DOI:
10.1039/D1RA04513J
(Paper)
RSC Adv., 2021,
11, 34613-34630
Microbial transglutaminase nanoflowers as an alternative nanomedicine for breast cancer theranostics†
Received
10th June 2021
, Accepted 18th October 2021
First published on 26th October 2021
Abstract
Breast cancer is the most common malignancy among women. With the aim of decreasing the toxicity of conventional breast cancer treatments, an alternative that could provide appropriate and effective drug utilization was envisioned. Thus, we contemplated and compared the in vitro effects of microbial transglutaminase nanoflowers (MTGase NFs) on breast cancer cells (MCF-7). Transglutaminase is an important regulatory enzyme acting as a site-specific cross-linker for proteins. With the versatility of MTGase facilitating the nanoflower formation by acting as molecular glue, it was demonstrated to have anti-cancer properties. The rational drug design based on a transglutaminase enzyme-assisted approach led to the uniform shape of petals in these nanoflowers, which had the capacity to act directly as an anti-cancer drug. Herein, we report the anti-cancer characteristics portrayed by enzymatic MTGase NFs, which are biocompatible in nature. This study demonstrated the prognostic and therapeutic significance of MTGase NFs as a nano-drug in breast cancer treatment. The results on MCF-7 cells showed a significantly improved in vitro therapeutic efficacy. MTGase NFs were able to exhibit inhibitory effects on cell viability (IC50-8.23 μg ml−1) within 24 h of dosage. To further substantiate its superior anti-proliferative role, the clonogenic potential was measured to be 62.8%, along with migratory inhibition of cells (3.76-fold change). Drastic perturbations were induced (4.61-fold increase in G0/G1 phase arrest), pointed towards apoptotic induction with a 58.9% effect. These results validated the role of MTGase NFs possessing a cytotoxic nature in mitigating breast cancer. Thus, MTGase bestows distinct functionality towards therapeutic nano-modality, i.e., nanoflowers, which shows promise in cancer treatment.
1. Introduction
Breast cancer is the most common malignancy and one of the leading causes of death among women.1,2 According to the GLOBOCAN (global cancer statistics) 2018 data, there are approximately 2.1 million new cases of breast cancer worldwide.3 It is well known that changes in the structure or behaviour of genes are responsible for the development of breast cancer (BC). For instance, activation of oncogenes and alteration of tumor suppressor gene pathways lead to the development of cancer.4,5 The choice of therapy is determined by the size of the primary tumor, the degree of malignancy, and the severity of the disease.6 During the treatment, it is crucial to inhibit the formation of new blood vessels (angiogenesis), which is responsible for the sustenance of tumor tissues.7
The potential risk and benefits associated with each option are taken into account as there is currently no specific way to determine the progressive potential of lesions terminating into tumors.4,6 One of the most important therapeutic methods for BCs is conventional chemotherapy along with surgical methods and radiotherapy.6,8,9 Patients with a metastatic stage (invasive BC) are primarily treated with systemic therapies, including chemotherapy, targeted therapy, hormonal therapy, and more recently, immunotherapy.5–8 To reduce the growth and division of cancerous cells, different drugs or their combinations are used in combating the disease.9 However, proven disadvantages such as side effects, inability to adjust the dosage, failure to reach the desired region effectively, and efficiency loss before the targeted area is reached are associated with the conventional chemotherapeutic drugs.10,11 Nevertheless, the relapse often occurs, which is the major drawback in the usual course of action following a diagnosis of BC.5,11 Besides, they damage healthy cells and tissues, thereby limiting the treatment responses.
About 83% of BCs are hormonal (endocrine) receptor-positive (HR+) and can be treated with hormonal therapy to block the effects of estrogen on the growth of breast cancer cells.6,12 For premenopausal women, Tamoxifen for up to 10 years is the gold standard treatment; however, the combination of ovarian suppression and either Tamoxifen or an aromatase inhibitor (i.e., Letrozole, Anastrozole, and Exemestane) is recommended for those women with a high risk of recurrence.12–14 Around 15–25% of patients with breast cancer have HER2-positive tumors, and overexpression of HER2 is observed in these patients.15 Trastuzumab has been shown to induce tumor regression in such patients.16 Sunitinib, Sorafenib, and Bevacizumab are multi-targeted tyrosine kinase inhibitors that inhibit tumor neovascularization and are currently in clinical trials.17,18 These drugs are associated with serious problems such as adverse effects, drug resistance, and low efficacy of single therapy, particularly against metastatic or recurrent BCs.19,20 Hormone therapy has also been used against hormone receptor-positive breast cancer.6,21 However, about 10 to 15% of BCs lack either estrogen or progesterone receptor (ER and PgR, respectively) and do not overexpress the HER2 gene.22
The response rate among patients with metastatic breast cancer has decreased, possibly due to the tumor's resistance to a wide range of cancer therapies.23 It must be noted that metastatic BC is resistant to all forms of systemic treatments (hormonal, chemotherapy, and targeted) resulted in an estimated 90% or more of patients reporting remission.24,25 Thus, an alternative drug option needs to be developed that can provide an appropriate and effective treatment without any side effects.26 With the limitations in the use of calibrated systems for targeted drug delivery systems, the enzyme-controlled drug delivery systems could be the way forward for breast-specific targeting bestowed with the regulated release of drugs.27–29 Despite the therapeutic benefits of first-generation pharmaceutical formulations (like improving the overall survival rate and progression-free survival of patients), the presence of corrosive chemicals like Cremophor EL and ethanol in the formulations exert serious adverse effects.30,31 Hence, with all these conventional approaches, there is a dire need for a safer and more efficacious therapeutic option.32
Nanoflowers are a distinctive subset of nanomaterials that resemble flowers when examined under a microscope. The study underlines that these cargo-loading nanoflowers have a branched floral morphology and tailored petal structure. The well-framed multi-dimensional conformation of nanoflowers conferred by enzyme MTGase is the highlight of the work. Nanoflowers (NFs) have garnered attention for their superior features, which have tackled the bottlenecks prevailing in conventional immobilized enzyme preparations and 1-D/2-D nanomaterials. With their exquisite topographical features of nanolayers, they have a high surface-to-volume ratio.
Nanodelivery is promising as it overcomes adverse side effects or inadequate pharmacodynamics parameters.33 It also helps in reducing the therapeutic dosage required for effective therapy while also minimizing the systemic side effects of chemotherapeutic agents. The conventional treatments which are used as in standard therapy develop chemoresistance leading to poor patient outcomes.34,35 Moreover, bulk chemotherapy and drug-release strategies for cancer treatment have been associated with a lack of specificity and high drug concentrations that often result in toxic side effects. Another drawback associated is the elimination of cancer stem cells (CSCs) which are the promising targets for cancer chemotherapy by conventional chemotherapies.36 Several chemotherapeutic agents delivered using nano-carriers like polymeric nanoparticles (NPs)/micelles, metallic/inorganic NPs, and lipid-based NPs [liposome, solid–lipid nanoparticles (SLNs), and nanostructured lipid carriers (NLCs)], etc. have been reported in the treatment of normal BC/triple negative (TNBC) along with breast cancer stem cells.9,37
Apart from the present well-known anti-cancer drugs, there's a need to develop more effective nano-formulations for safer and more efficacious therapy.32,33,38,39 With this perspective, we designed our novel study based on microbial transglutaminase nanoflowers, which to the best of our knowledge, is a new and state-of-art approach. For anti-cancer therapy, this will be the first anticipated novel enzymatic transglutaminase nanoflowers coming into action as a nano-drug.
The aim of decreasing toxicity levels of standard breast cancer treatment and increasing patients' survival chances has led towards exploring natural bio-active components.40,41 It has been anticipated to yield positive and encouraging results for BC treatment. Thus, we envisioned and compared in vitro effects of microbial transglutaminase nanoflowers (MTGase NFs) on human breast cancer MCF-7 cells. The enzymatic nature of nanoflower has certain defined advantages over conventional chemotherapeutic agents. It being nano-sized (<50 nm) will have greater penetration into the tumor. They can overcome the hindrance faced in cellular uptake as encountered in breast cancer cases and combat the limitations during drug conveyance. Its enzymatic nature shall ensure no adverse effect on surrounding normal cells. Our aim was to come up with the simplest design to avoid complex chemistry and not to use organic solvents to prevent non-specific uptake and biocompatibility along with economic benefits. Instantaneous self-assembly formation, efficient enzyme load complexation, and cellular uptake by cancer cells (shown by appropriate analytical methods) were successfully utilized to synthesize and choose the best formulation that could be used for a future nanomedicine for breast cancer. Microbial transglutaminase has the edge over mammalian origin on account of calcium independence for its action, which prevents the chances of by-product formation (calcium–protein complexes) and has greater thermal stability.42–44 Microbial transglutaminase nanoflowers, in itself, as an anti-cancer drug have not been reported so far. We believe that MTGase with inherent cross-linking ability has a higher propensity to them in spontaneously creating nanoflower-like structure much more easily as compared to other enzymes or proteins.43,45 This led us to explore the process for developing bio-catalytically active microbial transglutaminase nanoflowers. MTGase serves as a glue that binds the petals together in the nanoflower.46 Transglutaminase nanoflowers offer the potential to overcome the problems associated with conventional commercial drugs by their multiple synergistic functions of maintaining high specificity and selectivity.47,48 With its inherent characteristics of site-specific delivery along with sustained and controlled release will be a major breakthrough. MTGase nanoflowers will be expected to combat the prevailing delivery system's major challenges, including in vivo instability, poor bioavailability, poor solubility, poor absorption in the body, issues with target-specific delivery, and tonic effectiveness, and probable adverse effects of drugs.49
Moreover, NFs share the advantages of being a novel drug delivery system (NDDS), such as increased dissolution rate, elevated oral bioavailability, improved in vivo pharmacokinetics, passive targetability to solid tumors and inflammatory sites due to the enhanced permeability and retention (EPR) effect.48,50 Hopefully, this new approach in our present study of testing natural biological sources for breast cancer research will carefully explore the anti-cancer properties of enzyme-microbial transglutaminase. This study explored and assessed the enzymatic MTGase NFs properties as an anti-cancer drug to address the issues of chemotherapies applied.
