DOI:
10.1039/D1RA03813C
(Paper)
RSC Adv., 2021,
11, 24196-24205
Highly sensitive colorimetric sensing of copper(II) ions based on “CLICK-17” DNAzyme-catalyzed azide modified gold nanoparticles and alkyne capped dsDNA cycloaddition
Received
16th May 2021
, Accepted 28th June 2021
First published on 9th July 2021
Abstract
A click chemistry assay based on a newly discovered DNAzyme, CLICK-17, with azide modified gold nanoparticles (azide-AuNPs) and alkyne capped dsDNA (alkyne-linker DNA) was employed for novel and selective detection of Cu2+ visually. The strategy involved using CLICK-17 to mediate a catalytic reaction for triazole formation between azide-AuNPs and alkyne-linker DNA under the help of Cu2+ (without sodium ascorbate) or Cu+, which eventually led to the aggregation of AuNPs. The obvious color change from ruby red to bluish purple was then observed by the naked eye and the absorbance peak shifted from 525 to 570 nm. Interestingly, CLICK-17 and Cu+-catalyzed click reaction had the best performance compared to either Cu+ alone or CLICK-17 and Cu2+-mediated reaction in terms of the reaction time and sensitivity. This system has been demonstrated to allow quantitative measurement of Cu2+ with a detection limit as low as 26.8 nM and also has high specificity that can distinguish Cu2+ from other metal ions. Further, the method was tested with a real mineral water sample for Cu2+ concentration determination. Satisfactory recoveries of 90.8% and 99.8% were achieved.
1 Introduction
Copper is the third most abundant essential trace metal for all living organisms and also a cofactor for various enzymes and proteins.1,2 Copper can accumulate in the human body through the food chain and drinking water, and appropriate amounts of copper play a vital role in physiological processes.3 However, excessive intakes of copper cause bio-toxicity with nausea and vomiting and pose serious health risks, including liver and kidney damage, initiation of neurodegenerative diseases and Alzheimer's disease.4–6 In the worst cases, since copper has been widely utilized in many sectors including copper-coated surfaces and medical devices,7 when these copper-containing products are not disposed of properly, they could lead to contamination or environmental pollution. In fact, even up to now, high contamination of copper can still be detected in some areas.8–10 The United States Environmental Protection Agency (USEPA)11 and the World Health Organization (WHO)12 have set the permissible limit for copper in drinking water at 20.4 μM (1.3 mg L−1) and 31.5 μM (2.0 mg L−1), respectively. As such, it is important to develop efficient, sensitive and selective methods for determination of trace amounts of copper.
Some analytical techniques have been reported for copper determination including atomic absorption spectrometry (AAS),9,13,14 inductively coupled plasma-atomic emission spectrometry (ICP-AES),9 inductively coupled plasma-mass spectrometry (ICP-MS),15 and electrochemical methods.16 Although these methods have high selectivity and sensitivity, some drawbacks still exist. For example, they involve expensive and complex instruments with high skill requirement in operation. The sample treatment process is cumbersome, resulting in time-consuming detection. In contrast, during the past few years, colorimetric and fluorescent sensing have attracted interest in copper detection due to their simplicity, low cost, quickness and easy visualization without requiring expensive instruments and tedious sample preparation.8,17–19 However, the fluorescent method is less beneficial due to auto-fluorescence issues from other species in the samples in comparison to the colorimetry.
Click chemistry or Cu+-catalyzed azide and alkyne cycloaddition (CuAAC) has been offered as a highly selective and sensitive assay for copper detection.4,6,20–24 In a Cu+-catalyzed click reaction, the 1,4-disubstituted triazoles are created from azides and alkyes under mild conditions while Cu+ can be formed in situ by the reduction of Cu2+ in the presence of a reductant such as sodium ascorbate.4,6 Moreover, a click chemistry incorporated with gold nanoparticles (AuNPs) has been developed for colorimetric detection of Cu2+. AuNPs are of great interest due to their high absorption coefficient which can provide high sensitivity of the method.19 Rapid and visual detection can be observed through color changes from the dispersion to aggregation of AuNPs in the presence of compound of interest.21,25 Xu et al. presented a DNA-functionalized AuNPs and click chemistry for Cu2+ assay using alkyne or azide strand and thiol tags as template through oligonucleotide hybridization.21 In this case, Cu2+ was only detected by red-to-purple color change at high temperature. Usage of temperature in reaction is not only costly but also makes the method inconvenient in operation. Wang and co-workers developed a lateral flow biosensor using streptavidin modified AuNPs and Cu+-catalyzed azide-DNA and alkyne/biotin–DNA.22 This biosensor is simple and cost-effective, but requires a long sample preparation time at least 45 min. Shen et al. reported a visual assay for Cu2+ by unmodified AuNPs with click ligation-dependent DNA switch based on azide-modified double-stranded DNA (dsDNA) and alkyne-modified single-stranded DNA (ssDNA).24 Nevertheless, salt is needed as an initiator for aggregation of AuNPs. From mentioned points, some spaces still remain for development of copper detection based on click chemistry.
