DOI:
10.1039/D1RA00433F
(Paper)
RSC Adv., 2021,
11, 22530-22543
Effects of different ozone treatments on the storage quality and stability of fresh peeled garlic
Received
16th March 2021
, Accepted 2nd June 2021
First published on 28th June 2021
Abstract
In order to understand their impacts on the preservation of fresh garlic, varying concentrations of ozone gas and different storage temperatures were tested for this experiment. The results demonstrated that freshly peeled garlic was best preserved by an ozone concentration of 5 ppm and storage at 4 °C compared to other treatment groups. With these optimized conditions, after 25 days of storage, the weight of garlic decreased by only 1.89% and, under the same storage conditions, the water loss rate was only 65.17% that of the control group, with a decay rate of only 12.50%. The rate of decay in the blank control group was three times that of this group. The germination rate was also low: only 30.26%, which was 57.69% that of the blank control group. The hardness was measured at 7.48 kg cm−2, 19.79% higher than that of the blank group. The content of soluble solids was 9.15 g 100 mL−1, which was 10.27% higher than that of the blank group, again proving that the above storage parameters were effective. At the same time, the titratable acid (TA) in the garlic was 15.48%, which was 1.17 times that of the blank group and corresponds to the vitamin C content. Also, the content of diallyl trisulfide only decreased by 3.98% and was 11.2% higher (P < 0.01) than that of the blank group. Finally, the validity of this optimal result was also confirmed by sensory evaluation. These results, for garlic, support the application of ozone as a safe, non-thermal preservation technique benefiting both producers and consumers.
1. Introduction
Garlic, a semiannual herb, is related to the onion plant.1,2 The primary nutritional and health components in garlic are diallyl trisulfide, amino acids, vitamins, glycosides, organic germanium, selenium, and superoxide dismutase (SOD).17 In a dose-dependent manner, diallyl trisulfide can inhibit GSH depletion and GST decline in mouse liver tissue, increase the activity of glutathione S-transferase in liver cells, and enhance the binding and detoxification functions of the liver, thus protecting the liver and the whole body.29 As the pace of daily life has increased, freshly peeled garlic has become more popular, with its convenience greatly saving time.3–8 However, no or poor sanitation of peeled garlic after harvest can cause high losses due to deterioration and pathogenic microorganisms.9 Meanwhile, storage and preservation present a series of problems, such as loss of nutrients, surface yellowing, and shrinkage, all of which inhibit the commercial development of fresh peeled garlic.10 At present, there are practically no post-harvest fungicides or bactericides with a broad spectrum of action that are safe with no toxic residual effects. However, to minimize these problems, the use of sanitizers is efficient against microorganisms.9 As such, it is important to study safe, effective, green, and widely available technologies to preserve the freshness of peeled garlic.9,10
Ozone (O3), or triatomic oxygen, is naturally produced from oxygen as a result of lightning or UV light interaction.11 Using ozone as an alternative sanitizing technology can potentially replace regular sanitizers such as chlorine. Ozone at concentrations of 0.15–5.0 ppm was shown to inhibit the growth of spoilage bacteria and yeasts.12,14 For disinfection, ozone has been shown to reduce bacteria (e.g., E. coli O157:H7, L. monocytogenes, and Pseudomonas fluorescens) and viruses (e.g., murine norovirus, poliovirus, and human rotavirus) in suspension.17–19 Data have also shown that ozone can be an effective sanitizer for microorganisms present on fresh produce, but the results vary widely due to differences in experimental design and variation in control of the aforementioned technological factors.20,21 Overall, pathogens that are often associated with produce include E. coli O157:H7, Salmonella enterica, L. monocytogenes, and human noroviruses.23 Research has shown that the ozonation process causes clear and positive changes in the metabolism of glutathione in berries. After ozonation, the fruit was characterized by an increased level of glutathione (GSH) which resulted from the higher activity of glutathione synthetase.51 Ozone treatment of fruits and vegetables can significantly reduce the loss of nutrients during storage and better maintain nutrient contents in fruits and vegetables.22 After fruits and vegetables are treated with an appropriate concentration of ozone, the stomata of the epidermis can be completely closed, resulting in reduced water consumption. Consequently, transpiration can be effectively inhibited and the physiological state of the fruits and vegetables can be changed. Further, ozone treatment can also help maintain the firmness of fruits and vegetables.14
The major advantages of ozone make it a preferred technology within the food industry. Ozone is one of the most potent sanitizers for sterilizing the lactic acid bacteria responsible for much of the spoilage in food factories. Since excess ozone rapidly auto-decomposes to produce oxygen, it leaves no residue in food.15 In terms of the mechanism of how ozone impacts oxidation and the destruction of cell walls and cytoplasmic membranes, differences in ozone sensitivity likely correspond to differences in the structures of cell walls. Perhaps ozone first directly oxidizes and destroys the cell walls and cytoplasmic membranes of bacteria, then moves into the cell and acts on its DNA. If so, the bacteria will not develop a resistance to ozone.16 Ozone treatments have effectively extended the shelf lives of oranges, raspberries, grapes, pears, and apples.56 Ozone may induce oxidative stress in fresh fruit, which promotes various physiological responses, including the synthesis of antioxidants, polyamines, ethylene, phenolic compounds, and other secondary metabolites.13
In this study, fresh garlic was treated with different concentrations of ozone and stored at 4 °C or 20 °C. The weight loss rate, germination rate, decay rate, hardness, soluble solid content (SSC), titratable acid (TA), vitamin C, PPO, total number of colonies, total numbers of mold and yeast, diallyl trisulfide, and other indexes were measured regularly to identify the best ozone concentration and storage temperature for fresh garlic.
2. Materials and methods
2.1. Experimental preparation
After peeling, washing, and drying fresh garlic, pieces of uniform size and healthy appearance were selected. The garlic pieces were divided into 14 groups, each containing 50 pieces with a total weight of about 300 g. Each group was put into a 5 L closed container, then injected with ozone gas from the ozone measurement and control cabinet (YJF-002). Concentrations of 0 ppm, 1 ppm, 2 ppm, 3 ppm, 4 ppm, 5 ppm and 6 ppm were tested. After undergoing closed treatment for 15 min, the samples were removed. The 0 ppm ozone gas concentration served as the control group (CK). The samples were put into transparent plastic boxes for fruits and vegetables, labeled, and stored at 4 °C or 20 °C. The weight loss rate, germination rate, decay rate, hardness, SSC, TA, vitamin C, PPO activity, total number of colonies, diallyl trisulfide content, and other indexes of garlic in each treatment group were regularly measured. The garlic stored at 20 °C was measured every two days for a total of 10 days and that stored at 4 °C was measured every five days for a total of 25 days.
2.2. Determination of weight loss rate, germination rate, decay rate and hardness
The garlic in each replicate was marked and weighed (using a digital balance, EK-600H, Japan) before storage for weight loss determination. The garlic was then weighed at two-day intervals over the storage period. The same three specimens in each replicate were weighed repeatedly throughout the storage period. The results are expressed as percentage weight loss relative to the initial weight.
Eqn (1) was used to calculate the weight loss rate.24
|
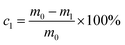 | (1) |
Here,
c1 is the weight loss rate of the garlic,
m0 is the weight of the garlic before storage, and
m1 is the weight of the garlic after storage.
The formula for calculating the germination rate (eqn (2)) is
|
 | (2) |
where
c2 is the germination rate of the garlic,
n0 is the quantity of garlic before storage, and
n1 is the quantity of sprouted garlic.
Eqn (3) was used to calculate the decay rate.25
|
 | (3) |
Here,
c3 is the decay rate of the garlic,
n0 is the quantity of garlic before storage, and
n1 is the quantity of rotten garlic after storage.
The garlic firmness was assessed using a fruit durometer (GY-3) manufactured by Shanghai HuYueming Scientific Instrument Co., Ltd. Three fruits in each replicate were penetrated using the 50 mm diameter probe at a speed of 50 mm min−1 at three points in the equatorial region of the piece of garlic.18 The compression force measured at the maximum peak of the recorded force on the chart was expressed in newtons (N). Parallel measurements were taken four times and the average value was taken.
