DOI:
10.1039/D1RA04450H
(Paper)
RSC Adv., 2021,
11, 22000-22004
Iridium-catalyzed regioselective C–H sulfonamidation of 1,2,4-thiadiazoles with sulfonyl azides in water†
Received
8th June 2021
, Accepted 14th June 2021
First published on 22nd June 2021
Abstract
We have developed a regioselective C–N cross-coupling of 1,2,4-thiadiazoles with sulfonyl azides through iridium catalysis in water. This method tactically linked the 1,2,4-thiadiazoles and sulfonamides together, and the novel molecules increased the diversity of 1,2,4-thiadiazoles which may have potential applications.
Introduction
1,2,4-Thiadiazole scaffolds exist as significant structural motifs in a myriad of biologically active compounds,1 natural products,2 and materials3 (Fig. 1). In particular, the 1,2,4-thiadiazole derivatives have been found to have a broad range of biological activities. However, due to the limitations of synthetic methods, these 1,2,4-thiadiazole derivatives are relatively simple, which limit the further optimization and screening of their activities. For the purpose of building drug like libraries, the development of efficient and practical approaches to construct diverse 1,2,4-thiadiazoles is of great significance.
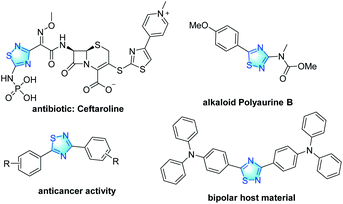 |
| Fig. 1 The application 1,2,4-thiadiazoles derivatives. | |
Carbon–hydrogen (C–H) bond functionalizations are attractive tools for the construction of valuable molecules.4–6 The heterocyclic core of 1,2,4-thiadiazole contains nitrogen-atom, which is generally used as directing group in C–H activation reactions.7 These considerations made us develop a straightforward C–H functionalization route for generating diverse 1,2,4-thiadiazole derivatives. Under the guidance of this strategy, our laboratory had successfully synthesized 1,2,4-thiadiazole compounds containing succinimide,8 and those compounds were found to have antitumor activity. Inspired by the fruitful previous work, in this context, we were interested in investigating the amidation of 1,2,4-thiadiazole substrates.
On the other hand, aryl amines are key components in a range of organic molecules as well.9 As a result, extensive studies have been devoted to transition-metal-catalyzed (such as Ru,10 Rh,11 Ir,12 Co,13 Mn14 and Cu15) direct C(sp2)–H amidation reactions (Scheme 1a). Despite the utilities represent, we want to get those valuable compounds in a relatively green way. Traditional chemical process rely heavily on organic solvents for a multitude of tasks, water, termed as a “green” solvent used as a substitute for organic solvents in organic synthesis because of its economical and safe, the development of reactions using water as a reaction medium has flourished recently.16 Fortunately, our group developed a regioselective C–N cross-coupling of 1,2,4-thiadiazoles with sulfonyl azides using water as the only solvent (Scheme 1b).
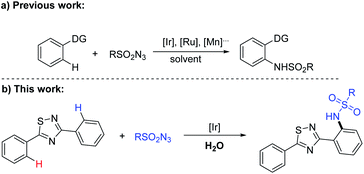 |
| Scheme 1 Transition-metal-catalyzed C–H sulfonamidation. | |
Results and discussion
Model investigations focused on the amidation of 1a (Table 1). Firstly, the catalyst and solvent were screened (Table 1, entries 1–13), the result showed that [Cp*IrCl2]2 was essential for the reaction and the solvent had an important effect on the reaction as well, no product 2aa were formed in most solvents, only 1,2-DCE and H2O offered moderate conversion. Although the conversion efficiency of 1a was very low in water, this positive result greatly encouraged us to continue to evaluate various additives for improving the C–H bond amidation yield (Table 1, entries 14–31). To our delight, a further significant improvement of the reaction was achieved by adding C6F5COOH (86%, Table 1, entry 28). Then, a number solvents were screened in the presence of [Cp*IrCl2]2 and C6F5COOH, and no better results were obtained than water (Table 1, entries 32–37). Similarly, AgSbF6 has an important effect on promoting the yield of 2aa. After an extensive survey of reaction parameters, we arrived at the optimized conditions in H2O at 90 °C, affording the desired product 2aa in 86% isolated yield.
