DOI:
10.1039/D1RA00651G
(Paper)
RSC Adv., 2021,
11, 8367-8374
Application of [PVI-SO3H]NO3 as a novel polymeric nitrating agent with ionic tags in preparation of high-energetic materials†
Received
25th January 2021
, Accepted 15th February 2021
First published on 26th February 2021
Abstract
In this paper, poly(vinyl imidazole) sulfonic acid nitrate [PVI-SO3H]NO3 was synthesized and fully characterized. Then, [PVI-SO3H]NO3 was applied for the preparation of energetic materials such as 1,1-diamino-2,2-dinitroethene (FOX-7), pentaerythritol tetranitrate (PETN), 1,3,5-trinitro-1,3,5-triazinane (RDX) and trinitrotoluene (TNT). The major advantages of the presented methodology are mild, facile workup, high yields and short reaction times. [PVI-SO3H]NO3 is a suitable nitrating agent for in situ generation of NO2 and without using any co-catalysts of the described nitrating reagent.
Introduction
Nitro materials have widely been used in the preparation of energetic materials, dyes, plastics and pharmaceuticals.1–4 The most well-known of energetic materials are FOX-7 (1,1-diamino-2,2-dinitroethene), pentaerythritol tetranitrate (PETN), 1,3,5-trinitro-1,3,5-triazinane (RDX) and trinitrotoluene (TNT). Furthermore, these nitro compounds have been applied in dyes, pharmaceuticals, fuels, perfumes, medicine and plastics.5–7 These materials and derivatives such as pentaerythritol tetranitrate (PETN) were applied in therapy antianginal activity, activator guanylate and active ingredients of pharmaceuticals.8,9 Also, PETN was used to treat cardiovascular disease through vasorelaxant enzyme release.10,11
Architecture of novel renewable and sustainable nitrating reagent in the organic transformation has attracted much attention in the science and industry because of their unique properties, such as efficiency, reusability, reactivity, stability, selectivity, generality and easy separation of the catalyst.12–18 These methods of preparation of energetic materials were previously reported in the presence of some various nitrating agents, co-catalysts, and solvents, mixed acid of concentrated nitric acid and sulfuric acid, high temperature, long reaction times, and low yields19–30 (Scheme 1).
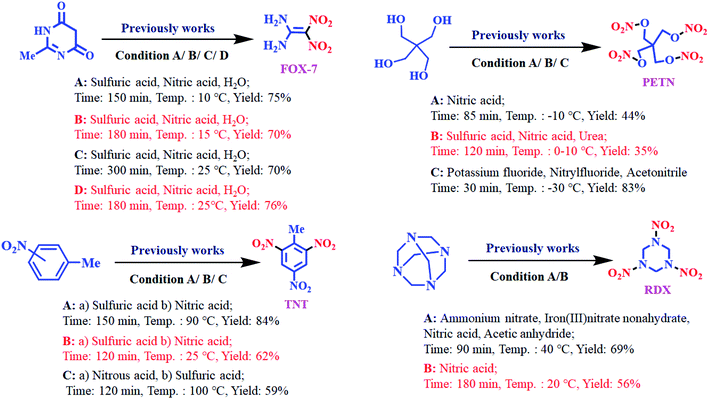 |
| Scheme 1 Synthesis of FOX-7 (1,1-diamino-2,2-dinitroethene), pentaerythritol tetranitrate (PETN), 1,3,5-trinitro-1,3,5-triazinane (RDX) and trinitrotoluene (TNT) according to the previously reports procedures. | |
Frequently, researcher groups have found that metal nitrates or nitrogen oxides can serve as nitrating reagents. Therefore, developing green nitrating agents has been growing fast in organic reaction. For this purpose, nitrating agents based on polymeric are highly effective systems. Polymeric nitrating agents have advantages such as (a) easy separation from the reaction mixture, (b) without excess use of nitrating reagents, (c) easy recyclability of nitrating polymer supports, (d) low toxicity, (e) and more stability and selectivity of nitrating polymer supports.31–34
In the recent years, development of catalysts and/or reagents based on N-heterocycles with sulfonic acids functional groups is our interest.35–39 In this regard, we have reported novel nitrating agents [Msim]NO3, [Py-SO3H]NO3 and [Dsim]NO3 for synthesis of nitro compounds and explosive materials.40–43 Herein, we wish to introduce poly(vinyl imidazole) sulfonic acid nitrate [PVI-SO3H]NO3 as a novel nitrating agent. [PVI-SO3H]NO3 was used for the synthesis of energetic materials such as 1,1-diamino-2,2-dinitroethene (FOX-7), pentaerythritol tetranitrate (PETN), 1,3,5-trinitro-1,3,5-triazinane (RDX) and trinitrotoluene (TNT) (Scheme 2).