2. Materials and methods
2.1. Microbial transglutaminase production for designing of nanoflowers
Streptomyces mobaraensis NCIM 5208 was procured from the National Collection of Industrial Microorganisms (NCIM), National Chemical Laboratory, Pune, India. The culture was maintained on MGYP (containing g L−1: malt extract 3, glucose 10, yeast extract 3, and peptone 5, adjusted to pH-6.0) slants and stored at 4 °C. The strain was routinely sub-cultured for the production of the enzyme transglutaminase. In all experiments, the enzyme transglutaminase was indigenously produced from this culture after optimizing the production of the enzyme.44 Commercial mammalian transglutaminase (cat no. T5398) and all analytical grade chemicals were purchased from Sigma-Aldrich (St Louis, USA). The microbial growth media such as malt extract, glucose, yeast extract, and peptone were procured from Hi-Media Laboratories (Mumbai, India). All stock solutions were prepared with MilliQ-purified distilled water.
2.2. Cell cultures and treatment
Human embryonic kidney (HEK-293), immortalized human keratinocytes (HaCaT), human breast cancer (MCF-7), and human colorectal (HCT-116) cells were obtained from National Centre for Cell Sciences (NCCS) Pune, India. Cells were grown as monolayer cultures in Dulbecco's modified Eagle's medium (DMEM) containing 10% fetal bovine serum (FBS), 1% penicillin–streptomycin (50 μg ml−1), and glutamine (2 mM) in a humidified atmosphere of 5% CO2 at 37 °C in T-25 culture flasks and were sub-cultured twice a week.
2.3. Synthesis of microbial transglutaminase nanoflowers
To carry out the rational synthesis of MTGase nanoflowers, 240 mmol l−1 aqueous CuSO4 solution in Milli-Q water was added to 2× PBS (pH-7.4) containing 3 mg ml−1 microbial transglutaminase enzyme, followed by incubation at 4 °C for three days. The processing of nanoflowers was carried by sonicating the suspension for 15 min, later dried and filtered for characterization and applications (United Kingdom Patent No. 2111966.4; U.S. Patent No. 17/406,928; Indian Patent No. 202011035812).
The facile design of bud to blooming nanoflowers was finalized after optimizing various parameters. The possible range for the parameters in the study was analyzed and tested, varying the concentration, volume, temperature, pH, and incubation time.47,51,52 The changes were noticed in the morphology and characteristics of nanoflowers on account of interplaying factors; for example, the seeding component, MTGase, CuSO4, temperature gradient from low to high, pH spectrum from acidic to alkaline, etc. were taken into consideration to check the robustness of nanoflowers.53–57 The enzyme produced from the microbial source Streptomyces mobaraensis NCIM 5208 was utilized for the experimentation. The detailed description of designing and optimization of the blooming transglutaminase nanoflowers, along with their characterization for functionality, are specified on our patent (United Kingdom Patent No. 2111966.4; U.S. Patent No. 17/406,928; Indian Patent No. 202011035812).
The process was accelerated so that the design template could be observed in the form of crystals which, after three days, formed perfect nanoflowers. MTGase nanoflowers were able to tolerate cold temperature and had higher durability. The present work also signifies the fact that the MTGase enzyme is itself acting as a nano-base. This study highlighted that microbial transglutaminase serves the dual purpose firstly, as a template (nano-support) of nanoflowers, and secondly, the enzyme itself gets immobilized in nanoflowers during the process.58 The facile synthesis was carried out at a very low temperature (4 °C).58
2.4. Characterization of microbial transglutaminase nanoflowers
The synthesized MTGase transglutaminase nanoflowers were subjected to characterization. Their size, shape/morphology, surface, charge, microstructure, stability, and other physico-chemical attributes were carried out by SEM, FESEM, TEM, DLS, zeta potential, XRD, TGA, and AFM.
2.5. Cytotoxicity assay of microbial transglutaminase nanoflowers
The cytotoxic effect of microbial transglutaminase nanoflowers was assessed in various cancer cell lines, i.e., MCF-7, HaCaT, and HCT-116 cells, by the MTT assay.59 These cells were exposed to different concentrations of MTGase NFs. Briefly, cells were seeded at a number of 1 × 104 per well in flat-bottom 96-well plates (200 μl per well) in triplicates; the cells were further allowed to attach and grow for 24 h and subsequently exposed to different concentrations of MTGase NFs (3.12–100 μg ml−1) for 24 h. At the end of the treatment, the media was removed, and cells were incubated with 20 μl of MTT (5 mg ml−1 in PBS) in a fresh medium for 4 h at 37 °C. After 4 h, formazan crystals formed by mitochondrial reduction of MTT were solubilized in DMSO (150 μl per well), and the absorbance was read at 570 nm after 10 min incubation on the iMark Microplate Reader (BioRad, USA).60 Percent inhibition of cytotoxicity was calculated as a fraction of control (without MTGase NFs; untreated), and the cytotoxicity of MTGase NFs was expressed as IC50.61
2.6. Clonogenic assay of microbial transglutaminase nanoflowers
The colony formation assay was executed as per the reported method with slight modifications.62 Briefly, MCF-7 cells were seeded at a density of 500 cells per well in a medium containing 10% FBS in 6-well plates, allowed to adhere to the plate in appropriate dilutions to form colonies. MCF-7 cells were then treated with the above described range of concentrations of MTGase NFs (3.12–100 μg ml−1) for 24 h, and its control. Following the treatment, the cells were incubated for two weeks in a humidified incubator at 37 °C and 5% CO2. Intermittent changing of the medium was carried out every fourth day but with no further addition of formulations. After 14 days, visible colonies were processed for staining and imaging. Colonies were fixed after aspirating out the spent media and stained with 0.05% crystal violet in 100% methanol and counted using a digital colony counter.63,64 The number of colonies formed was represented as bar graphs to represent the difference in the effect of the treatment group from the control group (untreated cells). The study deciphered the degree of MTGase NFs cytotoxicity by determining the percent of plating efficiency calculated based on the following formula:
Plating efficiency = number of colonies formed/number of cells seeded × 100% |
2.7. Cell migration assay of microbial transglutaminase nanoflowers
To ascertain the effect on cell migration rate on treatment with the developed formulation, the wound healing scratch assay was performed. The wound healing assay was performed as established in the lab.65 Wound healing response was recorded as a number of migratory cells between the scratched areas. MCF-7 cells (5 × 105 cells per well) were cultured in 6-well plates for 48 h. After attainment of 80–90% confluency in cells, the confluent monolayers were scratched.66 The wound was created through the diameter of each well, using a p10 pipette tip. Cells were treated with developed MTGase NFs formulations for 24 h. The media was removed, cells were washed gently with PBS followed by supplementing with fresh media containing 1% FBS.67,68 Cells that had migrated across the wound were photographed (10× magnification) under a phase contrast microscope (Nikon Instruments Inc. Japan) at regular time intervals (0 to 24 h) embedded by free ImageJ software (version 1.50i, National Institute of Health, Bethesda, MD, USA). Images of the wound gaps were measured at three locations perpendicular to the scratch and expressed as percentage wound healing relative to initial scratch that was calculated by the following formula:
% wound closure = (wound area at 0 h − wound area at 24 h)/wound area at 0 h × 100 |
2.8. DNA cell cycle analysis of microbial transglutaminase nanoflowers
2.8.1 Assessment of cell cycle phases of microbial transglutaminase nanoflowers. Flow cytometric analysis was performed to evaluate the cyclic distribution of cells as per the reported method with slight modifications.69 Cell cycle regulation is one of the crucial mechanisms of anti-proliferation in cancers.1,70 Abnormalities of cell cycle regulators are associated with many carcinogenic processes.71,72 So, cell cycle regulators can be targeted and changed to be useful for treatment. MCF-7 cells (1 × 105 cells per well) in a 6-well plate were treated with 8.23 μg ml−1 concentration of MTGase NFs and incubated for 24 h. Cells were trypsinized, fixed in 70% ethanol (chilled), and stored overnight at 2–8 °C. Cells were washed, treated with DNase-free RNase (10 μg ml−1) for 15 min, stained by propidium iodide reagent (5 μg ml−1), and incubated for 30 min at 37 °C in the dark. 10
000 events were analyzed after a doublet discrimination module using a flow cytometer (BD FACSVerse™, San Jose, CA, USA) for cell cycle phase distribution.
2.9. FACS analysis-apoptosis assay of microbial transglutaminase nanoflowers
Apoptosis is a highly conserved process involving numerous signaling pathways that coordinate cellular changes throughout the cell death process.73,74 To quantify apoptosis, the MCF-7 cells were seeded. Approximately 7.5 × 105 cells per well were plated in 100 mm plates and were subjected to treatment with MTGase NFs (8.23 μg ml−1). After 24 h, cells were harvested and processed for apoptotic assay stained with propidium iodide and Annexin V-FITC (BD Biosciences, Franklin Lakes, NJ, USA), as per its prescribed protocol and analyzed by flow cytometry.75,76 Flow cytometry was performed within 1 h using a flow cytometry system (BD FACSVerse™, San Jose, CA, USA) to score Annexin V and/or PI-positive cells. All the experiments were performed in triplicate.
