DOI:
10.1039/D1RA00798J
(Paper)
RSC Adv., 2021,
11, 10738-10746
Solvent-free synthesis of phytosterol linoleic acid esters at low temperature
Received
30th January 2021
, Accepted 5th March 2021
First published on 12th March 2021
Abstract
Phytosterol unsaturated fatty acid esters show much higher oil solubility than free phytosterol. Thus, development of a green and low-cost method for the preparation of phytosterol fatty acid esters is highly desirable in the food industry. Herein, we have developed a simple chemical method toward efficient preparation of phytosterol linoleic acid esters at very mild temperature (60 °C) using 4-dodecylbenzenesulfonic acid (DBSA) as the catalyst. In this work, low-temperature esterification of phytosterols (soybean sterol) with linoleic acid could produce the corresponding phytosterol esters above 95% conversion under solvent-free conditions. In addition, this simple method could be applied to produce phytosterol esters through esterification of phytosterol with an unsaturated fatty acid mixture resulting from the hydrolysis of various vegetable oils. Importantly, no extra organic solvents and no extra water-removal operations or equipment were required in this chemical esterification method. The mechanism investigation suggested that the DBSA-catalyzed low-temperature esterification would form micro-emulsions of water-in-oil (W/O), which could achieve automatic separation of water from the hydrophobic system to avoid reverse reaction hydrolysis and rapidly promote the equilibrium reaction towards phytosterol esters.
1. Introduction
Phytosterols have attracted increasing attention due to their excellent cholesterol-lowering, anti-inflammatory, anti-cancer, antioxidant and cardiovascular-protective activity.1–4 A study reported that a daily intake of 1.5–3 g of phytosterol could decrease low density lipoprotein cholesterol (LDL-C) by 8–15%.5 Although they have potential applications, the bioavailability of phytosterols is greatly limited by their high melting points (>135 °C) and low oil solubility.6 Esterification of phytosterols with fatty acids is one of the most efficient methods to improve the solubility of phytosterols.1,7 Previous studies have identified that the esterified phytosterols can effectively decrease LDL-C levels in a similar manner as the free phytosterols, which suggests phytosterol and its esters can be used as novel food ingredients.5,8
The esterification of phytosterol and fatty acids can be catalyzed by chemo-catalysts or enzyme.1 Up to now, chemical catalysis is still the main method of industrial production of phytosterol esters. Acid catalysts, including homogeneous and heterogeneous acid catalysts, were commonly used in chemical catalysis toward synthesis of phytosterol esters.9 The homogeneous acid catalysts, (e.g., sulfuric acid, p-toluenesulfonic acid and sodium bisulphate) were low cost and high efficiency,10,11 but were difficult to separation and easy corrosion of equipment. Considering these disadvantages of homogeneous acid catalysts, heterogeneous acid catalysts, including immobilized heteropolyacid and acidic ionic liquids, have been also applied in esterification of phytosterols.11–13 However, acid catalyst-promoted esterification of phytosterol with fatty acid generally requires harsh reaction temperature (usually 130–180 °C), which easily lead to side reactions (e.g., dehydration of phytosterols).11,12 Moreover, acid catalyst-promoted esterification of phytosterols with fatty acid is more suitable for saturated fatty acids (e.g., palmitic acid, stearic acid) due to their better stability under high heating temperature. However, the unsaturated fatty acids, especially the polyunsaturated fatty acids (e.g., linoleic acid, linolenic acid) will be easily oxidized at high heating temperature and have lower esterification activity.1 In recent years, the enzyme-catalyzed synthesis of phytosterol esters has attracted increasing attention as these methods have advantages of having mild and environmentally friendly reaction conditions and fewer side products.1,14–20 However, its high cost and low reliability have great limit its large-scale industrial applications.1
Previous studies have indicated that the solubility of phytosterol unsaturated fatty acid esters in edible oils (e.g., soybean oil) was up to 10 times than phytosterol saturated fatty acid esters.17,21 Meanwhile, polyunsaturated fatty acids, especially linoleic acid and linolenic acid, are essential fatty acids, which are more important for human nutrition than saturated fatty acids (e.g., stearic acid). Thus, developing an efficient and inexpensive acid-catalysis method to synthesize phytosterol unsaturated fatty acid (e.g., linoleic acid) esters at mild reaction temperatures (<70 °C) is highly desirable to meet the demand for commercial production of phytosterol esters in food industry. An early report has shown an interesting “brønsted acid-surfactant-combined catalysis” that 4-dodecylbenzenesulfonic acid (DBSA) could catalyze esterification of lauric acid with 3-phenyl-1-propanol in water.22 Inspired by the concept of “brønsted acid-surfactant-combined catalyst” in biphasic reaction systems,22–26 we envisage that DBSA can also promote the esterification of phytosterols with unsaturated fatty acids (e.g., linoleic acid) under solvent-free conditions.
With the aim to develop green and low-cost method for the synthesis of phytosterol unsaturated fatty acid esters at mild temperature, herein we demonstrated that DBSA-catalyzed esterification of phytosterols with linoleic acid can take place efficiently at 60 °C under solvent-free conditions with high phytosterols conversion (>95%). In addition, the low-temperature esterification mechanism catalyzed by DBSA has been also studied.
