 61.1; 60.5; 41.7; 59.3; 35.8; 35.4; 30.9; 30.7; 21.2; 14.3 ppm. IR (DRA): ν 2935, 2845, 1760, 1683, 1597, 1497, 1424, 1349, 1204, 1085, 969, 851, 722, 612, 548 cm−1. MS (EI): m/z 683 [M]+. Anal. calcd for C45H37N3O4: C, 79.04; H, 5.45; N, 6.15; O, 9.36. Found: C, 79.15; H, 5.71; N, 5.92.
General procedure for synthesis of azaheterocycles derivatives of 25,26,27,28-tetrahydroxycalix[4]arene 5a, b
In a round-bottom flask (50 mL) equipped with a magnetic stirrer, 4a (0.362 g, 0.0004 mol, 1 equiv.) or 4b (0.273 g, 0.0004 mol, 1 equiv.) was placed in DMF (25 mL, dry). Subsequently, iodocyclohexane (2.55 mL, 0.0178 mol, 44.5 equiv.) was added, and the reaction mixture was refluxed in oil bath for 6 hours. Then H2O (200 mL, distilled) was added, and the reaction mixture was extracted CHCl3 (4 × 70 mL). The organic phase was washed with saturated Na2S2O3 (2 × 100 mL), dried over Na2SO4 and concentrated in vacuo. The desired product was purified by column chromatography with the appropriated eluent (for 5a: benzene/methanol 100/1 mixture; for 5b: CHCl3).
2-(1,3,7-Triazapyrene-6-yl)-5,11,17,23-tetra-tert-butyl-25,26,27,28-tetrahydroxycalix[4]arene (5a). Yellow solid. Yield: 221 mg (65%), mp = 172–174 °C. Rf 0.4 (benzene/MeOH, 100
:
1). 1H NMR (DCCl3, 400 MHz): δ 11.78 (br s, 4H); 9.90 (s, 1H); 9.65 (s, 1H); 8.98 (d, 1H, J = 4 Hz); 8.655 (d, 1H, J = 12 Hz); 8.29 (d, 1H, J = 4 Hz); 8.255 (d, 1H, J = 12 Hz); 7.45–7.35 (m, 2H); 7.13–7.06 (m, 6H); 6.53–6.40 (m, 1H); 4.37–4.15 (m, 3H); 3.56–3.35 (m, 3H); 1.25 (s, 36H) ppm. 13C{1H} NMR (DCCl3, 100 MHz): δ 158.4; 155.5; 154.3; 149.3; 147.8; 143.8; 134.5; 132.5; 130.0; 129.7; 129.1; 128.8; 128.3; 127.8; 127.4; 126.1; 125.9; 122.2; 115.6; 34.2; 34.1; 33.3; 33.2; 31.6 ppm. IR (DRA): ν 3888, 3749, 3643, 3542, 3426, 3281, 2951, 1735, 1629, 1594, 1480, 1390, 1359, 1295, 1198, 987, 872, 815, 704, 589, 527 cm−1. MS (EI): m/z 852 [M]+. Anal. calcd for C57H61N3O4: C, 80.34; H, 7.22; N, 4.93; O, 7.51. Found: C, 80.32; H, 7.58; N, 4.75.
