DOI:
10.1039/D1RA04207F
(Paper)
RSC Adv., 2021,
11, 25639-25645
Synthesis of novel 2-methyl-3-furyl sulfide flavor derivatives as efficient preservatives†
Received
31st May 2021
, Accepted 17th July 2021
First published on 26th July 2021
Abstract
Foodborne microbial infestation seriously threatens food security, and the development of low-risk food preservatives is highly needed in food production. For discovering novel flavor molecules with antiseptic function, novel 2-methyl-3-furyl sulfide flavor derivatives were synthesized and evaluated. A wide range of 2-methyl-3-furyl sulfide derivatives were synthesized by reactions of 2-methyl-3-furyl disulfide with cyclic ethers, amides, ketones, and epoxides. All of these compounds have special aroma characteristics and low aroma thresholds. The antimicrobial activity of these compounds against test foodborne bacterial or fungal strains (Escherichia coli, Bacillus subtilis, Staphylococcus aureus, Salmonella paratyphi, Listeria monocytogenes, Vibrio parahemolyticus, Penicillium italicum, Aspergillus niger, Mucor racemosus, Rhizopus oryzae) was examined. It was found that fifteen compounds (3a, 3b, 3d, 3e, 3f, 3g, 3h, 3i, 3j, 3k, 3l, 3m, 5a, 5b, 5f) have antimicrobial activity against different foodborne bacterial or fungal strains. Significantly, the antimicrobial activity of the flavor compounds (3b, 3d, 3e, 3i, 3j, 3l, 3m) is better than that of the control group (penicillin, amphotericin B and thiram), and they are promising preservatives for food production.
Introduction
Globally, foodborne microbial infections are among the most serious problems that threaten public health.1,2 Foodborne microbial infections commonly occur during the production, processing, packaging, distribution, and consumption of foods, causing food to spoil and deteriorate, affecting food quality and safety, threatening human health, and causing death in serious cases.3,4 Foodborne microorganisms mainly include bacteria (Escherichia coli, Staphylococcus aureus, Salmonella spp., Listeria monocytogenes, Vibrio Parahemolyticus, etc.) and fungi (Aspergillus, Penicillium, Fusarium, etc.).5–9 The effective manual control of these foodborne microorganisms is the use of chemical preservatives due to their rapid response to foodborne microorganisms.10 However, the long-term abuse of chemical preservatives has led to the emergence of resistance in pathogenic organisms and may pose a risk to human health.11 Therefore, the development of novel, highly-efficient, and environmentally benign agents against foodborne microorganisms remains a daunting task in preservative sciences.
2-Methyl-3-furyl sulfide spice compounds are a kind of important sulfur-containing spice compounds due to their small dosage, strong characteristics and low fragrance threshold.12,13 2-Methyl-3-furyl sulfide spice compounds play an important role in the condiment field. In addition, 2-methyl-3-furyl sulfide spice compounds are also important fine chemical raw materials and organic synthesis intermediates, which are widely used in food, chemicals, pharmaceuticals, and agriculture.14 In recent years, some studies have shown that 2-methyl-3-furyl sulfide spice compounds possess a variety of biological functions, including anticancer and antibacterial properties (Fig. 1).15–17 Zhang et al. reported that methyl 2-methyl-3-furyl disulphide, bis (2-methyl-3-furyl)disulphide, methyl furfuryl disulphide and difurfuryl disulfide were able to induce DNA breakage to differing degrees in human leukemia Jurkat cells, and also induce reactive oxygen species production and caspase-3 activation, leading to apoptosis of leukemia Jurkat cells.15 Hou et al. reported that methyl 2-methyl-3-furyl disulfide could inhibit the formation of biofilm and the expression of the quorum sensing gene luxI, thereby inhibiting the growth of Hafnia alvei.16 Nicolaou et al. reported that bis (2-methyl-3-furyl)disulfide exhibits in vitro antibacterial activity against methicillin-resistant Staphylococcus aureus (MRSA).17 In view of these reports, we reasoned that the coupling of 2-methyl-3-furyl sulfide with functional fragments may produce novel spices with antimicrobial activity. To our knowledge, studies on the antimicrobial activity of 2-methyl-3-furyl sulfide flavor derivatives on foodborne microbial is limited. Therefore, design and synthesis of 2-methyl-3-furyl sulfide derivatives with unique flavor and multiple functions such as antimicrobial and sterilization is of great research value.
 |
| Fig. 1 Examples for functional furyl sulfide spice compounds selected from FEMA (Flavor and Extract Manufacturers Association of the United States). | |
Results and discussion
In this study, a series of 2-methyl-3-furyl sulfide derivatives with fragrance and flavor were synthesized by reactions of 2-methyl-3-furyl disulfide with cyclic ethers, amides, ketones, and epoxides, respectively (Scheme 1).18–21 These synthesized 2-methyl-3-furyl sulfide derivatives are expected to be volatile and may exhibit different bioactivities, because tetrahydrofuran,22 1,4-dioxane,23 amides,24 ketones,23 and epoxides,25 are important pharmacophore for drug design. Derivatives 3a–3i were synthesized by the C–H sulfurization of 2-methyl-3-furyl disulfide with cyclic ethers and amides, respectively.18,19 The reaction of 2-methyl-3-furyl disulfide with formamide would yield a mixture of isomers, e.g. compounds 3d and 3e, compounds 3g and 3h. Compounds 3j–3m were prepared by the nucleophilic substitution of 2-methyl-3-furyl disulfide with ketones in the presence of alkaline under heating conditions.20 Compounds 5a–5f were synthesized by ring-opening reaction of 2-methyl-3-furyl disulfide and epoxides.21 The reaction of 2-methyl-3-furyl disulfide with 2-phenyloxirane produce a mixture of compound 5e and 5f that can be isolated. It is noteworthy that, due to the rotation hindrance of the amide bond, the 1H NMR and 13C NMR of compounds 3c, 3d, 3d and 3i appear two sets of signal peaks.