2. Materials and methods
2.1 Materials
Phytosterols (soybean sterol, 95%) composed by 25% stigmasterol, 45% β-sitosterol and 30% campesterol, were purchased from Wuhan Yuancheng Creation Technology Co., Ltd. (Wuhan, China). Linoleic acid (98%) and 4-dodecylbenzenesulfonic acid (95%) were purchased from Sigma-Aldrich Chemical Co. Ltd (Shanghai, China). Linolenic acid (>70%) was purchased from TCI Chemical Co. Ltd (Shanghai, China). Soybean oil (SBO), sunflower oil (SFO) and camellia oil (CO) were purchased from a local supermarket. The fatty acid mixture from SBO, SFO and CO, containing only mixed free fatty acids, were prepared by basic hydrolysis with potassium hydroxide (KOH), respectively. All other chemicals were purchased from Macklin Inc. (Shanghai, China).
2.2 Esterification of phytosterols with linoleic acid
Phytosterols (soybean sterol), linoleic acid, DBSA and a magnetic stirrer were successively added into a reaction tube. Then the resulting mixture was heated to the specified temperature (40–80 °C) under a nitrogen atmosphere. After a certain reaction time (0–24 h), the mixture was quenched with a mixture solution of saturated aqueous NaHCO3 and brine (1
:
1, v/v). The phytosterol esters were obtained by extracting with n-hexane three times.
2.3 Analysis of conversion of phytosterols by GC
The compositions of crude products were analyzed by GC. An Agilent 7890 gas chromatograph (Hewlett-Packard Co., Avondale, PA, USA), equipped with a flame ionization detector (FID) and a fused silica capillary column (DB-1 HT, 30.0 m × 0.25 mm × 0.1 μm) was used. Nitrogen was used as carrier gas with a flow of 4.0 mL min−1. The oven temperature was programmed as follows: elevated from 100 °C to 220 °C at 50 °C min−1 and held for 2 min; increased to 290 °C at a rate of 15 °C min−1 and held for 2 min; increased to 320 °C at a rate of 40 °C min−1 and held for 6 min; increased to 360 °C at a rate of 20 °C min−1; held at 360 °C for another 5 min. Injection volume and the split ratio were1 μL and 5
:
1, respectively. The GC injection and detector temperature were both set at 350 °C.
Conversion of phytosterols to phytosterol esters (%) = [(the molar amount of phytosterols at the beginning of reaction – the molar amount of phytosterols at the end of reaction)/the molar amount of phytosterols at the beginning of reaction] × 100%.16,17
2.4 Purification of phytosterol esters by column chromatography
The phytosterol fatty acid esters (phytosterol linoleate) catalyzed by DBSA was purified with a silica gel column [100–200 mesh, eluted with n-hexane: diethyl ether: acetic acid (80
:
20
:
2, v/v/v)]. The fractions containing the desired products were collected and evaporated under reduced pressure. The obtained pure products were stored at −10 °C for GC analysis.
2.5 The FTIR analysis of pure phytosterol esters
FTIR analysis (BRUKER ALPHA II, scanning scope: 400–4000 cm−1, number of scans: 32) of the product (phytosterol esters) was performed as described previously.27
2.6 Confocal laser scanning microscopy (CLSM) analysis
Microstructures of reaction mixture were visualized by confocal laser scanning microscopy (CLSM) using FV3000 (Japan Olympus investment co., Ltd, Japan). The reaction mixture was dyed with 0.01% Nile red (Sigma, USA). The phytosterols (soybean sterol) and water were black. In order to better judge the distribution of hydrophobic phase and water in the reaction mixture, a water/linoleic acid/DBSA model mixture was prepared. 1.016 g of linoleic acid, 0.0788 g of DBSA, and 20–40% of water were added to the test tube and vortexed. 40 μl of the dye solution was added into 1 mL of reaction mixture and mixed well before slide preparation, which was ready for CLSM analysis.
3. Results and discussion
3.1 Catalytic activity of DBSA
Catalysts are key factors in the esterification reactions. One of the advantages of using an acid catalyst in the synthesis of phytosterol esters from fatty acids or oils is the no formation of soaps. Sulfuric acid (H2SO4) and p-toluenesulfonic acid (PTSA) are the commonly used catalysts in esterification and transesterification reactions.11 In addition, sodium bisulfate (NaHSO4) has been also reported as the acid catalyst in the synthesis of phytosterol esters at 130 °C.10 Since linoleic acid is the most common polyunsaturated fatty acid derived from plant oils, a comparative study was conducted to evaluate the catalytic activity of different acid catalysts (H2SO4, PTSA, NaHSO4 and DBSA) in the esterification of phytosterols with linoleic acid under the same conditions (solvent-free, 60 °C, 10 mol% of catalyst, 1
:
3 molar ratio of phytosterols to linoleic acid) (Fig. 1). The results showed that H2SO4, PTSA, and NaHSO4 were not active at 60 °C towards the esterification after 24 h (the conversions of phytosterols all less than 20%). When DBSA was used as an acid catalyst in the esterification reaction at 60 °C, the phytosterol conversion reached to 84% in 24 h. According to the study on the initial rates of the esterification in the presence of different acid catalysts at 60 °C (Table 1), DBSA was found to catalyze the esterification reaction 14–37 times faster than other three common acid catalysts (H2SO4, PTSA, NaHSO4). These results clearly demonstrated that the acidity of brønsted acid was not the key factor in this esterification reaction of phytosterol with linoleic acid. Indeed, DBSA has a good catalytic activity on the synthesis of phytosterol linoleic acid esters at such mild temperature (60 °C). Based on the above results, DBSA was selected as the optimal acid catalyst in the following experiments.