Unfortunately, in a conventional click reaction, Cu+ is generated together with destructive reactive oxygen species (ROS) and high Cu+ is toxic to living cells.26,27 As a result, bio-sensing of Cu2+ is quite limited. DNAzyme, known as deoxyribozyme or DNA enzyme, has emerged as a promising agent for sensing metal ions due to its high catalytic efficiency in many chemical and biological reactions.26–30 Wang et al. developed the system of unimolecular Cu2+-dependent self-cleaving DNAzyme and unmodified AuNPs.27 In this assay, in the presence of Cu2+ and ascorbic acid, DNAzyme was cleaved into ssDNA that stabilized AuNPs under high salt-induced aggregation. Recently, Liu et al. reported a new “CLICK-17” DNAzyme, a 79 nt single-stranded oligonucleotide, with specific and highly catalytic capacity for the CuACC reaction.29 In this case, Cu2+ as low as 5–20 μM was used in the CLICK-17-catalyzed click process, thus producing very low level (∼μM) of Cu+. With this advantage, the catalytic CLICK-17 is possible to be used as bio-compatible click DNA for labelling the living cell surfaces29 or functionalizing electrode surfaces.30
In this work, we developed a highly specific and sensitive method for visual detection of Cu2+ using the aggregation of azide-polyethylene glycol 3000-functionalized gold nanoparticles (azide-AuNPs) induced by a click reaction catalyzed by Cu+ alone or a recently discovered DNAzyme, CLICK-17, with either Cu2+ or Cu+ (i.e., with sodium ascorbate (SA) as a reductant) under the help of linker DNA. Unlike previous colorimetric strategies where the linker DNA was normally made by annealing of two kinds of ssDNA with complementary sequences, in our experiments, only one kind of alkyne-ssDNA was utilized which has a unique sequence and thus can form alkyne-dsDNA with a palindromic sequence under room temperature. Consequently, no annealing procedure is required, making the method more convenient and economic than the conventional strategies of using two kinds of ssDNA followed by annealing. The formed alkyne-dsDNA was then used for linkage of azide-AuNPs and hence was called alkyne linker-DNA in the following. Interestingly, we achieved three click colorimetric assays for Cu2+ detection with different sensitivities and linear detection ranges, i.e., method A based on a colorimetric assay of Cu+-triggered click reaction between azide-AuNPs and alkyne linker-DNA (azide-AuNPs/alkyne linker-DNA/SA probe), method B using CLICK-17 with Cu2+ to catalyze the reaction between azide-AuNPs and alkyne linker-DNA (azide-AuNPs/alkyne linker-DNA/CLICK-17 probe) and method C relying on CLICK-17 and Cu+-catalyzed reaction between azide-AuNPs and alkyne linker-DNA with added SA as the reductant to produce Cu+ (azide-AuNPs/alkyne linker-DNA/CLICK-17/SA probe). Under the optimum condition, in the presence of Cu2+ with (i.e., method B and C) and without CLICK-17 (i.e., method A), a click reaction of azides and alkynes was induced between the azide-AuNPs and alkyne linker-DNA, leading to AuNPs aggregation and a color change for the solution from ruby red to bluish purple, with an absorption peak shifting from 525 to 570 nm. The detection sensitivity of methods A, B, C was compared and method C was found to be the best in view of its fastest color development and least usage of Cu2+ among these three methods. Finally, the specificity of method C was evaluated and the practicality of this method has also been successfully demonstrated by measuring Cu2+ concentration in real water samples.
2 Experimental
2.1 Chemicals
Unless otherwise indicated, all chemicals and reagents were purchased in their highest available purity and used without further purification. Deionized water (Millipore Milli-Q, 18.2 MΩ cm) was used for solution preparation. Azide-polyethylene glycol 3000 functionalized-gold nanoparticles (Azide-AuNPs) were purchased from Nanocs Inc. (New York, USA). Copper(II) sulfate, sodium ascorbate, N-2-hydroxyethylpiperazine-N′-2-ethanesulfonic acid (HEPES), MgCl2·6H2O and LiOH·H2O were purchased from Sigma-Aldrich. The alkyne modified oligonucleotides (alkyne-ssDNA) were synthesized by Takara Biotechnology Co. Ltd (Dalian, China) and purified by high performance liquid chromatography (HPLC). Before use, the alkyne modified oligonucleotides were dissolved in deionized water. The concentration of oligonucleotides was determined by measuring its absorption at the wavelength of 260 nm. The sequence of oligonucleotides is 5′-CH
C-AGACGGCCGTCT-3′. Alkyne-ssDNAs can form alkyne-dsDNA (i.e., alkyne linker-DNA) at room temperature without additional annealing process and were further used for linkage of azide-AuNPs. The CLICK-17 DNAzyme was synthesized by Sangon Biotech (Shanghai, China) and purified by HPLC. CLICK-17 sequence is 5′-GGATCGTCAGTGCATTGAGATTATTATGCAACTCTATGGGTCCACTCTGTGAATGTGACGGTGGTATCCGCAACGGGTA-3′ (79 nt).
2.2 Colorimetric assay for copper(II) detection
CLICK-17 was activated by heating to 95 °C for 5 min, then lowering temperature by 1 °C per minute to 4 °C and refolded in a buffer (50 mM of Li-HEPES, pH 7.4, 20 mM of MgCl2) before adding to the final reaction to ensure that it folded properly. All other experiments were performed at 23 °C. For the best condition of Cu2+ detection (method C), 20 μL of azide-AuNPs and 1 μL of alkyne linker-DNA were transferred into a 250 μL micro-centrifuge tube. Further 1 μL of CLICK-17, 1 μL of sodium ascorbate and 1 μL of appropriate concentrations of Cu2+ were consecutively added to the reaction solution. For the other two detection methods (i.e., method A and B), Cu2+ detection was conducted in the presence of either 1 μL of sodium ascorbate or 1 μL of CLICK-17. Notice here that the concentration of sodium ascorbate needs to be twice higher than that of Cu2+ for the best detection. The final reaction was carried out in a buffer (50 mM of Li-HEPES, pH 7.4, 20 mM of MgCl2) and the final concentrations of azide-AuNPs, alkyne linker-DNA and CLICK-17 in the solution were 2.1 nM, 0.6 μM and 4.0 μM, respectively. The reaction mixture was thoroughly mixed and incubated for 30 min at room temperature to ensure that the final color of the solution became stable. Subsequently, photographs were taken with a mobile phone and the UV-vis spectra were collected on a Nanodrop 2000 spectrophotometer (Thermo Fisher Scientific, USA) in the wavelength range between 400 and 800 nm. To quantify the contrast effect of visual resolution, the absorbance ratio (A570/A525) of 0.94 is selected as the threshold or critical reference value in all experiments.
3 Results and discussion
3.1 Principle of detection system for Cu2+
In this work, we detected Cu2+ based on a click colorimetric assay using azide-polyethylene glycol 3000-functionalized gold nanoparticles (azide-AuNPs) as a probe. The detection can be visually monitored by naked eyes and the detection sensitivity was quantitatively evaluated using UV-vis spectrophotometry. The strategy of the assay depends on the aggregation of azide-AuNPs under click chemistry catalyzed by Cu+ alone or by CLICK-17 with either Cu+ or Cu2+ (no added SA). Only one kind of alkyne-ssDNA was used to form alkyne-dsDNA (alkyne linker-DNA) at room temperature without additional annealing and the latter was further used to link azide-AuNPs. In the case of Cu+-catalyzed reaction, SA acted as a reductant for converting Cu2+ to Cu+.