2.10. Statistical analysis
All the data generated were analyzed using GraphPad Prism 5.03 Software (GraphPad Software, San Diego, CA), and results are presented as the mean of three independent experiments. Statistical analysis was employed to assess the statistical significance between the control and treated groups. The p-values < 0.05 were considered statistically significant.
3. Results and discussion
3.1. Designing the enzymatic nano-formulations: MTGase nanoflowers
3.1.1 Insights into microbial transglutaminase nanoflowers. Nanoflowers, are nano-sized flower-like structures formed by intra-molecular interactions between the enzymes/proteins facilitated by metal ions leading to anisotropic growth of nano-petals and, consequently, the formation of a flower-like structure.47,48,77,78 Nanoflowers are endowed with diverse functionalities for biocatalytic applications.52,53,79 They represent a new and elegant approach in enzyme immobilization, possessing greatly enhanced activity, stability, durability, and even selectivity of entrapped organic biomolecules. The ultra-rapid, simple synthesis of producing immobilized enzymes acquires the hierarchical nanostructures showing structure similar to a flower with large surface-to-volume ratios (petals bloom).54,55,80 Thus, nanoflowers research is anticipated to take multiple directions for the development of drug delivery systems, biosensors, biocatalysts, and bio-related devices, etc.47,76Inorganic nanocrystals have been widely reported for applications in catalysis and analytical science. Organic–inorganic hybrid nanoflowers have received much attention due to their simple synthesis, high efficiency, and enzyme stabilizing ability.47,48,54,80 However, the preparation of enzymatic nanoflowers (organic) from microbial sources is yet to be explored. Thus, no enzymatic nanoflowers have been reported to date, which possesses a higher degree of cross-linking ability along with displaying a higher propensity in creating nanoflower structure. Further, none of the prior arts to date suggest a method of preparation of nanoflower utilizing the characteristic properties of transglutaminase.
There are a number of advantages of the MTGase nanoflowers as compared to the native transglutaminase enzyme. MTGase is a monomeric protein composed of 331 amino acids. It has a molecular weight of 38 kDa.43,82 These are a member of α + β folding class, with 10α-helices and 8β-strands. The characteristic features of MTGase with the secondary structure of a classical (α/β)8 sheets have a disk-like structure with a central groove.43 The active centre is comprised of a Cys–His–Asp triad. The wide active-site cleft accommodates the alpha-helix, leading to the broader substrate specificity with a higher reaction rate, which is the key to its cross-linking efficiency.83
This structural information reveals the presence of conserved amino acid residues responsible for MTGase catalytic activity. MTGase active site is specifically characterized by unique predominance of acidic (4 Asp and 2 Glu), aromatic and hydrophobic residues (7 Trp).43,84
The study discloses the synthesis of a new nanoflowers from transglutaminase of microbial origin at the lowest temperature (4 °C), which in turn provides catalytically more active, stable and reusable microbial transglutaminase nanoflower. It has an interesting flower-like structure on the nano-scale. The MTGase nanoflowers started appearing within a few hours in the bud stage; it bloomed completely on the third day and was envisaged to possess anti-cancerous properties along with a higher loading capacity of cargo for delivery of bio-actives or drugs. With its targeted binding on account of being non-toxic and biocompatible, the chances of any undesirable immunogenic reactions are negligible. Further, the high surface area to volume ratio presented by nanoflowers not only enhances the surface adsorption for accelerating the kinetics of bio-catalytic reactions, but also adds to higher loading and better charge transfer owing to its 3-D structure.77,85 The uniqueness of the present study was due to the replacement of the bovine serum albumin (BSA; the standard protein component) base by MTGase itself, thereby leading to unique morphology and size of the nanoflowers; after optimizing the concentration of MTGase, aging time, sonication, pH, temperature, PBS, and CuSO4 concentration for nano-formulation designing.47,78,79
3.2. Optimization of transglutaminase nanoflower design and their characterization
The design of MTGase nanoflowers under optimized reaction conditions is highlighted in Fig. 1a–f, which shows the formation of MTGase-incorporated CuSO4 nanoflowers captured by Scanning Electron Microscope (SEM) under optimized reaction conditions.
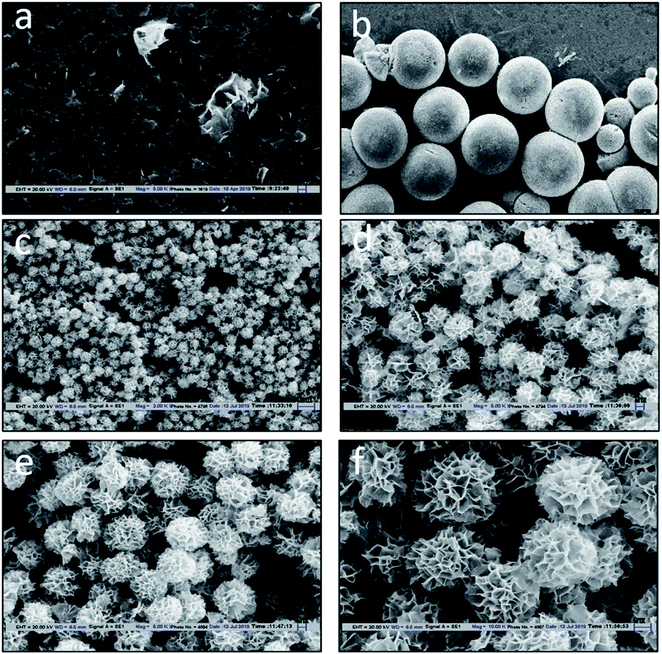 |
| Fig. 1 The process of MTGase nanoflowers design captured by Scanning Electron Microscope (SEM) images under optimized reaction conditions capturing the stages in the formation of MTGase mediated nanoflowers-bud to blooming stages of nanoflowers. Illustration of the architecture of MTGase nanoflowers; (a and b): flaky formation of petals crystals on the onset of floral bud synthesis; (c and d): buds transforming into flower-opening and (e and f): blooming nanoflowers pockets. Scale bar: 2 μm. | |
High-resolution of the porous structure of the petals was observed in SEM (Fig. 2a) and Field Emission Scanning Electron Microscope (FESEM) (Fig. 2b). The stages during the formation of MTGase mediated nanoflowers were well captured from flaky initiation to bud and later transforming into full-bloomed nanoflowers. The essence of the enzyme MTGase in nanoflowers was that its structure dictated the unique 3-D floral pattern.51 MTGase served as a molecular glue to bind the petals together in the nanoflowers.86 Copper ions were the inorganic elements for the initialization of nanoflowers and acted as chelating ions.47,79
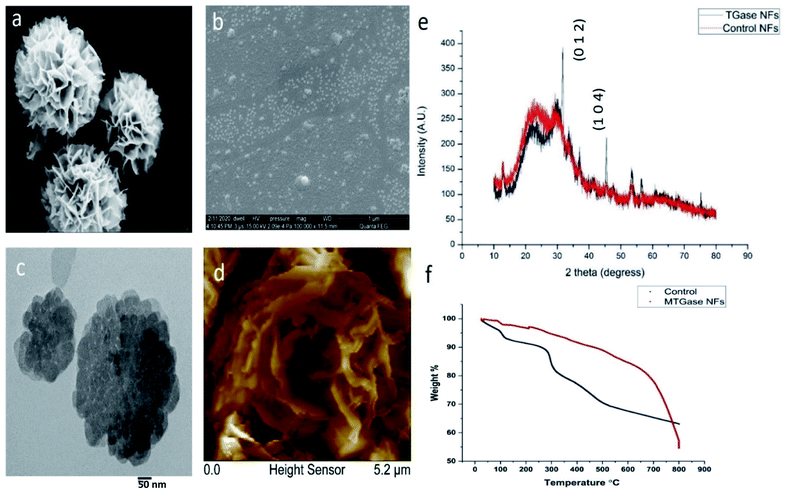 |
| Fig. 2 Microbial transglutaminase nanoflowers designing and characterization by (a) SEM, (b) FESEM, (c) TEM, (d) AFM, (e) XRD and (f) TGA analysis. The analysis of MTGase nanoflowers depicting uniform size using transmission electron microscopy (TEM); scale bar: 50 nm. The surface inspection in terms of topology and roughness measured by atomic force microscope (AFM). The MTGase enzyme structure presents a backbone more likelihood to acquire characteristic flower-like shape. The configuration and stereochemistry of MTGase enzyme led to shape nanoflowers as portrayed by X-ray diffraction (XRD), thermogravimetric analysis (TGA). In a nutshell, 3-D structure of enzyme was envisioned to explain the novelty of MTGase in ordaining the true nanoflowers blooming state which specifically diagnose and act as a therapeutic drug for breast cancer (United Kingdom Patent No. 2111966.4; U.S. Patent No. 17/406,928; Indian Patent No. 202011035812). | |
The characterization for the size of MTGase nanoflowers was carried out by Transmission Electron Microscopy (TEM) analysis. The image clearly showed the uniform size distribution of MTGase nanoflowers. The nucleation process was also remarkably marked by TEM micrographs (Fig. 2c). The TEM validated the nanoflowers being homo-dispersed and small-sized (<50 nm). Dynamic light scattering (DLS) analysis showed that the nanoparticles had a diameter of about 80–90 nm. The surface analysis in terms of topology and roughness was measured by Atomic Force Microscope (AFM). The surface of MTGase nanoflowers petals completely blooming was framed in AFM (Fig. 2d). Further, its properties were examined by XRD (Fig. 2e) and TGA (Fig. 2f), and the results showed that they exhibited a higher catalytic activity vis-à-vis enhanced operational stability in the resolution reaction as compared to the free MTGase enzyme.52,53 The reusability studies demonstrated that their activity was retained atleast till 10th cycle of reuse. The zeta potential of MTGase NFs was −4.09 mV. The MTGase nanoflowers have a high surface area and 3-D structure for possible confinement, which is not exhibited in transglutaminase native form.51 In terms of morphology, MTGase nanoflowers were found to be robust and highly durable to resist even harsh environmental conditions-high temperatures, solvents, pH fluctuations.53–55,77,80,87,88 The TGA analysis of MTGase revealed that it can endure high temperatures up to 280 °C, whereas the control set was degraded instantly at reaching 40 °C as a sharp dip in the peak was observed. This proves that MTGase NFs are so stable and robust in physiological conditions as compared to the control set, which can't bear any harsh environment.52,53,55,80 The functional groups of MTGase NFs were imparting their stability. They retained their structural integrity in different organic solvents tested like methanol, ethanol, propanol, DMSO.87 Hence, these MTGase nanoflowers obtained were intact and highly resistant due to the iso-peptide bond of MTGase that sustained the degradation process (ESI, Fig. S2†).43,89 The enzyme MTGase was solely responsible for nanoflowers structure. The enzyme itself is getting folded to a floral appearance on account of its structure. Thus, the floral shape remained preserved even in extreme conditions, which is not true for any other nanoflowers being claimed earlier.