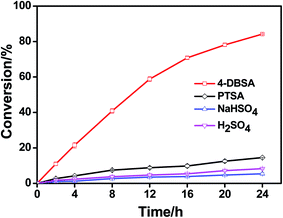 |
| Fig. 1 Conversion of phytosterols catalyzed by different acid catalysts. Reaction conditions: solvent-free, 10% catalyst (mol%), 60 °C, 1 : 3 molar ratio of phytosterols (soybean sterol) to linoleic acid. | |
Table 1 Initial rate of esterification of phytosterols catalyzed by acid catalysts
Catalyst |
PTSA |
NaHSO4 |
H2SO4 |
DBSA |
Initial rates (M.h−1) |
0.63 |
0.24 |
0.36 |
8.96 |
3.2 Effect of catalyst loading on the esterification of phytosterols
The influence of catalyst loading on the esterification of phytosterols was evaluated using different amount of DBSA from 10% to 25% (mol/mol) and the results were presented in Fig. 2(A). The esterification reaction was not occurred at 60 °C without DBSA as catalyst, suggesting that the acidity of the linoleic acid was not sufficient enough to auto-catalyze the esterification at such mild temperature. Previous studies indicated that auto-catalytic esterification of phytosterols with oleic acid could take place at 180–220 °C.28,29 Indeed, the esterification of phytosterols was accelerated when DBSA loading was increased from 10% to 25%. When the amount of catalyst was 10%, the phytosterol conversion could reach to 90% at 36 h. The same conversion could be achieved in 20 h and 12 h when the amount of catalyst was increased to 15% and 20%, respectively. Continuing increasing the DBSA loading further from 20% to 25%, the esterification reaction rate exhibited no remarkable rise. At the early stage of the esterification (4 h), the reaction using 20% catalyst led to higher conversion than that using 10–15% catalysts (Fig. 2(B)). Based on the above results, it could be calculated that the reaction order was 2. Thus, 20% DBSA was selected as the suitable catalyst amount for the following experiments.
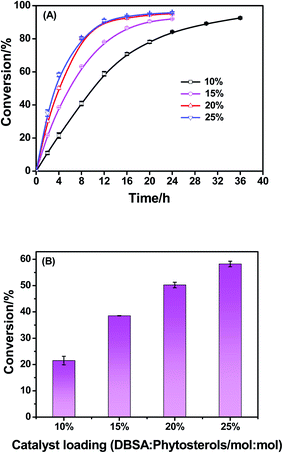 |
| Fig. 2 (A) Effect of DBSA loading (mol%) on the esterification of soybean sterol. (B) Effect of DBSA loading (mol%) on the esterification of soybean sterol (reaction time: 4 h). Reaction conditions: solvent-free, 60 °C, 1 : 3 molar ratio of soybean sterol to linoleic acid. | |
3.3 Effect of molar ratio of substrates on the esterification of phytosterols
The effect of the molar ratio of the substrates (phytosterols/linoleic acid) on the synthesis of phytosterol linoleic acid esters was investigated at 60 °C under solvent-free conditions (Fig. 3). The range of molar ratios of phytosterols (soybean sterol) to linoleic acid was between 1
:
1 (stoichiometric amounts) and 1
:
4 (3-fold excess). In general, the esterification reaction mixture had the high viscosity which caused by the high melting point of phytosterols (>135 °C). Thus, most of procedure for bio-catalysis or acid-catalysis of esterification of phytosterols requires the use of organic solvents (e.g., isooctane, n-hexane) or heating at higher temperature (usually 120–180 °C).1 Usually, a large excess of fatty acid is required to promote the esterification efficiency of phytosterols. As shown in Fig. 3, the initial reaction rate from the molar ratio of 1
:
1 to 1
:
4 were all similar. The maximal conversion increased with the increase of molar ratio from 1
:
1 to 1
:
3, the maximum conversion at a molar ratio of 1
:
3 was over 95.1% without adding extra organic solvents. There was no significant difference in both the initial reaction rate and the maximum phytosterol conversion when the molar ratio was further increased to 1
:
4. Therefore, the molar ratio of 1
:
3 (soybean sterol/linoleic acid) was selected for the following optimization experiments.