2.3. Soluble solids concentration (SSC), TA and vitamin C treatment
During storage, the SSC, TA and VC of the fruit pulp were analyzed. Individual 10 g fruit pulp samples from each replicate were homogenized using a kitchen blender (Model HR2094, Philip, Malaysia) with 40 mL of distilled water, then filtered through muslin cloth. The pulp was sampled from the equatorial region of the whole piece of fruit and was a pooled sample from each replicate. A drop of the filtrate was used to determine the SSC (°Brix) using a Palette digital refractometer (PR-32a) from Atago Co, Ltd (Japan) which was calibrated with distilled water prior to taking readings.14 The readings were multiplied by the dilution factor to obtain the original SSC (%) of the garlic pulp. The Palette digital refractometer was used for determination;26 three parallel measurements were conducted and the average value was taken. Using the Asgar Ali general analysis method for beverages,27 the temperature was corrected to obtain the final content of soluble solids in garlic.
The TA was analyzed using the titration method described by Ranganna.28 Individual 10 g samples of pulp tissue were homogenized with 40 mL of distilled water in a kitchen blender (Model HR2094, Philip, Malaysia) for two min. The mixture was then filtered through muslin cloth. 5 mL of filtrate with one to two drops of 0.1% phenolphthalein as a pH indicator was titrated against 0.1 M sodium hydroxide (NaOH) until a pink end point was reached (approximately 10 s). Titratable acidity was expressed as percentage of citric acid equivalents.
The method for detecting vitamin C incorporated several steps.30,70 The test method was developed and modified by Hiroshi.30 Depending on its redox state, sample oxidation shows as dark blue and the reduction state becomes colorless. In a conical flask containing 10 mL of filtrate, the liquid was titrated with a calibrated 2,6-dioxoindiglycol solution until the solution acquired and maintained a pink color for 15 s without fading. Eqn (4) was used to calculate vitamin C as follows.
|
 | (4) |
Here,
V is the volume of dye solution consumed when titrating the sample solution (mL),
V0 is the volume of dye solution consumed when titrating a blank (mL),
T is the 2,6-dichlorophenol dye concentration (mg mL
−1),
A is the dilution factor, and
W is the sample weight (g).
2.4. Analysis of PPO activity
Enzyme extraction and detection were carried out in accordance with Moscetti et al.31 with a slight modification. Five grams of garlic samples were homogenized with 8.0 mL of 0.1 mol L−1 chilled sodium phosphate buffer (pH 5.5). The mixture was centrifuged at 1409×g for 20 min at 4 °C. The supernatant was collected after centrifugation as a crude enzyme extraction solution. The assay was performed using a spectrophotometer. The absorbance at 420 nm was recorded at 15 s as the initial value and then every 30 s for continuous determination until stable. The determination was repeated three times. The enzyme activity calculation (eqn (5)) is as follows. |
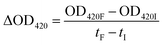 | (5) |
Here, OD420 is the change in reaction absorbance per minute, OD420F is the end absorbance of the reaction mixture, OD420I is the initial absorbance of the reaction mixture, tF is the reaction termination time (min), and tI is the initial reaction time (min).
2.5. Total number of colonies
Twenty-five grams of the sample was weighed and put into a sterile cup containing 225 mL of phosphate buffer solution, then homogenized for 2 min at 8000 rpm. A 1
:
10 sample homogenization solution was prepared.32 1 mL of the homogenized sample solution was extracted with a 1 mL sterile pipette after preliminary treatment and injected into a sterile tube containing 9 mL of diluent. After the mixture became uniform, a 1
:
100 sample homogenization solution was prepared. 10 mL of the diluted sample solution was injected into a prepared agar culture dish. The plate was rotated to effectively mix the materials. The plate was placed in Luria Bertani (LB) media at 37 °C with shaping for 48 h. After 48 h of culturing, the total number of colonies present on the plate was counted, with the number of colonies between 30 CFU and 300 CFU and no spread colonies. The total number of colonies was calculated following eqn (6), |
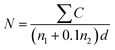 | (6) |
where N is the number of colonies in the sample, ∑C is the sum of the colonies, n1 is the number of first dilution plates, n2 is the number of second dilution plates, and d is the dilution factor.
2.6. Diallyl trisulfide treatment
Pretreatment of garlic samples entailed a multistep process. First, 2 g samples were weighed into a 50 mL beaker, transferred to a mortar with 4 mL of methanol and ground into a homogenate. The mixture was transferred into a 50 mL beaker, extracted by ultrasonication for 15 min, then diluted to 20 mL with methanol. Next, the sample was transferred to a 50 mL centrifuge tube, centrifuged for 10 min at 4000 rpm, and the resulting supernatant was removed. After filtration by microporous membrane, diallyl trisulfide was determined by liquid chromatography using a diallyl trisulfide standard.47 The chromatography column was an Eclipse Plus C18 (4.6 mm × 250 mm, 5 μm), the column temperature was 35 °C, the mobile phase was methanol
:
water (0.2% formic acid) at 80
:
20 (v/v), the flow rate was 1.0 mL min−1, the DAD detector wavelength was 240 nm, and the sample injection volume was 50 μL. A standard curve for diallyl trisulfide was obtained from the peak conditions, with a linear regression equation of y = 214.63x + 454.32 and a correlation coefficient of 0.9931, demonstrating a strong linear relationship within the concentration range 0–2.5 mg mL−1.
2.7. Sensory analysis
Analysis of the sensory characteristics was conducted by 25 semi-trained panelists in isolated cabins with the same lighting and temperature conditions.41 The evaluation was performed on a nine-point hedonic scale, in which 1 corresponded to ‘dislike extremely’ and 9 corresponded to ‘like extremely’. The panelists evaluated the garlic samples for the attributes of color, flavor, shape, texture and overall acceptability.41 A full score of 100 is 20 points for each criterion and less than 60 points was considered not edible.
2.8. Statistical analysis
The raw data of each parameter was subjected to analysis of variance (ANOVA). The statistical comparison of the treatments was performed using Tukey's HSD test at p ≤ 0.05. Eighteen days after storage (DAS), the fruits in some treatments were spoiled, making those samples unviable for comparison. Comparison of the remaining treatments was performed with an independent samples test. SPSS 20.0 software was used to perform the analysis. Differences were considered significant at p < 0.05 and p < 0.01.
3. Results and discussion
3.1. Weight loss and garlic firmness analysis
As shown in Fig. 1, the concentration of ozone impacted the quality of fresh peeled garlic, particularly in terms of weight loss. Some weight loss occurred in both the control and treatment groups. However, Fig. 1a details an important finding: compared to the control group, weight loss was slower in the ozone-treated garlic. The rate of water loss in the blank group significantly increased (p < 0.05) by 3.45%, contrary to other investigation results that reported increased water loss in ozone-treated fruit and vegetables.33 After treatment with different concentrations of ozone, the water loss in freshly peeled garlic could be significantly suppressed, as was the water evaporation. The study showed that the best effect was achieved after the 5 ppm ozone treatment, with only a 2.99% weight loss rate. In addition, the weight loss rate of fresh, peeled garlic stored at 4 °C displayed an upward trend, as seen in Fig. 1b. After 25 days of storage, the weight loss rate of the blank group was as high as 2.90%. However, after ozone treatment at 5 ppm, the weight loss rate was only 1.89%, which was the best effect documented herein. There were also other significant differences (p < 0.01) between the blank group and the 5 ppm group, including differences in firmness between the fresh and ozone-treated garlic. Compared to the control group, all ozone-treated garlic, except that treated with 5 ppm of ozone, was firmer at day 2. This suggests that treatment with appropriate ozone concentrations delays ripening, as shown in Fig. 2. The hardness of the fresh garlic decreased as storage time increased. The hardness of the garlic stored at 20 °C decreased slightly in the first 6 days, then rapidly decreased after 6 days, as seen in Fig. 2a. The degree of hardness present in the blank group was much lower than those in samples undergoing preservation treatment. This is primarily due to the fact that, as storage time increases, the pectin content in garlic decreases. In turn, this leads to a decrease in the adhesion between various substances, affecting the strength and density of garlic tissue. After 25 days of storage at 4 °C, the hardness of fresh, peeled garlic treated with a 5 ppm ozone concentration was 7.48 kg cm−2, 19.79% higher than that of the blank group (p < 0.01). Of the six treatment groups, this group achieved the best effect with regard to hardness. Flesh firmness is the parameter of greatest concern in garlic vegetable storage and marketing because flesh softening is associated with senescence and increases the susceptibility of vegetables to damage during handling, as outlined in Fig. 2b. In a study investigating overlapping concerns, Tzortzakis, Borland, Singleton, and Barnes38 reported that tomato fruit stored in ozone-enriched atmospheres (0.05–1.00 μmol mol−1) remained substantially firmer than fruit subjected to traditional storage conditions.