Table 1 Optimization of the reaction conditionsa,b

|
Entry |
Catalyst |
Solvent |
Additive |
Yieldc (%) |
Reaction conditions: 2a 0.2 mmol, TsN3 0.3 mmol, catalyst 2.0 mol%, AgSbF6 8 mol%, additive 40 mol%, solvent 1.0 mL, 90 °C, 24 h. No AgSbF6. Isolated yield. |
1 |
[Cp*RhCl2]2 |
1,2-DCE |
None |
15 |
2 |
[Cp*IrCl2]2 |
1,2-DCE |
None |
76 |
3 |
[CodIrCl]2 |
1,2-DCE |
None |
0 |
4 |
[CodIrOMe]2 |
1,2-DCE |
None |
0 |
5 |
Cp*Co(CO)I2 |
1,2-DCE |
None |
0 |
6 |
(Cp*)2CoPF6 |
1,2-DCE |
None |
0 |
7b |
[Cp*IrCl2]2 |
1,2-DCE |
None |
20 |
8 |
None |
1,2-DCE |
None |
0 |
9 |
[Cp*IrCl2]2 |
DMF |
None |
0 |
10 |
[Cp*IrCl2]2 |
DMSO |
None |
0 |
11 |
[Cp*IrCl2]2 |
H2O |
None |
35 |
12 |
[Cp*IrCl2]2 |
CH3CN |
None |
0 |
13 |
[Cp*IrCl2]2 |
CH3CH2OH |
None |
0 |
14 |
[Cp*IrCl2]2 |
H2O |
NaHCO3 |
0 |
15 |
[Cp*IrCl2]2 |
H2O |
KPF6 |
0 |
16 |
[Cp*IrCl2]2 |
H2O |
Na2CO3 |
0 |
17 |
[Cp*IrCl2]2 |
H2O |
K2CO3 |
0 |
18 |
[Cp*IrCl2]2 |
H2O |
NaOH |
0 |
19 |
[Cp*IrCl2]2 |
H2O |
KOH |
0 |
20 |
[Cp*IrCl2]2 |
H2O |
AcOH |
Trace |
21 |
[Cp*IrCl2]2 |
H2O |
PivOH |
Trace |
22 |
[Cp*IrCl2]2 |
H2O |
HBF4 |
Trace |
23 |
[Cp*IrCl2]2 |
H2O |
Benzoic acid |
37 |
24 |
[[Cp*IrCl2]2 |
H2O |
TsOH |
Trace |
25 |
[Cp*IrCl2]2 |
H2O |
MesCOOH |
46 |
26 |
[Cp*IrCl2]2 |
H2O |
o-Nitrobenzoic acid |
41 |
27 |
[Cp*IrCl2]2 |
H2O |
1-AdCOOH |
40 |
28 |
[Cp*IrCl2]2 |
H2O |
C6F5COOH |
86 |
29 |
[Cp*IrCl2]2 |
H2O |
N-Acetylglycine |
0 |
30 |
[Cp*IrCl2]2 |
H2O |
Dipicolinic acid |
0 |
31 |
[Cp*IrCl2]2 |
H2O |
Quinaldic acid |
0 |
32 |
[Cp*IrCl2]2 |
1,2-DCE |
C6F5COOH |
80 |
33 |
[Cp*IrCl2]2 |
DMF |
C6F5COOH |
0 |
34 |
[Cp*IrCl2]2 |
DMSO |
C6F5COOH |
0 |
36 |
[Cp*IrCl2]2 |
CH3CH2OH |
C6F5COOH |
0 |
37 |
[Cp*IrCl2]2 |
CH3CN |
C6F5COOH |
0 |
38b |
[Cp*IrCl2]2 |
H2O |
C6F5COOH |
56 |
With the optimized reaction conditions in hand, we began to explore the generality and selectivity of the Ir-catalyzed C–N bond coupling by using sulfonyl azide as coupling partner (Table 2). The substrates with various sulfonyl azides derivatives bearing desirable functionality, such as phenyl, benzyl, naphthyl, alkyl, and heterocyclyl, proceeded efficiently, providing access to functionalized 1,2,4-thiadiazole derivatives (2aa–2bh) with yields ranging from 50 to 90%. The substituent was at the m-position of benzene ring proved to be amenable to this reaction better, most substrates delivering the corresponding products in good yields (2aa–2aj). In contrast, for the substrates with para-substituents or no substituent exhibited diminished reactivity (2ak–2bg). We speculated that the reason for this result is that substituent in the m-position of the benzene ring, almost no diaminylation products are formed due to steric hindrance, however, when there is no substituent group on the benzene ring or the substituent group is in para position, there will be diaminylation products were formed, resulting in a corresponding decrease in the yield of monoaminylation product. When 3,5-di(naphthalen-2-yl)-1,2,4-thiadiazole or 3,5-diphenyl-1,2,4-oxadiazole as material, they can also react with 4-methylbenzenesulfonyl azide to obtain corresponding product (2bh, 2bi). In addition, the molecular structure of product 2ak was further confirmed by X-ray crystallography.