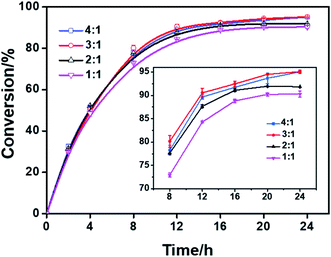 |
| Fig. 3 Effect of molar ratio of soybean sterol to linoleic acid on the esterification. Reaction conditions: solvent-free, 20% DBSA (mol%), 60 °C. | |
3.4 Effect of water on the esterification of phytosterols
Water is one of the products of esterification reaction. Generally speaking, the existence of water in starting materials or products affects the equilibrium of the esterification reaction of phytosterols, and even promotes the hydrolysis of phytosterol esters. Thus, the influence of water content (0–40%, related to the mass of phytosterols) on the esterification reaction was investigated (Fig. 4). When a small amount of water (20%) was added in the reaction mixture, the reaction rate and the equilibrium position was unchanged. With the water content further increasing (30–40%), the esterification reaction rate was reduced slightly. It was noteworthy that the equilibrium position of the reaction with different contents of water (0–40%) was similar when the reaction time extended to around 48 h. This phenomenon was similar to the results reported by Manabe et al.22 In their report involving DBSA-catalyzed esterification of lauric acid, it was found that the reaction under neat conditions was faster than that in water and the yield of the ester at the equilibrium position was unchanged. In our work, a small proportions of water (less than 20%) did not essentially alter the DBSA-catalyzed esterification reaction course, which was attributed to DBSA as “brønsted acid-surfactant-combined catalyst”.22 In previous studies, most of esterification reaction of phytosterols required to conduct under a water removal method conditions, such asazeotropic distillation, vacuum, and adding molecular sieves.1 Notably, even adding 20% water in our reaction mixture had no effect on the esterification rate and phytosterol conversion in the presence of DBSA at 60 °C. Thus, DBSA could be an excellent acid catalyst for the synthesis of phytosterol linoleic acid esters under such mild conditions. More importantly, the starting materials did not need to be dried before use.
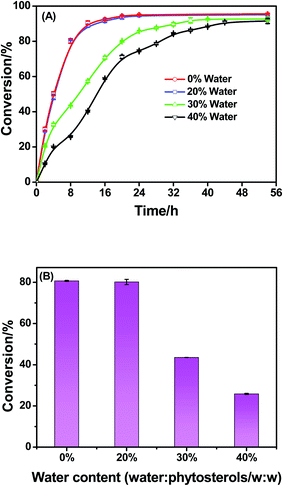 |
| Fig. 4 (A) Effect of water content on the esterification of soybean sterol. (B) Effect of water content on the esterification of soybean sterol (reaction time: 8 h). Reaction conditions: solvent-free, 20% DBSA (mol%), 60 °C, 1 : 3 molar ratio of soybean sterol to linoleic acid. | |
3.5 Effect of reaction temperature on the esterification of phytosterols
The temperature is a very important parameter in the esterification of phytosterols with fatty acids, and high reaction temperature (>130 °C) is required for most acid-catalyzed esterification of phytosterols. Therefore, the effect of temperature on the esterification reaction was investigated in a range of 40 °C to 80 °C (Fig. 5). It could be observed that the DBSA-catalyzed esterification reaction was still active even at 40 °C. When the reaction temperature (40 °C to 50 °C) was lower than 60 °C, the esterification reaction rates were steady (the conversions were 89.5% at 40 °C for 46 h, 90.5% at 50 °C for 38 h, respectively). Though the reaction rates at 70 °C and 80 °C were both higher than 60 °C, there was no significant difference in their conversions at equilibrium. In addition, the side reactions of phytosterols and linoleic acid (such as dehydration of phytosterols, oxidation of linoleic acid and isomerization of linoleic acid) would increase at elevated temperature. Indeed, DBSA as “brønsted acid-surfactant-combined catalyst” was efficient at very mild temperature. In our work, the activation energy (Ea) was 71.64 kJ mol−1, which was slightly higher than the Ea (58.5–63.4 kJ mol−1) of DBSA-catalyzed esterification of oleic acid with methanol.30 In fact, esterification of phytosterols is harder than the esterification of methanol. Therefore, 60 °C was suitable as the optimum esterification temperature.
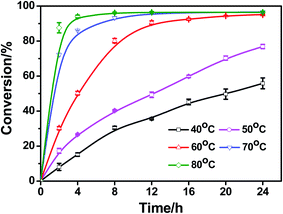 |
| Fig. 5 Effect of reaction temperature on the esterification of soybean sterol. Reaction conditions: solvent-free, 20% DBSA (mol%), 1 : 3 molar ratio of soybean sterol to linoleic acid. | |
3.6 Esterified product analysis with GC and FTIR
GC analysis was employed to calculate the conversion of phytosterols (soybean sterol) and determine the purity of phytosterol fatty acid esters.16,17 As shown in Fig. 6, reaction substrates (soybean sterol, linoleic acid) and esterification products (soybean sterol linoleic acid esters) could be clearly distinguished by GC method. The peaks of phytosterols (soybean sterol) after esterification reaction mixture almost disappeared, while a new group of peak of phytosterol linoleic acid esters appeared.
