DOI:
10.1039/D1RA01086G
(Paper)
RSC Adv., 2021,
11, 18576-18579
A formal intermolecular [4 + 1] cycloaddition reaction of 3-chlorooxindole and o-quinone methides: a facile synthesis of spirocyclic oxindole scaffolds†
Received
9th February 2021
, Accepted 8th May 2021
First published on 24th May 2021
Abstract
Herein, we developed an efficient and straightforward method for the rapid synthesis of spirocyclic oxindole scaffolds via the [4 + 1] cyclization reaction of 3-chlorooxindole with o-quinone methides (o-QMs), which were generated under mild conditions. The products could be obtained in excellent yields with numerous types of 3-chlorooxindole. This methodology features mild reaction conditions, high atom-economy and broad substrate scope.
The structural diversity of spirocyclic oxindole scaffolds is a reason for their frequent occurrence in many relevant natural products and medicinal agents (Fig. 1).1 In particular, natural spirocyclic-2-oxindole scaffolds have been proven to exhibit a broad range of biological activities and have attracted increasing attention in the synthetic field. For instance, XEN 907 is a novel pentacyclic spirooxindole with excellent activities as sodium channel blockers.2 Due to their unique structure and intriguing biological activity, numerous methodologies have been developed for the construction of these privileged frameworks.3 For example, in the past few years, transition-metal catalyzed or organocatalytic [3 + 2] cycloaddition reactions have been developed for the synthesis of spirocyclic oxindole scaffolds.4 Despite the emergence of these elegant approaches, exploiting new strategies for the construction of spirocyclic oxindole derivatives is still highly desirable.
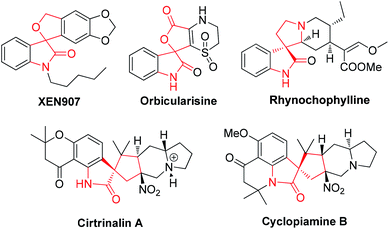 |
| Fig. 1 Examples of biologically active spirocyclic oxindole scaffolds. | |
Ortho-quinone methides (o-QMs) as highly reactive versatile intermediates have been of great interest to the chemical and biological community.5 o-QMs react with various classes of reagents by three typical reaction pathways: 1,4-addition of nucleophiles, [4 + 2] cycloaddition with dienophiles and oxa-6π-electrocyclization.6 Because most o-QMs are unstable, these reactions generally depend on the reaction conditions used for the generation of o-QMs in situ. Rokita et al. reported that o-silylated phenols when exposed to fluoride could also produce o-QMs under mild reaction conditions.7
Because of the dual nature (nucleophilic/electrophilic) of the C-3 position, 3-chlorooxindole serves as a highly reactive starting material in the synthesis of spirocyclic oxindole scaffolds. The introduction of a chloro group at the C-3 position of indoles serves as an excellent leaving group in favour of the subsequent cyclization. In addition, this also increases the acidity of the C–H bond for directly entering the C-3 quaternary centers.8 Inspired by this reactivity profile, 3-chlorooxindoles have been successfully utilized for [2 + 1]9 and [4 + 1]10 cyclization to synthesize spirocyclic oxindole scaffolds (Fig. 2).
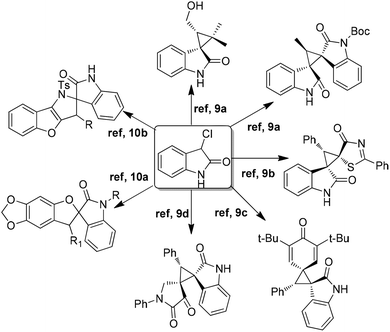 |
| Fig. 2 Representation of the synthesis and applications of 3-chlorooxindoles. | |
We designed an efficient and straightforward method for the rapid synthesis of spirocyclic oxindoles via the [4 + 1] cyclization reaction of 3-chlorooxindole with o-QMs, which were generated under mild conditions. In this study, using TBAF as the fluoride source and base ensures that the one-pot domino reaction will occur in mild reaction conditions, with high atom-economy and broad substrate scope.