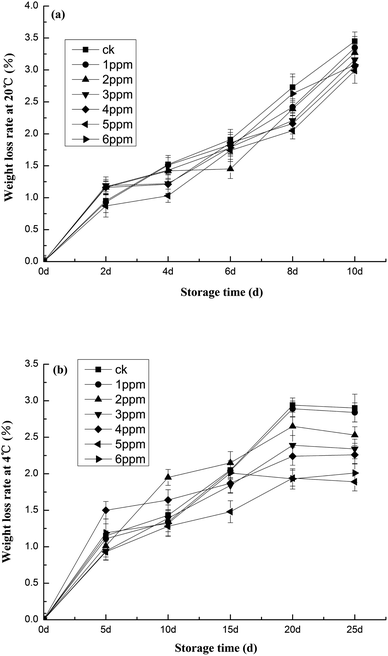 |
| Fig. 1 The effects of different ozone concentrations on the weight loss rate of garlic studied at (a) 20 °C and (b) 4 °C storage temperatures. | |
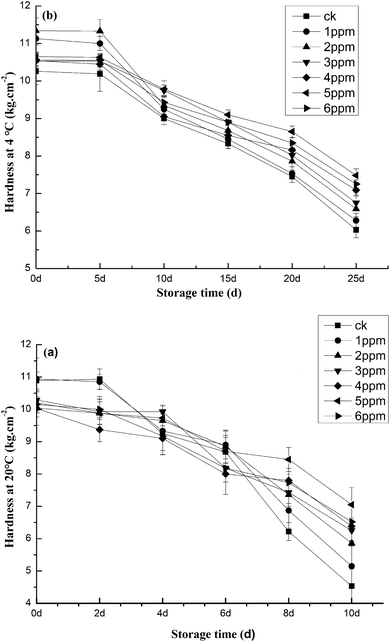 |
| Fig. 2 The effects of different ozone concentrations on the hardness of garlic studied at (a) 20 °C and (b) 4 °C storage temperatures. | |
It has been suggested that higher levels of ozone may result in damage to the cuticle and/or epidermal tissues.33 The reduced weight loss exhibited in the ozone-treated garlic could be attributed to its relatively thicker cuticle compared to other fruits and vegetables such as tomatoes and cranberries. Paull and Chen34 suggested that the major pathways for weight loss in garlic develop as a result of stem scarring, as well as from within the stomata and the cuticle. Consequently, the amount of water lost by garlic may differ depending on the cuticle thickness, which is cultivar- and maturity-dependent. Weight loss in fresh commodities was found to be unaffected by low-level ozone treatment, but increased when exposed to higher concentrations of ozone—an effect also observed in ‘Zee Lady’ peaches33,35 and tomatoes.36 As previously discussed, water loss and evaporation rates were significantly suppressed after treatment. Further, garlic firmness decreased as storage time increased. Since ozone reacts rapidly with ethylene, it is a promising tool for extending food storage life, particularly for commodities that benefit from ethylene removal during storage, such as banana, papaya, and persimmon. Ozone also possesses the added advantage of controlling disease proliferation. Moreover, ozone treatments were able to delay some processes associated with ripening in fruit, contributing to improved firmness retention during storage.
3.2. Germination rate and decay rate analysis
Fig. 3 details evidence that the germination rate of the blank group is higher than that of each treatment group. After 6 days of storage at 20 °C, the germination rate of the blank group increased sharply, with the fastest growth rate occurring between 6 days and 10 days. At 10 days, the germination rate of the blank group reached 85.26%. As seen in Fig. 3a, the germination rates of each treatment, from highest to lowest, were 80.00%, 82.36%, 70.25%, 44.29%, 35.58%, and 44.09%, respectively. The lowest germination rate occurred in the 5 ppm oxygen concentration treatment group. The germination rate of garlic stored at 4 °C was lower. Fig. 3b delineates the germination rates after 25 days of storage—in garlic treated with 6 ppm of ozone, the rate was only 30.26%. The germination rate was almost the same as that of 5 ppm ozone treatment, only 57.69% that of the blank control group. Putrefactive bacteria consume the nutrients found in garlic. The metabolites they produce also cause decay and deterioration.37 The effect of the different treatments on the decay rate of fresh, peeled garlic is shown in Fig. 4. With 20 °C storage, the decay rate of the blank control group without ozone treatment and the experimental group with low-concentration ozone treatment both reached 100% on the 10th day, thereby negating any storage value. In contrast, as Fig. 4a makes apparent, the group treated with an ozone concentration of 5 ppm fared the best, displaying the lowest decay rate of 42.86%. Storage at 4 °C further decreased the decomposition rate. When the garlic was stored at this temperature for 25 days, the rate at which the blank group rotted was 37.50%, while the rate of the ozone-treated group was only 12.50%, clearly indicating a better sustenance of freshness. Fig. 4b provides results pertaining to the germination rate, suggesting that the optimal ozone concentration for fresh, peeled garlic is 5 ppm and the optimal storage temperature is 4 °C.
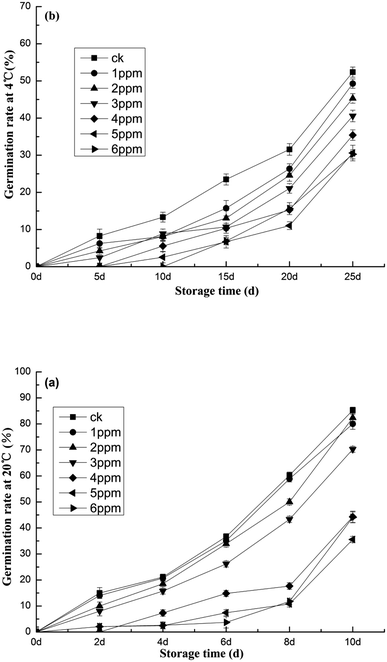 |
| Fig. 3 The effects of different ozone concentrations on garlic germination studied at (a) 20 °C and (b) 4 °C storage temperatures. | |
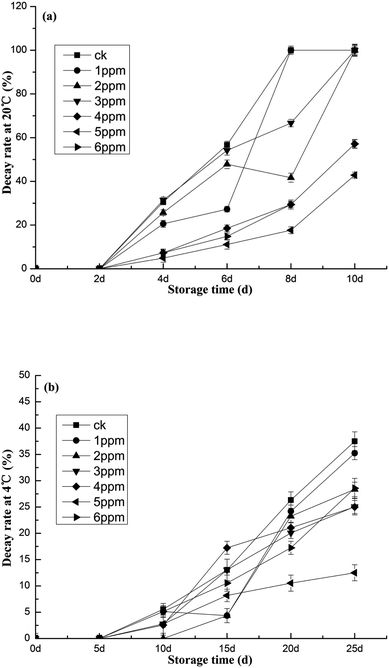 |
| Fig. 4 The effects of different ozone concentrations on the garlic decay rate studied at (a) 20 °C and (b) 4 °C storage temperatures. | |
Previous studies have demonstrated that S-alk(en)ylcysteine sulfoxide content depends mainly on genetic factors and the post-harvest storage conditions of garlic bulbs, while the edaphoclimatic conditions during the growth of the plant exert less influence.20 Horníčková et al.20 reported a significant increase in S-alk(en)ylcysteine sulfoxide content after prolonged storage of fresh garlic. However, these results were obtained after eight weeks of storage at 4 °C. Some companies that market fresh garlic store the product at −2 °C in order to keep it in its dormant state (dormancy) and avoid germination of the garlic clove (sprouting). Garlic germinates easily after peeling, then undergoing the transfer and consumption of its own nutrients. The bulb shrinks and shrivels, becoming inedible. Results from this study indicate that ozone-treated garlic refrigerated at 4 °C displays slower germination and decay rates, retaining better sensory palatability during storage. Compared to those at 20 °C storage, the germination and corruption rates were greatly reduced at 4 °C. Appearance is another important concern for consumers when assessing the quality of a vegetable. As reported by Ojagh et al.,54 ozone treatment incorporated with storage at 4 °C was found to prolong the healthy and desirable appearance of garlic during storage, especially with the 5 ppm ozone concentration. In conclusion, combining a 5 ppm ozone concentration with low-temperature storage at 4 °C is a safe method for prolonging vegetable freshness.