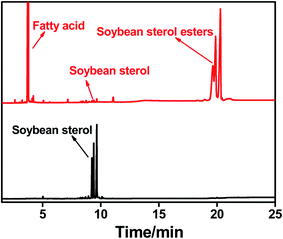 |
| Fig. 6 Gas chromatograms of esterification reaction of soybean sterol with linoleic acid. | |
FTIR spectrums of starting materials (soybean sterol, linoleic acid) and esterification product (soybean sterol esters) were presented in Fig. 7. In Fig. 7(a), the peak at 3008 cm−1 was the signal of C–H in –C
C–H, while the strong peak at 1711 cm−1 was the signal of C
O in COOH. The weak peak at 724 cm−1 indicated that the presence of four or more CH2 groups. In Fig. 7(b), the broad peak with strong absorption at 3420 cm−1 indicated the presence of –OH stretching vibration, while the peak at 1508 cm−1 was the stretch vibration signal of C–O. In Fig. 7(c), the peak at 3008 cm−1 was the signal of C–H in –C
C–H. The strong peaks at 1734 cm−1 and 1171 cm−1 were the characteristic absorption signal of C
O and C–O in –CO–OR. The weak peak at 727 cm−1 indicated the presence of four or more CH2 groups in the carbon chain. The disappearance of carboxyl and hydroxyl signals and the appearance of ester bonds indicated that the new product was soybean sterol linoleate.
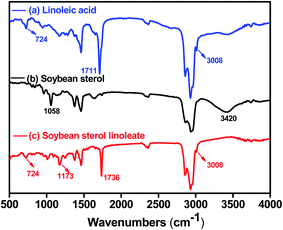 |
| Fig. 7 FTIR spectra of linoleic acid (a), soybean sterol (b) and soybean sterol linoleate (c). | |
3.7 Mechanism of phytosterols esterification catalyzed by DBSA
Manabe et al. previously investigated the esterification of lauric acid with 3-phenyl-1-propanol in the presence of DBSA in water.22 The formation of white turbid mixtures was observed in their reaction system, which was proved to be oil-in water (O/W) emulsions. In our work, the reaction mixture was almost clear despite the extra addition of water (20–40%). To further explore whether emulsions formed in our work, the reaction mixture was analyzed using confocal laser scan microscope (CLSM). As shown in Fig. 8, water droplets were surrounded by linoleic acid. Compared to the absence of acidic surfactant (DBSA), the water droplet diameter of the mixture with DBSA was several times smaller (Fig. 8(b and d)). Therefore, it could be concluded that the reaction mixture formed micro-emulsions of water-in-oil (W/O) in our study. In addition, the diameter of the water droplets increased as the amount of water added increases (Fig. 8(a–c)).
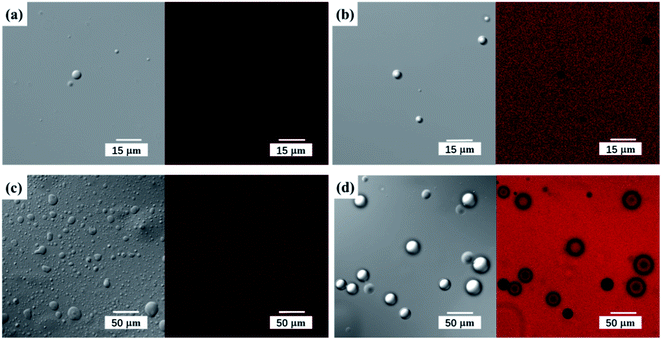 |
| Fig. 8 CLSM images of the linoleic acid/water/DBSA system. (a) Adding 5% water, (b) adding 20% water, (c) adding 40% water, (d) adding 20% water without DBSA. | |
The mechanism of low-temperature esterification catalyzed by DBSA is illustrated in Fig. 9. The hydrophobic tail and hydrophilic head in DBSA molecule make they form reverse micelles, which can separate water from hydrophobic substrates. Water molecules generated during the esterification reaction would automatically enter the hydrophilic interior phase, while the product phytosterol esters remained in the outer phase. Separation of water from the phytosterol esters avoids reverse reaction hydrolysis and rapidly promotes the equilibrium reaction towards ester product, which also indicated that the addition of small amount of extra water (20% related to the mass of phytosterols) had no effect on the esterification reaction rate and phytosterol conversion (Fig. 4). In addition, the formation of reverse micelles clusters would congregate catalytic species H+ at the reaction interface, which could catalyze the esterification more efficiently. Therefore, DBSA can achieve the efficient esterification of phytosterols with linoleic acid with high phytosterols conversion (95%) without using azeotropic distillation equipment or desiccant at such mild temperature (60 °C), which suggest that this low-temperature esterification method is suitable for a large-scale preparation of phytosterol esters.