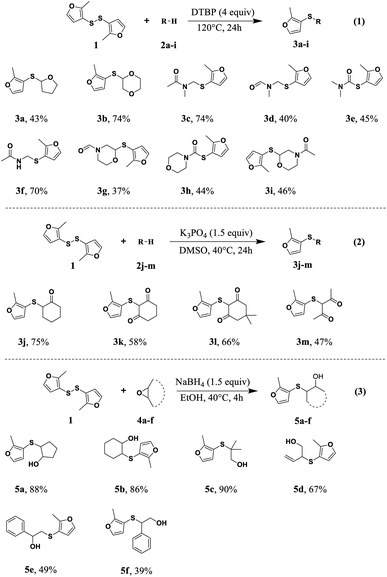 |
| Scheme 1 Synthesis of 2-methyl-3-furyl sulfide derivatives. | |
These synthetic 2-methyl-3-furyl sulfide derivatives have special aroma characteristics and low aroma thresholds (<5 μg mL−1). The aroma characteristics are mainly onion, garlic, nut, mushroom, radish or roast meat. The odor evaluation results are shown in Table 1. This is also in line with the characteristics of low aroma threshold and strong characteristics of sulfur-containing spice compounds, especially thioether spice compounds.12 Therefore, we speculate that the 2-methyl-3-furyl sulfide skeleton may be the structure that makes these derivatives produce unique odors. All synthetic 2-methyl-3-furyl sulfide flavor derivatives have relatively medium molecular weights (180–260) and their volatility are moderate, enabling to prolong the flavoring lifetime and improve the flavoring quality.26
Table 1 Odor evaluation of 2-methyl-3-furyl sulfide derivatives
Compound |
Odor characteristics |
Threshold (μg mL−1) |
3a |
Onion, garlic, roast meat |
0.4 |
3b |
Onion, garlic, roast meat |
0.4 |
3c |
Mushroom, nut, roast meat |
0.2 |
3d |
Mushroom, nut, roast meat |
0.4 |
3e |
Mushroom, nut, roast meat |
3.0 |
3f |
Mushroom, nut, roast meat |
0.4 |
3g |
Garlic, mushroom, roast meat |
0.2 |
3h |
Garlic, mushroom, roast meat |
0.4 |
3i |
Onions, garlic, nut |
3.0 |
3j |
Garlic, mushroom, roast meat |
0.4 |
3k |
Garlic, mushroom, roast meat |
0.4 |
3l |
Garlic, mushroom, roast meat |
0.4 |
3m |
Onion, mushroom, nut |
0.2 |
5a |
Onion, garlic, nut |
0.4 |
5b |
Onions, garlic, nut |
3.0 |
5c |
Onions, garlic, nut |
0.8 |
5d |
Onion, metal, nut |
0.4 |
5e |
Onion, garlic, radish |
3.0 |
5f |
Onion, garlic, radish |
0.8 |
The in vitro antimicrobial activities of the 2-methyl-3-furyl sulfide flavor derivatives were evaluated by disk diffusion test. This test was based on the measurement of the inhibition zone on a bacterial and fungal cells layer, after the spreading in the culture medium of the compounds to be tested. The antimicrobial activities of these compounds were determined by evaluating the dimension of the inhibition zone (mm in diameter). Among them, penicillin, amphotericin B and thiram represented the positive control. Bacterial and fungal strains include Escherichia coli (E. coli), Bacillus subtilis (B. subtilis), Staphylococcus aureus (S. aureus), Salmonella paratyphi (S. paratyphi), Listeria monocytogenes (L. monocytogenes), Vibrio parahemolyticus (V. parahemolyticus), Penicillium italicum (P. italicum), Aspergillus niger (A. niger), Mucor racemosus (M. racemosus) and Rhizopus oryzae (R. oryzae). The result of primary screening can be found in ESI file (Table S3†). The majority of these compounds showed excellent antimicrobial activity against a variety of bacterial or fungal strains. Compounds (3b, 3d, 3e, 3g, 3i, 3j, 3l, 5a, 5f) show significant antimicrobial activity against E. coli. Compounds (3a, 3b, 3f, 3g, 3h, 3i, 3j, 3l, 5f) show excellent antimicrobial activity against B. subtilis. Compounds (3a, 3b, 3d, 3g, 3h, 3i, 3j, 3k, 3l, 3m, 5a, 5f) show significant antimicrobial activity against S. aureus. Compounds (3a, 3b, 3d, 3f, 3g, 3h, 3i, 3l, 5f) show significant antimicrobial activity against S. paratyphi. Compounds (3b, 3f, 3g, 3h, 3i, 3l, 5f) show significant antimicrobial activity against L. monocytogenes. Compounds (3f, 3h, 3l) show significant antimicrobial activity against V. parahemolyticus. Compounds (3a, 3b, 3d, 3e, 3g, 3h, 3i, 3l, 3m, 5b, 5f) show significant antimicrobial activity against P. italicum. Compounds (3a, 3e, 3g, 3h, 3i, 3j, 3l, 3m) show significant antimicrobial activity against A. niger. Compounds (3a, 3b, 3d, 3f, 3g, 3h, 3i, 3j, 3k, 3l, 3m, 5f) show significant antimicrobial activity against M. racemosus. Compounds (3a, 3d, 3e, 3g, 3h, 3i, 3j, 3l, 3m, 5f) show significant antimicrobial activity against R. oryzae.
Based on these result of the primary screening, 96-well microtiter plates were used to determine the minimal inhibitory concentration (MIC) of these compounds with excellent antibacterial or antifungal activity (Table 2). Overall, the test compounds showed excellent antimicrobial activity, and some compounds showed better activity than those of the control group. For example, the antimicrobial activity of 3a against A. niger (MIC = 12.5 μg mL−1) is equal to that of thiram. The antimicrobial activity of 3b against B. subtilis (MIC = 6.25 μg mL−1) and S. paratyphi (1.56 μg mL−1) is better than that of penicillin, and is equal to that of penicillin when against L. monocytogenes (MIC = 3.125 μg mL−1). The antimicrobial activity of 3d against P. italicum (MIC = 3.125 μg mL−1) and M. racemosus (MIC = 1.56 μg mL−1) is better than that of amphotericin B and thiram. The antimicrobial activity of 3e on P. italicum (MIC = 1.56 μg mL−1) is better than that of amphotericin B and thiram. Compound 3g has the same antimicrobial activity as amphotericin B against A. niger (MIC = 6.25 μg mL−1). The antimicrobial activity of 3h against P. italicum (MIC = 12.5 μg mL−1) and M. racemosus (MIC = 3.125 μg mL−1) is equal to that of thiram. The antimicrobial activity of 3i to B. subtilis (MIC = 6.25 μg mL−1) is better than that of penicillin, to S. aureus (MIC = 6.25 μg mL−1) is equal to that of penicillin, to R. oryzae (MIC = 1.56 μg mL−1) is better than that of amphotericin B and thiram, and to P. italicum (MIC = 6.25 μg mL−1) and A. niger (MIC = 6.25 μg mL−1) is equal to that of amphotericin B. The antimicrobial activity of 3j against S. aureus (MIC = 1.56 μg mL−1) is better than that of penicillin, and to E. coli (MIC = 6.25 μg mL−1) and B. subtilis (MIC = 12.5 μg mL−1) is equal to that of penicillin. The antimicrobial activity of 3l on E. coli (MIC = 3.125 μg mL−1) and S. aureus (MIC = 1.56 μg mL−1) is better than that of penicillin, to M. racemosus (MIC = 1.56 μg mL−1) is better than that of amphotericin B and thiram, and to B. subtilis (MIC = 12.5 μg mL−1) is equal to that of penicillin. The antimicrobial activity of 3m against M. racemosus (MIC = 1.56 μg mL−1) is better than that of amphotericin B and thiram. The antimicrobial activity of 5f against P. italicum (MIC = 12.5 μg mL−1) and M. racemosus (MIC = 3.125 μg mL−1) is equal to that of thiram.