2-(1,3,7-Triazapyrene-6-yl)-25,26,27,28-tetrahydroxycalix[4]arene (5b). Yellow solid. Yield: 150 mg (60%), mp = 168–170 °C. Rf 0.45 (CHCl3, 100%). 1H NMR (DCCl3, 400 MHz): δ 11.58 (br s, 4H); 9.88 (s, 1H); 9.62 (s, 1H); 8.88 (d, J = 9.5 Hz, 1H); 8.61 (d, J = 9.2 Hz, 1H); 8.27–8.21 (m, 2H); 7.56–7.34 (m, 2H); 7.11–7.02 (m, 6H); 6.82–6.70 (m, 4H); 6.64–6.42 (m, 1H); 4.32–4.00 (m, 3H); 3.73–3.38 (m, 3H); ppm. 13C{1H} NMR (DCCl3, 100 MHz): δ 158.4; 155.4; 154.2; 151.4; 149.6; 143.6; 134.3; 132.2; 130.3; 130.1; 129.2; 129.0; 128.8; 128.7; 128.5; 127.4; 123.4; 122.1; 121.9; 121.7; 115.4; 32.3; 32.0 ppm. IR (DRA): ν 3841, 3747, 3655, 3566, 3436, 3192, 3039, 2945, 1667, 1628, 1591, 1501, 1448, 1385, 1251, 1083, 891, 788, 750, 560, 515 cm−1. MS (EI): m/z 627 [M]+. Anal. calcd for C41H29N3O4: C, 78.45; H, 4.66; N, 6.69; O, 10.20. Found: C, 78.37; H, 4.74; N, 6.43.
Conflicts of interest
There are no conflicts to declare.
Acknowledgements
The research was financially supported by the Council on Grants of the President of the Russian Federation for State Support of Young Russian Scientists – Candidates of Sciences (Project No. MK-1196.2020.3, Agreement No. 075-15-2020-506).
References
-
(a) K. Singh, A. M. Rotaru and A. A. Beharry, ACS Chem. Biol., 2018, 13(7), 1785–1798 CrossRef CAS;
(b) D. Wu, A. C. Sedgwick, T. Gunnlaugsson, E. U. Akkaya, J. Yoon and T. D. James, Chem. Soc. Rev., 2017, 46(23), 7105–7123 RSC;
(c) P. A. Lieberzeit and F. L. Dickert, Anal. Bioanal. Chem., 2009, 393(2), 467–472 CrossRef CAS.
-
(a) H. Heather, Iron, The Rosen Publishing Group, 2006, pp. 1–48 Search PubMed;
(b) H. Heather, Aluminum, The Rosen Publishing Group, 2006, pp. 1–48 Search PubMed.
-
(a) S. M. Saiyed and R. A. Yokel, Food Addit. Contam., 2005, 22(3), 234–244 CrossRef CAS;
(b) H. Zhang, J. Y. Zhang, H. L. Wang, P. J. Luo and J. B. Zhang, Biomed. Environ. Sci., 2016, 29(6), 461–466 Search PubMed.
-
(a) G. Crisponi, D. Fanni, C. Gerosa, S. Nemolato, V. M. Nurchi, M. Crespo-Alonso, J. I. Lachowicz and G. Faa, Biomol. Concepts, 2013, 4(1), 77–87 CAS;
(b) J. Nie, in Neurotoxicity of Aluminum, ed. Q. Niu, Springer, Singapore, 2018, vol. 1091 CrossRef.
- M. Sánchez, L. Sabio, N. Gálvez, M. Capdevila and J. M. Dominguez-Vera, IUBMB Life, 2017, 69(6), 382–388 CrossRef.
- H. D. A. Mohamed, S. M. D. Watson, B. R. Horrocks and A. Houlton, Nanoscale, 2012, 4(19), 5936–5945 RSC.
-
(a) N. S. Andrews, Annu. Rev. Genomics Hum. Genet., 2000, 1(1), 75–98 CrossRef CAS;
(b) F. Oliveira, S. Rocha and R. Fernandes, J. Clin. Lab. Anal., 2014, 28(3), 210–218 CrossRef CAS;
(c) A. Siddique and K. V. Kowdley, Aliment. Pharmacol. Ther., 2012, 35(8), 876–893 CrossRef CAS.
-
(a) D. M. Homden and C. Redshaw, Chem. Rev., 2008, 108(12), 5086–5130 CrossRef CAS;
(b) F. Yang, H. Guo and J. Vicens, J. Inclusion Phenom. Macrocyclic Chem., 2014, 80(3–4), 177–186 CrossRef CAS;
(c) R. Kumar, A. Sharma, H. Singh, P. Suating, H. S. Kim, K. Sunwoo, I. Shim, B. C. Gibb and J. S. Kim, Chem. Rev., 2019, 119(16), 9657–9721 CrossRef CAS.