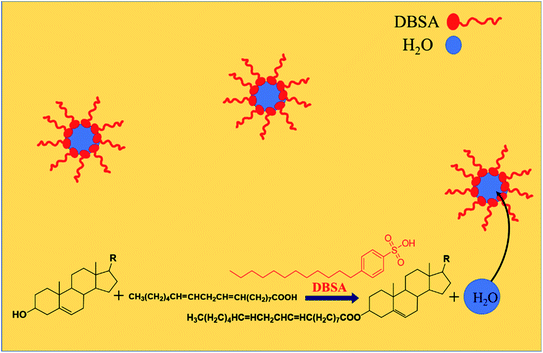 |
| Fig. 9 Illustration of auto-isolation of water produced during the esterification process. | |
3.8 Esterification of phytosterols with other unsaturated fatty acids
Under the optimized conditions (solvent-free, 60 °C, 1
:
3 molar ratio of soybean sterol/fatty acid), the catalytical effect of DBSA on the low-temperature esterification of soybean sterol with other unsaturated fatty acids was also investigated (Table 2). The esterification of phytosterols with monounsaturated fatty acids, such as oleic acid, could afford corresponding phytosterol oleic acid esters in a conversion of 96.3% at 60 °C. And the esterification of phytosterols with other polyunsaturated fatty acids, such as linolenic acid, could afford corresponding phytosterol esters in a conversion of 95.1%. Since unsaturated fatty acids (e.g., linoleic acid) with high purity are obtained from plant oils (e.g., camellia oil, safflower seeds oil), a fatty acid mixture resulting from the direct hydrolysis of commercial plant oils is also the ideal starting material of unsaturated fatty acids. From this perspective, low-cost fatty acid mixtures resulting from the hydrolysis of several commercial plant oils were used as unsaturated fatty acid donors in the esterification reaction. When DBSA was used as catalyst, the esterification of soybean sterol with different fatty acid mixtures resulting from the hydrolysis of soybean oil (SBO), sunflower oil (SFO) and camellia oil (CO) was also evaluated under solvent-free conditions (Table 2). Indeed, all tested fatty acid mixtures (rich in unsaturated fatty acids) took place esterification smoothly with soybean sterol and afforded the corresponding phytosterol esters above 95% conversions. Undoubtedly, it could be concluded that this low-temperature esterification of phytosterols with unsaturated fatty acids could produce different phytosterol esters in excellent phytosterol conversions (>95%), which provided an alternative for the synthesis of phytosterol esters in food industry.
Table 2 Esterification of phytosterols with different fatty acidsa
Fatty acid |
Conversion (%) |
Reaction conditions: 60 °C, 20% DBSA (mol%), 1 : 3 molar ratio of soybean sterol/fatty acid. SBO fatty acids was resulted from the hydrolysis of soybean oil. SFO fatty acids was resulted from the hydrolysis of sunflower oil. CO fatty acids was resulted from the hydrolysis of camellia oil. |
Linoleic acid |
95.1 ± 0.1 |
Oleic acid |
96.3 ± 1.0 |
Linolenic acid |
95.1 ± 0.7 |
SBO fatty acidsb |
95.3 ± 1.2 |
SFO fatty acidsc |
95.2 ± 1.3 |
CO fatty acidsd |
96.2 ± 0.9 |
3.9 Comparison with chemical methods used in other studies
Different chemical methods for esterification of phytosterols with unsaturated fatty acids (e.g., oleic acid) have been listed in Table 3. The homogeneous acid catalysts for esterification of phytosterols, such as sulfuric acid (H2SO4) and sodium bisulphate (NaHSO4) were low cost and high efficiency, but were easy corrosion of equipment and required high reaction temperature (130–170 °C).10,11 Considering the disadvantages of homogeneous acid catalysts, heterogeneous acid catalysts, including ZnO and immobilized heteropolyacid have been also applied in esterification of phytosterols.11,13 However, heterogeneous acid catalysts-promoted synthesis of phytosterol esters also required harsh reaction temperature (150–170 °C). In general, esterification of phytosterols at harsh reaction temperature easily lead to side reactions, including dehydration of phytosterols, oxidation of unsaturated fatty acids and isomerization of polyunsaturated fatty acids.11,12 In recent years, noncatalytic esterification of phytosterols with traditional heating has been reported for the synthesis of phytosterol esters, but harsh reaction temperature (220 °C) still required in the process.28 Moreover, microwave irradiation-mediated noncatalytic esterification could produce phytosterol esters at 180 °C.29 In our work, DBSA-catalyzed esterification of phytosterols with polyunsaturated fatty acid (e.g., linoleic acid) could afford corresponding phytosterol esters over 95% conversions at 60 °C. More importantly, no azeotropic distillation equipment or desiccant were required in the DBSA-catalyzed esterification process at such mild temperature, which suggests that this low-temperature esterification method is suitable for a large-scale preparation of phytosterol esters.