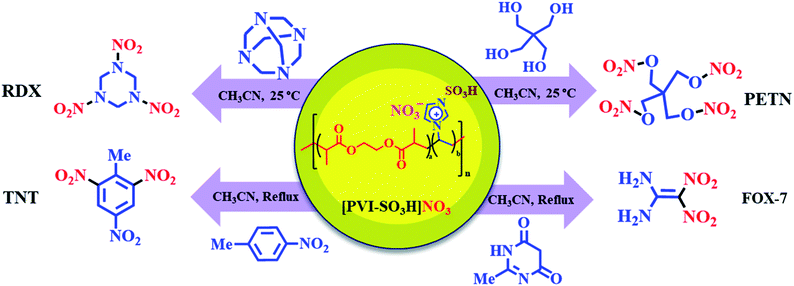 |
| Scheme 2 Synthesis of 1,1-diamino-2,2-dinitroethene (FOX-7), pentaerythritol tetranitrate (PETN), 1,3,5-triazinane (RDX) and trinitrotoluene (TNT) by [PVI-SO3H]NO3 as nitrating agent. | |
Experimental
General procedure for the preparation of poly(vinyl imidazole) sulfonic acid nitrate [PVI-SO3H]NO3
At first, poly(vinyl imidazole) (PVI) was synthesized according to the previously reported literature (Scheme 3).44,45 In a 25 mL round-bottomed flask, a mixture of poly(vinyl imidazole) (PVI) (1.410 g) and chlorosulfonic acid (5 mmol, 0.335 mL) in dry CH2Cl2 at 0 °C was stirred for 2 h.46 Then, nitric acid 100% (5 mmol, 0.20 mL) was added dropwise to [PVI-SO3H]Cl over a period of 5 minutes at room temperature. After this time, an orange powder appeared to give poly(vinyl imidazole) sulfonic acid nitrate [PVI-SO3H]NO3 (Scheme 3).
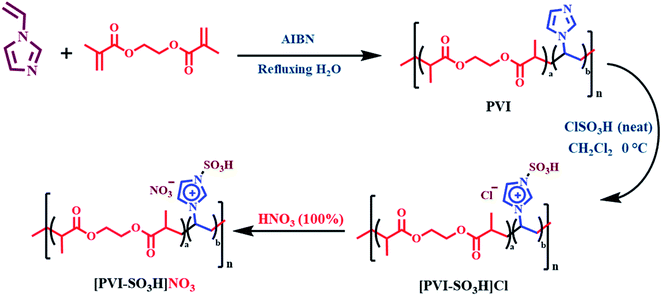 |
| Scheme 3 Preparation of poly(vinyl imidazole) sulfonic acid nitrate [PVI-SO3H]NO3. | |
General procedure for the preparation of pentaerythritol tetranitrate (PETN)
In a 25 mL round bottom flask containing 1.9 g of [PVI-SO3H]NO3 as reagent, of the pentaerythritol (1 mmol, 0.136 g) was added. Then, 10 mL of CH3CN was added to the reaction mixture and stirred at room temperature for 30 minutes. The reaction was followed by TLC technique. After completion of the reaction, the reagent residue was separated by filtration. The reaction solvent was evaporated. The mixture was extracted with ethyl acetate/water. The organic layer was dried over anhydrous sodium sulfate, filtered off and the solvent was evaporated. The residue was purified by column chromatography (silica gel, n-hexane: ethyl acetate 6/4) to give the desired product.