-
(a) F. N. Pur, Mol. Diversity, 2020 Search PubMed;
(b) Y. Zhou, H. Li and Y.-W. Yang, Chin. Chem. Lett., 2015, 26(7), 825–828 CrossRef CAS;
(c) B. Mokhtari and K. Pourabdollah, Asian J. Chem., 2013, 25(1), 13–18 CrossRef CAS.
-
(a) I. Leray and B. Valeur, Eur. J. Inorg. Chem., 2009, 24, 3525–3535 CrossRef;
(b) Y.-B. Ruan, A. Depauw and I. Leray, Org. Biomol. Chem., 2014, 12, 4335–4341 RSC;
(c) H. M. Chawla and T. Gupta, Tetrahedron Lett., 2015, 56, 793–796 CrossRef CAS.
-
(a) R. Lavendomme, S. Zahim, G. De Leener, A. Inthasot, A. Mattiuzzi, M. Luhmer, O. Reinaud and I. Jabin, Asian J. Org. Chem., 2015, 4(8), 710–722 CrossRef CAS;
(b) W. Sliva and M. Deska, Arkivoc, 2011, 2011(1), 496–551 Search PubMed;
(c) M. Deska, B. Dondela and W. Sliwa, Arkivoc, 2014, 2015(1), 29–47 Search PubMed;
(d) W. Sliwa and M. Deska, Arkivoc, 2012, 2012(1), 173–210 Search PubMed.
-
(a) I. Columbus, J. Org. Chem., 2008, 73(7), 2598–2606 CrossRef CAS;
(b) M. Bergamaschi, F. Bigi, M. Lanfranchi, R. Maggi, A. Pastorio, M. A. Pellinghelli, F. Peri, C. Porta and G. Sartori, Tetrahedron, 1997, 53(38), 13037–13052 CrossRef CAS.
-
(a) P. A. Scully, T. M. Hamilton and J. L. Bennett, Org. Lett., 2001, 3(17), 2741–2744 CrossRef CAS;
(b) C. Fischer, W. Seichter and E. Weber, Beilstein J. Org. Chem., 2011, 7, 1602–1608 CrossRef CAS.
-
(a) M. V. Varaksin, I. A. Utepova, O. N. Chupakhin and V. N. Charushin, Macroheterocycles, 2013, 6(4), 308–314 CrossRef CAS;
(b) M. V. Varaksin, O. N. Chupakhin, V. N. Charushin, K. A. Khlamkin and I. A. Utepova, Russ. Chem. Bull., 2015, 64(5), 1093–1096 CrossRef CAS.
-
(a) C. Wang, H. Dong, W. Hu, Y. Liu and D. Zhu, Chem. Rev., 2012, 112(4), 2208–2267 CrossRef CAS;
(b) H. Dong, X. Fu, J. Liu, Z. Wang and W. Hu, Adv. Mater., 2013, 25(43), 6158–6183 CrossRef CAS;
(c) A. Facchetti, Chem. Mater., 2011, 23(3), 733–758 CrossRef CAS.
- W. Zhang and W.-B. Yi, Pot, Atom, and Step Economy (PASE) Synthesis, Springer International Publishing, Cham, 2019 Search PubMed.
-
(a) M. Mąkosza, Chem. Soc. Rev., 2010, 39(8), 2855–2868 RSC;
(b) V. N. Charushin and O. N. Chupakhin, Russ. Chem. Bull., 2019, 68(3), 453–471 CrossRef CAS.