Three detection systems using azide-AuNPs were carried out with different combinations of compound, i.e., alkyne linker-DNA, CLICK-17, SA and target Cu2+ as illustrated in Fig. 1A. Clearly, the aggregation of azide-AuNPs in the presence of Cu2+ can be seen in Fig. 1A(h–j) from the color change from ruby red to bluish purple. For convenience, we named the three detection methods in Fig. 1A(h–j) as method A, method B and method C, respectively. Additionally, the colloidal azide-AuNPs probe in its dispersed form has an absorption peak at ∼525 nm with ruby red in color (Fig. 1B(a)). After azide-AuNPs reacted with alkyne linker-DNA in the presence of CLICK-17, SA and Cu2+ (method C), the aggregation occurred and the absorption peak of AuNPs shifted from 525 to 570 nm, resulting in a bluish purple solution (Fig. 1B(b)). Similar phenomena were observed for method A and method B as well.
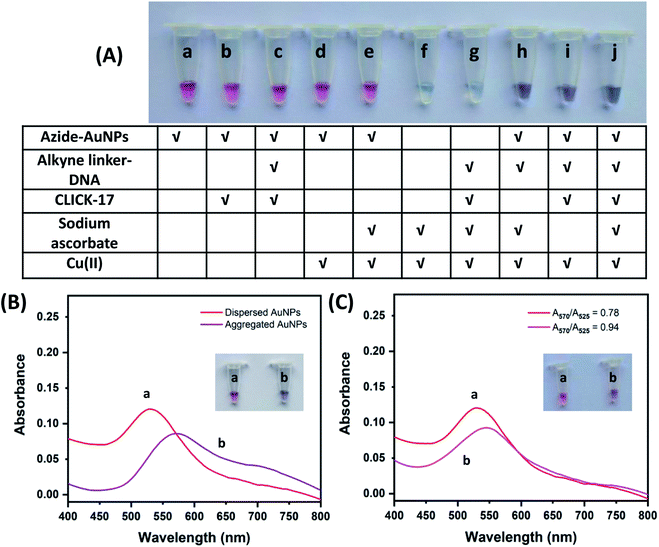 |
| Fig. 1 (A) Photograph of the color of AuNPs in the presence of other compounds (detection systems of a–j) after incubation for 30 min at room temperature. The √ in table shows the addition of compound into each system. The Cu2+ concentration used was 100, 1 and 0.5 μM for system (h–j), respectively. (B) UV-vis absorption spectra and photograph of (a) dispersed azide-AuNPs probe and (b) aggregated azide-AuNPs/alkyne linker-DNA/CLICK-17/SA probe after adding 300 nM Cu2+ (method C). (C) UV-vis absorption spectra for the critical value of azide-AuNPs probe (A570/A525 = 0.78) and azide-AuNPs/alkyne linker-DNA/CLICK-17/SA probe with 30 nM Cu2+ (A570/A525 = 0.94). | |
The principle of three detection methods was further illustrated in Fig. 2. In the absence of Cu2+, azide-AuNPs with alkyne linker-DNA remained dispersed and the solution showed the red-color (Fig. 2A). Even along with CLICK-17, the solution did not exhibit any color changes (Fig. 1A(c)). This is because azide-AuNPs and alkyne linker-DNA are both relatively stable in solution and do not aggregate easily, consistent with the previous report.20 For method A (azide-AuNPs/alkyne linker-DNA/SA) upon adding Cu2+ and SA as presented in Fig. 2B, Cu2+ was reduced to Cu+ which then catalyzed cycloaddition of –N3 (azide) of AuNPs and 5′-CH
C- (alkyne) of alkyne linker-DNA at both ends to form triazoles.4,6 AuNPs can then be bridged by the linker DNA. Here, Cu2+ and SA can also be claimed as a pre-catalyst for the reaction.31 As such the solution became bluish purple due to the aggregation of AuNPs.
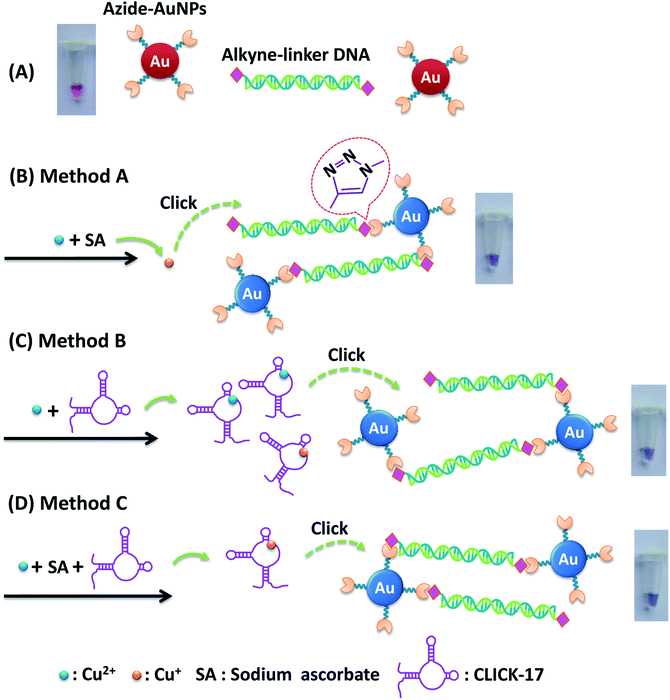 |
| Fig. 2 Schematic illustration of Cu2+ detection with (A) azide-AuNPs and alkyne linker-DNA, (B) azide-AuNPs/alkyne linker-DNA/SA probe (method A), (C) azide-AuNPs/alkyne linker-DNA/CLICK-17 probe (method B) and (D) azide-AuNPs/alkyne linker-DNA/CLICK-17/SA probe (method C). | |
In method B (azide-AuNPs/alkyne linker-DNA/CLICK-17) and method C (azide-AuNPs/alkyne linker-DNA/CLICK-17/SA), the CLICK-17 was introduced as a catalyst or co-catalyst for click chemistry with either Cu2+ or Cu+ (in the presence of SA). In Fig. 2C, AuNPs became aggregated through the CLICK-17-catalyzed cycloaddition of alkynes and azides with Cu2+ in a reaction involving no SA. It has been suggested that CLICK-17 DNAzyme has some active sites, which can provide binding sites for copper ions.29 Furthermore, HEPES buffer was used here to help providing a weak reducing environment.32,33 Finally, Cu2+ ions bound to CLICK-17's active sites to form a complex and then induced a click reaction and produced the triazole group. Notice here that, with the help of HEPES, it is possible that some Cu2+ ions could be further reduced to Cu+ that can help drive the CuACC reaction. The AuNPs can thus be linked together and the aggregation of AuNPs eventually led to the color change of the solution.