Table 2 MIC of 2-methyl-3-furyl sulfide flavor derivatives on foodborne bacteria and fungia
Compounds |
MIC (μg mL−1) |
Bacteria |
Fungi |
EC |
BS |
SA |
SP |
LM |
VP |
PI |
AN |
MR |
RO |
EC = E. coli. BS = B. subtilis. SA = S. aureus. SP = S. paratyphi. LM = L. monocytogenes. VP = V. parahemolyticus. PI = P. italicum. AN = A. niger. MR = M. racemosus. RO = R. oryzae. Pcn = penicillin. Amb = amphotericin B. TMTD = thiram. — = no test. |
3a |
— |
25 |
12.5 |
25 |
— |
— |
25 |
12.5 |
6.25 |
12.5 |
3b |
25 |
6.25 |
25 |
1.56 |
3.125 |
— |
>25 |
— |
>25 |
— |
3d |
>25 |
— |
>25 |
>25 |
— |
— |
3.125 |
— |
1.56 |
>25 |
3e |
>25 |
— |
— |
— |
— |
— |
1.56 |
>25 |
— |
>25 |
3f |
— |
>25 |
— |
>25 |
>25 |
>25 |
— |
— |
12.5 |
— |
3g |
25 |
25 |
25 |
12.5 |
6.25 |
— |
25 |
6.25 |
6.25 |
>25 |
3h |
— |
>25 |
>25 |
12.5 |
25 |
25 |
12.5 |
12.5 |
3.125 |
25 |
3i |
12.5 |
6.25 |
6.25 |
>25 |
>25 |
— |
6.25 |
6.25 |
25 |
1.56 |
3j |
6.25 |
12.5 |
1.56 |
— |
— |
— |
— |
>25 |
>25 |
12.5 |
3k |
— |
— |
>25 |
— |
— |
— |
— |
— |
>25 |
— |
3l |
3.125 |
12.5 |
1.56 |
12.5 |
25 |
25 |
>25 |
>25 |
1.56 |
>25 |
3m |
— |
— |
25 |
— |
— |
— |
>25 |
>25 |
1.56 |
12.5 |
5a |
>25 |
— |
>25 |
— |
— |
— |
— |
— |
— |
— |
5b |
— |
— |
— |
— |
— |
— |
>25 |
— |
— |
— |
5f |
>25 |
>25 |
>25 |
>25 |
>25 |
— |
12.5 |
— |
3.125 |
25 |
Pcn |
6.25 |
12.5 |
6.25 |
3.125 |
3.125 |
12.5 |
— |
— |
— |
— |
Amb |
— |
— |
— |
— |
— |
— |
6.25 |
6.25 |
3.125 |
3.125 |
TMTD |
— |
— |
— |
— |
— |
— |
12.5 |
12.5 |
3.125 |
3.125 |
Based on the above results, we analyze that the antimicrobial activity of the flavor derivatives may be caused by the following reasons. Since thioether is a type of important pharmacophore,27,28 the 2-methyl-3-furyl sulfide motif mainly contribute to the antimicrobial activity of compounds 3a, 3b, 3d, 3e, 3f, 3g, 3h, 3i, 3j, 3k, 3l, 3m, 5a, 5b, and 5f. As amides often possess certain bioactivity, the amide unit in these compounds (3d, 3e, 3f, 3g, 3h, 3i) may also help to improve the antimicrobial activity.29–32 The cyclic ketone fragments in compounds 3j and 3l also enhance the antimicrobial activity in some cases. These results demonstrated that 2-methyl-3-furyl sulfide flavor derivatives have potential application prospects in the field of food preservation, which is of great significance for the prevention and control of foodborne microorganisms in the food industry or other industries.
Conclusions
These synthesized 2-methyl-3-furyl sulfide derivatives with amide, ketone, cyclic ether, or cyclic alcohol motif have special aroma characteristics and low aroma thresholds. The majority of these compounds (3a, 3b, 3d, 3e, 3f, 3g, 3h, 3i, 3j, 3k, 3l, 3m, 5a, 5b, 5f) showed excellent antimicrobial activities against a variety of foodborne bacterial or fungal strains (E. coli, B. subtilis, S. aureus, S. paratyphi, L. monocytogenes, V. parahemolyticus, P. italicum, A. niger, M. racemosus, R. oryzae). Importantly, compounds 3b, 3d, 3e, 3i, 3j, 3l, and 3m showed better antimicrobial activity than the control group (penicillin, amphotericin B and thiram). Predictably, these flavor compounds with antimicrobial activity are promising in food industry as well as in other industries that need to guarantee safety criteria and to preserve freshness by slowing down microbial growth.
Experimental section
General
All reagents and solvents were purchased from commercial suppliers (Energy Chemical, Shanghai, China) and were used without further purification. Column chromatography or thin-layer chromatography was used for product separation, which was visualized by UV light. High-resolution mass spectrometer (MS) was carried out with a Thermo MAT95XP (Thermo Fisher Scientific, Bremen, Germany) apparatus. Infrared absorption spectrum (IR) were measured on a Nicolet IS10 (Thermo Fisher Scientific, Bremen, Germany). Nuclear magnetic resonance (NMR) spectra (δ, J in hertz) were recorded on a Bruker Avance-500 (Bruker, Fällanden, Switzerland) NMR spectrometer. Tetramethylsilane (TMS) was used as the internal reference (δ 0.00) for 1H NMR spectra measured in CDCl3. This solvent was also used for 13C NMR spectra.