Table 2 Reaction between 1,2,4-thiadiazoles and sulfonyl azidesa,b,c
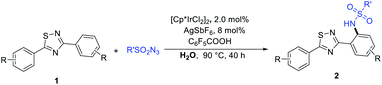
|
Reaction conditions: 1 0.2 mmol, R′SO2N3 0.3 mmol, [Cp*IrCl2]2 2.0 mol%, AgSbF6 8.0 mol%, C6F5COOH 40 mol%, H2O 1.0 mL, 90 °C, 40 h. Reaction conditions: 1 0.2 mmol, R′SO2N3 0.3 mmol, [Cp*IrCl2]2 2.0 mol%, AgSbF6 8.0 mol%, C6F5COONa 40 mol%, H2O 1.0 mL, 90 °C, 40 h. Isolated yield. |
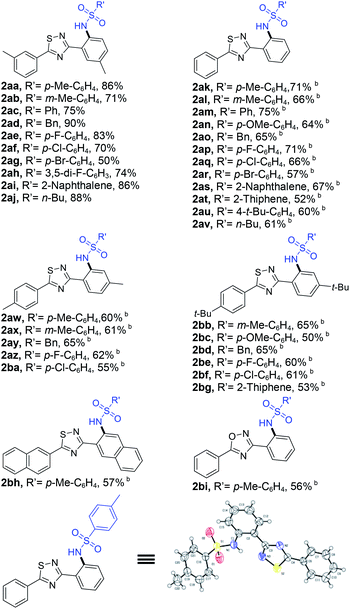 |
To demonstrate the applicability of the reaction system, we next investigated its efficacy on N,3-diphenyl-1,2,4-thiadiazol-5-amine derivatives. To our delight, the corresponding products can also be isolated in moderate yields (Table 3).
Table 3 Reaction between N,3-diphenyl-1,2,4-thiadiazol-5-amines and sulfonyl azidesa,b,c

|
Reaction conditions: 3 0.2 mmol, R′SO2N3 0.3 mmol, [Cp*IrCl2]2 2.0 mol%, AgSbF6 8.0 mol%, C6F5COOH 40 mol%, H2O 1.0 mL, 90 °C, 40 h. Reaction conditions: 3 0.2 mmol, R′SO2N3 0.3 mmol, [Cp*IrCl2]2 2.0 mol%, AgSbF6 8.0 mol%, C6F5COONa 40 mol%, H2O 1.0 mL, 90 °C, 40 h. Isolated yield. |
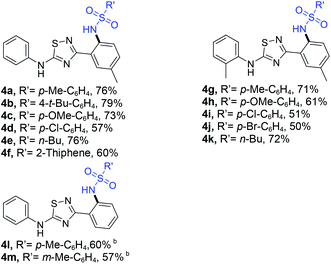 |
A series of control experiments were further carried out to study the reaction mechanism. A significant level of deuterium incorporation (95%) was observed at the ortho position of the red benzene ring when it was subjected to the Ir-catalytic system in D2O in the absence of sulfonyl azides to suggest that the C–H bond cleavage is reversible (Scheme 2a). Furthermore, the experimental KIE value indicated that the cleavage of the C–H bond might play a significant role in the reaction (Scheme 2b).