General procedure for the preparation of trinitrotoluene (TNT)
In a 25 mL flask containing 1.9 g of [PVI-SO3H]NO3 reagent and 1-methyl-4-nitrobenzene (1 mmol, 0.137 g) was added. Then, 10 mL of CH3CN solvent was added to the reaction mixture and stirred for 45 minutes under the reflux condition. The reaction was followed by TLC technique. After completion of the reaction, the reaction mixture was cooled and the reagent residue was separated by filtration. The reaction solvent was evaporated. The mixture was extracted with ethyl acetate/water. The organic layer was dried over anhydrous sodium sulfate, filtered and the solvent was evaporated. The residue was purified by column chromatography (silica gel, n-hexane: ethyl acetate 9/1) to give product.
General procedure for the preparation of 1,1-diamino-2,2-dinitroethene (FOX-7)
In a 25 mL flask containing 1.9 g of [PVI-SO3H]NO3 reagent and 2-methylpyrimidine-4,6(1H,5H)-dione (1 mmol, 0.126 g) was added. Then, 10 mL of CH3CN solvent was added to the reaction mixture and stirred for 60 minutes under the reflux condition. Reaction progress was followed by TLC technique (n-hexane: ethyl acetate 3/7). After completion of the reaction, the reagent was separated by filtration and 10 mL water was then added to the reaction mixture to perform the hydrolysis (for 10 minutes). The pure product was then recrystallized in water solvent.
General procedure for the preparation of 1,3,5-trinitro-1,3,5-triazinane (RDX)
In a 25 mL flask containing 1.9 g of [PVI-SO3H]NO3 reagent and hexamethylenetetramine (1 mmol, 0.140 g) was added. Then, 10 mL of CH3CN solvent was added to the reaction mixture and stirred at room temperature for 20 minutes. The reaction was followed by TLC technique and after completion of the reaction, the reagent was separated by filtration. The reaction solvent was evaporated. The mixture was extracted with ethyl acetate/water. The organic layer was dried over anhydrous sodium sulfate, filtered and the solvent was evaporated. The residue was purified by column chromatography (silica gel, n-hexane: ethyl acetate 6/4) to give product.
2,2-Bis((nitrooxy)methyl)propane-1,3-diyl dinitrate
White solid; Mp (°C): 142–145; FT-IR (KBr, cm−1): 3029, 2983, 1646, 1271, 868. 1H NMR (600 MHz, DMSO) δ: 4.70 (s, 8H). 13C NMR (101 MHz, DMSO) δ: 70.1, 40.6.
1,3,5-Trinitro-1,3,5-triazinane
White solid; Mp (°C): 203–205; FT-IR (KBr, cm−1): 3074, 3065, 3001, 1573. 1H NMR (600 MHz, DMSO) δ: 6.11 (t, J = 4.5 Hz, 6H). 13C NMR (101 MHz, DMSO) δ: 61.1.
2,2-Dinitroethene-1,1-diamine
Yellow solid; Mp (°C): 235–238; FT-IR (KBr, cm−1): 3424, 3332, 3300, 1637, 1521. 1H NMR (600 MHz, DMSO) δ: 9.00 (s, 2H), 8.58 (s, 2H). 13C NMR (101 MHz, DMSO) δ: 158.0, 127.7.
2-Methyl-1,3,5-trinitrobenzene
Brown solid; Mp (°C): 83–85; FT-IR (KBr, cm−1): 3096, 1602, 1617, 1538. 1H NMR (600 MHz, DMSO) δ: 9.03 (s, 2H), 2.57 (s, 3H). 13C NMR (101 MHz, DMSO) δ: 150.7, 145.6, 132.9, 122.5, 14.8.
Results and discussion
As above said, during three decades our investigations on the field of nitration of organic compounds,35–43 herein we report poly(vinyl imidazole) sulfonic acid nitrate [PVI-SO3H]NO3 as a novel polymeric nitrating agent for preparation of four energetic materials (Scheme 3). After synthesis of described [PVI-SO3H]NO3 its structure was fully characterized by FT-IR, energy dispersive X-ray spectroscopy (EDX), SEM-elemental mapping, scanning electron microscope (SEM), thermal gravimetric (TG) and derivative thermal gravimetric (DTG). Then, [PVI-SO3H]NO3 was applied for the preparation of energetic materials such as 1,1-diamino-2,2-dinitroethene (FOX-7), pentaerythritol tetranitrate (PETN), 1,3,5-trinitro-1,3,5-triazinane (RDX) and trinitrotoluene (TNT) (Scheme 2).
