DOI:
10.1039/D1RA02007B
(Paper)
RSC Adv., 2021,
11, 13848-13852
Poly(ethylene glycol) dimethyl ether mediated oxidative scission of aromatic olefins to carbonyl compounds by molecular oxygen†
Received
13th March 2021
, Accepted 5th April 2021
First published on 14th April 2021
Abstract
A simple, and practical oxidative scission of aromatic olefins to carbonyl compounds using O2 as the sole oxidant with poly(ethylene glycol) dimethyl ether as a benign solvent has been developed. A wide range of monosubstituted, gem-disubstituted, 1,2-disubstituted, trisubstituted and tetrasubstituted aromatic olefins was successfully converted into the corresponding aldehydes and ketones in excellent yields even with gram–scale reaction. Some control experiments were also conducted to support a possible reaction pathway.
Introduction
The selective oxidative scission of olefins is a practiced transformation in organic synthesis. The produced carbonyl compounds are valuable intermediates in pharmaceuticals, fragrances, agrochemicals and bulk chemical industries.1 The two-step ozonolysis is the conventional method to convert olefins into carbonyl compounds (Scheme 1a).2 In recent years, the ozone was replaced by other oxidants, such as H2O2,3 oxone,4 TBHP,5 m-CPBA,6 KMnO4,7 PhIO/HBF4
8 (Scheme 1b). However, the super stoichiometric use of expensive and toxic oxidants leads to a large amount of resource waste and environmental pollution.
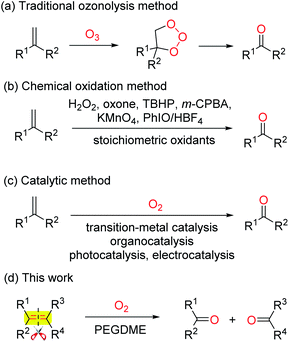 |
| Scheme 1 Oxidative scission of olefins to carbonyl compounds. | |
Molecular oxygen is regarded as an ideal oxidant due to its easy availability, cheapness, environmental benignity and good functional-group tolerance. Recently, a series oxidative scission of olefins to carbonyl compounds with O2 as the sole oxidant, catalysed by organocatalysts NHPI,9 AIBN,10 B2pin2,11 TEMPO,12 transitional-metal complexes Pd,13 Cu,14 Fe,15 Ni,16 CAN,17 as well as photocatalysts18 and electrocatalysts,19 have been reported (Scheme 1c). However, some shortcomings including non-commercial available catalysts, expensive additives, inevitable residual transitional-metals, and excess amount of volatile organic solvents limit their application in industry. Very recently, although a 1,2-diethoxyethane catalysed oxidative scission of olefins to ketones by air has been achieved, the olefins are limited to gem-disubstituted aromatic alkenes.20 Therefore, developing a wide applicable strategy for the oxidative scission of olefins to aldehydes and ketones is highly desirable but still remains a challenge.
Poly(ethylene glycol) dimethyl ether (PEGDME) has been attracting increasing interest due to its benign characteristics involving lower cost, non-volatilization, and non-toxicity, etc.21 To continue our interest in developing environmental benign synthetic reactions.22 Herein we report a simple and practical oxidative scission of a wide range of monosubstituted, gem- and 1,2-disubstituted, trisubstituted, and tetrasubstituted aromatic olefins to the corresponding aldehydes and ketones by O2 with PEGDME as a benign solvent.