Initially, we carried out optimization studies by examining the reaction between O-silylated phenol 2a and 3-chlorooxindole 1a. Indeed, when TBAF was employed as the fluoride source, a smooth [4 + 1] cyclization reaction occurred, affording the spirocyclic oxindole product 3a with 75% yield (entry 1, Table 1). This indicated that our design for the [4 + 1] cyclization reaction required is feasible. When the molar concentration of substrate 2a was raised to 1.5 equiv., the product yield increased to 87% (entry 2, Table 1). Other fluoride sources were then evaluated and TBAF was found to be the optimal one; however, when CsF was employed in this reaction, the product yield decreased to 11% (entry 4, Table 1). When the loading quantity of TBAF was decreased to 3.0 equiv., the desired product 3a yield decreased to 80% (entry 5, Table 1). Finally, numerous solvents including CHCl3, THF, toluene, DMF, MeCN, and MeOH were tested at room temperature, revealing THF as the optimal solvent for this reaction, affording the spirocyclic oxindole product 3a with 94% yield (entries 6–11, Table 1).
Table 1 Optimization of the reaction conditionsa
Reaction conditions: 1a (0.3 mmol), solvent (3.0 mL), 6 h. Isolated yield. TBAF (1 M in THF solution). |
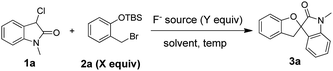 |
Entry |
F− source |
X |
Y |
Solvent |
Temp (°C) |
Yieldb |
1 |
TBAFc |
1.2 |
4.0 |
DCM |
rt |
75% |
2 |
TBAF |
1.5 |
4.0 |
DCM |
rt |
87% |
3 |
TBAF |
2.0 |
4.0 |
DCM |
rt |
85% |
4 |
CsF |
1.5 |
4.0 |
DCM |
rt |
11% |
5 |
TBAF |
1.5 |
3.0 |
DCM |
rt |
80% |
6 |
TBAF |
1.5 |
4.0 |
CHCl3 |
rt |
83% |
7 |
TBAF |
1.5 |
4.0 |
THF |
rt |
94% |
8 |
TBAF |
1.5 |
4.0 |
Toluene |
rt |
90% |
9 |
TBAF |
1.5 |
4.0 |
DMF |
rt |
72% |
10 |
TBAF |
1.5 |
4.0 |
MeCN |
rt |
84% |
11 |
TBAF |
1.5 |
4.0 |
MeOH |
rt |
ND |
With the optimal conditions known, we next investigated the substrate scope of substituted 3-chlorooxindole 1 using O-silylated phenol 2a as a representative (Table 2). First, we examined the substituents on the benzene ring of the indole core regardless of the electronic properties, such as 4-Me, 5-Me, 6-Me, 7-Me, 5-OMe, 7-OMe, 4-Cl, 5-Cl, 6-Cl, 7-Cl, 4-Br, 5-Br, 6-Br, 7-Br, 4-F, 5-F and 6-F. We found that all the reactions could proceed smoothly, affording the corresponding products generally with good yields (62–87%). Second, the substrates 1s with hydrogen atoms linked to the nitrogen were all tolerated to furnish the corresponding products in moderate yields (65%). Finally, different alkyl substituents at the nitrogen position of 3-chlorooxindole 1 did not affect the outcome significantly and gave the products 3t–3v in well-tolerated yields. For example, the reaction of the ethyl-substituted derivative 1t with 2a afforded the desired product 3t in 75% yield.
Table 2 Substrate scopea,b
Reaction conditions: 1 (0.3 mmol), 2a (1.5 eq.), TBAF (4.0 eq.), THF (3.0 mL), 6 h. Isolated yields. |
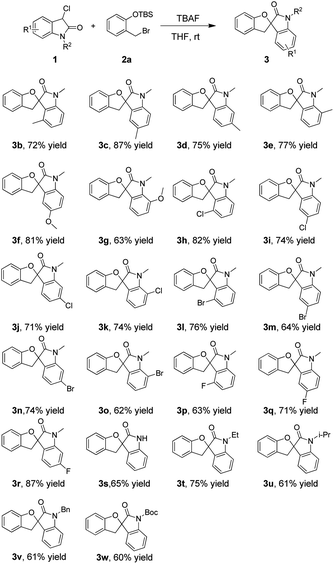 |
Next, we also explored the substrate scope of substituted 3-chlorooxindole 1 using O-silylated phenols 2a′ (Table 3). When the substrate 2a′ was substituted with a chlorine atom, the yield of the desired product 3 yield decreased to 50–62%. For all the obtained products, the substrates 2a had an influence on reaction yield.