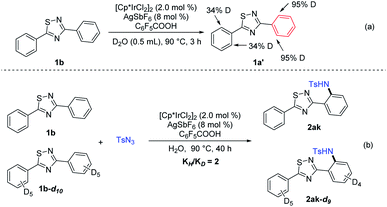 |
| Scheme 2 Mechanism studies. | |
Based on the experimental date and previous literature reports,12 a possible reaction mechanism was discussed (Scheme 3). First, treatment of the [Cp*IrCl2]2 precursor in the presence of AgSbF6 and C6F5COOH generates catalytic species (I) then, the catalytic species (I) coordinates with the nitrogen atom of 1,2,4-thiadiazoles (1b) and then undergoes the C–H metalation process to form intermediate II. After that, the catalyst in intermediate II coordinates with TsN3 to form complex III, which subsequently goes through migratory insertion directly by releasing a nitrogen molecule to deliver a six-membered cyclometalated intermediate IV. Finally, desired product (2ak) is obtained through protonation and the catalytic species (I) is released to continue the catalytic cycle.
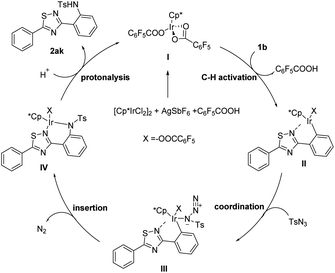 |
| Scheme 3 Proposed mechanism. | |
Conclusions
In summary, we have developed an iridium-catalyzed direct C–N cross-coupling of 1,2,4-thiadiazoles with sulfonyl azides in water. The amidation releases N2 as the single byproduct and the reaction showed excellent regioselectivity. Further efforts on the application of 1,2,4-thiadiazoles derivatives are currently underway in our laboratory.
Conflicts of interest
There are no conflicts to declare.
Acknowledgements
This work was supported by National Natural Science Foundation of China (No. 81671742), Doctoral Scientific Research Foundation of Jining Medical University (No. 6001/600763002), College Students' Innovative Training Program of Jining Medical University (No. cx2019052), NFSC cultivation Project of Jining Medical University (TYP2018KJ02), and Liaoning Revitalization Talents Program (No. XLYC1807037).
Notes and references
-
(a) A. Góblyös, H. Vries, J. Brussee and A. P. IJzerman, J. Med. Chem., 2005, 48, 1145–1151 CrossRef;
(b) M. Boulhaoua, A. Torvisco and T. Pasinszki, Res. Chem. Intermed., 2019, 46, 1507–1519 CrossRef;
(c) G. F. Makhaeva, N. V. Kovaleva, N. P. Boltneva, S. V. Lushchekina, E. V. Rudakova, T. S. Stupina, A. A. Terentiev, I. V. Serkov, A. N. Proshin, E. V. Radchenko, V. A. Palyulin, S. O. Bachurin and R. J. Richardson, Bioorg. Chem., 2020, 94, 103387–110400 CrossRef CAS;
(d) A. S. Mayhoub, L. Marler, T. P. Kondratyuk, E. J. Park, J. M. Pezzuto and M. Cushman, Bioorg. Med. Chem., 2012, 20, 510–520 CrossRef CAS PubMed;
(e) A. Pomeislova, M. Otmar, P. Rubesova, J. Benysek, M. Matousova, H. Mertlikova-Kaiserova, R. Pohl, L. Postova Slavetinska, K. Pomeisl and M. Krecmerova, Bioorg. Med. Chem., 2021, 32, 115998 CrossRef CAS PubMed;
(f) Y. J. Pragathi, R. Sreenivasulu, D. Veronica and R. R. Raju, Arabian J. Sci. Eng., 2020, 1–8 Search PubMed;
(g) M. Promzeleva, T. Volkova, A. Proshin, O. Siluykov, A. Mazur, P. Tolstoy, S. Ivanov, F. Kamilov and I. Terekhova, ACS Biomater. Sci. Eng., 2018, 4, 491–501 CrossRef CAS PubMed;
(h) R. M. Rodriguez Sarmiento, C. Bissantz, J. Bylund, A. Limberg, W. Neidhart, R. Jakob-Roetne, L. Wang and K. Baumann, J. Med. Chem., 2020, 63, 8534–8553 CrossRef CAS PubMed;
(i) C. Strecker, H. Peters, T. Hackl, T. Peters and B. Meyer, ChemMedChem, 2019, 14, 1336–1342 CrossRef CAS PubMed.