In Fig. 2D, with the treatment of CLICK-17, Cu2+ and SA into a solution of azide-AuNPs and alkyne linker-DNA (method C), the color of the AuNPs was found to change from ruby red to bluish purple. This result was comparable to the method B, except that CLICK-17 bound to Cu+ ions derived from in situ Cu2+ reduction by SA. Further, the aggregation was achieved. The CLICK-17 with SA plays a main role in the catalytic process, increasing the reaction rate in method C, thus the visual detection of Cu2+ by method C is faster than method B. In addition, with various concentrations of Cu2+ tested, we found that the method C had the highest sensitivity of visual detection and the detectable concentration of Cu2+ can be as low as 0.05 μM whereas the method A and B were sensitive at higher concentration. In addition, since in method C, Cu2+ was used very less, the resulting amount of Cu+ in the solution shall be small, i.e., ∼μM or nM level and is thus less toxic. The result also showed that the required reaction time of method C is about 15 min in contrast with the method B (15–30 min) and method A (at least 30 min). Though not as good as method C, the reaction in method B was still improved by CLICK-17 in comparison with that using Cu+ alone in a solution (method A). From these results, the method C was chosen as the best strategy to detect Cu2+ and further applied to the subsequent studies.
To evaluate the visual difference between ruby red (dispersed state) and bluish purple (aggregated state), we defined a threshold or critical value for the absorbance ratio (A570/A525) which corresponds to the situation at which the color change in the reaction is just visually discernable. When the value of A570/A525 is lower than this critical value, the color change cannot be distinguished or noticed by naked eyes. In the experiment, we made the color change under treatment with various concentrations of Cu2+ and the absorbance ratio (A570/A525) under each condition was then measured. The result was shown in Fig. 1C. We found that when the absorbance ratio (A570/A525) is below 0.94, the color difference between this solution and that without Cu2+ (the absorbance ratio is 0.78) can barely be told by eye. Therefore, in the following experiments, the value of 0.94 for the absorbance ratio (A570/A525) was selected as the criterion for visual discernibility.
3.2 Optimization of detection parameters
In order to achieve the best sensitivity for Cu2+ detection, the experimental parameters including concentrations of azide-AuNPs, alkyne linker-DNA, CLICK-17 as well as the ratio of sodium ascorbate and Cu2+ have to be optimized for method C (Fig. 3). During the optimization experiments, each parameter was studied while others were kept constant. Since the absorbance peaks of 525 and 570 nm represent the dispersed and aggregated state of AuNPs, respectively, we used the absorbance intensity ratio (A570/A525) as the signal to determine Cu2+, with the result reported here as mean ± standard deviation (n = 3).
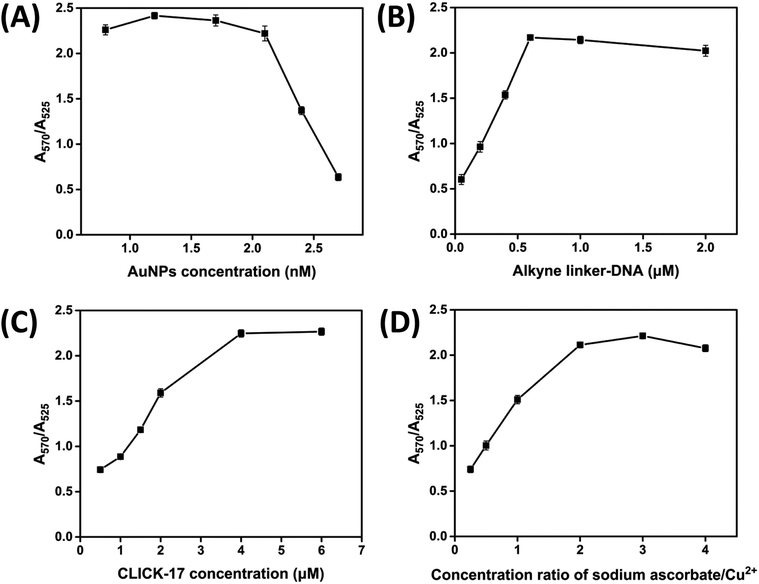 |
| Fig. 3 Effect of each parameter on the click reaction based on method C. (A) Effect of azide-AuNPs concentration. (B) Effect of alkyne linker-DNA concentration. (C) Effect of CLICK-17 concentration. (D) Effect of concentration ratio of sodium ascorbate to Cu2+. | |
3.2.1 Concentration of azide-AuNPs. One main key for a click reaction is the presence of azide group with alkynes and a catalyst in a solution. As can be seen in Fig. 1A(f) where SA and Cu2+ and Fig. 1A(g) where alkyne linker-DNA, CLICK-17, SA and Cu2+ were mixed without azide-AuNPs, we did not find any color change in the solution. Herein, we investigated the effect of azide-AuNP when its concentration varied from 0.8 to 2.7 nM. As shown in Fig. 3A, the absorbance ratio (A570/A525) remained constant up to 2.1 nM but quickly decreased when the concentration of azide-AuNP increased more. Hence 2.1 nM was selected as an optimal concentration for azide-AuNPs.
3.2.2 Concentration of alkyne linker-DNA. Fig. 3B shows the effect of varying alkyne linker-DNA concentration from 0.05 to 2.00 μM. As indicated by the absorbance ratio (A570/A525), the reaction became more efficient when the concentration of alkyne linker-DNA increased up to 0.6 μM and then gradually became less efficient at higher concentrations of linker-DNA. The decrease in absorbance ratio may be due to the fact that with increasing linker-DNA concentration more than 0.6 μM, the crosslink sites for alkyne and azide group on the surface of AuNPs were saturated. The left free DNA in solution may then have a weak effect on limiting AuNPs aggregation possibly by changing the charge density environment on AuNPs surfaces. As the concentration of alkyne linker-DNA of 0.6 μM led to the highest degree of the click reaction, it was chosen for subsequent experiments.
3.2.3 Concentration of CLICK-17. In general, DNAzyme is a class of catalytic DNA with its catalytic activity depending on cofactors such as metal ions or small molecules.26 Therefore it is selective for metal ions. CLICK-17 is one good DNAzyme candidate for copper detection, particularly due to its high efficiency and specificity in catalyzing CuAAC reaction.29,30 We noticed that in method B and C, when CLICK-17 was present, compared to method A (no CLICK-17), even with much lower amount of Cu2+ added, the faster color change of solution (ruby red to bluish purple) was observed. Method B and C thus exhibited higher sensitivity for copper detection than method A. We then investigated the effect of CLICK-17 concentration in the range of 0.5–6.0 μM as shown in Fig. 3C. The absorbance ratio (A570/A525) increased evidently with increased concentrations of CLICK-17 before it reached 4.0 μM. After that, the absorbance ratio reached a plateau. A similar result has also been reported by Gan et al.30 Therefore, here we selected 4.0 μM as an optimal concentration for CLICK-17.