The comparison FT-IR spectrum of [PVI-SO3H]NO3 and [PVI-SO3H]Cl were showed in (Fig. S1 see in ESI†). As it is shown in Fig. S1,† a broad peak was displayed at about 2400–3500 cm−1 related to OH of SO3H groups. The peak at about 1376 cm−1 is corresponded to asymmetric and symmetric vibration of O–N
O. Also, the peak at about 1722 cm−1 was attributed to C
O group. Furthermore, peaks at about 1234 and 1239 cm−1 were corresponded to N–SO2 and O–SO2 bonds respectively.
The structure of the [PVI-SO3H]NO3 was also approved by SEM-EDS analysis (Fig. S2 see in ESI†). The existence of C, O, N and S atoms was verified the structure of the described nitrating agent [PVI-SO3H]NO3. Then, SEM-elemental mapping (C, O, N and S) in the reagent with a well-dispersed over the reagent surface was confirmed (Fig. S2 see in ESI†).
The scanning electron microscopy (SEM) micrographs of [PVI-SO3H]NO3 was shown in Fig. 1. As can be seen from SEM micrographs, the morphology of reagent indicating a complete surface morphology change caused by adding of HNO3 to [PVI-SO3H]Cl.46 However, the shape of particles is cauliflower and nano-scales.
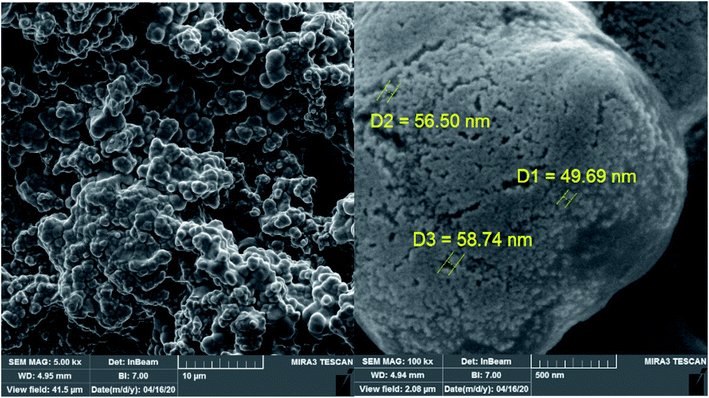 |
| Fig. 1 Scanning electron microscope (SEM) images of [PVI-SO3H]NO3. | |
In another investigation, structural and thermal stability of [PVI-SO3H]NO3 was also determined using thermal gravimetric (TG), derivative thermal gravimetric (DTG), as well as the differential thermal analysis (DTA) (Fig. 2). Initial stage weight losing is about 100 °C, associated with the removal of possible solvents (organic and water) which was used in the course of reagent preparation. Then, the loss of weight of the reagent at 160 °C was related to release of NO2. Also, another step of weight losing was occurred about 300 °C which is the onset of the structural degradation of [PVI-SO3H]NO3.
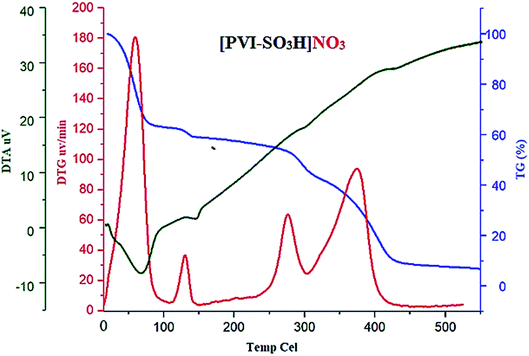 |
| Fig. 2 Thermal gravimetric (TG), derivative thermal gravimetric (DTG), as well as the differential thermal analysis (DTA) of [PVI-SO3H]NO3. | |
After the synthesis and full characterization of [PVI-SO3H]NO3 it was applied for the preparation of high-energetic materials such as FOX-7 (1,1-diamino-2,2-dinitroethene), pentaerythritol tetranitrate (PETN), 1,3,5-trinitro-1,3,5-triazinane (RDX) and trinitrotoluene (TNT). The mentioned nitro materials derivatives were synthesized via a nitration reaction of the corresponding starting materials (pentaerythritol, 1-methyl-4-nitrobenzene, 2-methylpyrimidine-4,6(1H,5H)-dione, hexamethylenetetramine) in the presence of [PVI-SO3H]NO3. The nitration of starting materials (1 mmol) and [PVI-SO3H]NO3 as a nitrating agent was optimized. As shown in Table 1, the worthy results were obtained when the reaction was achieved in CH3CN as solvent (Table 1, entry 11). No improvement was detected in the yield of reaction using different solvent and temperature (Table 1, entry 1–10). The results are summarized in Table 1. As shown in Table 1, no improvement the best yield for the synthesis of high-energetic materials were obtained using other molar ratios of [PVI-SO3H]NO3 at room temperature or refluxing CH3CN (Table 1, entry 12).