Table 3 Substrate scopea,b
Reaction conditions: 1 (0.3 mmol), 2a′ (1.5 eq.), TBAF (4.0 eq.), THF (3.0 mL), 6 h. Isolated yields. |
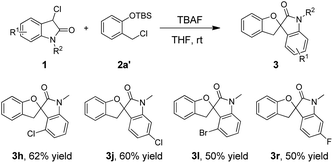 |
On the basis of above-mentioned results, a plausible mechanism for this formal [4 + 1] cycloaddition reaction is depicted in Scheme 1. Initially, the highly reactive o-QMs are generated via the desilylation/elimination reaction. Then, 3-chlorooxindole 1a as a nucleophile attacks the external carbon of o-QMs, affording zwitterion Int-1. Finally, the zwitterion Int-1 loses one molecular HCl through a nucleophilic attack, yielding the spirocyclic oxindole product 3a.
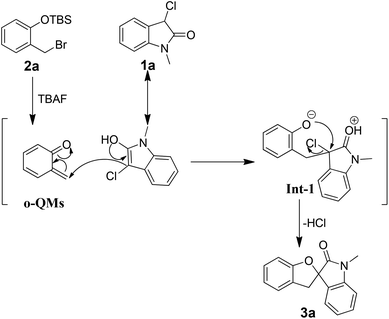 |
| Scheme 1 Possible mechanism. | |
Conclusions
In summary, we have established a formal [4 + 1] cycloaddition reaction of 3-chlorooxindole with O-silylated phenols. This transformation provides an efficient method for the synthesis of the spirocyclic oxindoles in good yields (up to 94%). This methodology features mild reaction conditions and a broad substrate scope.
Conflicts of interest
There are no conflicts to declare.
Acknowledgements
The study was supported by the Science and Technology Innovation Development Planning Project of Yantai (2019MSGY128).
Notes and references
-
(a) N. Ye, H. Chen, E. A. Wold, P.-Y. Shi and J. Zhou, ACS Infect. Dis., 2016, 2, 382 CrossRef CAS PubMed;
(b) T. L. Pavlovska, R. G. Redkin, V. V. Lipson and D. V. Atamanuk, Mol. Diversity, 2016, 20, 299 CrossRef CAS PubMed;
(c) R. Narayan, M. Potowski, Z.-J. Jia, A. P. Antonchick and H. Waldmann, Acc. Chem. Res., 2014, 47, 1296 CrossRef CAS PubMed;
(d) E. F. Pimenta, A. M. Vita-Marques, A. Tininis, M. H. R. Seleghim, L. D. Sette, K. Veloso, A. G. Ferreira, D. E. Williams, B. O. Patrick, D. S. Dalisay, R. J. Andersen and R. G. S. Berlinck, J. Nat. Prod., 2010, 73, 1821 CrossRef CAS PubMed;
(e) B. Zhang, W. Zheng, X. Wang, D. Sun and C. Li, Angew. Chem., Int. Ed., 2016, 55, 10435 CrossRef CAS PubMed;
(f) A. E. Fraley, K. C. Haatveit, Y. Ye, S. P. Kelly, S. A. Newmister, F. Yu, R. M. Williams, J. L. Smith, K. N. Houk and D. H. Sherman, J. Am. Chem. Soc., 2020, 142, 2244 CrossRef CAS PubMed.