Synthesizes
Synthesis of derivatives 3a–i (Scheme 1 (1)). A mixture of bis(2-methyl-3-furyl)disulfide (0.2 mmol), di-tert-butyl peroxide (DTBP, 0.8 mmol) and compounds 2a–i (1 mL, including tetrahydrofuran, 1,4-dioxane, N,N-dimethylacetamide, N,N-dimethylformamide, N-methylacetamide, N-formylmorpholine or N-acetylmorpholine) were added to a pressure vessel tube and sealed.18,19 The reaction mixture was stirred at 120 °C for 24 h. After the reaction completion, the mixture was poured into ethyl acetate and washed with saturated NaCl. The aqueous layer was extracted with ethyl acetate and the combined organic layers were dried over anhydrous Na2SO4, filtered through a celite pad, and evaporated under vacuum. The residue was purified by flash column chromatography (petroleum ether/ethyl acetate) to afford the desired products (Note: 3d and 3e, 3g and 3h were isolated in a single reaction).
Synthesis of derivatives 3j–m (Scheme 1 (2)). A mixture of bis(2-methyl-3-furyl)disulfide (0.2 mmol), K3PO4 (0.3 mmol) and compounds 2j–m (0.4 mmol, including cyclohexanone, 1,3-cyclohexanedione, 5,5-dimethyl-1,3-cyclohexanedione or acetylacetone) in dimethyl sulfoxide (DMSO, 1 mL) were added to a pressure vessel tube and sealed.20 The reaction mixture was stirred at 40 °C for 24 h. After the reaction completion, the mixture was poured into ethyl acetate and washed with saturated NaCl. The aqueous layer was extracted with ethyl acetate and the combined organic layers were dried over anhydrous Na2SO4, filtered through a celite pad, and evaporated under vacuum. The residue was purified by flash column chromatography (petroleum ether/ethyl acetate) to afford the desired products.
Synthesis of derivatives 5a–f (Scheme 1 (3)). A mixture of bis(2-methyl-3-furyl)disulfide (0.2 mmol), NaBH4 (0.3 mmol), compounds 4a–f (0.4 mmol, including cyclopentene oxide, cyclohexene oxide, isobutylene oxide, butadiene monoxide or styrene oxide), and ethanol (EtOH, 1 mL) were added to a pressure vessel tube and sealed.21 The reaction mixture was stirred at 80 °C for 4 h. After the reaction completion, the mixture was poured into ethyl acetate and washed with saturated NaCl. The aqueous layer was extracted with ethyl acetate and the combined organic layers were dried over anhydrous Na2SO4, filtered through a celite pad, and evaporated under vacuum. The residue was purified by flash column chromatography (petroleum ether/ethyl acetate) to afford the desired products (Note: 5e and 5f were isolate in a single reaction mixture).
2-Methyl-3-((tetrahydrofuran-2-yl)thio)furan (3a). Yield (16 mg, 43%); yellow oil; 1H NMR (500 MHz, CDCl3, Me4Si) δ: 7.27 (d, J = 1.9 Hz, 1H, ArH), 6.40 (d, J = 1.9 Hz, 1H, ArH), 5.31 (dd, J = 7.1, 3.7 Hz, 1H, CH), 4.01–3.87 (m, 2H, CH2), 2.34 (s, 3H, Me), 2.29–1.97 (m, 2H, CH2), 1.95–1.83 (m, 2H, CH2); 13C NMR (126 MHz, CDCl3, Me4Si) δ: 155.4, 140.4, 115.6, 109.4, 87.8, 67.3, 32.4, 24.9, 11.9.
2-((2-Methylfuran-3-yl)thio)-1,4-dioxane (3b). Yield (30 mg, 74%); yellow oil; 1H NMR (500 MHz, CDCl3, Me4Si) δ: 7.27 (d, J = 1.8 Hz, 1H, ArH), 6.37 (d, J = 1.8 Hz, 1H, ArH), 4.75 (dd, J = 6.4, 2.9 Hz, 1H, CH), 4.16–4.11 (m, 1H, CH2), 3.89 (dd, J = 11.7, 2.9 Hz, 1H, CH2), 3.66–3.65 (m, 2H, CH2), 3.64–3.58 (m, 2H, CH2), 2.34 (s, 3H, Me); 13C NMR (126 MHz, CDCl3, Me4Si) δ: 155.8, 140.6, 115.7, 107.5, 82.9, 69.9, 66.4, 64.5, 12.0.
N-Methyl-N-(((2-methylfuran-3-yl)thio)methyl)acetamide (3c). Yield (29 mg, 74%); yellow oil; rotation hindrance of the amide bond leads to paired signal peaks in 1H NMR and 13C NMR; 1H NMR (500 MHz, CDCl3, Me4Si) δ: 7.18/7.13 (d, J = 1.7 Hz, 1H, ArH), 6.21/6.20 (d, J = 1.7 Hz, 1H, ArH), 4.44/4.31 (s, 2H, CH2), 2.87/2.82 (s, 3H, Me), 2.19/2.15 (s, 3H, Me), 1.88/1.62 (s, 3H, Me); 13C NMR (126 MHz, CDCl3, Me4Si) δ: 170.3/170.0, 156.7/155.1, 140.9/140.4, 115.3/115.0, 108.4/107.4, 56.6/52.3, 35.1/32.4, 21.5/20.3, 11.5/11.4.
N-Methyl-N-(((2-methylfuran-3-yl)thio)methyl)formamide (3d). Yield (15 mg, 40%); yellow oil; rotation hindrance of the amide bond leads to paired signal peaks in 1H NMR and 13C NMR; 1H NMR (500 MHz, CDCl3, Me4Si) δ: 7.95/7.53 (s, 1H, CHO), 7.30/7.25 (d, J = 1.8 Hz, 1H, ArH), 6.34/6.27 (d, J = 1.7 Hz, 1H, ArH), 4.51/4.30 (s, 2H, CH2), 2.98/2.93 (s, 3H, Me), 2.32/2.25 (s, 3H, Me); 13C NMR (126 MHz, CDCl3, Me4Si) δ: 162.4/161.6, 157.3/155.5, 141.5/140.9, 115.0/115.0, 108.2/107.2, 56.4/49.2, 33.5/28.9, 11.8/11.7; IR (KBr, cm−1): 2922, 2854, 1673 (C
O), 1387, 1253, 1224, 1088, 1060, 736; HRMS (ESI) m/z calcd for C8H12NO2S+ (M + H)+ 186.0583, found 186.0578; C8H11NaNO2S+ (M + Na)+ 208.0403, found 208.0397.
S-(2-Methylfuran-3-yl)dimethylcarbamothioate (3e). Yield (17 mg, 45%); yellow oil; 1H NMR (500 MHz, CDCl3, Me4Si) δ: 7.31 (d, J = 1.8 Hz, 1H, ArH), 6.33 (d, J = 1.8 Hz, 1H, ArH), 3.06 (s, 3H, Me), 2.98 (s, 3H, Me), 2.28 (s, 3H, Me); 13C NMR (126 MHz, CDCl3, Me4Si) δ: 166.1, 156.9, 140.6, 115.7, 104.8, 36.8 (2C), 11.9; IR (KBr, cm−1): 3120, 2921, 1673 (C
O), 1514, 1363, 1227, 1127, 1101, 1086, 691; HRMS (ESI) m/z calcd for C8H12NO2S+ (M + H)+ 186.0583, found 186.0579; C8H11NaNO2S+ (M + Na)+ 208.0403, found 208.0397.