3.3. SSC, titratable acid (TA) and vitamin C analysis
Soluble solids can reflect the content of soluble sugar, acid, vitamins, minerals, and other major nutrients in fruits and vegetables. An appropriate soluble solids content provides robust flavor to fruits.26 SSC in whole garlic tends to increase with vegetable maturation. The SSC for the control group (9%) peaked at day 8 when the fruit ripened. SSC increased during storage, with a significant and observable difference between treated and untreated samples. It can be seen in Fig. 5 that the content of soluble solids in garlic does not correlate to the extension of storage time as might be expected. This may be an effect of the depletion of nutrients caused by respiration during storage, which may also be converted from other polysaccharides. In general, after being treated with the 5 ppm ozone concentration and stored at 4 °C, the soluble solid content of garlic remained at a high level. After being stored for 25 days, its SSC was measured at 9.15 g 100 mL−1. This result demonstrates that ozone treatment with a concentration of 5 ppm combined with low temperature storage at 4 °C can effectively maintain the soluble solid content of fresh peeled garlic. As Fig. 5a makes clear, ozone treatment and low-temperature storage promote and help maintain freshness.
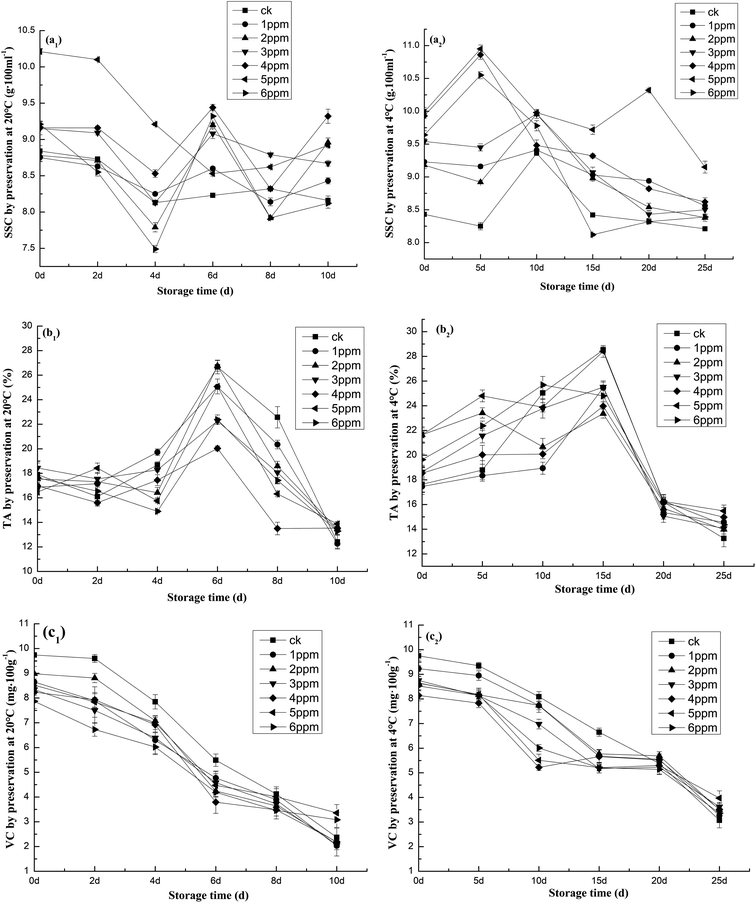 |
| Fig. 5 Effects of different ozone concentrations on soluble solids at (a1) 20 °C and (a2) 4 °C, titratable acidity at (b1) 20 °C and (b2) 4 °C and vitamin C at (c1) 20 °C and (c2) 4 °C storage temperatures. | |
The trend of the change in titratable acid follows that of SSC: they are both slower and stable in the later stages. On the final day, titratable acid levels were lower than on the first. Fig. 5b shows the ascorbic acid contents of control and ozone-treated fruits. The ascorbic acid contents of both control and ozone-treated fruits increased as the fruit ripened. The overall trend of ascorbic acid content reached the highest value on the 8th day and the decreasing trend of the ozone treatment group was slower than that of the control group in 20 °C storage. This indicates that ozone treatment can effectively inhibit titratable acid degradation in garlic (Fig. 5b1). At 4 °C, it peaked at 18 days and the overall trend was the same as that for 20 °C. Upon exposure to 5 ppm of ozone for 15 min, the concentration of TA increased significantly (p < 0.05) to 13.87% after 10 days of storage compared to the control (12.38%). The results obtained were significantly different for each treatment (p < 0.05) as determined by this test (Fig. 5b). The content of TA in garlic treated with ozone was increased. This has also been observed in ozone-treated strawberries.39 Pérez39 reported that the content of TA in strawberries increased significantly by 0.15 mg 100 g−1 after exposure to 0.35 ppm ozone for 3 days at 2 °C. It has been proposed that the increase in ascorbic acid in ozone-treated fruits and vegetables may be due to the inhibitory effects of ozone on the activity of several enzymes, such as ascorbate peroxidase and ascorbate oxidase.
As storage time increased, garlic acids continued to decrease. Similarly, vitamin C also decreased. Certain concentrations of ozone can stimulate antioxidant activity. Treatment incorporating a low concentration of ozone can enhance the ability of fruits and vegetables to synthesize vitamin C. Ozone treatment can also help vitamin C content decrease more slowly. However, ozone may destroy fruits‘ and vegetables’ antioxidant systems when deployed at higher concentrations and may also contribute to vitamin C oxidation.39 Fig. 5c shows that during storage, the vitamin C content of garlic follows a downward trend. In garlic treated with different ozone concentrations, the vitamin C decreased relatively slowly. In garlic stored at 20 °C, the vitamin C content was, at its highest, 3.36 mg 100 g−1 and also displayed the slowest decrease (Fig. 5c1). After 25 days of storage at 4 °C, the vitamin C content of the 5 ppm ozone concentration treatment group was 3.97 mg 100 g−1 and demonstrated the smallest decrease and the best preservation effect (Fig. 5c2).
Ali27 observed a slight increase in SSC as the storage time increased. The effect was more apparent in samples treated with the higher ozone concentration. The highest SSC (11.25%) in Allium sativum was found at day 10 after treatment with 2.5 ppm of ozone, whereas the untreated vegetables reached 9% SSC at day 8. The delayed peak of SSC at day 10 for the ozone-treated garlic was associated with an ozone-induced delay in ripening compared to the untreated fruit. However, the SSC for both the control and ozone-treated garlic decreased after 8 days and 10 days, respectively, and was associated with over-ripeness or senescence. In related research, Tzortzakis et al.14,38 reported that the soluble sugar content of tomato fruits previously stored in an ozone-enriched atmosphere (0.05–1.00 μmol mol−1) was significantly higher than that present in control fruit. Overall, ozone-treated garlic was sweeter or had higher SSC in comparison to non-treated fruit. As explained by Tzortzakis et al.,38 the levels of the dominant non-structural carbohydrate fractions (fructose and glucose) were sustained following ozone treatment. This is consistent with the recognized influence of non-structural carbohydrate and organic acid balance on the taste and flavor of ozone-treated fruit, which is perceived as the degree of sweetness over sourness. The change in titratable acid is much more rapid than that of soluble solids. Ascorbate oxidase is a copper-containing enzyme responsible for the enzymatic degradation of ascorbic acid (AA). Ascorbate oxidase oxidizes ascorbic acid to dehydroascorbic acid (DHA). However, high ozone concentrations can be injurious to the commodity. These higher concentrations may reduce ascorbic acid by promoting the activity of ascorbate oxidase. In this case, the ascorbic acid content significantly increased in ozonated garlic during storage. Pérez39 and Shah40 suggested that ozone stress may lead to the biosynthesis of ascorbic acid from carbohydrate reserves. The toxicity of ozone is related to its potential to form different reactive oxygen species (ROS) inside the cell. As an antioxidant, ascorbic acid plays a significant role in the detoxification process that results from the formation of different ROS, such as hydrogen peroxide (H2O2), superoxide radicals (O−), and hydroxyl radicals (OH2+), inside the plant cell. However, the vitamin C content declines concurrently with the degradation of fruit tissues when the fruit becomes overripe. When stored at 4 °C, vitamin C content decreased slowly. The ozone treatment at 4 °C inhibited the enzymatic activity, restricting the rate of degradation,42 and could be ascribed to enzymatic (via ascorbic acid oxidase) oxidation in the presence of oxygen. In frozen products, enzymatic reactions are slow but not completely eliminated because they can occur in the presence of non-frozen water.43 However, it should also be noted that since it is a water-soluble compound, part of the vitamin C content may be lost through drip loss44–46 as reported by Martins and Peter.48
3.4. PPO activity analysis
The activity of PPO was inhibited to different degrees after treatment with different concentrations of ozone, as seen in Fig. 6. After 10 days of storage at 20 °C, the activity of PPO was obviously inhibited. At 10 days, the activity of PPO was 5.40 × 10−4 ΔOD420/min g−1 in the 5 ppm ozone concentration treatment group (Fig. 6a). After 25 days of storage at 4 °C, the activity of PPO was 6.25 × 10−4 ΔOD420/min g−1, which was 32.80% higher than that of the blank group (Fig. 6b). At the early stages of storage at 20 °C, due to mechanical damage, the intracellular phenols of garlic were released and contacted polyphenol oxidase, thus increasing the enzyme activity. The activity of polyphenol oxidase decreased gradually with the prolonging of storage time. On the tenth day, the activity of PPO in the blank control group (CK) showed a significant downward trend, which indicated that the cells in CK group began to deteriorate on the tenth day, while the activity of PPO in the ozone-treated group was well maintained and high under the freshness-retaining effect of ozone. At the same time, there was a significant difference between the CK group and experimental group (P < 0.01), indicating that ozone treatment can promote organism wound healing. The results showed that ozone treatment could promote wound healing and prolong the storage time. The highest activity, found after 5 ppm treatment, should indicate the optimal treatment concentration.