-
(a) D. G. Anstis, A. C. Lindsay, T. Sohnel and J. Sperry, J. Nat. Prod., 2020, 83, 1721–1724 CrossRef CAS;
(b) M. Chen, S. Lin, L. Li, C. Zhu, X. Wang, Y. Wang, B. Jiang, S. Wang, Y. Li, J. Jiang and J. Shi, Org. Lett., 2012, 14, 5668–5671 CrossRef CAS PubMed;
(c) C. D. Pham, H. Weber, R. Hartmann, V. Wray, W. Lin, D. Lai and P. Proksch, Org. Lett., 2013, 15, 2230–2233 CrossRef CAS PubMed.
-
(a) T. V. Volkova, E. N. Domanina, M. V. Chislov, A. N. Proshin and I. V. Terekhova, J. Therm. Anal. Calorim., 2019, 140, 2305–2315 CrossRef;
(b) W. Yu, H. Zhang, P. A. Yin, F. Zhou, Z. Wang, W. Wu, Q. Peng, H. Jiang and B. Z. Tang, iScience, 2020, 23, 101587 CrossRef CAS;
(c) R. Guo, W. Zhang, Q. Zhang, X. Lv and L. Wang, Front. Optoelectron., 2019, 11, 375–384 CrossRef;
(d) E. Podda, M. Arca, S. J. Coles, M. Crespo Alonso, F. Isaia, A. Pintus, V. Lippolis and M. C. Aragoni, Supramol. Chem., 2020, 32, 267–275 CrossRef CAS.
-
(a) D. J. Abrams, P. A. Provencher and E. J. Sorensen, Chem. Soc. Rev., 2018, 47, 8925–8967 RSC;
(b) Z. Cao, Q. Zhu, Y. W. Lin and W. M. He, Chin. Chem. Lett., 2019, 30, 2132–2138 CrossRef CAS;
(c) X. L. Han, P. P. Lin and Q. Li, Chin. Chem. Lett., 2019, 30, 1495–1502 CrossRef CAS;
(d) K. J. Jiao, Y. K. Xing, Q. L. Yang, H. Qiu and T. S. Mei, Acc. Chem. Res., 2020, 53, 300–310 CrossRef CAS;
(e) N. Y. S. Lam, K. Wu and J. Q. Yu, Angew. Chem., Int. Ed., 2021, 60, 2–26 CrossRef;
(f) S. Rej, Y. Ano and N. Chatani, Chem. Rev., 2020, 120, 1788–1887 CrossRef CAS;
(g) M. Shankar, R. K. Rit, S. Sau, K. Mukherjee, V. Gandon and A. K. Sahoo, Chem. Sci., 2020, 11, 10770–10777 RSC;
(h) Q. Shao, K. Wu, Z. Zhuang, S. Qian and J. Q. Yu, Acc. Chem. Res., 2020, 53, 833–851 CrossRef CAS PubMed;
(i) C. Shen, A. Wang, J. Xu, Z. An, K. Y. Loh, P. Zhang and X. Liu, Chem, 2019, 5, 1059–1107 CrossRef CAS;
(j) S. Tian, T. Luo, Y. Zhu and J.-P. Wan, Chin. Chem. Lett., 2020, 31, 3073–3082 CrossRef CAS;
(k) Y. Cheng, S. Yu, Y. He, G. An, G. Li and Z. Yang, Chem. Sci., 2021, 12, 3216–3225 RSC;
(l) H. Zhang, J. Xu, M. Zhou, J. Zhao, P. Zhang and W. Li, Org. Biomol. Chem., 2019, 17, 10201–10208 RSC.