-
(a) A. A. Akulov, M. V. Varaksin, P. Mampuys, V. N. Charushin, O. N. Chupakhin and B. U. W. Maes, Org. Biomol. Chem., 2021, 19, 297–312, 10.1039/D0OB01580F;
(b) T. D. Moseev, M. V. Varaksin, D. A. Gorlov, V. N. Charushin and O. N. Chupakhin, J. Org. Chem., 2020, 85(17), 11124–11133 CrossRef CAS;
(c) L. A. Smyshliaeva, M. V. Varaksin, P. A. Slepukhin, O. N. Chupakhin and V. N. Charushin, Beilstein J. Org. Chem., 2018, 14, 2618–2626 CrossRef CAS;
(d) A. A. Akulov, M. V. Varaksin, V. N. Charushin and O. N. Chupakhin, ACS Omega, 2019, 4(1), 825–834 CrossRef CAS.
-
(a) I. Borovlev, O. Demidov, G. Amangasieva, E. Avakyan and N. Kurnosova, J. Heterocycl. Chem., 2017, 54(1), 406–412 CrossRef CAS;
(b) I. V. Borovlev, O. P. Demidov, N. A. Kurnosova, G. A. Amangasieva and E. K. Avakyan, Chem. Heterocycl. Compd., 2015, 51(2), 170–175 CrossRef CAS.
- T. Läppchen, R. P. M. Dings, R. Rossin, J. F. Simon, T. J. Visser, M. Bakker, P. Walhe, T. van Mourik, K. Donato, J. R. van Beijnum, A. W. Griffioen, J. Lub, M. S. Robillard, K. H. Mayo and H. Grüll, Eur. J. Med. Chem., 2015, 89, 279–295 CrossRef.
- J. F. W. McOmie, M. L. Watts and D. E. West, Tetrahedron, 1968, 24(5), 2289–2292 CrossRef CAS.
- L. T. Carroll, P. A. Hill, C. Q. Ngo, K. P. Klatt and J. L. Fantini, Tetrahedron, 2013, 69(24), 5002–5007 CrossRef CAS.
- S. E. Biali, V. Bohmer, S. Cohen, G. Ferguson, C. Gruttner, F. Grynszpan, E. F. Paulus, I. Thondorf and W. Vogt, J. Am. Chem. Soc., 1996, 118, 12938–12949 CrossRef CAS.
- C. Fischer, T. Gruber, W. Seichter and E. Weber, Org. Biomol. Chem., 2011, 9, 4347–4352 RSC.
- The emission spectra have been measured at the excitation wavelength corresponding to the long wavelength maximum in the absorption spectra.
- S. Belattar, N. Debbache, N. Seraghni and T. Sehili, Int. J. Chem. React. Eng., 2016, 14(1), 225–234 CAS.
- J. R. Isaac and H. Xu, OSA Continuum, 2018, 1(3), 899–909 CrossRef CAS.
- M. Hazra, T. Dolai, A. Pandey, S. K. Dey and A. Patra, J. Saudi Chem. Soc., 2017, 21, S240–S247 CrossRef CAS.
- J. S. Renny, L. L. Tomasevich, E. H. Tallmadge and D. B. Collum, Angew. Chem., Int. Ed., 2013, 52(46), 11998–12013 CrossRef CAS.
-
(a) R. L. Scott, Recl. Trav. Chim. Pays-Bas, 1956, 75(7), 787–789 CrossRef CAS;
(b) A. Sahana, A. Banerjee, S. Lohar, S. Panja, S. Kanti Mukhopadhyay, J. Sanmartín Matalobos and D. Das, Chem. Commun., 2013, 49(65), 7231–7233 RSC.
- A. V. Aksenov, I. V. Borovlev, I. V. Aksenova, S. V. Pisarenko and D. A. Kovalev, Tetrahedron Lett., 2008, 49(4), 707–709 CrossRef CAS.
Footnote |
† Electronic supplementary information (ESI) available: Copies of 1H, 13C, 1H–13C HSQC and 1H–13C HMBC, 1H–1H COSY spectra for calixarenes 4 and 5. Absorbance, emission spectra; emission spectra with the presence of various metal ions; determining the stoichiometry of ligand: metal complex; binding constants measurement; emission spectra depending on pH (PDF). See DOI: 10.1039/d0ra10605d |
|
This journal is © The Royal Society of Chemistry 2021 |
Click here to see how this site uses Cookies. View our privacy policy here.