Table 3 Esterification of phytosterols with unsaturated fatty acid through different methods
Catalyst |
Fatty acid |
Reaction Temperature (oC) |
Conversion (%) |
References |
H2SO4 |
Oleic acid |
170 |
98 |
11 |
NaHSO4 |
Polyunsaturated fatty acid |
130 |
96 |
10 |
ZnO |
Oleic acid |
170 |
84.2 |
11 |
Tungstosilicic acid in silica gel |
Oleic acid |
150 |
90 |
13 |
Catalyst free (traditional heating) |
Oleic acid |
220 |
99.1 |
28 |
Catalyst free (microwave irradiation) |
Oleic acid |
180 |
95.4% |
29 |
DBSA |
Linoleic acid |
60 |
95.1 |
This study |
4. Conclusions
In conclusion, we have developed a simple chemical method toward efficient synthesis of phytosterol linoleic acid esters at mild temperature (60 °C) using DBSA as catalyst. In this work, low-temperature esterification of phytosterols (soybean sterol) with unsaturated fatty acids, including linoleic acid, oleic acid and linolenic acid could produce the corresponding phytosterol esters above 95% conversions under solvent-free conditions. The mechanism investigation suggested that the DBSA-catalyzed low-temperature esterification would form micro-emulsions of water-in-oil (W/O), which could achieve automatic separation water from the hydrophobic system to avoid reverse reaction hydrolysis and rapidly promote the equilibrium reaction towards phytosterol ester product. The merit of this developed chemical esterification method was low-temperature, solvent-free, high conversion and non-water removal method required.
Conflicts of interest
All authors have no conflict of interest to declare.
Acknowledgements
This work was supported by the Funds of National Natural Science Foundation of China (No. 31801501), the Key Scientific and Technological project in Henan Province (No. 212102110317) and China Postdoctoral Science Foundation funded project (No. 2018T110730).
References
- W. S. He, H. Zhu and Z. Y. Chen, Plant Sterols: Chemical and Enzymatic Structural Modifications and Effects on Their Cholesterol-Lowering Activity, J. Agric. Food Chem., 2018, 66, 3047–3062 CrossRef CAS PubMed.
- J. Plat and R. P. Mensink, Plant stanol and sterol esters in the control of blood cholesterol levels: mechanism and safety aspects, Am. J. Cardiol., 2005, 96, 15–22 CrossRef PubMed.
- P. G. Bradford and A. B. Awad, Phytosterols as anticancer compounds, Mol. Nutr. Food Res., 2007, 51, 161–170 CrossRef CAS PubMed.
- R. A. Othman and M. H. Moghadasian, Beyond cholesterol-lowering effects of plant sterols: clinical and experimental evidence of antiinflammatory properties, Nutr. Rev., 2011, 69, 371–382 CrossRef PubMed.
- R. Chawla, S. Sivakumar and N. Goel, Phytosterol and its esters as novel food ingredients: A review, Asian J. Dairy & Food Res., 2016, 35, 217–226 Search PubMed.
- H. Vaikousi, A. Lazaridou, C. G. Biliaderis and J. Zawistowski, Phase transitions, solubility, and crystallization kinetics of phytosterols and phytosterol-oil blends, J. Agric. Food Chem., 2007, 55, 1790–1798 CrossRef CAS PubMed.
- N. Weber, P. Weitkamp and K. D. Mukherjee, Fatty acid steryl, Stanyl, and steroid esters by esterification and transesterification in vacuo using candida rugosa lipase as catalyst, J. Agric. Food Chem., 2001, 49, 67–71 CrossRef CAS PubMed.
- Z. Tan, K. Le, M. Moghadasian and F. Shahidi, Enzymatic synthesis of phytosteryl docosahexaneates and evaluation of their antiatherogenic effects in apo-E deficient mice, Food Chem., 2012, 134, 2097–2104 CrossRef CAS PubMed.
- Y. Pouilloux, G. Courtois, M. Boisseau, A. Piccirilli and J. Barrault, Solid base catalysts for the synthesis of phytosterol esters, Green Chem., 2003, 5, 89–91 RSC.
- Q. Deng, P. Zhang, Q. Huang, F. Huang, F. Wei, M. Zheng, X. Yu, Q. Zhou and C. Zheng, Chemical synthesis of phytosterol esters of polyunsaturated fatty acids with ideal oxidative stability, Eur. J. Lipid Sci. Technol., 2011, 113, 441–449 CrossRef CAS.
- X. H. Meng, Q. Y. Pan and T. K. Yang, Synthesis of phytosteryl esters by using alumina-supported Zinc Oxide (ZnO/Al2O3) from esterification production of phytosterol with fatty acid, J. Am. Oil Chem. Soc., 2011, 88, 143–149 CrossRef CAS.
- Y. Yang, W. He, C. Jia, Y. Ma, X. Zhang and B. Feng, Efficient synthesis of phytosteryl esters using the lewis acidic ionic liquid, J. Mol. Catal. A: Chem., 2012, 357, 39–43 CrossRef CAS.
- X. H. Meng, P. L. Sun, Q. Y. Pan, Z. P. Shi, K. Yang and R. J. He, Synthesis of plant sterol esters catalyzed by heteropolyacid in a solvent-free system, Eur. J. Lipid Sci. Technol., 2006, 108, 13–18 CrossRef CAS.
- B. H. Kim and C. C. Akoh, Modeling and optimization of lipase catalyzed synthesis of phytosteryl esters of oleic acid by response surface methodology, Food Chem., 2007, 102, 336–342 CrossRef CAS.