N-(((2-Methylfuran-3-yl)thio)methyl)acetamide (3f). Yield (26 mg, 70%); yellow oil; 1H NMR (500 MHz, CDCl3, Me4Si) δ: 7.27 (d, J = 1.8 Hz, 1H, ArH), 6.34 (d, J = 1.8 Hz, 1H, ArH), 6.20 (s, 1H, NH), 4.34 (d, J = 6.4 Hz, 2H, CH2), 2.32 (s, 3H, Me), 1.93 (s, 3H, Me); 13C NMR (126 MHz, CDCl3, Me4Si) δ: 169.8, 155.8, 140.9, 115.1, 108.7, 44.8, 23.2, 11.8; IR (KBr, cm−1): 3280 (NH), 3065, 2922, 1659 (C
O), 1538, 1514, 1371, 1262, 1088, 733; HRMS (ESI) m/z calcd for C8H12NO2S+ (M + H)+ 186.0583, found 186.0579; C8H11NaNO2S+ (M + Na)+ 208.0403, found 208.0398.
2-((2-Methylfuran-3-yl)thio)morpholine-4-carbaldehyde (3g). Yield (17 mg, 37%); yellow oil; rotation hindrance of the amide bond leads to paired signal peaks in 1H NMR and 13C NMR; 1H NMR (500 MHz, CDCl3, Me4Si) δ: 7.90/7.43 (s, 1H, CHO), 7.29/7.23 (d, J = 1.6 Hz, 1H, ArH), 6.35/6.27 (d, J = 1.4 Hz, 1H, ArH), 5.56/4.59 (d, J = 2.2 Hz, 1H, CH), 4.11–4.08 (m, 1H, CH2), 4.02–3.92 (m, 2H, CH2), 3.83–3.62 (m, 1H, CH2), 3.50–3.35 (m, 2H, CH2), 2.33/2.24 (s, 3H, Me); 13C NMR (126 MHz, CDCl3, Me4Si) δ: 160.7/160.2, 157.5/156.5, 141.7/140.8, 115.6/115.3, 107.7/107.4, 69.8/69.2, 67.3/66.7, 64.2/57.0, 41.4/35.9, 11.9/11.8; IR (KBr, cm−1): 2969, 2920, 2857, 1681 (C
O), 1415, 1275, 1118, 1101, 1012, 739; HRMS (ESI) m/z calcd for C10H14NO3S+ (M + H)+ 228.0689, found 228.0681; C10H13NaNO3S+ (M + Na)+ 250.0508, found 250.0501.
S-(2-Methylfuran-3-yl)morpholine-4-carbothioate (3h). Yield (20 mg, 44%); yellow oil; 1H NMR (500 MHz, CDCl3, Me4Si) δ: 7.33 (d, J = 1.8 Hz, 1H, ArH), 6.34 (d, J = 1.7 Hz, 1H, ArH), 3.71–3.68 (m, 4H, CH2), 3.59–3.56 (m, 4H, CH2), 2.29 (s, 3H, Me); 13C NMR (126 MHz, CDCl3, Me4Si) δ: 165.6, 157.3, 140.9, 115.7, 104.1, 66.5 (2C), 45.2 (2C), 12.0; IR (KBr, cm−1): 2966, 2919, 2855, 1667 (C
O), 1401, 1270, 1213, 1114, 1017, 836; HRMS (ESI) m/z calcd for C10H14NO3S+ (M + H)+ 228.0689, found 228.0682; C10H13NaNO3S+ (M + Na)+ 250.0508, found 250.0501.
1-(2-((2-Methylfuran-3-yl)thio)morpholino)ethan-1-one (3i). Yield (22 mg, 46%); yellow oil; rotation hindrance of the amide bond leads to paired signal peaks in 1H NMR and 13C NMR; 1H NMR (500 MHz, CDCl3, Me4Si) δ: 7.30/7.24 (d, J = 1.5 Hz, 1H, ArH), 6.36/6.33 (d, J = 1.4 Hz, 1H, ArH), 5.80/4.93 (s, 1H, CH), 4.25–4.23 (m, 1H, CH2), 4.05–3.92 (m, 2H, CH2), 3.78–3.63 (m, 1H, CH2), 3.51–3.38 (m, 2H, CH2), 2.35/2.29 (s, 3H, Me), 1.98/1.70 (s, 3H, Me); 13C NMR (126 MHz, CDCl3, Me4Si) δ: 169.1/168.9, 157.5/156.7, 141.4/140.7, 116.0/115.9, 107.9/107.8, 69.6/69.5, 67.3/66.7, 64.5/57.8, 42.0/36.7, 21.3/20.0, 11.9/11.8; IR (KBr, cm−1): 2966, 2919, 2850, 1650 (C
O), 1409, 1291, 1226, 1120, 998; HRMS (ESI) m/z calcd for C11H16NO3S+ (M + H)+ 242.0845, found 242.0837; C11H15NaNO3S+ (M + Na)+ 264.0665, found 264.0656.
2-((2-Methylfuran-3-yl)thio)cyclohexan-1-one (3j). Yield (32 mg, 75%); yellow oil; 1H NMR (500 MHz, CDCl3, Me4Si) δ: 7.24 (d, J = 1.8 Hz, 1H, ArH), 6.28 (d, J = 1.8 Hz, 1H, ArH), 3.48 (t, J = 5.6 Hz, 1H, CH), 2.30 (s, 3H, Me), 2.29–2.22 (m, 2H, CH2), 2.20–2.08 (m, 2H, CH2), 1.84–1.76 (m, 2H, CH2), 1.69–1.60 (m, 2H, CH2); 13C NMR (126 MHz, CDCl3, Me4Si) δ: 207.5, 156.2, 140.8, 115.3, 108.2, 39.1, 33.6, 27.2, 22.6 (2C), 11.9.