Table 1 Effect of different temperature and solvent on the synthesis of explosive materials
Entry |
Solvent |
Temp. (°C) |
Amount of reagent (g) |
Time (min) |
Yield (%) |
PETN |
RDX |
FOX-7 |
TNT |
1 |
EtOH |
Reflux |
1.9 |
120 |
— |
— |
— |
— |
2 |
MeOH |
Reflux |
1.9 |
120 |
— |
— |
— |
— |
3 |
n-Hexane |
Reflux |
1.9 |
120 |
— |
— |
— |
— |
4 |
Acetone |
Reflux |
1.9 |
120 |
28 |
34 |
— |
17 |
5 |
CHCl3 |
Reflux |
1.9 |
120 |
32 |
38 |
28 |
30 |
6 |
H2O |
Reflux |
1.9 |
120 |
— |
— |
— |
— |
7 |
DMF |
100 |
1.9 |
120 |
— |
15 |
40 |
38 |
8 |
CH2Cl2 |
Reflux |
1.9 |
120 |
45 |
50 |
15 |
35 |
9 |
EtOAc |
Reflux |
1.9 |
120 |
— |
— |
— |
— |
10 |
Toluene |
Reflux |
1.9 |
120 |
15 |
— |
— |
32 |
11 |
CH3CN |
25 |
1.9 |
30 |
85 |
— |
— |
— |
Reflux |
1.9 |
60 |
— |
— |
82 |
— |
25 |
1.9 |
20 |
— |
75 |
— |
— |
Reflux |
1.9 |
45 |
— |
— |
— |
70 |
12 |
CH3CN |
— |
1.5 |
— |
62 |
58 |
72 |
62 |
1.3 |
— |
48 |
43 |
38 |
40 |
1 |
— |
— |
— |
— |
— |
0.5 |
— |
— |
— |
— |
— |
— |
— |
— |
— |
— |
— |
CAUTION: During the synthesis of high-energetic materials the temperature of the reaction should be slowly increased and on workup, shock, heat, friction, and impact on the product must be avoided.
After the optimization of the reaction conditions (Table 1), the best result for the synthesis of explosive materials such as 1,1-diamino-2,2-dinitroethene (FOX-7), pentaerythritol tetranitrate (PETN), 1,3,5-trinitro-1,3,5-triazinane (RDX) and trinitrotoluene (TNT) were obtained using [PVI-SO3H]NO3 1.9 g at room temperature and refluxing CH3CN (Table 2).
Table 2 Synthesis of 1,1-diamino-2,2-dinitroethene (FOX-7), pentaerythritol tetranitrate (PETN), 1,3,5-triazinane (RDX) and trinitrotoluene (TNT) by [PVI-SO3H]NO3
Plausible mechanisms for the preparation of explosive materials were show in Scheme 4 here and Schemes S1–S3 in the ESI.† At first, starting materials (pentaerythritol, 1-methyl-4-nitrobenzene, 2-methylpyrimidine-4,6(1H,5H)-dione, hexamethylenetetramine) reacts with the in situ generated NO2 radical released from [PVI-SO3H]NO3 to produce intermediate I and nitrous acid (HNO2). Then, intermediate I reacts with the NO2 radical (released from [PVI-SO3H]NO3) to afford intermediate II. Then, these processes are repeated to obtain the final products.