3.2.4 Ratio of sodium ascorbate and Cu2+. To reduce Cu2+ to Cu+ for a click reaction, reducing reagents such as ascorbic acid or its sodium salt have often been used.4,29 In this part, we utilized sodium ascorbate as it is also compatible to most reagents used here. The effect of different concentration ratios (such as 0.25, 0.5, 1.0, 2.0, 3.0, 4.0) of sodium ascorbate to Cu2+ was then investigated. As can be seen in Fig. 3D, the absorbance ratio (A570/A525) increased quickly until the concentration ratio of SA to Cu2+ reached ∼2.0 and then it became stable, indicating that no significant improvement in Cu2+ reduction after that. We thus chose 2.0 as the optimal concentration ratio of SA to Cu2+ for our colorimetric experiments. As a result, the final concentration of sodium ascorbate was always twice of that of Cu2+ in the subsequent test.
3.3 Linearity, limit of detection and selectivity for Cu2+ detection
The different concentrations of Cu2+ were tested to find out a minimum Cu2+ concentration that can be detected by naked eyes and UV-vis spectrophotometry as shown in Fig. 4. Three detection methods, i.e., method A (azide-AuNPs/alkyne-linker DNA/SA), method B (azide-AuNPs/alkyne-linker DNA/CLICK-17) and method C (azide-AuNPs/alkyne-linker DNA/CLICK-17/SA), were studied and compared here. For these three methods, the absorbance ratio (A570/A525) has been plotted as a function of Cu2+ concentration ranged from 15 to 400 μM (Fig. 4A), 0.2 to 5.0 μM (Fig. 4B) and 0.05 to 5.00 μM (Fig. 4C), respectively. Notice that, although for all these three methods, the absorbance ratio exhibited a similar behavior and increased with the concentration of Cu2+, the linear range and sensitivity were quite different. The calibration curves of method A, B and C from linear fittings were then obtained over the Cu2+ concentration range of 15–100 μM (Fig. 4D), 0.20–1.25 μM (Fig. 4E), 0.05–0.5 μM (Fig. 4F), respectively, with the equations obtained as follows:
Method A: A570/A525 = 0.0095 Cu2+ + 0.9453 (R2 = 0.9941) |
Method B: A570/A525 = 0.7306 Cu2+ + 0.9289 (R2 = 0.9934) |
Method C: A570/A525 = 1.7873 Cu2+ + 1.0429 (R2 = 0.9961) |
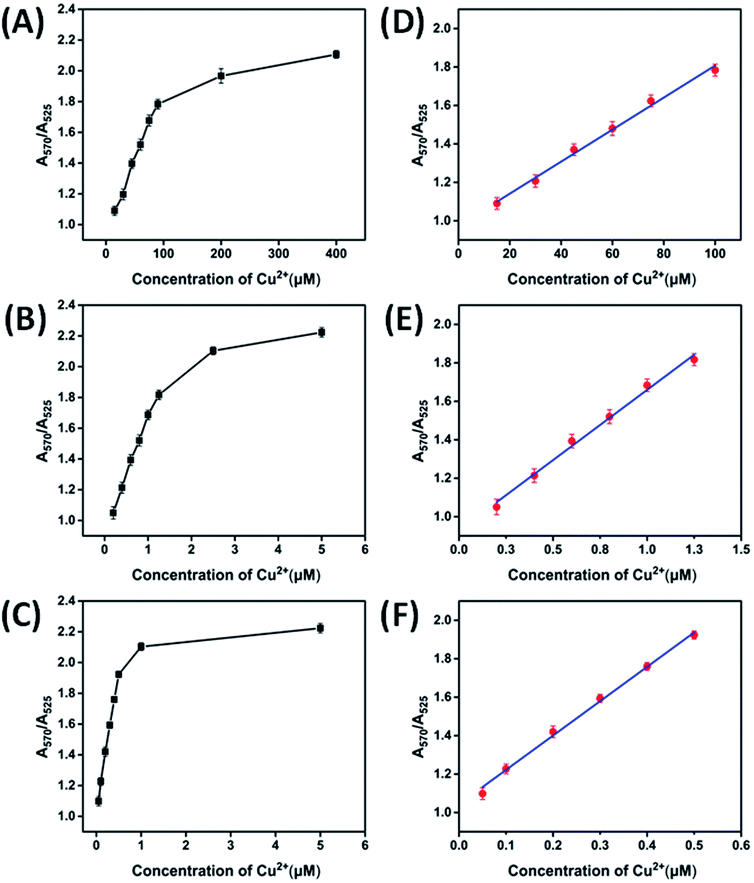 |
| Fig. 4 The absorbance ratio (A570/A525) versus Cu2+ concentration for (A) method A (azide-AuNPs/alkyne-linker DNA/SA), (B) method B (azide-AuNPs/alkyne-linker DNA/CLICK-17) and (C) method C (azide-AuNPs/alkyne-linker DNA/CLICK-17/SA). Linear calibration curves for (D) method A, (E) method B and (F) method C. | |
According to the signal to noise ratio of 3, the limit of detection (LOD) was estimated as 6.6 μM, 102 nM and 26.8 nM for method A, B and C, respectively. Compared to method A and method B, method C achieved an LOD about 246 times and 4 times lower, respectively, making it the most sensitive method for Cu2+ detection among these three methods. Therefore, we applied method C to the subsequent experiments to test its selectivity and practicality. It is also worth mentioning that LODs of the three detection methods were all found to be much lower than the maximum allowable concentration for Cu2+ in drinking water, according to the USEPA limit (20.4 μM)11 and the WHO limit (31.5 μM).12
The selectivity was evaluated to prove the robustness of the colorimetric detection of Cu2+ (Fig. 5). Real water samples may contain several metal ions that may interfere the visual detection. Thus, some interested metal ions, i.e., K+, Na+, Ag+, Mg2+, Ca2+, Zn2+, Ba2+, Mn2+, Cd2+, Fe3+ and Al3+ were evaluated using the same procedure as Cu2+ assay. Either a mixture of various metal ions or Cu2+ alone (final concentration of 2 μM each) were added into a colloidal solution of azide-AuNPs/alkyne-linker DNA/CLICK-17/SA probe (method C). As a positive control, metal ions and Cu2+ were also mixed together and added to another reaction solution of method C.