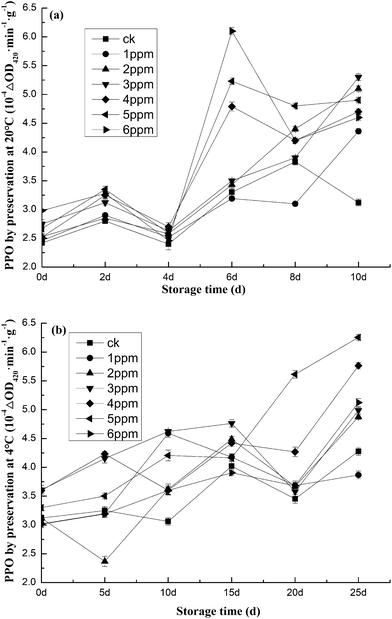 |
| Fig. 6 Effects of different ozone concentrations on the activity of polyphenol oxidase (ΔOD420/min g−1) at (a) 20 °C and (b) 4 °C. | |
Under the storage temperature of 4 °C, 1 ppm and 2 ppm ozone did not significantly promote the wound healing of the organism and the PPO activity was lower than that of the CK group at 5 days. This is possibly due to the effects of low temperature on enzyme activity and ozone delaying the browning (Fig. 6b). At 25 days, the PPO activity showed an overall upward trend. Combined with the sense testing, the color changes were not significant at 5 ppm and the browning phenomenon was not as obvious as that in the CK group. It can be seen that the main reason for the increase of PPO activity in the 5 ppm group was not browning, but the increase of garlic germination rate and the decrease of water content.52 This corresponds to the germination rate of the second part and the water loss rate of the first part. Therefore, it can be shown that PPO activity remained the best after 5 ppm treatment and PPO activity was relatively well retained after 5 ppm treatment at 4 °C, effectively prolonging the storage time. Previous studies have reported that the methanolic extract from Aristolochia species (A. indica, Aristolochia baetica, Aristolochia paucinervis, and Aristolochia bodamae) contains a high amount of phenols.53 However, the phenol and flavonoid contents vary from study to study.58,60,61 Several factors may affect how much of each is present in a given commodity. Studies have shown that extrinsic factors (such as geographic and climatic factors) and genetic factors play a role. However, the degree of maturation of the plant, as well as the length of storage time, also have a strong influence on the polyphenol content.62–64
3.5. Analysis of the total number of colonies
The sterilization mechanism of ozone occurs through the release of new ecological oxygen and its decomposition diffusion inside the space; it can quickly penetrate the cell membranes and cell walls of fungi, bacteria and other microorganisms, causing cell membrane damage, and continue to penetrate the membrane tissues, causing bacterial enzyme system damage, protein denaturation, and the disorder and suspension of normal physiological metabolism, shocking bacteria with disinfection, sterilization and antisepsis effects.39 The higher the ozone concentration, the more effective it is at killing the microorganisms present on the garlic surface. As can be seen in Fig. 7, on day 0, under different ozone concentration treatments and different storage temperatures, the total number of microbial colonies was zero. This may be due to the secretion of garlic having a certain bactericidal effect.55,57 Strictly speaking, the number of colonies on day 0 was less than 40 CFU g−1 according to the dilution ratio in the scheme and the weight of the sample. In garlic stored at 20 °C, the largest increase was observed between 2 days and 4 days. At 10 days, the total number of garlic bacteria after 6 ppm treatment was 38.63% lower than that of the blank group, demonstrating the best inhibitory effect on the bacteria (Fig. 7a). After storage at 4 °C for 25 days, the total number of garlic bacteria after treatment with 6 ppm ozone was 1.2 × 105 CFU g−1, 50% lower than the blank group, showing the best inhibitory effect on the bacteria. Freshness was particularly enhanced under these conditions (Fig. 7b). It can be seen that even as storage temperatures were changed, the 6 ppm ozone concentration produced the best bacterial inhibition effect to extend freshness. In the two different temperature storage environments, the degree of microbial growth varied with length of storage. When ozone concentration was consistent across groups, the storage temperature of 4 °C prevailed as a better choice for inhibiting microbial growth. For example, microbial logarithms measured after treatment with 6 ppm of ozone and storage for 25 days at 4 °C were 55.6% less than those measured after 10 days of storage at 20 °C.
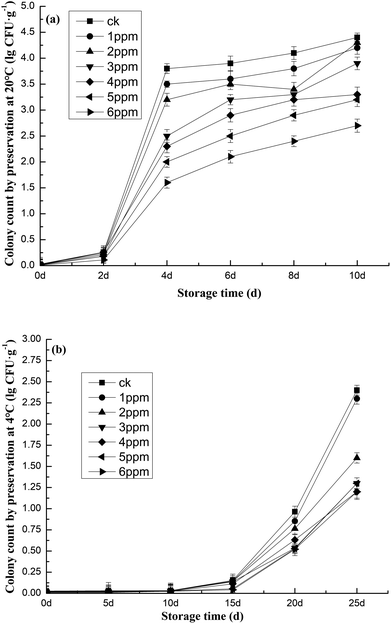 |
| Fig. 7 Colony counts (log CFU g−1) of garlic throughout storage time at (a) 20 °C and (b) 4 °C after treatment with different ozone concentrations. | |
Microbiological detection in food is a major issue and a focus of global food safety regulation. The results of this study indicated enhanced microbial inhibition during low temperature refrigeration, which likely points to a higher vulnerability to sub-lethal damage from O3 exposure.59,65 When microorganisms are subjected to environmental stress such as low temperature refrigeration, several outcomes can occur. Some cells may not exhibit any detrimental effects, some are totally destroyed, and still others may experience sublethal or metabolic injury. In a nonselective environment, injured cells repair their damage and proceed with growth and multiplication.48,49 Extracellular solute concentrations, intracellular solute toxicity, temperature shock, ice crystal production, and dehydration are all factors that have a destructive impact on specimens during low-temperature cold storage.50,64
3.6. Ozone treatment on fresh strip of diallyl trisulfide analysis
Diallyl trisulfide not only gives fresh garlic its characteristic odor,66 but has also been shown to kill many pathogenic bacteria in vitro.67,68 These results indicate that it is an important indicator of the freshness of garlic, as well as the quality of its storage. Fig. 8 shows that with the extension of storage time, the treatment groups resisted diallyl trisulfide reduction.47 As reflected in Fig. 8a, garlic stored at 20 °C after treatment with 5 ppm of ozone maintained relatively high diallyl trisulfide contents up to 0.965 mg mL−1. Similarly, Fig. 8b shows that, in garlic stored at 4 °C, diallyl trisulfide content was highest in the group treated with an ozone concentration of 5 ppm. Under these conditions, the initial content of diallyl trisulfide was 1.04 mg mL−1 and the final content of diallyl trisulfide was only reduced by 3.98% and was 11.2% higher (P < 0.01) than that of the blank group. This shows that an ozone concentration of 5 ppm is beneficial to preservation. Horev-Azaria et al.70 treated vascular endothelial cells with diallyl trisulfide and found that it could effectively increase the level of GSH in cells. A possible role was suggested for diallyl trisulfide in preventing reactive oxygen species damage by increasing the cellular GSH level.