Results and discussion
Our studies were started with gem-diphenylethylene (1a) as a model substrate (Table 1). When the oxidation scission of 1a was performed in N,N-dimethylformamide (DMF), methyl tert-butyl ether (MTBE), tetrahydrofuran (THF), 2-methyltetrahydrofuran (2-MeTHF) at 130 °C or under reflux condition with O2 as the sole oxidant, only trace amount of diphenylmethanone (2a) was observed (entries 1–4). To our delight, the desired product 2a was detected by HPLC with 73% yield in 1,4-dioxane at 100 °C for 10 hours (entry 5). However, when 1,4-dioxane was replaced by morpholine, the yield decreased to 49% (entry 6). To improve the reaction efficiency, ethylene glycol (EG), triethylene glycol (TEG), and a series of ethers including ethylene glycol dimethyl ether (EGDME), ethylene glycol diethyl ether (EGDEE), diethylene glycol monomethyl ether (DEGMME), diethylene glycol dimethyl ether (DEGDME), dipropylene glycol monomethyl ether (DPGMME), and dipropylene glycol dimethyl ether (DPGDME) were screened as a solvent at 130 °C for 10 hours, providing 54–94% yields (entries 7–14). Moreover, it was found that the environmentally friendly poly(ethylene glycol) (PEG) and poly(ethylene glycol) dimethyl ether (PEGDME) gave 96% and 99% yield, respectively (entries 15–16). Therefore, PEGDME was demonstrated to be the best solvent. Next, the effect of the reaction temperature was examined. The yield of 2a remained at 99% when the reaction temperature dropped to 110 °C, but lower yield of 78% was observed at 100 °C (entries 17–18). Shorter reaction time was also attempted, the results revealed that the yield remained at 99% at 8 hours (entries 19–20). When the reaction was performed under air atmosphere, the yield decreased to 35% (entry 21). In addition, it was found that the higher concentration of 1a led to lower yield of 2a (entries 22–23). Finally, the entry 19 was regarded as the optimal reaction conditions: 1a (0.5 mmol), PEGDME (1 mL), under O2 atmosphere, 110 °C, and 8 hours.
Table 1 Optimization of the reaction conditionsa,b
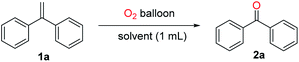
|
Entry |
Solvent |
Temp. (°C) |
t (h) |
Yieldc (%) |
Abbreviation: N,N-dimethylformamide (DMF), methyl tert-butyl ether (MTBE), tetrahydrofuran (THF), 2-methyltetrahydrofuran (2-MeTHF), ethylene glycol (EG), triethylene glycol (TEG), ethylene glycol dimethyl ether (EGDME), ethylene glycol diethyl ether (EGDEE), diethylene glycol monomethyl ether (DEGMME), diethylene glycol dimethyl ether (DEGDME), dipropylene glycol monomethyl ether (DPGMME), dipropylene glycol dimethyl ether (DPGDME), poly(ethylene glycol) (average Mn, 400) (PEG400), poly(ethylene glycol) dimethyl ether (average Mn, 250) (PEGDME250). Reaction conditions: 1a (0.5 mmol), solvent (1 mL), O2 balloon. The yields were determined by HPLC. Under air atmosphere. 1 mmol of 1a. 5 mmol of 1a. |
1 |
DMF |
130 |
10 |
<2% |
2 |
MTBE |
55 |
10 |
<2% |
3 |
THF |
66 |
10 |
<2% |
4 |
2-MeTHF |
78 |
10 |
<2% |
5 |
1,4-Dioxane |
100 |
10 |
73 |
6 |
Morpholine |
130 |
10 |
49 |
7 |
EG |
130 |
10 |
54 |
8 |
TEG |
130 |
10 |
85 |
9 |
EGDME |
86 |
10 |
61 |
10 |
EGDEE |
130 |
10 |
90 |
11 |
DEGMME |
130 |
10 |
91 |
12 |
DEGDME |
130 |
10 |
93 |
13 |
DPGMME |
130 |
10 |
94 |
14 |
DPGDME |
130 |
10 |
94 |
15 |
PEG |
130 |
10 |
96 |
16 |
PEGDME |
130 |
10 |
99 |
17 |
PEGDME |
110 |
10 |
99 |
18 |
PEGDME |
100 |
10 |
78 |
19 |
PEGDME |
110 |
8 |
99 |
20 |
PEGDME |
110 |
6 |
83 |
21d |
PEGDME |
110 |
8 |
35 |
22e |
PEGDME |
110 |
8 |
64 |
23f |
PEGDME |
110 |
8 |
52 |
With the optimized reaction conditions in hand, the scope of various olefins was investigated. As shown in Scheme 2, the oxidative scission of gem-diphenylethylenes bearing electron-donating group (Me, OMe) at the ortho-, meta- or para position, of the phenyl proceeded successfully to give the corresponding 2b–2g with >98% yield. In addition, the halogen element (F, Cl, Br) substituted gem-diphenylethylenes were also gave corresponding ketones 2h–2l in 90–99% yields. The halogen substituents are useful entities amenable to further transformation in organic synthesis. The gem-diphenylethylenes with meta-substituted electron-withdrawing group CF3 gave higher yield than the ortho-substituted one (2m: 73%, 2n: 99%). The gem-diphenylethylenes with two or three substituents on the phenyls also gave the desired products 2o–2u in 86–99% yields. When phenyl of gem-diphenylethylene was replaced by naphthyl, thienyl and pyridyl, the oxidative scission also proceeded smoothly, affording the corresponding products 2v–2x in 60–95% yields. To our delighted, the substrates containing fluorene or thioxanthene moiety also provided the desired ketones 2y and 2z in 87% and 85% yields. Furthermore, the aryl-alkyl disubstituted olefins like α-methylstyrene and α-cyclopropylstyrene were also applicable to this oxidative scission. The corresponding ketones 2aa and 2ab were obtained in 99% and 93% yields.