2-((2-Methylfuran-3-yl)thio)cyclohexane-1,3-dione (3k). Yield (26 mg, 58%); brown oil; 1H NMR (500 MHz, CDCl3, Me4Si) δ: 8.15 (s, 1H, CH), 7.18 (d, J = 1.8 Hz, 1H, ArH), 6.27 (d, J = 1.7 Hz, 1H, ArH), 2.65–2.60 (m, 2H, CH2), 2.46–2.43 (m, 2H, CH2), 2.41 (s, 3H, Me), 1.99–1.95 (m, 2H, CH2); 13C NMR (126 MHz, CDCl3, Me4Si) δ: 194.2, 178.1, 154.6, 140.6, 114.5, 109.9, 109.6, 37.3, 28.4, 20.0, 12.1; IR (KBr, cm−1): 2923, 1650 (C
O), 1573 (Ar), 1514 (Ar), 1376, 1325, 1221, 1133, 1087; HRMS (ESI) m/z calcd for C11H13O3S+ (M + H)+ 225.0580, found 225.0572; C11H12NaO3S+ (M + Na)+ 247.0399, found 247.0391.
5,5-Dimethyl-2-((2-methylfuran-3-yl)thio)cyclohexane-1,3-dione (3l). Yield (33 mg, 66%); brown oil; 1H NMR (500 MHz, CDCl3, Me4Si) δ: 8.14 (s, 1H, CH), 7.16 (d, J = 1.6 Hz, 1H, ArH), 6.23 (d, J = 1.5 Hz, 1H, ArH), 2.48 (s, 2H, CH2), 2.40 (s, 3H, Me), 2.30 (s, 2H, CH2), 1.03 (s, 6H, Me); 13C NMR (126 MHz, CDCl3, Me4Si) δ: 194.0, 176.5, 154.4, 140.6, 114.3, 109.6, 108.6, 51.1, 42.0, 31.7, 28.2 (2C), 12.1; IR (KBr, cm−1): 2958, 2926, 1651 (C
O), 1573 (C
O), 1369, 1221, 1160, 1142, 1087; HRMS (ESI) m/z calcd for C13H17O3S+ (M + H)+ 253.0893, found 253.0885; C13H16NaO3S+ (M + Na)+ 275.0712, found 275.0703.
3-((2-Methylfuran-3-yl)thio)pentane-2,4-dione (3m). Yield (20 mg, 47%); brown oil; 1H NMR (500 MHz, CDCl3, Me4Si) δ: 7.24 (d, J = 1.6 Hz, 1H, ArH), 6.13 (d, J = 1.5 Hz, 1H, ArH), 2.42 (s, 6H, Me), 2.33 (s, 3H, Me); 13C NMR (126 MHz, CDCl3, Me4Si) δ: 197.1 (2C), 150.6, 141.2, 112.8, 112.3, 104.7, 24.7 (2C), 12.0; IR (KBr, cm−1): 2921, 1584 (C
O), 1513, 1411, 1222, 1088, 1019, 935, 732; HRMS (ESI) m/z calcd for C10H13O3S+ (M + H)+ 213.0580, found 212.0574; C10H12NaO3S+ (M + Na)+ 235.0399, found 235.0391.
2-((2-Methylfuran-3-yl)thio)cyclopentan-1-ol (5a). Yield (35 mg, 88%); yellow oil; 1H NMR (500 MHz, CDCl3, Me4Si) δ: 7.25 (d, J = 1.9 Hz, 1H, ArH), 6.33 (d, J = 1.9 Hz, 1H, ArH), 3.96 (dd, J = 11.2, 5.1 Hz, 1H, CH), 3.04–2.90 (m, 1H, CH), 2.46 (s, 1H, OH), 2.32 (s, 3H, Me), 2.11–1.96 (m, 2H, CH2), 1.76–1.60 (m, 2H, CH2), 1.58–1.45 (m, 2H, CH2); 13C NMR (126 MHz, CDCl3, Me4Si) δ: 155.8, 140.6, 115.7, 108.9, 78.0, 55.3, 32.8, 30.3, 21.5, 11.9.
2-((2-Methylfuran-3-yl)thio)cyclohexan-1-ol (5b). Yield (36 mg, 86%); yellow oil; 1H NMR (500 MHz, CDCl3, Me4Si) δ: 7.24 (d, J = 1.9 Hz, 1H, ArH), 6.28 (d, J = 1.9 Hz, 1H, ArH), 3.22–3.17 (m, 1H, CH), 3.09 (s, 1H, OH), 2.44–2.38 (m, 1H, CH), 2.31 (s, 3H, Me), 2.07–1.59 (m, 2H, CH2), 1.66–1.59 (m, 2H, CH2), 1.28–1.17 (m, 4H, CH2); 13C NMR (126 MHz, CDCl3, Me4Si) δ: 156.8, 140.4, 116.6, 106.1, 71.4, 55.9, 33.7, 32.0, 26.1, 24.3, 11.9.
2-Methyl-2-((2-methylfuran-3-yl)thio)propan-1-ol (5c). Yield (33 mg, 90%); yellow oil; 1H NMR (500 MHz, CDCl3, Me4Si) δ: 7.23 (d, J = 1.8 Hz, 1H, ArH), 6.32 (d, J = 1.7 Hz, 1H, ArH), 2.80 (s, 2H, CH2), 2.43 (s, 1H, OH), 2.31 (s, 3H, Me), 1.23 (s, 6H, Me); 13C NMR (126 MHz, CDCl3, Me4Si) δ: 153.7, 140.7, 114.4, 111.5, 70.7, 50.3, 28.5 (2C), 11.9; IR (KBr, cm−1): 3417 (OH), 2972, 2920, 1514, 1373, 1222, 1129, 1088, 889, 731; HRMS (ESI) m/z calcd for C9H15O2S+ (M + H)+ 187.0787, found 187.0782; C9H14NaO2S+ (M + Na)+ 209.0607, found 186.0601.
2-((2-Methylfuran-3-yl)thio)but-3-en-1-ol (5d). Yield (25 mg, 67%); yellow oil; 1H NMR (500 MHz, CDCl3, Me4Si) δ: 7.28 (d, J = 1.7 Hz, 1H, ArH), 6.35 (d, J = 1.6 Hz, 1H, ArH), 5.87–5.79 (m, 1H, CH), 5.29 (d, J = 17.2 Hz, 1H,
CH), 5.15 (d, J = 10.5 Hz, 1H,
CH), 4.10–4.05 (m, 1H, OH), 2.83–2.80 (m, 2H, CH2), 2.68–2.64 (m, 1H, CH), 2.34 (s, 3H, Me); 13C NMR (126 MHz, CDCl3, Me4Si) δ: 155.1, 140.9, 138.6, 116.1, 114.9, 109.4, 70.5, 43.5, 11.9; IR (KBr, cm−1): 3416 (OH), 2954, 1643 (C
C), 1513, 1223, 1127, 1088, 990, 933, 888, 733; HRMS (ESI) m/z calcd for C9H13O2S+ (M + H)+ 185.0631, found 185.0626; C9H12NaO2S+ (M + Na)+ 207.0450, found 207.0444.