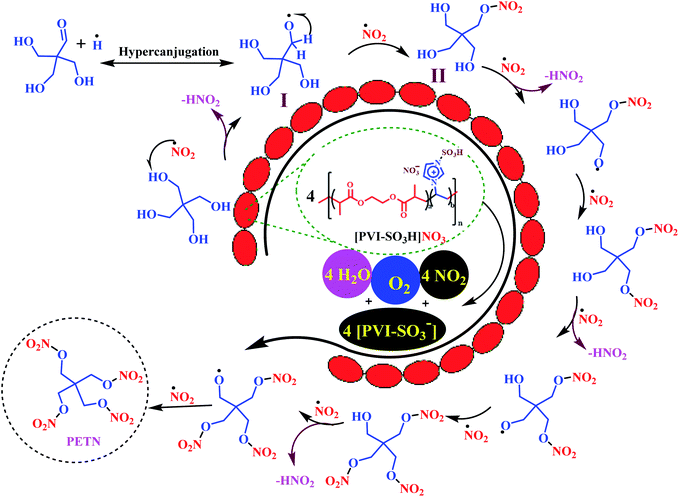 |
| Scheme 4 A plausible mechanism for the preparation of PETN. | |
To compare the performance of [PVI-SO3H]NO3 for the synthesis of desired nitro materials such as 1,1-diamino-2,2-dinitroethene (FOX-7), pentaerythritol tetranitrate (PETN), 1,3,5-trinitro-1,3,5-triazinane (RDX) and trinitrotoluene (TNT), we have used various organic and inorganic nitrating reagents. As Table 3 indicates, [PVI-SO3H]NO3 is the best of choice for the synthesis of explosive materials (Table 3).
Table 3 Comparison of the performance of [PVI-SO3H]NO3 for the synthesis of explosive materials with other nitrating reagents
Entry |
Nitrating system |
Solvent |
Time (min) |
Yield (%) |
PETN |
RDX |
FOX-7 |
TNT |
This work. |
1 |
HNO3/AIBN |
— |
120 |
52 |
43 |
32 |
28 |
2 |
Cr(NO3)3. 6H2O/HNO3 |
— |
120 |
20 |
— |
Trace |
— |
3 |
Cr(NO3)3·6H2O |
CH3CN |
120 |
— |
Trace |
— |
Trace |
4 |
Cu(NO3)2·3H2O |
CH3CN |
120 |
— |
— |
— |
— |
5 |
Fe(NO3)3·9H2O |
CH3CN |
120 |
Trace |
Trace |
— |
— |
6 |
SSA/NaNO3 (ref. 47) |
CH3CN |
120 |
— |
20 |
25 |
— |
7 |
HNO3 |
— |
120 |
38 |
32 |
18 |
27 |
8 |
SSA/HNO3 (ref. 47) |
— |
120 |
Trace |
22 |
— |
20 |
9 |
[Msim]NO3 |
— |
120 |
56 |
60 |
48 |
40 |
10 |
Fe(NO3)3·9H2O/FeCl3 |
CH3CN |
120 |
Trace |
— |
— |
— |
11 |
Ce(HSO4)3·7H2O/HNO3 |
— |
120 |
28 |
15 |
Trace |
20 |
12a |
[PVI-SO3H]NOa |
CH3CN |
30 |
85 |
— |
— |
— |
60 |
— |
— |
82 |
— |
20 |
— |
75 |
— |
|
45 |
— |
— |
— |
70 |
Conclusion
We have prepared poly(vinyl imidazole) sulfonic acid nitrate [PVI-SO3H]NO3 as a novel polymeric nitrating agent for preparation of four energetic materials. [PVI-SO3H]NO3 successfully used for the preparation of FOX-7 (1,1-diamino-2,2-dinitroethene), pentaerythritol tetranitrate (PETN), 1,3,5-trinitro-1,3,5-triazinane (RDX) and trinitrotoluene (TNT). Also, major advantages of the described methodology are high yield of products without using any co-catalysts, low temperature, short reaction times and generality for the preparation of energetic materials.
Conflicts of interest
There are no conflicts to declare.