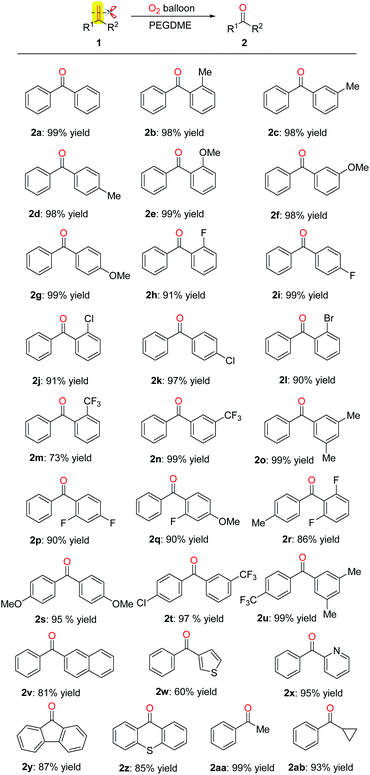 |
| Scheme 2 Scope of gem-disubstituted aromatic olefins. Reaction conditions: 1 (0.5 mmol), PEGDME (1 mL), O2 balloon, 110 °C, 8 hours, isolated yield. | |
Aldehydes are also important building blocks in fine chemicals. And then, we examined this oxidative scission of olefins to prepare aldehydes. As shown in Scheme 3, the monosubstituted, 1,2-disubstituted, trisubstituted and tetrasubstituted aromatic olefins were also subjected to this transformation. For example, the monosubstituted aromatic olefine 1ac was cleavaged to give 4-bromobenzaldehyde (2ac) in 92% yield under standard conditions. Benzaldehyde 2ad was obtained as the sole product in 95% yield by the oxidative scission of 1,2-disubstituted aromatic olefine 1ad. Interestingly, the 1,4-bis(2-methylstyryl)benzene (1ae) can be cleavaged to 1,4-phthalaldehyde (2ae) and 2-methylbenzaldehyde (2ae') in 83% and 82% yield. When trisubstituted 1,1-diphenyl-2-(4-bromophenyl)ethene (1af) and triphenylethylene (1ag) were used as substrates, the corresponding products 4-bromobenzaldehyde (2ac), benzaldehyde (2ad) and diphenylmethanone (2a) were obtained in about 90% yields. Finally, the tetraphenylethylene (1ah) was also subjected to this oxidative scission, less than 5% yield was obtained even if elevating the temperature to 150 °C and extending the reaction time to 24 hours.
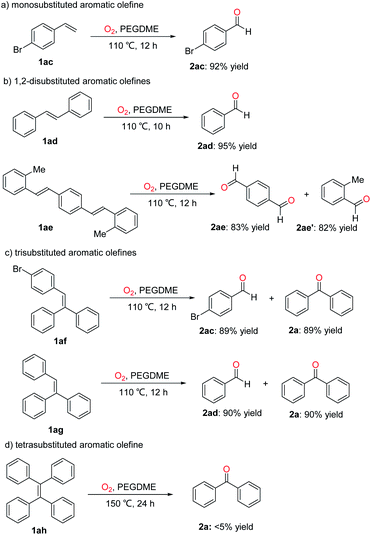 |
| Scheme 3 Scope of mono, di-, tri-, and tetrasubstituted aromatic olefins. | |
To understand the reaction pathway, control experiments were conducted. As shown in Scheme 4, when the oxidative scission of gem-diphenylethylene (1a) was carried out under N2 atmosphere, no product 2a was observed. Only a trace amount of oxidation product 2a was detected in the presence of a radical scavenger 2,2,6,6-tetramethylpiperidine-N-oxyl (TEMPO) under optimal conditions. It means that a radical reaction pathway might be involved. When 2,2-diphenyloxirane (3a) was used as the starting material, the desired ketone 2a could be obtained in 99% yield, indicating that 3a might be a key intermediate of this oxidative scission reaction.