2-((2-Methylfuran-3-yl)thio)-1-phenylethan-1-ol (5e). Yield (23 mg, 49%); yellow oil; 1H NMR (500 MHz, CDCl3, Me4Si) δ: 7.33–7.25 (m, 6H, Ph and ArH), 6.34 (d, J = 1.9 Hz, 1H, ArH), 4.58 (dd, J = 9.5, 3.5 Hz, 1H, CH), 3.02 (s, 1H, OH), 2.99–2.95 (m, 1H, CH2), 2.80–2.76 (m, 1H, CH2), 2.34 (s, 3H, Me); 13C NMR (126 MHz, CDCl3, Me4Si) δ: 155.1, 142.2, 141.0, 128.5 (2C), 127.9, 126.0 (2C), 114.8, 109.3, 71.8, 45.5, 11.9.
2-((2-Methylfuran-3-yl)thio)-2-phenylethan-1-ol (5f). Yield (18 mg, 39%); yellow oil; 1H NMR (500 MHz, CDCl3, Me4Si) δ: 7.29–7.23 (m, 3H, Ph), 7.22 (d, J = 1.6 Hz, 1H, ArH), 7.15–7.11 (m, 2H, Ph), 6.17 (d, J = 1.8 Hz, 1H, ArH), 3.98–3.95 (m, 1H, CH), 3.89–3.86 (m, 2H, CH2), 2.16 (s, 1H, OH), 1.98 (s, 3H, Me); 13C NMR (126 MHz, CDCl3, Me4Si) δ: 157.3, 140.6, 139.0, 128.6 (2C), 128.1 (2C), 127.7, 116.0, 107.3, 64.3, 55.8, 11.4; IR (KBr, cm−1): 3417 (OH), 2920, 1514 (Ph), 1452, 1223, 1087, 1056, 1019, 733, 697; HRMS (ESI) m/z calcd for C13H15O2S+ (M + H)+ 235.0787, found 235.0779; C13H14NaO2S+ (M + Na)+ 257.0607, found 257.0598.
Bacterial and fungal cultures
Bacterial strains. E. coli CMCC 44102, B. subtilis GDMCC 1.372, S. aureus JCSC 2172, S. paratyphi CMCC 50094, L. monocytogenes NCTC 7974, V. parahemolyticus GDMCC 1.306.
Fungal strains. P. italicum BNCC 336886, A. niger GDMCC 3.237, M. racemosus GDMCC 3.86, R. oryzae GDMCC 3.131.
These bacterial and fungal strains were generously supplied by the School of Oceanography, South China Agricultural University (Guangzhou, China). All bacterial strains were routinely grown in tryptic soy broth (HuanKai Microbial, Guangzhou, China) at 37 °C for 24 h. All fungal strains were routinely grown in potato dextrose broth (HuanKai Microbial, Guangzhou, China) at 28 °C for 7 d. The bacterial and fungal cultures were stored in glycerol (70
:
30, v/v, culture
:
glycerol) at −80 °C prior to usage.
Evaluation of in vitro antimicrobial activity
A disk diffusion test was carried out in order to evaluate the antimicrobial activity of the 2-methyl-3-furyl sulfide flavor derivatives.33 The test was performed by applying a 100 μL bacterial inoculum of approximately 1–2 × 108 colony forming units (CFU) mL−1 to the surface of a tryptose soya agar plate (fungus, potato dextrose agar) (HuanKai Microbial, Guangzhou, China). The inoculum was allowed to dry for 15 min, then two sterile disks of 6 mm were placed on the inoculated agar surface. One was impregnated with 2.5 μL of one of the 2-methyl-3-furyl sulfide flavor derivatives, which represents a concentration of 10 mg mL−1. Another with 2.5 μL of 2 mg mL−1 penicillin (fungi, amphotericin B and thiram), which represented the positive control. All the tested compounds were dissolved in methanol. The plates were left 15 min at room temperature to allow the diffusion of the compounds, and then they were incubated for 24 h at 37 °C (fungi was incubated for 5–7 d at 28 °C). After incubation, the zones of growth inhibition around each of the disks were measured in millimeters. The diameter of the zone was related to the susceptibility of the strains to the 2-methyl-3-furyl sulfide flavor derivatives. The experiments were carried out in triplicate.
Determination of MIC
The dilutions of the 2-methyl-3-furyl sulfide flavor derivatives were established based on the inhibitory profile with the disk diffusion test. The assay was based on the procedure of the Clinical and Laboratory Standards Institute with 96-well microtiter plates.34 The MIC was considered the lowest concentration of 2-methyl-3-furyl sulfide flavor derivatives at which bacteria failed to grow, as detected by the unaided eye, matching with the positive control penicillin (fungi, amphotericin B and thiram) included in the test. An aliquot of 200 μL of the 1–2 × 108 CFU mL−1 microbial suspension (bacteria or fungi) was distributed in each well containing two-fold serial dilution of the positive controls and tested derivatives. The final concentrations of positive controls and tested compounds were 25, 12.5, 6.25, 3.125, 1.56 and 0.78 μg mL−1. Initially all the tested compounds were dissolved in DMSO. The microplates were incubated for 16 h at 37 °C (fungi was incubated for 5–7 d at 28 °C), which were examined for visible microbial growth, as evidenced by turbidity. The experiments were carried out in triplicate.
Odor evaluation
The odor evaluation of 2-methyl-3-furyl sulfide derivatives was carried out by a group of trained personnel. Dilute the synthesized derivatives with deionized water in different concentration gradients, allowing the team members to smell them from low concentration to high concentration. If more than half of the people smell a certain concentration, the concentration is deemed to be the threshold value of the derivatives in the water.35
Statistical analysis
The antimicrobial activity of different 2-methyl-3-furyl sulfide flavor derivatives against the same strain was compared and evaluated. Statistical elaborations of the results obtained from antimicrobial experiments were performed with IBM SPSS Statistics 26 (IBM, New York, USA), analysis of variance (ANOVA test). Differences were considered to be significant if the value of p < 0.05.
Conflicts of interest
The authors declare no competing financial interest.
Acknowledgements
We thank the Guangdong Basic and Applied Basic Research Foundation (No. 2019B151502052) and Guangzhou Scientific and Technological Project (No. 202002030295) for its financial support.
References
- H. Cui, C. Zhang, C. Li and L. Lin, Food Control, 2018, 94, 140–146 CrossRef CAS.