The study represented that MTGase nanoflowers had a higher encapsulating yield (84.39%) and enzyme efficiency (6.92-fold increment) than any other enzyme-based nanoflowers to date (United Kingdom Patent No. 2111966.4; U.S. Patent No. 17/406,928; Indian Patent No. 202011035812) (ESI, Table S1†). Further, these MTGase nanoflowers were assessed for their anti-cancerous properties on breast cancer cells.
3.3. Characterization of MTGase NFs for suitability as the drug potential
The encapsulation of MTGase enzyme in its nanoflower was envisaged to be therapeutically superior to free drug combination in vivo, with prolonged systemic circulation, enhanced tumor accumulation, and maintenance of synergistic drug ratios.54,56,80,87 Enzymatic nanoflowers would be one of the most anticipated and promising drug delivery agents, especially from the viewpoint of drug economic value on account of the simplest design and synthesis.54,77,78,80,90 Being formulated by utilizing a biological source (MTGase) can greatly reduce the risks (side effects) which are particularly associated with commercial pharmaceutical adjuvants.44,91,92
Transglutaminase is an important regulatory enzyme acting as a site-specific cross-linker for proteins/peptides.44,92–95 The cellular physiology gets perturbed if the dysregulation of the functionality of transglutaminase occurs.43,95–97 With the versatility of MTGase facilitating the nanoflowers formation by acting as molecular glue, it was demonstrated that they have anti-cancer properties. The unique rational drug design based on an incentive immobilized enzyme approach led to the uniform shape of petals in these nanoflowers, which had the capacity to act as an anti-cancerous drug.97,98
We explored, for the first time, the potential encapsulated MTGase in its NFs form as an anti-cancer drug. The study implemented MTGase nanoflowers on breast cancer cells to validate their function of anti-cancerous properties. MTGase NFs possess the anti-cancer characteristic portrayed by any enzymatic nanoflowers synthesized, which are biocompatible, possessing anti-cancer properties towards breast cancer and are non-cytotoxic to normal cells.
3.4. Cytotoxicity assessment of MTGase NFs on cancer cell lines
The in vitro anti-cancer activity of MTGase NFs was evaluated by MTT assay on various cancer cell lines.59 Untreated cells i.e., without MTGase NFs served as a positive control for the experiment. Out of three cell lines tested viz. HaCaT, HCT-116 and MCF-7, the breast cancer cell line MCF-7 showed a significant effect in a dose-dependent manner by retarding the cellular viability after 24 h of treatment. The inhibitory effect (IC50) value of MTGase NFs in case of breast cancer cell lines was found to be 8.23 μg ml−1 (Fig. 3).
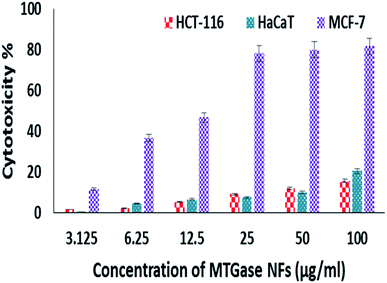 |
| Fig. 3 Cytotoxicity induced by microbial transglutaminase nanoflowers. Breast cancer (MCF-7), skin (HaCaT), and colorectal (HCT-116) cells cell line was treated with indicated MTGase NFs concentrations, and percent cytotoxicity was assessed at 24 h post-treatment. Results are expressed as mean ± S.D of three replicates. | |
In the case of skin and colorectal cancer cell lines, the effect was not prominent like breast cancer cell line; they only showed effect at higher doses in comparison to MCF-7 cell line. Moreover, the results also indicated that MTGase NFs did not significantly induce cytotoxicity at lower doses in HaCaT and HCT-116 cancer cells (IC50 33.07 and 28.73 respectively). Additionally, we have also assessed the effect of the free form of MTGase enzyme (ESI, Fig. S1†). MTGase concentrations were calculated based on the amount of enzyme formulated in the nanoflowers such that the delivered dose was equivalent to the free enzyme MTGase dose.
MTGase NFs were found to be potent anti-cancerous nano-formulation against the aforementioned cancer cell lines. The results highlighted the degree of cytotoxicity of MTGase NFs exhibited towards the breast cell line in question. MTGase NFs displayed a dose-dependent anti-proliferative effect on these cancer cells.56,60
Assessment of cytotoxic effects of MTGase NFs was investigated on control cells (non-cancerous HEK-293 cells). The treatment of normal cells with MTGase NFs (indigenously produced and functionalized compound in this study) did not lead to any adverse effect on the physiology of cells IC50-43.75 μg ml−1 (Fig. 4). This attribute makes way to be a promising cytotoxic compound against cancer cell lines.56,98 Thus, MCF-7 was found to be mainly inhibited by the MTGase NFs.
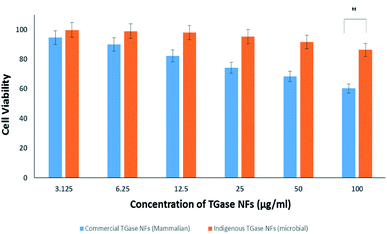 |
| Fig. 4 Cytotoxic effect of transglutaminase nanoflowers on control cells. **p-value < 0.01. | |
It was found that MTGase NFs profoundly sensitized breast cancer cells MCF-7 for cytotoxicity. After evaluating the anti-cancerous property of these MTGase NFs on the breast cell line, we proceeded with these MCF-7 cells. We realized it as a highly enthusiastic result with high efficacy at a lower dose and negligible toxicity on normal cells when compared with the previous reports.97
The commercially available mammalian TGase NFs were also tested to figure out the potency in cancer cells (Fig. 5). It was found that cell viability was higher even after 24 h compared with MTGase NFs treated cells. This points towards the mammalian transglutaminase NFs playing a role in cancer progression.99,100
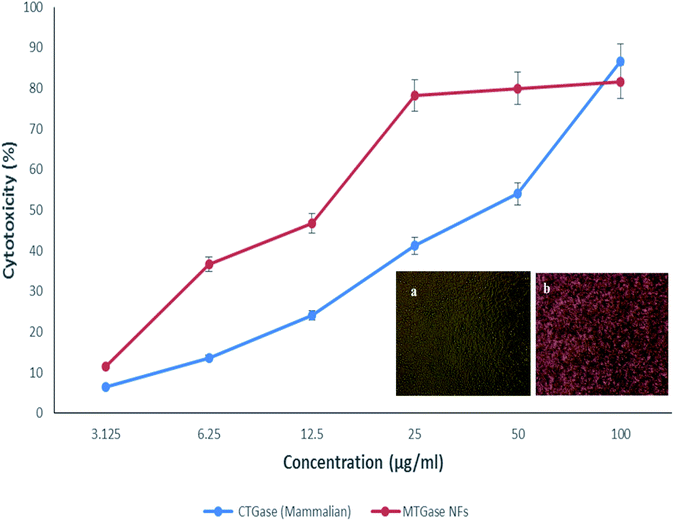 |
| Fig. 5 Cell viability and IC50. MTT assay showing the viability of MCF-7 cells after exposure to increasing concentrations of transglutaminase nanoflowers, CTGase-commercial mammalian source of transglutaminase nanoflowers; MTGase-indigenous microbial transglutaminase nanoflowers (inset-microscopic images after treatment for MTT). | |
After the assessment, we could define the mode of action of the two drugs tested (mammalian vs. microbial TGase), both in nanoflowers. The effect of microbial MTGase NFs was pronounced as cytotoxic to cancerous cells; hence it is efficient in killing cancerous cells in lower concentrations as observed by its IC50. With a higher dose of mammalian TGase NFs, a killing of 50% was achieved; hence its IC50 is also higher (∼4-fold) as compared to MTGase NFs. The results show a clear change in morphology of MCF-7 on the administration of MTGase NFs. Thus, this study established that MTGase NFs are anti-cancerous based on the proliferative assay.101 This suggests MTGase NFs are potent in inhibiting the proliferation of breast cancer cells.