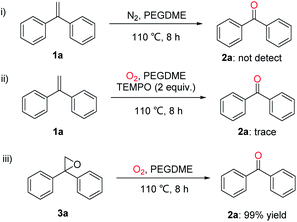 |
| Scheme 4 Control experiments. | |
Based on the control experiments and reported work,20 a plausible reaction pathway was proposed as shown in Scheme 5. Firstly, PEGDME was oxidized by O2 to produce a peroxyl radical 3a′. Secondly, 3,3-diphenyl-1,2-dioxetane (1a′) was formed by the oxidation of gem-diphenylethylene (1a) with 3a′ as an oxidant, regenerating PEGDME concurrently. And then, 1a′ is converted to the more stable key intermediate 2,2-diphenyloxirane (3a) with one equivalent of 1a. Finally, 3a was converted to give the product 2a with 3a′ as an oxidant.
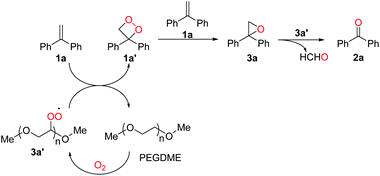 |
| Scheme 5 Plausible reaction pathway. | |
To further demonstrate the practicality of this oxidative scission reaction, as shown in Scheme 6, a gram–scale reaction of gem-diphenylethylene (1a; 1.80 g, 10 mmol) was conducted under O2 atmosphere in PEGDME at 110 °C for 10 hours, the product diphenylmethanone (2a) was isolated in 96% yield (1.75 g).
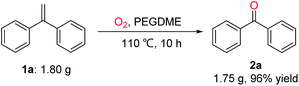 |
| Scheme 6 Gram-scale synthesis. | |
Conclusions
In summary, we have developed an oxidative scission of aromatic olefins to carbonyl compounds using molecular oxygen as the sole oxidant with PEGDME as solvent. A wide range of monosubstituted, gem- and 1,2-disubstituted, trisubstituted, and tetrasubstituted aromatic olefins were oxidized to aldehydes and ketones in excellent yields. A reaction pathway was proposed based on some control experiments. A successful gram–scale reaction also demonstrated its practicability.
Experimental section
General information
Unless otherwise noted, all reagents, catalysts and solvents were purchased from commercial suppliers and used without further purification. Column chromatography was performed with silica gel (200–300 mesh). NMR spectra were recorded on Bruker AVANCE III (400 MHz) spectrometers. CDCl3 was the solvent used for the NMR analysis, with tetramethyl silane as an internal standard. Chemical shifts were reported up field to TMS (0.00 ppm) for 1H NMR and relative to CDCl3 (77.0 ppm) for 13C NMR. HPLC analysis was conducted on an Agilent 1200 Series instrument with 5C18-MS-II Packed Column (4.6 mm I.D. × 250 mm).
General procedure for oxidation scission of aromatic olefin
The corresponding aromatic olefin 1 (0.5 mmol), PEGDME (1 mL) were added to a 10 mL Schlenk tube. The tube was evacuated and filled with oxygen three times. The mixture was stirred at 110 °C for 8 hours under O2 atmosphere using a balloon. After cooling, the mixture was subjected to silica gel column chromatography (PE
:
EA = 15
:
1) to give the product 2.
Gram-scale oxidation scission of gem-diphenylethylene (1a)
The gem-diphenylethylene (1a, 1.80 g, 10 mmol), PEGDME (20 mL) were added to a 50 mL of round-bottomed flask equipped with a three-way jointer. The flask was then evacuated and filled with oxygen three times. The mixture was stirred at 110 °C for 10 hours under O2 atmosphere using a balloon. After cooling, the mixture was subjected to silica gel column chromatography (PE
:
EA = 15
:
1) to give the product 2a (1.75 g, 96% yield).
Conflicts of interest
There are no conflicts to declare.
Acknowledgements
This work was supported by the 111 Project (D20015) and the Natural Science Foundation of Hubei Province of China (2018CFB241).