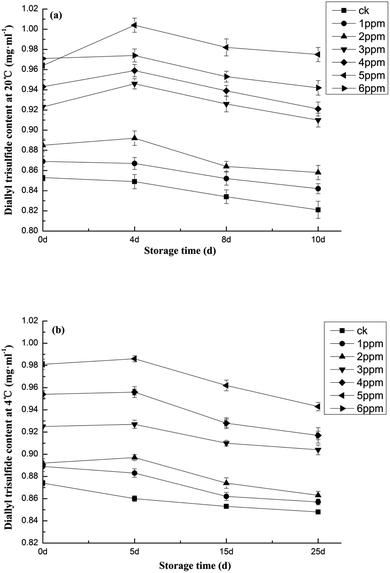 |
| Fig. 8 Effects of different concentrations of ozone treatment on diallyl trisulfide content during garlic storage at (a) 20 °C and (b) 4 °C. | |
Diallyl trisulfide is a particularly noteworthy compound found in garlic. The therapeutic properties of garlic are largely derived from diallyl trisulfide, but it may come as a surprise to understand that the fresh, whole garlic does not contain diallyl trisulfide. Rather, fresh, whole garlic contains unstable diallyl trisulfide, the precursor of diallyl trisulfide. Diallyl trisulfide, an odor-free, unstable substance, is abundant in garlic. After fresh garlic is sliced or broken, the unstable diallyl trisulfide produces diallyl trisulfide as a result of enzymatic action.47 Diallyl trisulfide is an active component in garlic and an efficient thiol trap. It readily passes through cell membranes into the cytosol, where it behaves like a redox toxin by oxidizing the cellular glutathione (GSH) pool and producing S-allylmercaptoglutathione (GSSA). It positively impacts many physiological functions, such as cholesterol level maintenance, tumor prevention, and blood pressure reduction. It also possesses a wide spectrum of antibacterial properties. Its inhibitory effects on Gram-positive bacteria, Gram-negative bacteria, and fungi have been documented69 and it serves as a natural preservative. It has also been observed that diallyl trisulfide affects the level of GSH.70
3.7. Ozone treatment on fresh strip of sensory analysis
Fig. 9 shows a chart based on the scores obtained in the sensory tests.41 According to Fig. 9a, with the prolongation of time, the sensory properties of all garlic samples stored at 20 °C showed a downward trend. On the tenth day of storage, the CK group had the lowest sensory score, which was lower than 60 points and is considered inedible. The sensory score of garlic treated with 5 ppm ozone was the highest and was significantly different (P < 0.01) from that of the CK group. The CK group, the 1 ppm group and the 6 ppm group showed a sharp decrease in sensory scores after 6 days. This may be because the increase in microorganisms on day 6 is associated with a sudden increase in soluble solids. Sensory scores in the 2 ppm and 5 ppm groups tended to be stable and did not show a sharp decline. The 5 ppm group had the most stable trend in sensory evaluation and the smallest decrease. All garlic groups stored at 4 °C also showed a downward trend in sensory scores (Fig. 9b). However, compared with those stored at 20 °C, the 4 °C sensory scores were higher and all remained higher than the minimum usable value score. This may be because at a low temperature of 4 °C, the activity of enzymes was reduced and the germination and decay rates were inhibited, extending the preservation time. Day 25 showed a minimum sensory score of 80 for the CK group and a maximum score of 92 for the 5 ppm ozone concentration treatment group (p < 0.05). There was no significant difference between these scores and those of day 0 (P > 0.05). In conclusion, the group treated with an ozone concentration of 5 ppm and stored at 4 °C was the group with the best sensory score.
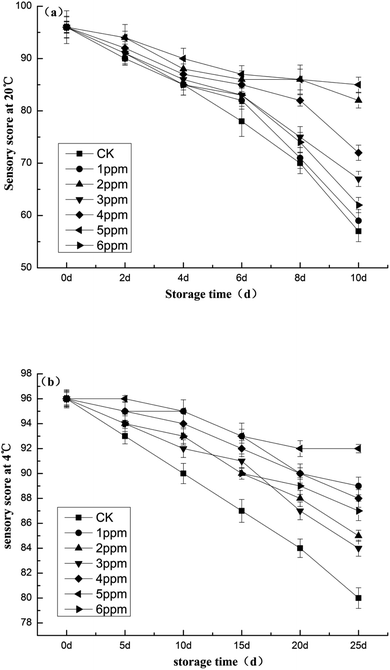 |
| Fig. 9 Effects of different concentrations of ozone treatment on sensory score during garlic storage at (a) 20 °C and (b) 4 °C. | |
4. Conclusions
This study found that ozone treatment exhibited various impacts on garlic fruit. The results indicate that gaseous 1.5–6 ppm ozone treatment for 15 min is useful in enhancing the nutritional properties of garlic. The indexes of garlic stored at different temperatures were determined throughout storage. It was found that storage at 4 °C better inhibited the growth of bacteria and mold. Combined with the bactericidal effect of different concentrations of ozone, the effect of prolonging the storage period of fresh peeled garlic was obvious. At the same time, all indexes were maximized with the ozone concentration of 5 ppm, which effectively extended the shelf life of garlic and showed significant differences from the blank control group (P < 0.05). In summary, garlic maintains optimal freshness when stored at 4 °C and treated with 5 ppm of ozone gas. In the bacterial study, however, we only measured the total number of garlic colonies. For better preservation, we still need to carry out further research on pathogenic garlic bacteria and the inhibitory effect of ozone on pathogenic bacteria.
Conflicts of interest
There are no conflicts to declare.
Acknowledgements
This work is supported by the Science and technology support program of Sichuan [2019YFN0174, 2018NZ0090, 2019NZZJ0028 and 2016FZ0019], Science and technology support program of Yibin [2018ZSF002], Chengdu Science and Technology Project-key research and development program [2018-YF05-00213-SN], Sichuan Provincial Key Laboratory Open Fund Project [GR-2018-E-01], and Innovation Team Construction Program of Sichuan Education Department [15TD0017]. Entrepreneurship Training Program (Sichuan Province) of Xihua University [201710623070].
References
- T. M. M Ángeles, P.-A. Jesús, M.-O. Alicia and M.-R. Rafael, Influence of Variety and Storage Time of Fresh Garlic on the Physicochemical and Antioxidant Properties of Black Garlic, Foods, 2019, 8(8), 314 CrossRef PubMed.
- K. Rahman, Garlic and aging: New insights into an old remedy, Ageing Res. Rev., 2003, 2, 39–56 CrossRef PubMed.
- H.-J. Lee, D.-K. Yoon, N.-Y. Lee and C.-H. Lee, Effect of Aged and Fermented Garlic Extracts as Natural Antioxidants on Lipid Oxidation in Pork Patties, Food Sci. Anim. Resour., 2019, 39(4), 610–622 CrossRef PubMed.
- M. H. Kim, M. J. Kim, J. H. Lee, J. I. Han, J. H. Kim, D.-E. Sok and M. R. Kim, Hepatoprotective effect of aged black garlic on chronic alcohol-induced liver injury in rats, J. Med. Food, 2011, 14, 7–8 Search PubMed.
- S. Jin-ichi, L. Chao, M. Einosuke, T. Mami and H. Katsunori, Processed Black Garlic Extracts Enhance Anti-Tumor Potency against Mouse Tumors, J. Medicinal Aromat. Plants, 2007, 1, 278–281 Search PubMed.
- E. K. Jang, J. H. Seo and S. P. Lee, Physiological activity and antioxidative effects of aged black garlic extract, Korean J. Food Sci. Technol., 2008, 40, 443–448 Search PubMed.
- Y.-J. Seo, O.-C. Gweon, J. Im, Y.-M. Lee, M.-J. Kang, J.-I. Kim and P. Nutr, Effect of Garlic and Aged Black Garlic on Hyperglycemia and Dyslipidemia in Animal Model of Type 2 Diabetes Mellitus, Food Sci., 2009, 14, 1–7 CAS.
- J. H. Ryu and D. Kang, Physicochemical properties, biological activity, health benefits, and general limitations of aged black garlic, Molecules, 2017, 22, 919 CrossRef PubMed.
- M. B Gabriela, F.-S. Otniel and F. J. Murillo, Understanding the Role of Chlorine and Ozone to Control Postharvest Diseases in Fruit and Vegetables: A Review, Curr. Nutr. Food Sci., 2020, 16(4), 455–461 CrossRef.