Acknowledgements
We thank the Bu-Ali Sina University and Iran National Science Foundation ((INSF), Grant Number: 98001912) for financial support to our research group.
References
- A. K. Sikder and N. Sikder, J. Hazard. Mater., 2004, 112, 1 CrossRef CAS
. - S. Elbasuney, Y. H. El-Sharkawy, G. S. El-Sayyad and M. Gobara, Talanta, 2020, 211, 120695 CrossRef CAS
. - G. Yan and M. Yang, Org. Biomol. Chem., 2013, 11, 2554 RSC
. - D. M. Badgujar, M. B. Talawar and P. P. Mahulikar, Propellants, Explos., Pyrotech., 2016, 41, 24 CrossRef CAS
. - P. F. Pagoria, G. S. Lee, A. R. Mitchell and R. D. Schmidt, Thermochim. Acta, 2002, 384, 187 CrossRef CAS
. - J. S. Lee, C. K. Hsu and C. L. Chang, Thermochim. Acta, 2002, 392, 173 CrossRef
. - Z. A. Alothman, A. H. Bahkali, A. M. Elgorban, M. S. Al-Otaibi, A. A. Ghfar, S. A. Gabr and A. Y. B. H. Ahmed, Molecules, 2020, 25, 1393 CrossRef CAS
. - P. F. Pagoria, G. S. Lee, A. R. Mitchell and R. D. Schmidt, Thermochim. Acta, 2002, 384, 187 CrossRef CAS
. - D. M. Badgujar, M. B. Talawar, S. N. Asthana and P. P. Mahulikar, J. Hazard. Mater., 2008, 151, 289 CrossRef CAS
. - D. Olender, J. Zwawiak and L. Zaprutko, J. Pharm., 2018, 11, 54 Search PubMed
. - T. M. Klapotke, C. Petermayer, D. G. Piercey and J. Stierstorfer, J. Am. Chem. Soc., 2012, 134, 20827 CrossRef
. - K. Zhang, B. Jelier, A. Passera, G. Jeschke and D. Katayev, Chem. - Eur. J., 2019, 25, 12929 CrossRef CAS
. - H. B. Sun, R. Hua and Y. Yin, J. Org. Chem., 2005, 70, 9071 CrossRef CAS
. - G. S. Prakash, C. Panja, T. Mathew, V. Surampudi, N. A. Petasis and G. A. Olah, Org. Lett., 2004, 6, 2205 CrossRef CAS
. - L. Lu, H. Liu and R. Hua, Org. Lett., 2018, 20, 3197 CrossRef CAS
. - R. Calvo, K. Zhang, A. Passera and D. Katayev, Nat. Commun., 2019, 10, 1 CrossRef CAS
. - K. Zhang, A. Budinská, A. Passera and D. Katayev, Org. Lett., 2020, 22, 2714 CrossRef CAS
. - U. Kloeckner and B. J. Nachtsheim, Chem. Commun., 2014, 50, 10485 RSC
. - R. C. Dorey and W. R. Carper, J. Chem. Eng. Data, 1984, 29, 93 CrossRef CAS
. - M. Kroger and G. Fels, J. Intern. Isot. Soc., 2000, 43, 217 CAS
. - L. F. Fieser, R. C. Clapp and W. H. Daudt, J. Am. Chem. Soc., 1942, 64, 2052 CrossRef CAS
. - K. Lange, A. Koenig, C. Roegler, A. Seeling and J. Lehmann, Bioorg. Med. Chem. Lett., 2009, 19, 3141 CrossRef CAS
. - B. S. Fedorov and L. T. Eremenko, Russ. Chem. Bull., 1997, 46, 1022 CrossRef CAS
. - G. M. Blackburn, I. G. Beadham, H. Adams, A. P. Hutchinson and S. Nicklin, J. Chem. Soc., Perkin Trans. 2, 2000, 2, 225 RSC
. - D. Fischer, T. M. Klapotke and J. Z. Stierstorfer, Z. Anorg. Allg. Chem., 2011, 637, 660 CrossRef CAS
. - L. Shi, M. Yu, Y. Zhang, G. Qin, J. Lu and G. Zhao, Chin. J. Chem., 2011, 29, 283 CrossRef CAS