-
(a) A. K. Ghosh, P. Ghosh and A. Hajra, J. Org. Chem., 2020, 85, 15752–15759 CrossRef CAS PubMed;
(b) Y. Q. Han, Q. Zhang, X. Yang, M. X. Jiang, Y. Ding and B. F. Shi, Org. Lett., 2021, 23, 97–101 CrossRef CAS PubMed;
(c) Q. H. Nguyen, S. M. Guo, T. Royal, O. Baudoin and N. Cramer, J. Am. Chem. Soc., 2020, 142, 2161–2167 CrossRef CAS PubMed;
(d) P. J. Sarver, V. Bacauanu, D. M. Schultz and D. A. DiRocco, Nat. Chem., 2020, 12, 459–467 CrossRef CAS PubMed;
(e) Q. Wang, P. Wang, X. Gao, D. Wang, S. Wang, X. Liang, L. Wang, H. Zhang and A. Lei, Chem. Sci., 2020, 11, 2181–2186 RSC;
(f) S. Yu, N. Lv, Z. Liu and Y. Zhang, Adv. Synth. Catal., 2019, 362, 118–125 CrossRef;
(g) J. Xu, K. Cheng, C. Shen, R. Bai, Y. Xie and P. Zhang, ChemCatChem, 2018, 10, 965–970 CrossRef CAS;
(h) B. B. Zhan, M. X. Jiang and B. F. Shi, Chem. Commun., 2020, 56, 13950–13958 RSC;
(i) Y. Zhang, Z. Lin and L. Ackermann, Chem, 2021, 27, 242–246 CrossRef CAS;
(j) Z. Zhuang, A. N. Herron, S. Liu and J. Q. Yu, J. Am. Chem. Soc., 2021, 143, 687–692 CrossRef CAS PubMed;
(k) Y. Cheng, Y. He, J. Zheng, H. Yang, J. Liu, G. An and G. Li, Chin. Chem. Lett., 2021, 32, 1437–1441 CrossRef CAS;
(l) J. Xu, L. Huang, L. He, Z. Ni, J. Shen, X. Li, K. Chen, W. Li and P. Zhang, Green Chem., 2021, 23, 2123–2129 RSC.
-
(a) Y. Kong, W. Xu, X. Liu and J. Weng, Chin. Chem. Lett., 2020, 31, 3245–3249 CrossRef CAS;
(b) S. Peng, Y. X. Song, J. Y. He, S. S. Tang, J. X. Tan, Z. Cao, Y. W. Lin and W. M. He, Chin. Chem. Lett., 2019, 30, 2287–2290 CrossRef CAS;
(c) J. Shen, J. Xu, L. Huang, Q. Zhu and P. Zhang, Adv. Synth. Catal., 2019, 362, 230–241 CrossRef;
(d) Z. Wei, S. Qi, Y. Xu, H. Liu, J. Wu, H. Li, C. Xia and G. Duan, Adv. Synth. Catal., 2020, 361, 5490–5498 CrossRef;
(e) J. Xu, H. Yang, L. He, L. Huang, J. Shen and W. Li, Org. Lett., 2021, 23, 195–201 CrossRef CAS PubMed;
(f) J. Xu, H. Zhang, J. Zhao, Z. Ni, P. Zhang, B. F. Shi and W. Li, Org. Chem. Front., 2020, 7, 4031–4042 RSC;
(g) C. Zhang, Y. Cheng, F. Li, Y. Luan, P. Li and W. Li, Adv. Synth. Catal., 2020, 362, 1286–1291 CrossRef CAS;
(h) C. Tian, Q. Wang, X. Wang, G. An and G. Li, J. Org. Chem., 2019, 84, 14241–14247 CrossRef CAS;
(i) C. Shen, M. Yang, J. Xu, C. Chen, K. Zheng, J. Shen and P. Zhang, RSC Adv., 2017, 7, 49436–49439 RSC.