- S. Kimura, Y. C. Tung, M. H. Pan, N. W. Su, Y. J. Lai and K. C. Cheng, Black garlic: A critical review of its production, bioactivity, and application, J. Food Drug Anal., 2017, 25, 62–70 CrossRef CAS PubMed.
- J. G. Kim, A. E. Yousef and S. Dave, Application of ozone for enhancing the microbiological safety and quality of foods: a review, J. Food Protect., 1999, 62(9), 1071–1087 CrossRef CAS PubMed.
- J. M. Jay, M. J. Loessner, D. A. Golden, Modern food microbiology, Springer, US, 2005–01–01 Search PubMed.
- D. Xu, M. Shi, B. Jia, Zh. Yan, L. Gao, W. Guan, Q. Wang and J. Zuo, Effect of ozone on the activity of antioxidant and chlorophyll-degrading enzymes during postharvest storage of coriander (Coriandrum sativum L.), J. Food Process. Preserv., 2019, 43(8), e14020 Search PubMed.
- A. Asgar, K. O. Mei and F. F. Charles, Effect of ozone pre-conditioning on quality and antioxidant capacity of papaya fruit during ambient storage, Food Chem, 2014, 142, 19–26 CrossRef PubMed.
- S. Naito and H. Takahara, Ozone Contribution in Food Industry in Japan, Science & Engineering, 2006, 28(6), 425–429 Search PubMed.
- N. Shigezo, O. Kazuhisa and I. Youzi, Swelling spoilage of smoked squid products caused by lactic acid bacteria and inactivation of these bacteria by ozonated water, Bokin Bobai, 2001, 29, 497–505 Search PubMed.
- K. A. Hirneisen, S. M. Markland and K. E. Kniel, Ozone Inactivation of Norovirus Surrogates on Fresh Produce, J. Food Protect., 2011, 74(5), 836–839 CrossRef CAS PubMed.
- M. Y. Lim, J.-M. Kim, J. E. Lee and G. P. Ko, Characterization of ozone disinfection of murine norovirus, J. Appl. Environ. Microbiol., 2010, 76(4), 1120–1124 CrossRef CAS PubMed.
- A. T.-E. Jeanette, N. H. Charles, J. Joseph and P. G. Charles, Inactivation
of enteric adenovirus and feline calicivirus by ozone, Water Res, 2005, 39(15), 3650–3656 CrossRef PubMed .
- J. Horníčková, R. Kubec, K. Cejpek, J. Velíšek, J. Ovesná and H. Stavělíková, Profiles of S-alk(en)ylcysteine sulfoxides in various garlic genotypes, Czech J. Food Sci., 2010, 28(4), 298–308 CrossRef.
- S. Horvitz and M. J. Cantalejo, Application of Ozone for the Postharvest Treatment of Fruits and Vegetables, Crit. Rev. Food Sci. Nutr., 2014, 54(3), 312–339 CrossRef CAS PubMed.
- E. G. Kristen, A. Giselle, L. J. Sarah, W. Kacy and A. L. Jung, Inactivation of bacteria on fresh produce by batch wash ozone sanitation, Food Contr., 2019, 106, 106747 CrossRef.
- R. M. Callejón, R.-N. M. Isabel, U. Cristina, H.-O. Ruth, P. M. C Garcia and T. M. Ana, Reported foodborne outbreaks due to fresh produce in the United States and European Union: trends and causes, Foodborne Pathog. Dis., 2015, 12(1), 32–38 CrossRef PubMed.
- G. Romanazzi, A. Lichter, F. M. Gabler and J. L. Smilanick, Recent advances on the use of natural and safe alternatives to conventional methods to control postharvest gray mold of table grapes, Postharvest Biol. Technol., 2011, 63(1), 141–147 CrossRef.
- A. K. Stephen, J. M. Linda, E. M. Dirk and P. W. Charles, Efficacy and fumigation characteristics of ozone in stored maize, J. Stored Prod. Res., 2001, 37(4), 371–382 CrossRef.
- G. Manousaridis, A. Nerantzaki, E. K. Paleologos, A. Tsiotsias, I. N. Savvaidis and M. G. Kontominas, Effect of ozone on microbial, chemical and sensory attributes of shucked mussels, Food Microbiol., 2005, 22(1), 1–9 CrossRef CAS.
- A. Asgar, K. O. Mei and F. F. Charles, Effect of ozone pre-conditioning on quality and antioxidant capacity of papaya fruit during ambient storage, Food Chem., 2014, 142, 19–26 CrossRef PubMed.
- S. Ranganna, Handbook of analysis and quality control for fruit and vegetable products, Tata McGraw-Hill Education Pvt. Ltd., 1999 Search PubMed.
- S. Nithyanantha, G. Shreyoshi, M. I. Hassan and C. Thirunavukkarasu, Enhanced hepatocyte survival and anti-apoptosis via Akt by Diallyl trisulfide, augments hepatic regeneration through hydrogen sulfide in partially hepatectomized rats, Phytomedicine, 2021 DOI:10.1016/J.PHYPLU.2021.100048.
- H. Uğur, J. Çatak, Ö. F. Mızrak, N. Çebi and M. Yaman, Determination and evaluation of in vitro bioaccessibility of added vitamin C in commercially available fruit-, vegetable-, and cereal-based baby foods, Food Chem., 2020, 330, 127166 CrossRef PubMed.
- R. Massantini, R. Moscetti, D. Monarca, M. Cecchini, M.-M. Contini and L. M. Alfani, The influence of cover crops and double harvest on storage of fresh hazelnuts (Corylus avellana L.), Adv. Hortic. Sci., 2009, 23(4), 231–237 Search PubMed.
- J. Wang and L. Fan, Effect of ultrasound treatment on microbial inhibition and quality maintenance of green asparagus during cold storage, Ultrason. Sonochem., 2019, 58, 104631 CrossRef CAS PubMed.
- L. Palou, C. H. Crisosto, J. L. Smilanick, J. E. Adaskaveg and J. P. Zoffoli, Effects of continuous 0.3 ppm ozone exposure on decay development and physiological responses of peaches and table grapes in cold storage, Postharvest Biol. Technol., 2002, 24(1), 39–48 CrossRef CAS.
- R. E. Paull and N. J. Chen, Waxing and plastic wraps inflfluence water loss from papaya fruit during storage and ripening, J. Am. Soc. Hortic. Sci., 1989, 114, 937–942 Search PubMed.
- M. K. Ong, i F. K. Kaz, C. F. Forney and A. Ali, Effect of Gaseous Ozone on Papaya Anthracnose, Food Bioprocess Technol., 2013, 6(11), 2996–3005 CrossRef CAS.
- N. G. Shah, S. S. Zambre, K. V. Venkatesh, and R. C. Anantheswaran, in Annual meeting, Las Vegas, Nevada, USA, Institute of Food Technologists (IFT), 2004, 12–16 July Search PubMed.
- A. M. Fátima, L. M. S. Cristina and R. S. B. Teresa, A Review on Ozone-Based Treatments for Fruit and Vegetables Preservation, Food Eng. Rev., 2013, 5(2), 77–106 CrossRef.
- N. Tzortzakis, A. Borland, I. Singleton and J. Barnes, Impact of atmospheric ozone- enrichment on quality-related attributes of tomato fruit, Postharvest Biol. Technol., 2007, 45, 317–325 CrossRef CAS.
- A. G. Pérez, C. Sanz, J. J. Ríos and R. Olías, Effects of ozone treatment on postharvest strawberry quality, J. Agric. Food Chem., 1999, 47(4), 1652–1656 CrossRef PubMed.
- N. G. Shah, S. S. Zambre, K. V. Venkatesh and R. C. Anantheswaran, Ozone gas exposure to extend shelf life of tomatoes in hot and humid region, in Annual meeting, Institute of Food Technologists (IFT), Las Vegas, Nevada, USA, 2004 Search PubMed.
- R. M. Rahmatuzzaman, B. Majharulislam and A. A. Sabuz, Traceability of Sweeteners in Soy Yogurt using Linear Discriminant Analysis of Physicochemical and Sensory Parameters, J. Sci. Food Agric., 2021 DOI:10.1016/J.JAFR.2021.100155.
- A. Alhamdan, B. Hassan, H. Alkahtani, D. Abdelkarim and M. Younis, Freezing of Fresh Barhi Dates for Quality Preservation during Frozen Storage, Saudi J. Biol. Sci., 2016, 25, 1552–1561 CrossRef PubMed.