- K. H. Baek, H. I. Yong, J. H. Yoo, J. W. Kim, Y. S. Byeon, J. Lim, S. Y. Yoon, S. Ryu and C. Jo, J. Phys. D: Appl. Phys., 2020, 53, 124002 CrossRef CAS.
- J. H. Yoo, K. H. Baek, Y. S. Heo, H. I. Yong and C. Jo, Food Microbiol., 2021, 93, 103611 CrossRef CAS PubMed.
- C. Nerín, M. Aznar and D. Carrizo, Trends Food Sci. Technol., 2016, 48, 63–68 CrossRef.
- W. Kewcharoen and P. Srisapoome, Fish Shellfish Immunol., 2019, 94, 175–189 CrossRef CAS PubMed.
- J. Ju, Y. Xie, H. Yu, Y. Guo, Y. Cheng, R. Zhang and W. Yao, Food Chem., 2020, 310, 125974 CrossRef CAS PubMed.
- N. S. Higazy, A. E. Saleh, Z. U. Hassan, R. Al Thani, Q. Migheli and S. Jaoua, Food Control, 2021, 119, 107464 CrossRef CAS.
- P. Luu, V. S. Chhetri, M. E. Janes, J. M. King and A. Adhikari, Foods, 2020, 9, 1259 CrossRef CAS PubMed.
- P. Y. Chung, Phytomedicine, 2020, 73, 152933 CrossRef CAS PubMed.
- N. Khorshidian, M. Yousefi, E. Khanniri and A. M. Mortazavian, Innovative Food Sci. Emerging Technol., 2018, 45, 62–72 CrossRef CAS.
- C. L. Bouarab, P. Degraeve, H. Ferhout, J. Bouajila and N. Oulahal, J. Sci. Food Agric., 2019, 99, 1457–1474 CrossRef PubMed.
- B. Sun, Sulfur-Containing Fragrance Chemistry, Science Press, Beijing, 2007, pp. 72–124 Search PubMed.
- W. Tang, D. Jiang, P. Yuan and C. Ho, J. Sulfur Chem., 2012, 34, 38–47 CrossRef.
- M. Wang, C. Wang and X. Jiang, Chin. J. Org. Chem., 2019, 39, 2139–2147 CrossRef CAS.
- G. Zhang, Y. Liang, J. Zhu, Q. Jia, W. Gan, L. Sun and H. Hou, Food Chem., 2015, 180, 1–8 CrossRef CAS PubMed.
- H. Hou, W. Yifang, Z. Gongliang, Z. Yaolei, X. Longquan, H. Hongshun, W. Yue and L. Meishan, J. Food Sci., 2018, 83, 2550–2559 CrossRef CAS PubMed.
- K. C. Nicolaou, R. Hughes, J. A. Pfefferkorn, S. Barluenga and A. J. Roecker, Chem.–Eur. J., 2013, 15, 4654–4657 Search PubMed.
- S. Guo, Y. Yuan and J. Xiang, Org. Lett., 2001, 7, 4280–4295 Search PubMed.
- R. Tang, Y. Xie, Y. Xie, J. Xiang and J. Li, Chem. Commun., 2011, 47, 12867 RSC.
- B. Movassagh and A. Yousefi, Monatsh. Chem., 2014, 145, 1173–1177 CrossRef CAS.
- P. K. Patra, K. Shanmugasundaram, M. Matoba, K. Nishide, T. Kajimoto and M. Node, Synthesis, 2005, 2005, 447–457 CrossRef.
- W. Sukbangnop, A. Hosen, S. Hongthong, C. Kuhakarn, P. Tuchinda, S. Chaturonrutsamee, S. Thanasansurapong, R. Akkarawongsapat, J. Limthongkul, C. Napaswad, A. Chairoungdua, K. Suksen, N. Nuntasaen and V. Reutrakul, Fitoterapia, 2021, 151, 104885 CrossRef CAS PubMed.
- A. Chilin, M. T. Conconi, G. Marzaro, A. Guiotto, L. Urbani, F. Tonus and P. Parnigotto, J. Med. Chem., 2010, 53, 1862–1866 CrossRef CAS PubMed.
- Z. Huang, X. Xia, Z. Huang, L. Xu, X. Zhang and R. Tang, Org. Biomol. Chem., 2020, 18, 1176–1369 Search PubMed.
- N. S. Korde, S. T. Gaikwad, B. C. Khade and A. S. Rajbhoj, Chem. Sci. Trans., 2013, 2, 407–412 CrossRef.
- Y. Liu, H. Chen, D. Yin and B. Sun, Molecules, 2010, 15, 5104–5111 CrossRef CAS PubMed.
- A. Shang, S. Cao, X. Xu, R. Gan, G. Tang, H. Corke, V. Mavumengwana and H. Li, Foods, 2019, 8, 246 CrossRef CAS PubMed.
- J. Xie, B. Liao and R. Tang, J. Agric. Food Chem., 2020, 68, 12505–12526 CrossRef CAS PubMed.
- J. Chen, C. Yi, S. Wang, S. Wu, S. Li, D. Hu and B. Song, Bioorg. Med. Chem. Lett., 2019, 29, 1203–1210 CrossRef CAS PubMed.
- Y. Lu, J. L. Papa, S. Nolan, A. English, J. T. Seffernick, N. Shkolnikov, J. Powell, S. Lindert, D. J. Wozniak, J. Yalowich and M. J. Mitton-Fry, ACS Med. Chem. Lett., 2020, 11, 2446–2454 CrossRef CAS PubMed.
- S. X. Guo, F. He, A. L. Dai, R. F. Zhang, S. H. Chen and J. Wu, RSC Adv., 2020, 10, 35658–35670 RSC.
- K. Lal, N. Poonia, P. Rani, A. Kumar and A. Kumar, J. Mol. Struct., 2020, 1215, 128234 CrossRef CAS.
- X. Liang, X. Nong, Z. Huang and S. Qi, J. Agric. Food Chem., 2017, 65, 5114–5121 CrossRef CAS PubMed.
- J. García-Díez, J. Alheiro, A. L. Pinto, L. Soares, V. Falco, M. J. Fraqueza and L. Patarata, Foods, 2017, 6, 44 CrossRef PubMed.
- Y. Miyazawa, Y. Masuda, Y. Ohmori, R. Katsuta, T. Nukada and K. Ishigami, Flavour Fragrance J., 2019, 34, 43–51 CrossRef CAS.
Footnote |
† Electronic supplementary information (ESI) available. See DOI: 10.1039/d1ra04207f |
|
This journal is © The Royal Society of Chemistry 2021 |
Click here to see how this site uses Cookies. View our privacy policy here.