3.5. Clonogenic assay of microbial transglutaminase nanoflowers
To further substantiate the anti-proliferation effect of MTGase NFs, the clonogenic potential of MCF-7 was determined for two weeks after treatment with MTGase NFs.102
The positive control was represented by untreated cells; without MTGase NFs. This study was performed to investigate the influence of MTGase NFs on the clonogenic forming ability of breast cancer cells (MCF-7). In vivo growth and multiplicity of cancer cells are reflected by their colony-forming ability.61,103 The ability of cancerous cells to produce progeny (single cell to form a colony of 50 or more cells) and the long-term effect of MTGase NFs were assessed.
So, in order to assess MCF-7 cell viability in terms of reproductive capacity after treatments with the MTGase NFs formulation, a colony formation assay was performed.67,104
As observed in Fig. 6(a and b), in contrast to untreated cells at all tested concentrations, there was a significant inhibition of clonogenicity with MTGase NFs. The results showed that MTGase NFs treatment significantly inhibited the colony-forming ability of MCF-7 cells. Interestingly, the result was effective only after 8 h treatment. In the case of the control group, more than 300 colonies were formed (314); whereas in MTGase NFs treated set, colony formation was much reduced, leading to the formation of only 30 colonies.
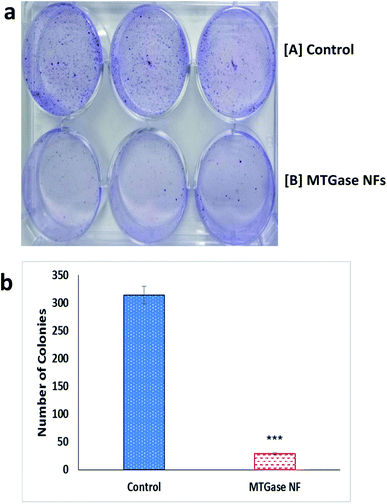 |
| Fig. 6 Effect of MTGase NFs on colony formation (a) culture dishes with stained colonies of a representative experiment; (b) statistical results of colony-forming assays presented as colonies number in respect of untreated cells used as control. The data in the graphs are expressed as mean number ± SD of three different experiments. ***p-value < 0.001. | |
The plating efficiency was found to be 6% in case of the treated group of cells with MTGase NFs over 62.8% found in the untreated group (control, without MTGase NFs), inferring the anti-cancerous MTGase NFs nature. The results clearly validated the role of MTGase NFs possessing cytotoxic nature in mitigating breast cancer. The cells were sensitive to growth inhibition upon a treatment; thus, MTGase NFs enzymatic formulations inhibited clonogenic growth and resulted in only a small fraction of colony survival.68,81
The significant result observed in colony inhibition of MCF-7 cell line further depicted that MTGase NFs have a very defined anti-tumor role in the case of breast cancer cell line MCF-7. MTGase NFs alone inhibited breast cancer MCF-7 cell lines as depicted by a reduction in proliferation and the number of colonies formed using proliferation and clonogenicity assays.
3.6. Inhibition of breast cancer invasion by wound healing/closure assay
Wound healing assay or migration assay presents a great correlation with cell proliferation and migration.1 To study the migratory and invasive nature of cancer cells and the effect of MTGase NFs, we conducted the cell migration assay on MCF-7 cells.66 Cells were treated with MTGase NFs (8.23 μg ml−1) and allowed to migrate across the well plates for 24 h as shown in Fig. 7(a and b). No obvious inhibition was seen in control (untreated cells), whereas MTGase NFs showed significant migratory inhibition at the tested concentration, with 3.76-fold inhibition. The cell line with microbial enzyme transglutaminase formulations NFs showed a significant reduction in their ability to fill the area between scratched edges. Control cells covered almost complete area between the scratched edges, whereas treatment with MTGase NFs has a more profound effect on inhibition of migratory potential of MCF-7 cells compared to control. The migration was found to be suppressed as compared with the control group observed in a time-dependent manner post-treatment of MTGase NFs.
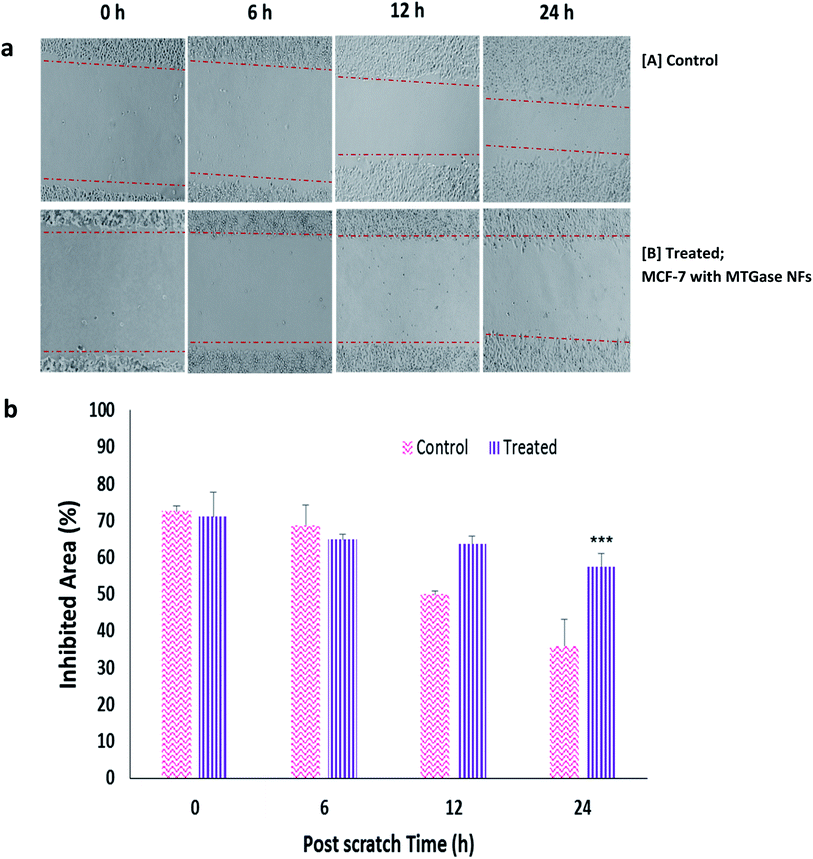 |
| Fig. 7 Effect of MTGase NFs on MFC-7 cells invasion and migration properties. (a) MCF-7 breast cancer cells were seeded in 12-well plates. (b) Cell migration was assessed at time intervals of 0, 6, 12, 24 and 48 h post wound creation. Wound width was calculated at indicated times. Results are expressed as mean ± S.D of three replicates. ***p-value < 0.001. | |
The migration of cells was seen more significantly after 6 h post-treatment. After 24 h, the wound was almost healed in case of the controlled group; in comparison to the MTGase NFs treated group, the wound was not able to heal, which indicated that MTGase NFs is able to restrict the migration of cancer cells. MTGase NFs had drastic inhibitory effects on invasive breast cancer cells with 19.06% inhibition of cells in contrast to 50.61% inhibition observed with an untreated batch of cells (control).