- B.-S. Cristina, S. Amanda, J. P.-P. Matthew, D. Lan, T. Gary, W. Tina, W. Delilah, U. Gideon, A. Justin, F. B. David, C. H. W. Vivian, R. Boris and M. H. Tara, Preservation of sweet cherry by isochoric (constant volume) freezing, Innovative Food Sci. Emerging Technol., 2019, 52, 108–185 CrossRef.
- A. Serpen, V. Gökmen, K. S. Bahçeci and J. Acar, Reversible degradation kinetics of vitamin C in peas during frozen storage, Eur. Food Res. Technol., 2007, 224(6), 749–753 CrossRef CAS.
- M. G. Elsa, A. Marta, R. S. B. Teresa and L. M. S. Cristina, Degradation kinetics of colour, vitamin C and drip loss in frozen broccoli (Brassica oleracea L. ssp. Italica) during storage at isothermal and non-isothermal conditions, Int. J. Refrig., 2011, 34(8), 2136–2144 CrossRef.
- E. M. Gonçalves, J. Pinheiro, M. Abreu, T. R. S. Brandão and C. L. M. Silva, Kinetics of quality changes of pumpkin (Curcurbita maxima L.) stored under isothermal and non-isothermal frozen conditions, J. Food Eng., 2011, 106(1), 40–47 CrossRef.
- J. N. Jeremic, V. L. Jakovljevic, V. I. Zivkovic, I. M. Srejovic, J. V. Bradic, I. M. Milosavljevic, S. L. Mitrovic, N. U. Jovicic, S. B. Bolevich, A. A. Svistunov, S. C. Tyagi and N. S. Jeremic, Garlic Derived Diallyl Trisulfide in Experimental Metabolic Syndrome: Metabolic Effects and Cardioprotective Role, Int. J. Mol. Sci., 2020, 21(23), 9100 CrossRef CAS PubMed.
- P. H. Calcott and R. A. MacLeod, Survival of Escherichia coli from freeze–thaw damage: a theoretical and practical study, Can. J. Microbiol., 1974, 20(5), 671–681 CrossRef CAS PubMed.
- P. S. Vasafi, N. Hamdami and J. Keramat, Quality and microbial stability of part-baked ‘Barbari bread’ during freezing storage, LWT–Food Sci. Technol., 2019, 104, 173–179 CrossRef CAS.
- H. T. Lin, Y. F. Xi and S. J. Chen, Post Harvest Softening Physiological Mechanism of Huang Hua Pear Fruit, Sci. Agric. Sin., 2003, 36, 349–352 Search PubMed.
- P. Tomasz, Effect of ozone treatment on glutathione (GSH) status in selected berry fruit, Phytochemistry, 2021, 187, 112767 CrossRef PubMed.
- C. B. S. Tong and K. B. Hicks, Sulfated polysaccharides inhibit browning of apple juice and diced apples, J. Agric. Food Chem., 1991, 39, 1719–1722 CrossRef CAS.
- A. J. McEvily, R. Iyengar and W. S. Otwell, Inhibition of enzymatic browning in foods and beverages, Crit. Rev. Food Sci. Nutr., 1992, 32(3), 253–273 CrossRef CAS PubMed.
- R. Sara, M. O. Seyed, P. Parastoo, S. Fabien, Y. Q. Siew, Shelf-life and quality of chicken nuggets fortified with encapsulated fish oil and garlic essential oil during refrigerated storage, Springer India, 2020, vol. 1–8 Search PubMed.
- V. U. Olugbue, V. O. Nwaugo, R. A. Onyeagba, S. S. Ogundapo, M. O. Okata and I. Oko, Antimicrobial Effects of Garlic Extracts against Multidrug Resistant Staphylococcus aureus Isolates from Clinical Specimens, J. Adv. Microbiol., 2017, 1–11 Search PubMed.
- E. O. Nasreddine, S. Karima, F. Saad, E. B. Omar, B. Abdelhakim, F. M. E. Abbes, K. Rabie and B. Malika, Evaluation of In Vitro Antioxidant and Antidiabetic Activities of Aristolochia longa Extracts, Evid. base Compl. Alternative Med., 2019, 2019, 7384735 Search PubMed.
- J. Wang and L. Fan, Effect of ultrasound treatment on microbial inhibition and quality maintenance of green asparagus during cold storage, Ultrason. Sonochem., 2019, 58, 104631 CrossRef CAS PubMed.
- A. Djeridane, M. Yousfi, B. Nadjemi, S. Maamri, F. Djireb and P. Stocker, Phenolic extracts from various Algerian plants as strong inhibitors of porcine liver carboxylesterase, J. Enzym. Inhib. Med. Chem., 2006, 21(6), 719–726 CrossRef CAS PubMed.
- D. Sivaraj, S. Shanmugam, M. Rajan, S. P. Sasidharan, S. Sathyanarayanan, K. Muniyandi, P. Thangaraj and A. A. de S. Araújo, Evaluation of Aristolochia indica L. and Piper nigrum L. methanol extract against centipede Scolopendra moristans L. using Wistar albino rats and screening of bioactive compounds by high pressure liquid chromatography: a polyherbal formulation, Biomed. Pharmacother., 2018, 97, 1603–1612 CrossRef CAS PubMed.
- T. Ozen, N. Bora, S. Yenigun and H. Korkmaz, An investigation of chemical content, enzyme inhibitory propert, antioxidant and antibacterial activity of Aristolochia bodamae Dingler (develiotu) (Aristolochiaceae) root extracts from Samsun, Turkey, Flavour Fragrance J., 2020, 35, 3 CrossRef.
- G. M. Ivana, L. Ivica, S. M. Sonja, A. Helena, Š. Vida, K. Ana, N. Tina and S. Danijela, Abiotic factors during a one-year vegetation period affect sage phenolic metabolites, antioxidants and antimicrobials, Ind. Crop. Prod., 2019, 141, 111741 CrossRef.
- C. Zhang, D. Yang, Z. Liang, J. Liu, K. Yan, Y. Zhu and S. Yang, Climatic factors control the geospatial distribution of active ingredients in Salvia miltiorrhiza Bunge in China, Sci. Rep., 2019, 9, 1 Search PubMed.
- K. Boudieb, A. S.-A. Kaki S and H. A. Chibane, Effect of maturation degree on the fixed oil chemical composition, phenolic compounds, mineral nutrients and antioxidant properties of Pistacia lentiscus L. Fruits, Not. Bot. Horti Agrobot. Cluj-Napoca, 2019, 47, 836–847 CAS.
- M. Mashabela, P. V. Mahajan and D. Sivakumar, Influence of different types of modified atmosphere packaging films and storage time on quality and bioactive compounds in fresh-cut cauliflower, Food Packag. Shelf Life, 2019, 22, 100374 CrossRef.
- L. Lawson, S. Wood and B. Hughes, HPLC Analysis of Allicin and Other Thiosulfinates in Garlic Clove Homogenates, Planta Med., 1991, 57(3), 263–270 CrossRef CAS PubMed.
- W. H. H. Gunther, Garlic and other alliums–the lore and the science, J. Sulfur Chem., 2013, 34, 1–2 CrossRef.
- R. Jana, B. Jan, D. Philipp, S. Wolfgang, G. Martin, C. H. Gruhlke, K. Michael and S. Alan, Investigation of the deposition behaviour and antibacterial effectivityof allicin aerosols and vapour using a lung model, Exp. Ther. Med., 2020, 19(2), 1541–1549 Search PubMed.
- J. Reiter, N. Levina, M. van der Linden, M. Gruhlke, C. Martin and A. J. Slusarenko, Diallylthiosulfinate (Allicin), a Volatile Antimicrobial from Garlic (Allium sativum), Kills Human Lung Pathogenic Bacteria, Including MDR Strains, as a Vapor, Molecules, 2017, 22(10), 1711 CrossRef PubMed.
- I. Hirosh, Use of an amino acid in the mobile phase for the determination of ascorbic acid in food by high-performance liquid chromatography with electrochemical detection, J. Chromatogr. A, 2000, 881(1), 317–326 Search PubMed.
- H.-A. Limor, E. Shlomit, I. Nira, P.-C. Sarah, M. David, M. Talia, R. Aharon, W. Meir, J.-H. Jasmine, A. Ninette and S. Naphtali, Allicin up-regulates cellular glutathione level in vascular endothelial cells, Eur. J. Nutr., 2009, 48(2), 67–74 CrossRef PubMed.
Footnote |
† Hong Liu and Lin Xu should be considered as first authors. |
|
This journal is © The Royal Society of Chemistry 2021 |
Click here to see how this site uses Cookies. View our privacy policy here.