-
(a) S. Cembellín, T. Dalton, T. Pinkert, F. Schäfers and F. Glorius, ACS Catal., 2019, 10, 197–202 CrossRef;
(b) Z. Duan, L. Zhang, W. Zhang, L. Lu, L. Zeng, R. Shi and A. Lei, ACS Catal., 2020, 10, 3828–3831 CrossRef CAS;
(c) Y. Huang, C. Pi, Z. Tang, Y. Wu and X. Cui, Chin. Chem. Lett., 2020, 31, 3237–3240 CrossRef CAS;
(d) M. Liu, L. J. Li, J. Zhang, H. Xu and H. X. Dai, Chin. Chem. Lett., 2020, 31, 1301–1304 CrossRef CAS;
(e) N. Lv, S. Yu, C. Hong, D. M. Han and Y. Zhang, Org. Lett., 2020, 22, 9308–9312 CrossRef CAS PubMed;
(f) W. Wang, J. Wu, R. Kuniyil, A. Kopp, R. N. Lima and L. Ackermann, Chem, 2020, 6, 3428–3439 CrossRef CAS;
(g) S. Zhang, B. Wang, X. Jia and Y. Yuan, Adv. Synth. Catal., 2018, 361, 451–455 CrossRef;
(h) W. Zhang, S. Lou, Y. Liu and Z. Xu, J. Org. Chem., 2013, 78, 5932–5948 CrossRef CAS PubMed.
- T. Tian, A. S. Dong, D. Chen, X. T. Cao and G. Wang, Org. Biomol. Chem., 2019, 17, 7664–7668 RSC.
-
(a) L. J. Scott and C. M. Perry, Drugs, 2000, 60, 1411–1444 CrossRef CAS PubMed;
(b) J. Q. Tran, J. G. Gerber and B. M. Kerr, Clin. Pharmacokinet., 2001, 40, 207–226 CrossRef CAS PubMed;
(c) A. Hauschild, J. J. Grob, L. V. Demidov, T. Jouary, R. Gutzmer, M. Millward, P. Rutkowski, C. U. Blank, W. H. Miller Jr and E. Kaempgen, Lancet, 2012, 380, 358–365 CrossRef CAS.
-
(a) V. S. Thirunavukkarasu, K. Raghuvanshi and L. Ackermann, Org. Lett., 2014, 47, 281–295 Search PubMed;
(b) M. Bakthadoss, P. V. Kumar, R. Kumar, M. Surendar and D. S. Sharada, New J. Chem., 2019, 43, 14190–14195 RSC;
(c) J. Kim, J. Kim and S. Chang, Chem.–Eur. J., 2013, 19, 7328–7333 CrossRef CAS PubMed;
(d) C. Pan, A. Abdukader, J. Han, Y. Cheng and C. Zhu, Chem.–Eur. J., 2014, 20, 3606–3609 CrossRef CAS PubMed;
(e) X. Xiao, G. Jia, F. Liu, G. Ou and Y. Xie, J. Org. Chem., 2018, 83, 13811–13820 CrossRef CAS PubMed;
(f) L. L. Zhang, L. H. Li, Y. Q. Wang, Y. F. Yang, X. Y. Liu and Y. M. Liang, Organometallics, 2014, 33, 1905–1908 CrossRef CAS;
(g) Q. Z. Zheng, Y. F. Liang, C. Qin and N. Jiao, Chem. Commun., 2013, 49, 5654–5656 RSC.
-
(a) J. Y. Kim, S. H. Park, J. Ryu, S. H. Cho, S. H. Kim and S. Chang, J. Am. Chem. Soc., 2012, 134, 9110–9113 CrossRef CAS PubMed;
(b) S. H. Park, J. Kwak, K. Shin, J. Ryu, Y. Park and S. Chang, J. Am. Chem. Soc., 2014, 136, 2492–2502 CrossRef CAS PubMed;
(c) R. Yamada, N. Iwasawa and J. Takaya, Angew. Chem., Int. Ed., 2019, 58, 17251–17254 CrossRef CAS PubMed.