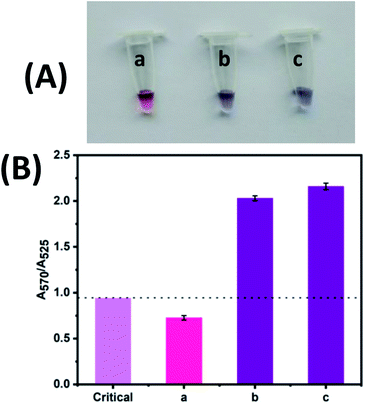 |
| Fig. 5 Selectivity result for Cu2+ detection. (A) Photograph and (B) absorbance ratio (A570/A525) of the assay based on azide-AuNPs/alkyne-linker DNA/CLICK-17/SA probe (method C) for (a) a mixture of metal ions (K+, Na+, Ag+, Mg2+, Ca2+, Zn2+, Ba2+, Mn2+, Cd2+, Fe3+, Al3+), (b) Cu2+ only and (c) a mixture of Cu2+ and the other eleven metal ions. The pink bar (in B) is the critical value for color discernibility which corresponds to an absorption ratio (A570/A525) of 0.94. Concentrations of other metal ions and Cu2+ are 2 μM. | |
The photograph and absorbance ratio (A570/A525) of Cu2+ compared with those of other ions were shown in Fig. 5. As mentioned before, the absorbance ratio (A570/A525) of 0.94 was used here as the reference value for visual discernibility (pink bar in Fig. 5B). When the absorbance ratio (A570/A525) is less than 0.94, it suggests that either no Cu2+ is in the solution or the concentration of Cu2+ is lower than the LOD of the method (i.e., 26.8 nM). As can be seen, the solution with only Cu2+ exhibits an obvious color change (Fig. 5A(b)) and the absorbance ratio is well above the critical value (violet bar labelled with b in Fig. 5B); while the mixture of all other ions except Cu2+ shows no color changes (Fig. 5A(a)) and the absorbance ratio is below the critical value (red bar labelled with a in Fig. 5B). Moreover, in the presence of Cu2+ with other ions, an absorption ratio (A570/A525) similar to that of Cu2+ alone were obtained (violet bar labelled with c in Fig. 5B). Thus, these results demonstrate that our method based on azide-AuNPs/alkyne-linker DNA/DNAzyme/SA probe is highly selective to Cu2+ even in the presence of various metal ions.
3.4 Determination of Cu2+ in real samples and recovery
To assess the potential applicability of our method based on azide-AuNPs/alkyne-linker DNA/CLICK-17/SA probe (method C), we quantified the concentrations of Cu2+ in a bottled mineral water. Different concentrations of Cu2+ in various water samples have been reported in the range of 70.8 nM to 15.6 μM.8,9,14 Our LOD is far below reported contents and thus, the proposed method can be used for a wide range of samples. For the mineral water samples chosen here, they were sonicated for 20 min to remove any dissolved gases prior to use and then analyzed with our colorimetric assay (method C).
As shown in Table 1, Cu2+ was not detected in this mineral water sample. Therefore, we further verified the accuracy of the method based on a recovery test by spiking concentrations of 230 and 400 nM Cu2+ to the prepared samples. A linear calibration curve of A570/A525 = 1.7873 Cu2+ + 1.0429 was used for determining Cu2+ in spiked samples. In Table 1, recoveries as high as 99.8% and 90.8% were achieved for 230 and 400 nM, respectively, confirming that this method can be applied for the analysis of Cu2+ in real samples. Our result also met the acceptable recovery values ranging from 80 to 110%, defined by AOAC standard.34
Table 1 Determination of Cu2+ in bottled mineral water samples using the method C (azide-AuNPs/alkyne-linker DNA/CLICK-17/SA probe)
Spiked Cu2+ concentration (nM) |
Found Cu2+ concentration (nM) (mean ± RSD, n = 3) |
Recoverya (%) |
RSDa (%) (n = 3) |
RSD = relative standard deviation. Recovery (%) = (C2 − C1) × 100/C0. C1 is the Cu2+ concentration found in an un-spiked sample. C2 is the Cu2+ concentration found in a spiked sample. C0 is the Cu2+ concentration spiked to the test sample. |
0 |
Not detectedb |
— |
— |
230d |
229.5 ± 3.4c |
99.8 |
3.4 |
400d |
363.2 ± 2.8c |
90.8 |
2.8 |
3.5 Comparison of proposed assay with previously reported work for Cu2+
A comparison of detection method, linear range and LOD of our method and reported methods is shown in Table 2. Our methods have wide linear ranges comparable to other methods with the reaction time significantly shortened from 24 h to 15–30 min.21,24,35 The methods also proceed at room temperature, similar to other reports.24,35–37,39,40 The result shows that method C has a lower LOD (∼26.8 nM) than method A and B as well as most previous aggregation-based and fluorescence-based methods.21,24,35–41 A silver nanoclusters-based fluorescence method can sense very low concentration of Cu2+ (10 nM) but is quite sensitive to temperature and requires an UV-light excitation for fluorescence detection.42 Other click colorimetric sensors require annealing of two different ssDNAs to form dsDNA, but our design allows direct interaction of alkyne-ssDNAs under room temperature. The annealing procedure can thus be skipped. Last but not the least, the present assay also offers other advantages in terms of specificity, robustness and low toxic Cu+ produced in μM to nM range due to the use of highly efficient catalyst CLICK-17 and hence low Cu2+ concentration in the reaction.