These results suggest that MTGase NFs not only deliver inherent enhanced therapeutic benefits but also inhibit two other important tumor marker events, cell migration and invasion, or possibly limit metastasis-related death for clinical applications.105–107 MTGase NFs developed seems to be a potential anti-cancer drug, MTGase enzyme encapsulated in the form of nanoflower granting easy access into the metastatic cells and thereby showcasing their anti-cancer action.108 The immediate action was pronounced on the fact that CD44 receptors are less on the cancer stem cells. At the same time, CD44+ cell population eradication becomes necessary as they are mainly responsible for tumor recurrence and disease progression, which was carried out by MTGase NFs.109,110
3.7. DNA cell cycle analysis: MTGase NFs stimulated G0/G1 phase arrest of cell cycle
The aim was to determine the therapeutic effects of MTGase NFs by evaluating their DNA content. We used MTGase NFs with their IC50 values concentrations in breast cancer cells and compared treatment results based upon the percentage of cells present in different stages of the cell cycle in comparison to the control.69 We found that with MTGase NFs treatment, the G0/G1 percentage of cells increased from 17.1% in control to 78.8%; whereas, in the S phase, it decreased from 31.1% in control to 4.5%. Furthermore, there was a decrement in G2/M phase cells by MTGase NFs treatment from 51.8% in control to 16.6%. The results showed that MTGase NFs treatment after 24 h is effective in restricting MCF-7 breast cancer cells to undergo mitosis.1
A noteworthy arrest in cell cycle phases can be observed (Fig. 8). When compared to control, MTGase NFs showed a 4.61-fold increase in G0/G1 phase arrest. Moreover, a decrease in cell accumulation in S and G2/M phases was noticed upon treatment with MTGase NFs. In comparison with control, a drastic reduction in the cell was observed in S from 31.1% to 4.5% and G2/M (51.8% to 16.6%), respectively. A small percentage of cells were seen in the S and G2/M phase of the cell cycle phase in MTGase NFs treatment group. However, the endpoint effects i.e., both in G1 phase (preferably) and G2/M arrest, has led to the increased cytotoxicity.70,71
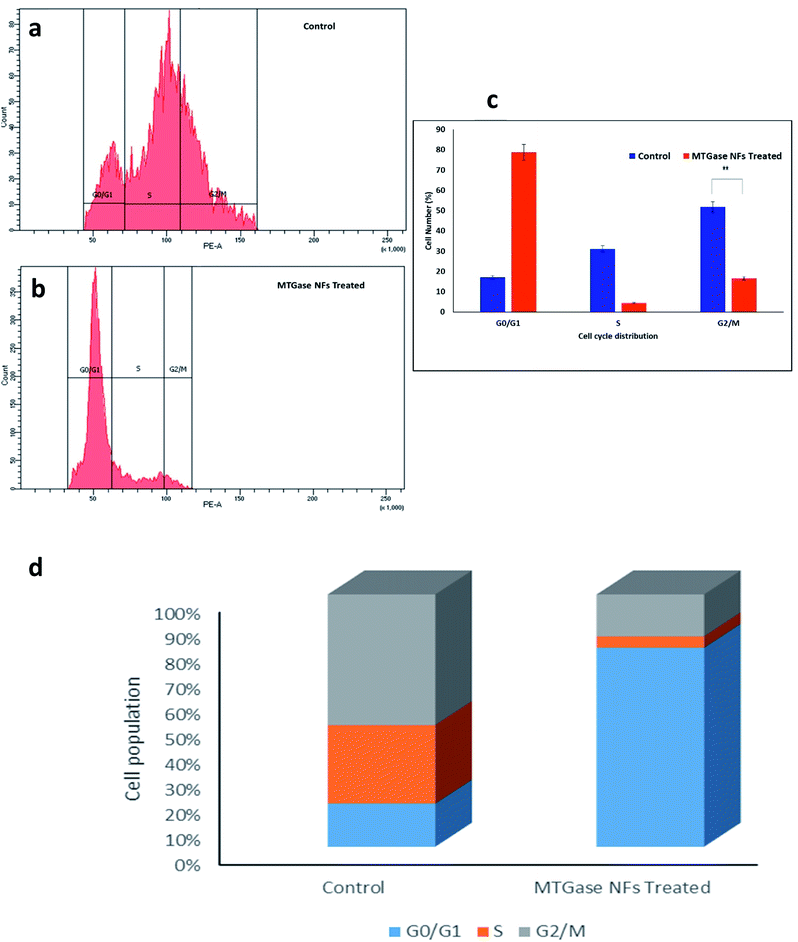 |
| Fig. 8 Effect of MTGase NFs against cell cycle progression (a–c): breast cancer MCF-7 cells were treated with IC50 microbial transglutaminase nanoflowers concentration for 24 h, and the effect on cell cycle progression was assessed through FACS using propidium iodide. (d) Cell progression was restricted in G1/G0 stage of the cell cycle by MTGase NFs treatment. **p-value < 0.01. | |
It was inferred that in breast cancer MCF-7 cells, MTGase NFs were highly effective in arresting the cell growth.111 The significant gain in G0/G1 phase was subsequently dropped in S and G2/M phase. Hence, MTGase NFs suppressed the cells at the initial checkpoints (G0/G1) rather than halting in the later stages of cell division at mitosis phases (G2/M).72
3.8. MTGase NFs induced apoptosis by Annexin V apoptosis assay
After examining the alterations in the cell cycle, we further investigated the influence of MTGase NFs to induce apoptosis in cancer cell line MCF-7.73 The results depicted in Fig. 9 shows the plots portraying the distribution of quarters categorizing the cells into four as early apoptotic cells [Annexin V (+) and PI (−)], late apoptotic or dead cells [Annexin V (+) and PI (+)], dead cells [Annexin V (−) and PI (+)], and live cells [Annexin V (−) and PI (−)].74,75
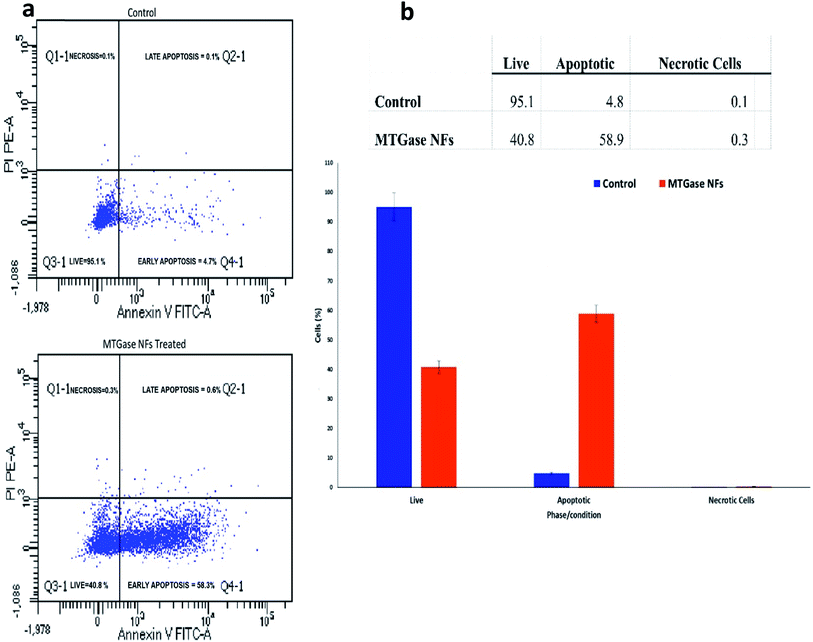 |
| Fig. 9 Detection of apoptosis of MCF-7 cells after treatment with MTGase NFs: breast cancer MCF-7 cells were treated with IC50 transglutaminase nanoflowers concentration for 24 h. Annexin V/PI staining combined with flow cytometry analysis was used to count apoptotic cells. (a) Dot plots showing apoptosis of MCF-7 cells in response to exposure to MTGase NFs. (b) Percentage of cell population of live apoptotic and necrotic was compared. Graphs represent means ± SD of data from three independent biological replicates. | |
To understand whether cell viability impairment was due to apoptosis, we analyzed the cell cycle perturbation and the downstream signalling triggered by MTGase NFs. MCF-7 cells were treated with MTGase NFs for 24 h, and untreated cells were used as control. Apoptosis was investigated by flow cytometry analysis with Annexin V FITC/PI.75 The results clearly indicate that MTGase NFs determine a growing apoptotic induction during the time, reaching about 58.9% at 24 h (Fig. 9). On the contrary, in the untreated cells, we did not detect any apoptotic increase over time.
After treatment with MTGase NFs, the percentage of live cells decreased from 95.1% to 40.8%, while there was an increment in the population of apoptotic cells from 4.8% to 58.9%. Thus, MCF-7 cells showcased high sensitivity on account of treatment with MTGase NFs. From these results, we concluded that apoptosis was induced by microbial transglutaminase nanoflowers after 24 h treatment on MCF-7 cells. MTGase NFs have induced apoptosis by arresting cells in G0/G1 phase in MCF-7 cells. Usually, the cells in the sub G1 phase represent apoptotic cells bearing damaged DNA.4 It indicates the onset of cell death which is beneficial in killing the oncogenic cells.
Based on the key findings of the present study, it could be demonstrated clinical success against breast cancer cells on further investigation and would be the first MTGase based nano-therapy to improve the survival of patients with BCs.98 MTGase NFs would be suitable novelized drug anti-cancer activity against BCs.
MTGase mediated nanoflowers worked well in giving targeted activity based on good anti-cancerous efficacy, specifically on breast cancer cells. They showed controlled and sustained action on cancer cell lines. MTGase NFs as a nano-drug evinced to have surpassed the efficacy of the conventional carrier-based drug delivery systems reported in the literature.97 Our comparison of the effect of MTGase NFs on MCF-7 cells clearly showed promising results for anti-cancer properties using lesser dosages and experiment times over standard drugs as reported in the previous studies.76,97,112–115 MTGase NFs demonstrated the significantly improved efficacy of targeted therapy as an anti-breast cancer drug. Hence, MTGase NFs exhibiting high anti-cancer efficacy is the promising and potential anti-cancer drug for breast cancer.
4. Conclusions
The most common cancer with high mortality among women worldwide is breast cancer.1,3,6,110 The important aspect of any anti-cancer therapy is to destroy cancerous cells without destroying the normal cells. However, precise and relatively novel enzymatic MTGase nano-formulations could lead to a new track for the eradication of BC.5,77 They may also result in a promising approach for early recognition and its treatment. Targeted enzymatic MTGase therapy is likely to work by attacking specific proteins on cancer cells (or nearby cells) that normally help them grow.8 This work presents, for the first time, the results of an experimental study of enzymatic nano-formulations, i.e., MTGase NFs designed as an anti-breast cancer drug for the specific targeting and treatment on MCF-7 cancer cells. Thus, NFs are the new class of nanostructure with unique functional nature imparted by their lattice structure, whose multi-dimensional aspects have recently been explored. The networked and porous matrix interface of NFs has been a subject of interest, owing to the large specific surface area, high adsorption efficiency, catalytic ability, along with high loading capability. The recovery of enzymes in industrial applications, feasibility on account of operational stability is displayed by 3-D NFs. Thus, designing of controlled morphological enzymatic NFs will deliver feasible and improved practical applications and usher nano-supports for immobilizing biomolecules/drugs for drug interaction studies.
The in vitro anti-cancer activity of MTGase NFs was successfully evaluated on breast cancer MCF-7 cells. These novel drug enzymatic based anti-cancer nano-formulations: microbial transglutaminase nanoflowers is shown to efficiently suppress cancer cell growth and significantly induce apoptosis.48,55,56,76,77 Overall, our work identifies in development of a novel anti-cancer agent in the form of enzymatic nano-formulations: microbial transglutaminase nanoflowers, suggesting its therapeutic significance as a nano-drug against BCs. NFs not only impart solubilization of MTGase but also contribute to chemosensitization. The generation of the MTGase NFs was established as a promising candidate as an anti-cancer nanodrug. Enzymatic transglutaminase loaded flower-shaped nanoplatform will be a versatile promise with multi-dimensional functional aspects in cancers.