. - A. A. Astrat'ev, D. V. Dashko, A. Y. Mershin, A. I. Stepanov and N. A. Urazgil'deev, Russ. J. Org. Chem., 2001, 37, 729 CrossRef
. - P. Gilinsky-Sharon, H. E. Gottlieb, D. E. Rajsfus, K. Keinan-Adamsky, V. Marks, P. Aped and A. A. Frimer, Magn. Reson. Chem., 2012, 50, 672 CrossRef CAS
. - J. Zhang, J. Zhang, D. A. Parrish and M. S. Jean'ne, J. Mater. Chem., 2018, 6, 22705 RSC
. - H. Huang, Y. Shi, Y. Yu and J. Yang, Eur. J. Org. Chem., 2018, 2018, 113 CrossRef CAS
. - G. S. Prakash, L. Gurung, K. E. Glinton, K. Belligund, T. Mathew and G. A. Olah, Green Chem., 2015, 17, 3446 RSC
. - M. A. K. Zarchi and A. Zarei, J. Chin. Chem. Soc., 2005, 52, 309 CrossRef CAS
. - A. F. Tarchoun, D. Trache, T. M. Klapötke and B. Krumm, Fuel, 2020, 277, 118258 CrossRef CAS
. - H. Goudarziafshar, Chin. Chem. Lett., 2012, 23, 458 CrossRef CAS
. - A. R. Moosavi-Zare, M. A. Zolfigol, M. Zarei, A. Zare, V. Khakyzadeh and A. Hasaninejad, Appl. Catal., A, 2013, 467, 61 CrossRef CAS
. - A. R. Moosavi-Zare, M. A. Zolfigol, M. Zarei, A. Zare and J. Afsar, Appl. Catal., A, 2015, 505, 224 CrossRef CAS
. - A. R. Moosavi-Zare, M. A. Zolfigol, M. Zarei, A. Zare and V. Khakyzadeh, J. Mol. Liq., 2015, 211, 373 CrossRef CAS
. - M. Rajabi-salek, M. A. Zolfigol, M. Zarei, E. Noroozizadeh, I. Mohammadpoor-Baltork and H. A. Rudbari, ChemistrySelect, 2018, 3, 12791 CrossRef CAS
. - M. Zarei, H. Sepehrmansourie, M. A. Zolfigol, R. Karamian and S. H. M. Farida, New J. Chem., 2018, 42, 14308 RSC
. - M. A. Zolfigol, A. Khazaei, A. R. Moosavi-Zare, A. Zare, H. G. Kruger, Z. Asgari and M. Kazem-Rostami, J. Org. Chem., 2012, 77, 3640 CrossRef CAS
. - H. Ghaderi, M. A. Zolfigol, Y. Bayat, M. Zarei and E. Noroozizadeh, Synlett, 2016, 27, 2246 CrossRef CAS
. - M. Zarei, E. Noroozizadeh, A. R. Moosavi-Zare and M. A. Zolfigol, J. Org. Chem., 2018, 83, 3645 CrossRef CAS
. - A. R. Moosavi-Zare, M. A. Zolfigol, M. Zarei, E. Noroozizadeh and M. H. Beyzavi, RSC Adv., 2016, 6, 89572 RSC
. - B. Osman, A. Kara, L. Uzun, N. Beşirli and A. Denizli, J. Mol. Catal. B: Enzym., 2005, 37, 88 CrossRef CAS
. - A. Kara, L. Uzun, N. Beşirli and A. Denizli, J. Hazard. Mater., 2004, 106, 93 CrossRef CAS
. - H. Sepehrmansourie, M. Zarei, R. Taghavi and M. A. Zolfigol, ACS Omega, 2019, 4, 17379 CrossRef CAS
. -
(a) M. A. Zolfigol, Tetrahedron, 2001, 57, 9509 CrossRef CAS
;
(b) H. Sepehrmansourie, Iran. J. Catal., 2020, 10, 175 CAS
.
Footnote |
† Electronic supplementary information (ESI) available. See DOI: 10.1039/d1ra00651g |
|
This journal is © The Royal Society of Chemistry 2021 |
Click here to see how this site uses Cookies. View our privacy policy here.