Notes and references
-
(a) M. B. Smith and J. March, Advanced Organic Chemistry, Wiley-VCH, New York, 2007 Search PubMed;
(b) J. E. Backvall, Modern Oxidation Methods, Wiley-VCH, New York, 2004 CrossRef;
(c) R. C. Larock, Comprehensive Organic Transformations, 2nd edn, Wiley-VCH, New York, 1999 Search PubMed;
(d) F. Puls and H. J. Knolker, Angew. Chem., Int. Ed., 2018, 57, 1222 CrossRef CAS PubMed;
(e) N. E. Thornburg, A. B. Thompson and J. M. Notestein, ACS Catal., 2015, 5, 5077 CrossRef CAS;
(f) A. Kumar, A. K. Gupta, M. Devi, K. E. Gonsalves and C. P. Pradeep, Inorg. Chem., 2017, 56, 10325 CrossRef CAS PubMed.
-
(a) R. Criegee, Angew. Chem., Int. Ed., 1975, 14, 745 CrossRef;
(b) A. J. Kendall, J. T. Barry, D. T. Seidenkranz, A. Ryerson, C. Hiatt, C. A. Salazar, D. J. Bryant and D. R. Tyler, Tetrahedron Lett., 2016, 57, 1342 CrossRef CAS;
(c) T. J. Fisher and P. H. Dussault, Tetrahedron, 2017, 73, 4233 CrossRef CAS;
(d) S. G. V. Ornum, R. M. Champeau and R. Pariza, Chem. Rev., 2006, 106, 2990 CrossRef PubMed.
-
(a) W. Yu and Z. Zhao, Org. Lett., 2019, 21, 7726 CrossRef CAS PubMed;
(b) C. P. Yap, J. K. Ng, S. Madrahimov, A. A. Bengali, T. S. Chwee and W. Y. Fan, New J. Chem., 2018, 42, 11131 RSC;
(c) T. Wang, X. Jing, C. Chen and L. Yu, J. Org. Chem., 2017, 82, 9342 CrossRef CAS PubMed.
-
(a) J. N. Moorthy and K. N. Parida, J. Org. Chem., 2015, 79, 11431 CrossRef PubMed;
(b) D. Yang and C. Zhang, J. Org. Chem., 2001, 66, 4814 CrossRef CAS PubMed.
-
(a) D. J. Lippincott, P. J. Trejo-Soto, F. Gallou and B. H. Lipshutz, Org. Lett., 2018, 20, 5094 CrossRef CAS PubMed;
(b) M. M. Hossain, W. Huang, H. J. Chen, P. Wang and S. G. Shyu, Green Chem., 2014, 16, 3013 RSC;
(c) D. Xing, B. Guan, G. Cai, Z. Fang, L. Yang and Z. Shi, Org. Lett., 2006, 8, 693 CrossRef CAS PubMed.
- F. V. Singh, H. M. S. Milagre, M. N. Eberlin and H. A. Stefani, Tetrahedron Lett., 2009, 50, 2312 CrossRef CAS.
- S. Lai and D. G. Lee, Synthesis, 2001, 11, 1645 CrossRef.
- K. Miyamoto, N. Tada and M. Ochiai, J. Am. Chem. Soc., 2007, 129, 2772 CrossRef CAS PubMed.
- R. Lin, F. Chen and N. Jiao, Org. Lett., 2012, 14, 4158 CrossRef CAS PubMed.
- G. Wang, X. Li, J. Dai and H. Xu, J. Org. Chem., 2014, 79, 7220 CrossRef CAS PubMed.
- Z. Cheng, W. Jin and C. Liu, Org. Chem. Front., 2019, 6, 841 RSC.
- T. Wang and N. Jiao, J. Am. Chem. Soc., 2013, 135, 11692 CrossRef CAS PubMed.
-
(a) C. A. Hone, A. O'Kearney-McMullan, R. Munday and C. O. Kappe, ChemCatChem, 2017, 9, 3298 CrossRef CAS;
(b) A. Wang and H. Jiang, J. Org. Chem., 2010, 75, 2321 CrossRef CAS PubMed;
(c) B. Feng, Z. Hou, X. Wang, Y. Hu, H. Li and Y. Qiao, Green Chem., 2009, 11, 1446 RSC.
-
(a) Y. Liu, D. Xue, C. Li, J. Xiao and C. Wang, Catal. Sci. Technol., 2017, 7, 5510 RSC;
(b) M. M. Hossain and S. G. Shyu, Tetrahedron, 2014, 70, 251 CrossRef CAS;
(c) M. Tokunaga, Y. Shirogane, H. Aoyama, Y. Obora and Y. Tsuji, J. Organomet. Chem., 2005, 690, 5378 CrossRef CAS.