Table 2 Comparison of proposed method with previously reported methods for Cu2+
Media/probe |
Detection method |
Linear range |
LOD (nM) |
Reference |
AgNCs = silver nanoclusters; CQDs = carbon quantum dots. |
Azide/alkyne-AuNPs |
UV-vis |
50–500 μM |
50 000 |
35 |
Alkyne/azide DNA-AuNPs |
UV-vis |
20–100 μM |
20 000 |
21 |
L-Cysteine-AuNPs |
UV-vis |
10–500 μM |
10 000 |
36 |
Azide-AuNPs/alkyne linker-DNA/SA |
UV-vis |
15–100 μM |
6600 |
Method A |
Azide-AuNPs/1,4-diethynylbenzene |
UV-vis |
1.8–200 μM |
1800 |
37 |
N-(5-Nitro-2-pyridyl)-1,2-ethanediamine/4-(diethylamino)-2-hydroxybenzaldehyde-Cu2+ complex |
UV-vis |
5–40 μM |
880 |
38 |
AuNPs/azide-dsDNA/alkyne-ssDNA |
UV-vis |
0.5–10 μM |
250 |
24 |
Azide-coumarin/N-propargylacetamide |
Fluorescence |
— |
200 |
39 |
Azide-AuNPs/alkyne linker-DNA/CLICK-17 |
UV-vis |
0.20–1.25 μM |
102 |
Method B |
3-Azido-7-hydroxycoumarin/propargyl alcohol |
Fluorescence |
0.25–2.5 μM |
80 |
40 |
Alkyne/azide dumbbell-DNA/exonuclease cleavage |
Fluorescence |
0.1–10 μM |
39 |
41 |
Hyperbranched polyethyleneimine-AgNCsa |
Fluorescence |
10 nM to 7.7 μM |
10 |
42 |
Branched poly(ethylenimine)-CQDsa |
Fluorescence |
10–1100 nM |
6 |
8 |
Azide-AuNPs/alkyne linker-DNA/CLICK-17/SA |
UV-vis |
50–500 nM |
26.8 |
Method C |
4 Conclusions
The novel selective colorimetric assay for Cu2+ is developed based on a newly discovered DNAzyme, CLICK-17, and click reaction between azide-AuNPs and alkyne linker-DNA. We proposed three methods, allowing the ruby red to bluish purple colorimetric detection with naked eyes. The color change was confirmed in the UV-vis absorption spectra and the aggregation degree of AuNPs was assessed by the ratio of absorbance at 570 and 525 nm (A570/A525). Cu+ catalyzed the cycloaddition of azide-AuNPs and alkyne linker-DNA (method A) with LOD of 6.6 μM. CLICK-17 can mediate click chemistry in the presence of Cu2+ (method B) and Cu+ (method C, added SA), resulting in lower LODs of 102 nM and 26.8 nM, respectively. Method C is preferred in terms of speediness and highest sensitivity. Specificity of our method is excellent towards Cu2+. Aside from its application to environmental analysis, we expect that the assay can be useful for Cu2+ detection in food areas, other bioassays and on-site applications in combination with lab-on-chip, microfluidic platforms etc.
Author contributions
Z. Z. proposed the idea; Z. Z., J. M. and T. R. designed the experiments; W. Y. performed the experiments and data analysis; W. Y., T. R., J. M. and Z. Z. wrote the manuscript; J. M. and T. R. supervised the project.
Conflicts of interest
There are no conflicts to declare.
Acknowledgements
This research was sponsored by the funding for the Science and Technology Planning Project of Guangdong Province, China (2018A050506034), and the Natural Science Foundation of Guangdong Province, China (2017A030310085). The Division of Physical Science and the Center of Excellence for Innovation in Chemistry (PERCH-CIC), Ministry of Higher Education, Science, Research and Innovation, Faculty of Science, Prince of Songkla University are also acknowledged.
References
- S. Lutsenko, C. Washington-Hughes, M. Ralle and K. Schmidt, Copper and the brain noradrenergic system, J. Biol. Inorg Chem., 2019, 24, 179–1188 CrossRef.
- E. L. Que, D. W. Domaille and C. J. Chang, Metals in neurobiology: Probing their chemistry and biology with molecular imaging, Chem. Rev., 2008, 108, 1517–1549 CrossRef CAS PubMed.
- D. W. Domaille, E. L. Que and C. J. Chang, Synthetic fluorescent sensors for studying the cell biology of metals, Nat. Chem. Biol., 2008, 4, 168–175 CrossRef CAS.
- M. Tarnowska and T. Krawczyk, Click chemistry as a tool in biosensing systems for sensitive copper detection, Biosens. Bioelectron., 2020, 169, 112614 CrossRef CAS.
- D. J. Waggoner, T. B. Bartnikas and J. D. Gitlin, The role of copper in neurodegenerative disease, Neurobiol. Dis., 1999, 6, 221–230 CrossRef CAS.
- L. Liu, Z. Fang, X. Zheng and D. Xi, Nanopore-based strategy for sensing of copper(II) ion and real-time monitoring of a click reaction, ACS Sens., 2019, 4, 1323–1328 CrossRef PubMed.
- D. A. Montero, C. Arellano and M. Pardo, et al., Antimicrobial properties of a novel copper based composite coating with potential for use in healthcare facilities, Antimicrob. Resist. Infect. Control, 2019, 8, 1–10 CrossRef.
- Y. Dong, R. Wang and G. Li, et al., Polyamine-functionalized carbon quantum dots as fluorescent probes for selective and sensitive detection of copper ions, Anal. Chem., 2012, 84, 6220–6224 CrossRef CAS.
- M. Soleimani and Z. H. Siahpoosh, Determination of Cu(II) in water and food samples by Na+-cloisite nanoclay as a new adsorbent: Equilibrium, kinetic and thermodynamic studies, J. Taiwan Inst. Chem. Eng., 2016, 59, 413–423 CrossRef CAS.
- N. Amphalop, N. Suwantarat and T. Prueksasit, et al., Ecological risk assessment of arsenic, cadmium, copper, and lead contamination in soil in e-waste separating household area, Buriram province, Thailand, Environ. Sci. Pollut. Res., 2020, 27, 44396–44411 CrossRef PubMed.
- USEPA, Drinking Water Requirements for States and Public Water Systems, https://www.epa.gov/dwreginfo/lead-and-copper-rule, accessed 26. 03. 2021 Search PubMed.
- WHO 2011, Guidelines for Drinking-water Quality, the World Health Organization, 4th edn, Geneva, Switzerland Search PubMed.
- M. Žemberyová, J. Barteková, M. Závadská and M. Šišoláková, Determination of bioavailable fractions of Zn, Cu, Ni, Pb and Cd in soils and sludges by atomic absorption spectrometry, Talanta, 2007, 71, 1661–1668 CrossRef.
- N. Goudarzi, Determination of trace amounts of copper in river and sea water samples by flame atomic absorption spectrometry (FAAS) after cloud-point preconcentration, J. Braz. Chem. Soc., 2007, 18(7), 1348–1352 CrossRef CAS.
- T. Kato, S. Nakamura and M. Morita, Determination of nickel, copper, zinc, silver, cadmium and lead in seawater by isotope dilution inductively coupled plasma mass spectrometry, Anal. Sci., 1990, 6, 623–626 CrossRef CAS.