Data availability
The authors confirm that the data for this study findings are available within the article; ESI.†
Author contributions
Syeda Warisul Fatima: conceptualization, investigation, methodology, visualization, data curation, validation, formal analysis, funding acquisition, writing – original draft, writing – review & editing. Khalid Imtiyaz: investigation, visualization, validation, formal analysis, writing – review & editing. Mohammad M. Alam Rizvi: supervision, resources, writing – review & editing. Sunil K. Khare: supervision, resources, writing – review & editing. All authors have given approval to the final version of the manuscript.
Conflicts of interest
The authors declare no competing financial interest. The patent rights related to the synthesis and designing of transglutaminase nanoflowers are owned by S. W. F., S. K. K.
Acknowledgements
SWF gratefully appreciates the financial assistance provided by the Department of Science and Technology (DST), Government of India (INSPIRE Fellowship [no. IF160621]). The authors also acknowledge the Department of Chemistry, Central Research facility (CRF) of IITD, Central Instrument Facility (CIF), and University Grant Commission Special Assistance Programme Departmental Research Support Phase II (UGC-SAP-DRS-II) Lab of Department of Biosciences, JMI for the infrastructure facilities.
Notes and references
- P. Chowdhury, P. K. Nagesh, E. Hatami, S. Wagh, N. Dan, M. K. Tripathi, S. Khan, B. B. Hafeez, B. Meibohm, S. C. Chauhan and M. Jaggi, Tannic acid-inspired paclitaxel nanoparticles for enhanced anticancer effects in breast cancer cells, J. Colloid Interface Sci., 2019, 535, 133–148 CrossRef CAS PubMed.
- F. Bahreini, E. Rayzan and N. Rezaei, microRNA-related single-nucleotide polymorphisms and breast cancer, J. Cell. Physiol., 2021, 236, 1593–1605 CrossRef CAS PubMed.
- F. Bray, J. Ferlay, I. Soerjomataram, R. L. Siegel, L. A. Torre and A. Jemal, Global cancer statistics 2018: GLOBOCAN estimates of incidence and mortality worldwide for 36 cancers in 185 countries, Ca-Cancer J. Clin., 2018, 68, 394–424 CrossRef PubMed.
- Y. Feng, M. Spezia, S. Huang, C. Yuan, Z. Zeng, L. Zhang, X. Ji, W. Liu, B. Huang, W. Luo and B. Liu, Breast cancer development and progression: risk factors, cancer stem cells, signaling pathways, genomics, and molecular pathogenesis, Genes Dis., 2018, 5, 77–106 CrossRef CAS PubMed.
- U. Hani, M. Rahamathulla, R. A. Osmani, H. Y. Kumar, D. Urolagin, M. Y. Ansari, K. Pandey, K. Devi and S. Yasmin, Recent advances in novel drug delivery systems and approaches for management of breast cancer: a comprehensive review, J. Drug Delivery Sci. Technol., 2020, 56, 101505 CrossRef CAS.
- American Cancer Society, Breast cancer facts & figures 2019–2020, Am. Cancer Soc., 2019, pp. 1–44 Search PubMed.
- K. C. Aalders, K. Tryfonidis, E. Senkus and F. Cardoso, Anti-angiogenic treatment in breast cancer: facts, successes, failures and future perspectives, Cancer Treat. Rev., 2017, 53, 98–110 CrossRef CAS PubMed.
- K. D. Miller, L. Nogueira, A. B. Mariotto, J. H. Rowland, K. R. Yabroff, C. M. Alfano, A. Jemal, J. L. Kramer and R. L. Siegel, Cancer treatment and survivorship statistics, Ca-Cancer J. Clin., 2019, 69, 363–385 CrossRef PubMed.
- V. Jain, H. Kumar, H. V. Anod, P. Chand, N. V. Gupta, S. Dey and S. S. Kesharwani, A review of nanotechnology-based approaches for breast cancer and triple-negative breast cancer, J. Controlled Release, 2020, 326, 628–647 CrossRef CAS PubMed.
- J. D. Obayemi, A. A. Salifu, S. C. Eluu, V. O. Uzonwanne, S. M. Jusu, C. C. Nwazojie, C. E. Onyekanne, O. Ojelabi, L. Payne, C. M. Moore and J. A. King, LHRH-conjugated drugs as targeted therapeutic agents for the specific targeting and localized treatment of triple negative breast cancer, Sci. Rep., 2020, 10, 1–18 CrossRef PubMed.
- R. L. Costa and B. J. Czerniecki, Clinical development of immunotherapies for HER2+ breast cancer: a review of HER2-directed monoclonal antibodies and beyond, npj Breast Cancer, 2020, 6, 1–11 CrossRef.
- J. Cortés, S. A. Im, E. Holgado, J. M. Perez-Garcia, P. Schmid and M. Chavez-MacGregor, The next era of treatment for hormone receptor-positive, HER2-negative advanced breast cancer: triplet combination-based endocrine therapies, Cancer Treat. Rev., 2017, 61, 53–60 CrossRef PubMed.
- G. L. Gierach, R. E. Curtis, R. M. Pfeiffer, M. Mullooly, E. A. Ntowe, R. N. Hoover, S. J. Nyante, H. S. Feigelson, A. G. Glass and A. B. de Gonzalez, Association of adjuvant tamoxifen and aromatase inhibitor therapy with contralateral breast cancer risk among US women with breast cancer in a general community setting, JAMA Oncol., 2017, 3, 186–193 CrossRef PubMed.
- I. Kramer, M. Schaapveld, H. S. Oldenburg, G. S. Sonke, D. McCool, F.
E. van Leeuwen, K. K. Van de Vijver, N. S. Russell, S. C. Linn, S. Siesling and M. K. Schmidt, The influence of adjuvant systemic regimens on contralateral breast cancer risk and receptor subtype, J. Natl. Cancer Inst., 2019, 111, 709–718 CrossRef PubMed.
- J. Wang and B. Xu, Targeted therapeutic options and future perspectives for HER2-positive breast cancer, Signal Transduction Targeted Ther., 2019, 4, 1–22 CrossRef PubMed.
- G. Von Minckwitz, C. S. Huang, M. S. Mano, S. Loibl, E. P. Mamounas, M. Untch, N. Wolmark, P. Rastogi, A. Schneeweiss, A. Redondo and H. H. Fischer, Trastuzumab emtansine for residual invasive HER2-positive breast cancer, N. Engl. J. Med., 2019, 380, 617–628 CrossRef CAS.
- D. F. Hayes, HER2 and breast cancer—a phenomenal success story, N. Engl. J. Med., 2019, 381, 1284–1286 CrossRef PubMed.
- Y. H. Xie, Y. X. Chen and J. Y. Fang, Comprehensive review of targeted therapy for colorectal cancer, Signal Transduction Targeted Ther., 2020, 5, 1–30 CrossRef PubMed.
- V. Yang, M. J. Gouveia, J. Santos, B. Koksch, I. Amorim, F. Gärtner and N. Vale, Breast cancer: insights in disease and influence of drug methotrexate, RSC Med. Chem., 2020, 11, 646–664 RSC.
- X. Ji, Y. Lu, H. Tian, X. Meng, M. Wei and W. C. Cho, Chemoresistance mechanisms of breast cancer and their countermeasures, Biomed. Pharmacother., 2019, 114, 108800 CrossRef CAS PubMed.
- N. A. Seebacher, A. E. Stacy, G. M. Porter and A. M. Merlot, Clinical development of targeted and immune based anti-cancer therapies, J. Exp. Clin. Cancer Res., 2019, 38, 1–39 CrossRef PubMed.
- A. Godoy-Ortiz, A. Sanchez-Muñoz, M. R. Chica Parrado, M. Álvarez, N. Ribelles, A. Rueda Dominguez and E. Alba, Deciphering HER2 breast cancer disease: biological and clinical implications, Front. Oncol., 2019, 9, 1124 CrossRef PubMed.
- A. I. Riggio, K. E. Varley and A. L. Welm, The lingering mysteries of metastatic recurrence in breast cancer, Br. J. Cancer, 2020, 124, 1–14 Search PubMed.
- N. Harbeck, F. Penault-Llorca, J. Cortes, M. Gnant, N. Houssami, P. Poortmans, K. Ruddy, J. Tsang and F. Cardoso, Breast cancer, Nat. Rev. Dis. Primers, 2019, 5, 66 CrossRef PubMed.
- A. M. Gonzalez-Angulo, F. Morales-Vasquez and G. N. Hortobagyi, Overview of resistance to systemic therapy in patients with breast cancer. Overview of resistance to systemic therapy in patients with breast cancer, Adv. Exp. Med. Biol., 2007, 608, 1–22 CrossRef CAS PubMed.
- Y. Gao, M. Tang, E. Leung, D. Svirskis, A. Shelling and Z. Wu, Dual or multiple drug loaded nanoparticles to target breast cancer stem cells, RSC Adv., 2020, 10, 19089–19105 RSC.
- H. J. Burstein, G. Curigliano, S. Loibl, P. Dubsky, M. Gnant, P. Poortmans, M. Colleoni, C. Denkert, M. Piccart-Gebhart, M. Regan, H.-J. Senn, E. P. Winer and B. Thurlimann, Estimating the benefits of therapy for early-stage breast cancer: the St. Gallen International Consensus Guidelines for the primary therapy of early breast cancer 2019, Ann. Oncol., 2019, 30, 1541–1557 CrossRef CAS PubMed.