-
(a) B. Xiong, X. Zeng, S. Geng, S. Chen, Y. He and Z. Feng, Green Chem., 2018, 20, 4521 RSC;
(b) A. Toru and F. Hayato, Tetrahedron Lett., 2018, 59, 2657 CrossRef;
(c) A. Gonzalez-De-Castro and J. Xiao, J. Am. Chem. Soc., 2015, 137, 8206 CrossRef CAS PubMed.
- G. Urgoitia, R. SanMartin, M. T. Herrero and E. Domínguez, Adv. Synth. Catal., 2016, 358, 1150 CrossRef CAS.
- L. Yu, Y. Huang, Z. Bai, B. Zhu, K. Ding, T. Chen, Y. Ding and Y. Wang, J. Chin. Chem. Soc., 2015, 62, 479 CrossRef CAS.
-
(a) S. S. Han, J. Y. Park, H. S. Hwang, H. R. Choe, K. M. Nam and E. J. Cho, ChemSusChem, 2019, 12, 3018 CrossRef CAS PubMed;
(b) K. Ohkubo, T. Nanjo and S. Fukuzumi, Org. Lett., 2005, 7, 4265 CrossRef CAS PubMed;
(c) Y. Ren, Y. Che, W. Ma, X. Zhang, T. Shen and J. Zhao, New J. Chem., 2004, 28, 1464 RSC;
(d) Y. Deng, X. Wei, H. Wang, Y. Sun, T. Noel and X. Wang, Angew. Chem., Int. Ed., 2017, 56, 832 CrossRef CAS PubMed.
- Y. Imada, Y. Okada, K. Noguchi and K. Chiba, Angew. Chem., Int. Ed., 2019, 58, 125 CrossRef CAS PubMed.
- K. Liu, J. Deng, T. Zeng, X. Chen, Y. Huang, Z. Cao, Y. Lin and W. He, Chin. Chem. Lett., 2020, 31, 1868 CrossRef CAS.
-
(a) L. J. Xu, K. H. Lam, J. X. Ji, J. Wu, Q. H. Fan, W. H. Lo and A. S. C. Chan, Chem. Commun., 2005, 1390 RSC;
(b) H. F. Zhou, Q. H. Fan, Y. M. He, L. Q. Qu and A. S. C. Chan, Prog. Chem., 2009, 17, 1517 Search PubMed;
(c) S. J. Ha, E.-Y. Jung, W. M. Kim and J. C. Lee, Bull. Korean Chem. Soc., 2014, 35, 629 CrossRef CAS;
(d) K. J. Liu, J. H. Deng, J. Yang, S. F. Gong, Y. W. Lin, J. Y. He, Z. Cao and W. M. He, Green Chem., 2020, 22, 433 RSC.
-
(a) H. F. Zhou, Q. H. Fan, W. J. Tang, L. J. Xu, Y. M. He, G. J. Deng, L. W. Zhao, L. Q. Gu and A. S. C. Chan, Adv. Synth. Catal., 2006, 348, 2172 CrossRef CAS;
(b) H. F. Zhou, Q. H. Fan, Y. Y. Huang, L. Wu, Y. M. He, W. J. Tang, L. Q. Gu and A. S. C. Chan, J. Mol. Catal. A: Chem., 2007, 275, 47 CrossRef CAS;
(c) H. Zhou, Z. Li, Z. Wang, T. Wang, L. Xu, Y. He, Q. H. Fan, J. Pan, L. Gu and A. S. C. Chan, Angew. Chem., Int. Ed., 2008, 47, 8464 CrossRef CAS PubMed;
(d) P. Cui, Q. Liu, J. Wang, H. Liu and H. Zhou, Green Chem., 2019, 21, 634 RSC;
(e) B. Yang, P. Cui, Y. Chen, Q. Liu and H. Zhou, Tetrahedron Lett., 2020, 61, 152530 CrossRef CAS.
Footnotes |
† Electronic supplementary information (ESI) available. See DOI: 10.1039/d1ra02007b |
‡ These authors contributed equally to this work. |
|
This journal is © The Royal Society of Chemistry 2021 |
Click here to see how this site uses Cookies. View our privacy policy here.