- S. Chowdhury, S.-F. Liu, J. A. Cadieux, T. Hsieh, M. Chafeev, S.-Y. Sun, Q. Jia, J.-Y. Sun, M. Wood, J. Langille, S. Sviridov, J.-M. Fu, Z.-H. Zhang, R. Chui, A. Wang, X. Cheng, J. Zhong, S. Hossain, K. Khakh, I. Rajlic, H. Verschoof, R. Kwan and W. Young, Med. Chem. Res., 2013, 22, 1825 CrossRef CAS.
-
(a) Y. M. Cao, F. F. Shen, F. T. Zhang and R. Wang, Chem.–Eur. J., 2013, 19, 1184 CrossRef CAS PubMed;
(b) L. Tian, X.-Q. Hu, Y.-H. Li and P.-F. Xu, Chem. Commun., 2013, 49, 7213 RSC;
(c) D. Jiang, S. Dong, W. Tang, T. Lu and D. Du, J. Org. Chem., 2015, 80, 11593 CrossRef CAS PubMed;
(d) G.-J. Mei and F. Shi, Chem. Commun., 2018, 54, 6607 RSC;
(e) X.-L. Jiang, S.-J. Liu, Y.-Q. Gu, G.-J. Mei and F. Shi, Adv. Synth. Catal., 2017, 359, 3341 CrossRef CAS;
(f) Q. Wan, L. Chen, S. Li, Q. Kang, Y. Yuan and Y. Du, Org. Lett., 2020, 22, 9539 CrossRef CAS PubMed;
(g) J. W. Wang, L. Zhao, Q. Rong, C. Lv, Y. Lu, X. Pan, L. Zhao and L. Hu, Org. Lett., 2020, 22, 5833 CrossRef CAS PubMed;
(h) X.-B. Huang, X.-J. Li, T.-T. Li, B. Chen, W.-D. Chu, L. He and Q.-Z. Liu, Org. Lett., 2019, 21, 1713 CrossRef CAS PubMed.
-
(a) W. Tan, X. Li, Y. X. Gong, M. D. Ge and F. Shi, Chem. Commun., 2014, 50, 15901 RSC;
(b) Q. S. Sun, H. Zhu, Y. J. Chen, X. D. Yang, X. W. Sun and G. Q. Lin, Angew. Chem., Int. Ed., 2015, 54, 13253 CrossRef CAS PubMed;
(c) G. Zhan, M. L. Shi, Q. He, W. J. Lin, Q. Ouyang, W. Du and Y. C. Chen, Angew. Chem., Int. Ed., 2016, 55, 2147 CrossRef CAS PubMed;
(d) T. Fan, H. H. Zhang, C. Li, Y. Shen and F. Shi, Adv. Synth. Catal., 2016, 358, 2017 CrossRef CAS;
(e) Y. Chen, B. D. Cui, Y. Wang, W. Y. Han, N. W. Wan, M. Bai, W. C. Yuan and Y. Z. Chen, J. Org. Chem., 2018, 83, 10465 CrossRef CAS PubMed;
(f) K. Singh, S. Pramanik, T. A. Hamlin, B. Mondal, D. Das and J. Saha, Chem. Commun., 2019, 55, 7069 RSC;
(g) Y. Lin, B. L. Zhao and D. M. Du, J. Org. Chem., 2019, 84, 10209 CrossRef CAS PubMed;
(h) R.-R. Liu, Y. Xu, R.-X. Liang, B. Xiang, H.-J. Xie, J.-R. Gao and Y.-X. Jia, Org. Biomol. Chem., 2017, 15, 2711 RSC;
(i) B. Tan, N. R. Candeias and C. F. Barbas, J. Am. Chem. Soc., 2011, 133, 4672 CrossRef CAS PubMed;
(j) J. Zhang, C. Cheng, D. Wang and Z. Miao, J. Org. Chem., 2017, 82, 10121 CrossRef CAS PubMed.