- T. Kobayashi, A. Ogino, Y. Miyake, H. Mori, A. Hosoda, M. Fujita, T. Tsuno and S. Adachi, Lipase-catalyzed esterification of triterpene alcohols and phytosterols with oleic acid, J. Am. Oil Chem. Soc., 2014, 91, 1885–1890 CrossRef CAS.
- M. M. Zheng, Y. Lu, L. Dong, P. M. Guo, Q. C. Deng, W. L. Li, Y. Q. Feng and F. H. Huang, Immobilization of Candida rugosa lipase on hydrophobic/strong cation-exchange functional silica particles for biocatalytic synthesis of phytosterol esters, Bioresour. Technol., 2012, 115, 141–146 CrossRef CAS PubMed.
- M. M. Zheng, Y. Lu, F. H. Huang, L. Wang, P. M. Guo, Y. Q. Feng and Q. C. Deng, Lipase immobilization on hyper-cross-linked polymer-coated silica for biocatalytic synthesis of phytosterol esters with controllable fatty acid composition, J. Agric. Food Chem., 2013, 61, 231–237 CrossRef CAS PubMed.
- D. S. No, T. Zhao, J. Lee, J. S. Lee and I. H. Kim, Synthesis of phytosteryl ester containing pinolenic acid in a solvent-free system using immobilized Candida rugosa lipase, J. Agric. Food Chem., 2013, 61, 8934–8940 CrossRef CAS PubMed.
- Z. Dong, Z. S. Liu, J. Shi, H. Tang, F. H. Huang and M. M. Zheng, Carbon Nanoparticle-stabilized Pickering Emulsion as a Sustainable and High-performance Interfacial Catalysis Platform for Enzymatic Esterification/Transesterification, ACS Sustain. Chem. Eng., 2019, 8, 7619–7629 CrossRef.
- Y. Xiao, M. M. Zheng, Z. S. Liu, J. Shi, F. H. Huang and G. Luo, Constructing a Continuous Flow Bioreactor Based on a Hierarchically Porous Cellulose Monolith for Ultrafast and Nonstop Enzymatic Esterification/Transesterification, ACS Sustain. Chem. Eng., 2019, 7, 2056–2063 CrossRef CAS.
- M. Miao, H. Liu, B. Jiang, C. Yang, X. Xia and T. Zhang, Enzyme-catalysed synthesis of plant steryl laurate in non-aqueous media using salt hydrate pairs and its characterisation, J. Funct. Foods., 2014, 7, 452–461 CrossRef CAS.
- K. Manabe, S. Iimura, X. M. Sun and S. Kobayashi, Dehydration Reactions in Water. Brønsted Acid-Surfactant-Combined Catalyst for Ester, Ether, Thioether, and Dithioacetal Formation in Water, J. Am. Chem. Soc., 2002, 124, 11971–11978 CrossRef CAS PubMed.
- C. J. Li and L. Chen, Organic chemistry in water, Chem, Soc. Rev., 2006, 35, 68–82 RSC.
- T. Igarashi, D. Yagyu, T. Naito, Y. Okumura, T. Nakajo, Y. Mori and S. Kobayashi, Dehydrative esterification of carboxylic acids with alcohols catalyzed by diarylammonium p-dodecylbenzenesulfonates in water, Appl. Catal. B-Environ., 2012, 119, 304–307 CrossRef.
- W. S. He, Y. Ma, X. X. Pan, J. J. Li, M. G. Wang, Y. B. Yang, C. S. Jia, X. M. Zhang and B. Feng, Efficient solvent-free synthesis of phytostanyl esters in the presence of acid-surfactant-combined catalyst, J. Agric. Food Chem., 2012, 60, 9763–9769 CrossRef CAS PubMed.
- A. Toth, S. Schnedl, D. Painer, M. Siebenhofer and S. Lux, Interfacial Catalysis in Biphasic Carboxylic Acid Esterification with a Nickel-Based Metallosurfactant, ACS Sustain. Chem. Eng., 2019, 7, 18547–18553 CrossRef CAS.
- W. S. He, D. Hu, Y. Wang, X. Y. Chen, C. S. Jia and H. L. Ma, A novel chemoenzymatic synthesis of hydrophilic phytosterol derivatives, Food Chem., 2016, 192, 557–565 CrossRef CAS PubMed.
- B. He, T. Deng, J. Li, F. Yan, H. Wang, Y. Huang and C. Peng, An innovative auto-catalytic esterification for the production of phytosterol esters: experiment and kinetics, RSC Adv., 2014, 4, 64319–64327 RSC.
- H. C. Nguyena, K. Huang and C. Su, Green process for the preparation of phytosterol esters: Microwave-mediated noncatalytic synthesis, Chem. Eng. J., 2020, 382, 1–8 Search PubMed.
- A. Alegría and J. Cuellar, Esterification of oleic acid for biodiesel production catalyzed by 4-dodecylbenzenesulfonic acid, Appl. Catal. B-Environ., 2015, 179, 530–541 CrossRef.
|
This journal is © The Royal Society of Chemistry 2021 |