- L. Cui, J. Wu and H. Ju, Electrochemical sensing of heavy metal ions with inorganic, organic and bio-materials, Biosens. Bioelectron., 2015, 63, 276–286 CrossRef CAS.
- D. Udhayakumari, S. Naha and S. Velmathi, Colorimetric and fluorescent chemosensors for Cu2+. A comprehensive review from the years 2013–15, Anal. Methods, 2017, 9, 552–578 RSC.
- J. Y. Noh, G. J. Park and Y. J. Na, et al., A colorimetric “naked-eye” Cu(II) chemosensor and pH indicator in 100% aqueous solution, Dalton Trans., 2014, 43, 5652–5656 RSC.
- T. Lou, L. Chen and Z. Chen, et al., Colorimetric detection of trace copper ions based on catalytic leaching of silver-coated gold nanoparticles, ACS Appl. Mater. Interfaces, 2011, 3, 4215–4220 CrossRef CAS PubMed.
- Q. Tu, L. Zhao and X. Han, et al., A visualized method for Cu2+ ion detection by self-assembling azide functionalized free graphene oxide using click chemistry, RSC Adv., 2016, 6, 95628–95632 RSC.
- X. Xu, W. L. Daniel, W. Wei and C. A. Mirkin, Colorimetric Cu2+ detection using DNA-modified gold-nanoparticle aggregates as probes and click chemistry, Small, 2010, 6, 623–626 CrossRef CAS.
- D. Wang, C. Ge and L. Wang, et al., A simple lateral flow biosensor for the rapid detection of copper(II) ions based on click chemistry, RSC Adv., 2015, 5, 75722–75727 RSC.
- Z. Yao, Y. Yang and X. Chen, et al., Visual detection of copper(II) ions based on an anionic polythiophene derivative using click chemistry, Anal. Chem., 2013, 85, 5650–5653 CrossRef CAS PubMed.
- Q. Shen, W. Li and S. Tang, et al., A simple “clickable” biosensor for colorimetric detection of copper(II) ions based on unmodified gold nanoparticles, Biosens. Bioelectron., 2013, 41, 663–668 CrossRef CAS PubMed.
- K. Zhu, Y. Zhang and S. He, et al., Quantification of proteins by functionalized gold nanoparticles using click chemistry, Anal. Chem., 2012, 84, 4267–4270 CrossRef CAS.
- H. Fan, X. Zhang and Y. Lu, Recent advances in DNAzyme-based gene silencing, Sci. China Chem., 2017, 60, 591–601 CrossRef CAS.
- Y. Wang, F. Yang and X. Yang, Label-free colorimetric biosensing of copper(II) ions with unimolecular self-cleaving deoxyribozymes and unmodified gold nanoparticle probes, Nanotechnology, 2010, 21, 205502 CrossRef.
- P. Zuo, B.-C. Yin and B.-C. Ye, DNAzyme-based microarray for highly sensitive determination of metal ions, Biosens. Bioelectron., 2009, 25, 935–939 CrossRef CAS PubMed.
- K. Liu, P. K. Lat, H.-Z. Yu and D. Sen, CLICK-17, a DNA enzyme that harnesses ultra-low concentrations of either Cu+ or Cu2+ to catalyze the azide-alkyne ‘click’ reaction in water, Nucleic Acids Res., 2020, 48, 7356–7370 CAS.
- N. Gan, K. Liu and L. Qi, et al., DNAzyme-catalyzed click chemistry for facilitated immobilization of redox functionalities on self-assembled monolayers, J. Phys. Chem. C, 2020, 124, 19083–19090 CrossRef CAS.
- L. Liang and D. Astruc, The copper(I)-catalyzed alkyne-azide cycloaddition (CuAAC) “click” reaction and its applications. An overview, Coord. Chem. Rev., 2011, 255, 2933–2945 CrossRef CAS.
- S. Saverot, X. Geng and W. Leng, et al., Facile, tunable, and SERS-enhanced HEPES gold nanostars, RSC Adv., 2016, 6, 29669–29673 RSC.
- D. H. M. Dam, J. H. Lee and P. N. Sisco, et al., Direct observation of nanoparticle-cancer cell nucleus interactions, ACS Nano, 2012, 6, 3318–3326 CrossRef CAS.
- AOAC 2016, Guidelines for Standard Method Performance Requirements. AOAC Official Methods of Analysis, AOAC International, Appendix F, vol. 1–17, Gaithersburg, MD, USA Search PubMed.
- Y. Zhou, S. Wang, K. Zhang and X. Jiang, Visual detection of copper(II) by azide- and alkyne-functionalized gold nanoparticles using click chemistry, Angew. Chem., Int. Ed., 2008, 47, 7454–7456 CrossRef CAS.
- W. Yang, J. J. Gooding and Z. He, et al., Fast colorimetric detection of copper ions using L-cysteine functionalized gold nanoparticles, J. Nanosci. Nanotechnol., 2007, 7, 712–716 CrossRef CAS.
- C. Hua, W. H. Zhang and S. R. M. De Almeida, et al., A novel route to copper(II) detection using ‘click’ chemistry-induced aggregation of gold nanoparticles, Analyst, 2012, 137, 82–86 RSC.
- J. H. Kang, S. Y. Lee, H. M. Ahn and C. Kim, Sequential detection of copper(II) and cyanide by a simple colorimetric chemosensor, Inorg. Chem. Commun., 2016, 74, 62–65 CrossRef CAS.
- S. Park and H.-J. Kim, Highly selective and sensitive fluorescence turn-on probe for a catalytic amount of Cu(I) ions in water through the click reaction, Tetrahedron Lett., 2012, 53, 4473–4475 CrossRef CAS.
- C. Wang, L. Lu and W. Ye, et al., Fluorescence sensor for Cu(II) in the serum sample based on click chemistry, Analyst, 2014, 139, 656–659 RSC.
- Y. Qin, M. Li and Y. Yang, et al., A unimolecular DNA fluorescent probe for determination of copper ions based on click chemistry, RSC Adv., 2020, 10, 6017–6021 RSC.
- Z. Yuan, N. Cai and Y. Du, et al., Sensitive and selective detection of copper ions with highly stable polyethyleneimine protected silver nanoclusters, Anal. Chem., 2014, 86, 419–426 CrossRef CAS PubMed.
Footnote |
† These authors contributed equally to this paper. |
|
This journal is © The Royal Society of Chemistry 2021 |
Click here to see how this site uses Cookies. View our privacy policy here.