- For selected reviews on o-QMs, see:
(a) N. J. Willis and C. D. Bray, Chem.–Eur. J., 2012, 18, 9160 CrossRef CAS PubMed;
(b) M. S. Singh, A. Nagaraju, N. Anand and S. Chowdhury, RSC Adv., 2014, 4, 55924 RSC;
(c) T. P. Pathak and M. S. Sigman, J. Org. Chem., 2011, 76, 9210 CrossRef CAS PubMed;
(d) A. A. Jaworski and K. A. Scheidt, J. Org. Chem., 2016, 81, 10145 CrossRef CAS PubMed;
(e) B. Yang and S. Gao, Chem. Soc. Rev., 2018, 47, 7926 RSC;
(f) S. Mukhopadhyay, C. Gharui and S. C. Pan, Asian J. Org. Chem., 2019, 8, 1970 CrossRef CAS.
- For selected examples of o-QMs in reactions, see:
(a) P. Chen, K. Wang, W. Guo, X. Liu, Y. Liu and C. Li, Angew. Chem., Int. Ed., 2017, 56, 3689 CrossRef CAS PubMed;
(b) X. Gu, H. Yuan, J. Jiang, Y. Wu and W. J. Bai, Org. Lett., 2018, 20, 7229 CrossRef CAS PubMed;
(c) M. Sun, C. Ma, S. J. Zhou, S. F. Lou, J. Xiao, Y. Jiao and F. Shi, Angew. Chem., Int. Ed., 2019, 58, 8703 CrossRef CAS PubMed;
(d) Y. You, T. T. Li, S. P. Yuan, K. X. Xie, Z. H. Wang, J. Q. Zhao, M. Q. Zhou and W. C. Yuan, Chem. Commun., 2020, 56, 439 RSC;
(e) Z. Zhu, M. Odagi, N. Supantanapong, W. Xu, J. Saame, H.-U. Kirm, K. A. Abboud, I. Leito and D. Seidel, J. Am. Chem. Soc., 2020, 142, 15252 CrossRef CAS PubMed;
(f) F. Göricke, S. Haseloff, M. Laue, M. Schneider, T. Brumme and C. Schneider, J. Org. Chem., 2020, 85, 11699 CrossRef PubMed.
-
(a) S. E. Rokita, J. H. Yang, P. Pande and W. A. Greenberg, J. Org. Chem., 1997, 62, 3010 CrossRef CAS PubMed;
(b) A. F. Barrero, J. F. Q. del Moral, M. M. Herrador, P. Arteaga, M. Corte's, J. Benites and A. Rosellón, Tetrahedron, 2006, 62, 6012 CrossRef CAS;
(c) X. T. Lei, C.-H. Jiang, X. A. Wen, Q.-L. Xu and H. B. Sun, RSC Adv., 2015, 5, 14953 RSC.
-
(a) G. Bergonzini and P. Melchiorre, Angew. Chem., 2012, 124, 995 CrossRef;
(b) A. Noole, I. Jarving, F. Werner, M. Lopp, A. Malkov and T. Kanger, Org. Lett., 2012, 14, 4922 CrossRef CAS PubMed.
-
(a) A. Noole, M. Oseka, T. Pehk, M. Oeren, I. Jarving, M. R. J. Elsegood, A. V. Malkov, M. Lopp and T. Kanger, Adv. Synth. Catal., 2013, 355, 829 CrossRef CAS;
(b) Y.-X. Song and D.-M. Du, Org. Biomol. Chem., 2019, 17, 5375 RSC;
(c) J.-R. Zhang, H.-S. Jin, J. Sun, J. Wang and L.-M. Zhao, Eur. J. Org. Chem., 2020, 31, 4988 CrossRef;
(d) J.-B. Wen and D.-M. Du, Org. Biomol. Chem., 2020, 18, 1647 RSC.
-
(a) X. L. Jiang, S. J. Liu, Y. Q. Gu, G. J. Mei and F. Shi, Adv. Synth. Catal., 2017, 359, 3341 CrossRef CAS;
(b) C.-S. Wang, T.-Z. Li, Y.-C. Cheng, J. Zhou, G.-J. Mei and F. Shi, J. Org. Chem., 2019, 84, 3214 CrossRef CAS PubMed.
Footnote |
† Electronic supplementary information (ESI) available. See DOI: 10.1039/d1ra01086g |
|
This journal is © The Royal Society of Chemistry 2021 |
Click here to see how this site uses Cookies. View our privacy policy here.