-
(a) S. Chen, B. Feng, X. Zheng, J. Yin, S. Yang and J. You, Org. Lett., 2017, 19, 2502–2505 CrossRef CAS PubMed;
(b) D. Das and R. Samanta, Adv. Synth. Catal., 2018, 360, 379–384 CrossRef CAS;
(c) Y. Feng, Z. Zhang, Q. Fu, Q. Yao, H. Huang, J. Shen and X. Cui, Chin. Chem. Lett., 2020, 31, 58–60 CrossRef CAS;
(d) G. N. Hermann, P. Becker and C. Bolm, Angew. Chem., Int. Ed., 2016, 55, 3781–3784 CrossRef CAS PubMed;
(e) H. Hou, Y. Zhao, S. Sheng and J. Chen, Adv. Synth. Catal., 2019, 361, 4393–4398 CrossRef CAS;
(f) L. Y. Jiao, Z. H. Ning, Q. Hong, X. H. Peng, X. M. Yin, S. Liu, H. Chen, Z. Li, M. Sun and X. X. Ma, RSC Adv., 2020, 10, 29712–29722 RSC;
(g) J. Kim and S. Chang, Angew. Chem., Int. Ed., 2014, 53, 2203–2207 CrossRef CAS PubMed;
(h) V. Lanke and K. R. Prabhu, Chem. Commun., 2017, 53, 5117–5120 RSC;
(i) D. Lee, Y. Kim and S. Chang, J. Org. Chem., 2013, 78, 11102–11109 CrossRef CAS PubMed;
(j) M. Maraswami, G. Chen and T.-P. Loh, Adv. Synth. Catal., 2018, 360, 416–421 CrossRef CAS;
(k) H. Xiong, Y. Gu, S. Zhang, F. Lu, Q. Ji, L. Liu, P. Ma, G. Yang, W. Hou and H. Xu, Chem. Commun., 2020, 56, 4692–4695 RSC;
(l) S. W. Yuan, H. Han, Y. L. Li, X. Wu, X. Bao, Z. Y. Gu and J. B. Xia, Angew. Chem., Int. Ed., 2019, 58, 8887–8892 CrossRef CAS PubMed;
(m) H. Xie, M. Zhong, X. T. Wang, J. Q. Wu, Y. Q. Cai, J. Liu, B. Shu, T. Che and S. S. Zhang, Org. Chem. Front., 2021, 8, 635–642 RSC.
- P. Patel and S. Chang, ACS Catal., 2015, 5, 853–858 CrossRef CAS.
- X. Kong, L. Lin and B. Xu, Adv. Synth. Catal., 2018, 360, 2801–2805 CrossRef CAS.
- J. Peng, Z. Xie, M. Chen, J. Wang and Q. Zhu, Org. Lett., 2014, 16, 4702–4705 CrossRef CAS.
-
(a) T. Kitanosono, T. Hisada, Y. Yamashita and S. Kobayashi, Angew. Chem., Int. Ed., 2021, 60, 3407–3411 CrossRef CAS PubMed;
(b) T. Kitanosono and S. Kobayashi, Chem.–Eur. J., 2020, 26, 9408–9429 CrossRef CAS PubMed;
(c) T. R Pradhan and P. J. Kyoon, Adv. Synth. Catal., 2020, 362, 4833–4860 CrossRef CAS;
(d) Y. Huang, L. Xu, F. Yu, W. Shen, X. Lu, L. Ding, L. Zhong, G. Zhong and J. Zhang, J. Org. Chem., 2020, 85, 7225–7237 CrossRef CAS;
(e) T. Mitra, M. Kundu and B. Roy, J. Org. Chem., 2020, 85, 345–359 CrossRef CAS PubMed;
(f) P. Kang and Z. B. Dong, Adv. Synth. Catal., 2021, 363, 1185–1201 CrossRef;
(g) X. Wang, J. Zhang, Y. He, D. Chen, C. Wang, F. Yang, W. Wang, Y. Ma and M. Szostak, Org. Lett., 2020, 22, 5187–5192 CrossRef CAS PubMed;
(h) R. Nie, R. Lai, S. Lv, Y. Xu, L. Guo, Q. Wang and Y. Wu, Chem. Commun., 2019, 55, 11418–11421 RSC;
(i) A. Mandal, R. Bera and M. Baidya, J. Org. Chem., 2021, 86, 62–73 CrossRef CAS PubMed.
Footnote |
† Electronic supplementary information (ESI) available. CCDC 2081944. For ESI and crystallographic data in CIF or other electronic format see DOI: 10.1039/d1ra04450h |
|
This journal is © The Royal Society of Chemistry 2021 |
Click here to see how this site uses Cookies. View our privacy policy here.