- S. K. Singh, S. Singh, J. W. Lillard Jr and R. Singh, Drug delivery approaches for breast cancer, Int. J. Nanomed., 2017, 12, 6205 CrossRef CAS PubMed.
- Y. J. Cheng, G. F. Luo, J. Y. Zhu, X. D. Xu, X. Zeng, D. B. Cheng, Y. M. Li, Y. Wu, X. Z. Zhang, R. X. Zhuo and F. He, Enzyme-induced and tumor-targeted drug delivery system based on multifunctional mesoporous silica nanoparticles, ACS Appl. Mater. Interfaces, 2015, 7, 9078–9087 CrossRef CAS PubMed.
- C. Luo, Y. Wang, Q. Chen, X. Han, X. Liu, J. Sun and Z. He, Advances of paclitaxel formulations based on nanosystem delivery technology, Mini-Rev. Med. Chem., 2012, 12, 434–444 CrossRef CAS PubMed.
- F. Wang, M. Porter, A. Konstantopoulos, P. Zhang and H. Cui, Preclinical development of drug delivery systems for paclitaxel-based cancer chemotherapy, J. Controlled Release, 2017, 267, 100–118 CrossRef CAS PubMed.
- V. Buocikova, I. Rios-Mondragon, E. Pilalis, A. Chatziioannou, S. Miklikova, M. Mego, K. Pajuste, M. Rucins, N. E. Yamani, E. M. Longhin and A. Sobolev, Epigenetics in Breast Cancer Therapy—New Strategies and Future Nanomedicine Perspectives, Cancers, 2020, 12, 3622 CrossRef CAS PubMed.
- V. T. Chivere, P. P. Kondiah, Y. E. Choonara and V. Pillay, Nanotechnology-based biopolymeric oral delivery platforms for advanced cancer treatment, Cancers, 2020, 12, 522 CrossRef CAS PubMed.
- S. Senapati, A. K. Mahanta, S. Kumar and P. Maiti, Controlled drug delivery vehicles for cancer treatment and their performance, Signal Transduction Targeted Ther., 2018, 3, 1–19 CrossRef CAS PubMed.
- C. Bailleux, L. Eberst and T. Bachelot, Treatment strategies for breast cancer brain metastases, Br. J. Cancer, 2021, 124, 142–155 CrossRef PubMed.
- L. Yang, P. Shi, G. Zhao, J. Xu, W. Peng, J. Zhang, G. Zhang, X. Wang, Z. Dong, F. Chen and H. Cui, Targeting cancer stem cell pathways for cancer therapy, Signal Transduction Targeted Ther., 2020, 5, 1–35 CrossRef PubMed.
- M. Chakravarty and A. Vora, Nanotechnology-based antiviral therapeutics, Drug Delivery Transl. Res., 2020, 3, 1–40 Search PubMed.
- X. Fu, Y. Shi, T. Qi, S. Qiu, Y. Huang, X. Zhao, Q. Sun and G. Lin, Precise design strategies of nanomedicine for improving cancer therapeutic efficacy using subcellular targeting, Signal Transduction Targeted Ther., 2020, 5, 1–15 CrossRef PubMed.
- R. van der Meel, E. Sulheim, Y. Shi, F. Kiessling, W. J. Mulder and T. Lammers, Smart cancer nanomedicine, Nat. Nanotechnol., 2019, 14, 1007–1017 CrossRef CAS PubMed.
- A. S. Choudhari, P. C. Mandave, M. Deshpande, P. Ranjekar and O. Prakash, Phytochemicals in cancer treatment: from preclinical studies to clinical practice, Front. Pharmacol., 2020, 10, 1614 CrossRef PubMed.
- V. Schirrmacher, From chemotherapy to biological therapy: a review of novel concepts to reduce the side effects of systemic cancer treatment, Int. J. Oncol., 2019, 54, 407–419 CAS.
- L. Duarte, C. R. Matte, C. V. Bizarro and M. A. Z. Ayub, Review transglutaminases: part II—industrial applications in food, biotechnology, textiles and leather products, World J. Microbiol. Biotechnol., 2020, 36, 1–20 CrossRef PubMed.
- S. W. Fatima and S. K. Khare, Current insight and futuristic vistas of microbial transglutaminase in nutraceutical industry, Microbiol. Res., 2018, 215, 7–14 CrossRef CAS PubMed.
- S. W. Fatima, S. Barua, M. Sardar and S. K. Khare, Immobilization of Transglutaminase on multi-walled carbon nanotubes and its application as bioinspired hydrogel scaffolds, Int. J. Biol. Macromol., 2020, 163, 1747–1758 CrossRef CAS PubMed.
- J. Zhu, H. Deng, A. Yang, Z. Wu, X. Li, P. Tong and H. Chen, Effect of microbial transglutaminase cross-linking on the quality characteristics and potential allergenicity
of tofu, Food Funct., 2019, 10, 5485–5497 RSC.
- J. Huang, Z. Huang, Y. Liang, W. Yuan, L. Bian, L. Duan, Z. Rong, J. Xiong, D. Wang and J. Xia, 3D Printed Gelatin/Hydroxyapatite Scaffolds for Stem Cell Chondrogenic Differentiation and Articular Cartilage Repair, Biomater. Sci., 2021, 9, 2620–2630 RSC.
- J. Ge, J. Lei and R. N. Zare, Protein–inorganic hybrid nanoflowers, Nat. Nanotechnol., 2012, 7, 428–432 CrossRef CAS PubMed.
- Y. Liu, X. Ji and Z. He, Organic–inorganic nanoflowers: from design strategy to biomedical applications, Nanoscale, 2019, 11, 17179–17194 RSC.
- J. K. Patra, G. Das, L. F. Fraceto, E. V. R. Campos, M. del Pilar Rodriguez-Torres, L. S. Acosta-Torres, L. A. Diaz-Torres, R. Grillo, M. K. Swamy, S. Sharma and S. Habtemariam, Nano based drug delivery systems: recent developments and future prospects, J. Nanobiotechnol., 2018, 16, 1–33 CrossRef PubMed.
- T. Huang, Y. Wang, Y. Shen, H. Ao, Y. Guo, M. Han and X. Wang, Preparation of high drug-loading celastrol nanosuspensions and their anti-breast cancer activities in vitro and in vivo, Sci. Rep., 2020, 10, 1–9 CrossRef PubMed.
- H. Kaur, N. K. Bari, A. Garg and S. Sinha, Protein morphology drives the structure and catalytic activity of bio-inorganic hybrids, Int. J. Biol. Macromol., 2021, 176, 106–116 CrossRef CAS PubMed.
- Y. Yin, Y. Xiao, G. Lin, Q. Xiao, Z. Lin and Z. Cai, An enzyme–inorganic hybrid nanoflower based immobilized enzyme reactor with enhanced enzymatic activity, J. Mater. Chem. B, 2015, 3, 2295–2300 RSC.
- J. Cui, Y. Zhao, R. Liu, C. Zhong and S. Jia, Surfactant-activated lipase hybrid nanoflowers with enhanced enzymatic performance, Sci. Rep., 2016, 6, 1–13 CrossRef PubMed.
- J. Cui and S. Jia, Organic–inorganic hybrid nanoflowers: a novel host platform for immobilizing biomolecules, Coord. Chem. Rev., 2017, 352, 249–263 CrossRef CAS.
- S. Dube and D. Rawtani, Understanding intricacies of bioinspired organic-inorganic hybrid nanoflowers: a quest to achieve enhanced biomolecules immobilization for biocatalytic, biosensing and bioremediation applications, Adv. Colloid Interface Sci., 2021, 295, 102484 CrossRef CAS PubMed.
- X. Jing, Y. Xu, D. Liu, Y. Wu, N. Zhou, D. Wang, K. Yan and L. Meng, Intelligent nanoflowers: a full tumor microenvironment-responsive multimodal cancer theranostic nanoplatform, Nanoscale, 2019, 11, 15508–15518 RSC.
- W. Ren, Y. Li, J. Wang, L. Li, L. Xu, Y. Wu, Y. Wang, X. Fei and J. Tian, Synthesis of magnetic nanoflower immobilized lipase and its continuous catalytic application, New J. Chem., 2019, 43, 11082–11090 RSC.
- U. Wais, A. W. Jackson, T. He and H. Zhang, Nanoformulation and encapsulation approaches for poorly water-soluble drug nanoparticles, Nanoscale, 2016, 8, 1746–1769 RSC.
- M. A. Khan, M. Zafaryab, S. H. Mehdi, J. Quadri and M. M. A. Rizvi, Characterization and carboplatin loaded chitosan nanoparticles for the chemotherapy against breast cancer in vitro studies, Int. J. Biol. Macromol., 2017, 97, 115–122 CrossRef CAS PubMed.
- S. J. Mehdi, A. Ahmad, M. Irshad, N. Manzoor and M. M. A. Rizvi, Cytotoxic effect of carvacrol on human cervical cancer cells, Biol. Med., 2011, 3, 307–312 CAS.
- S. J. Gilani, M. Bin-Jumah, M. Rizwanullah, S. S. Imam, K. Imtiyaz, S. Alshehri and M. M. A. Rizvi, Chitosan Coated Luteolin Nanostructured Lipid Carriers: Optimization, In Vitro-Ex Vivo Assessments and Cytotoxicity Study in Breast Cancer Cells, Coatings, 2021, 11, 158 CrossRef CAS.
- N. A. Franken, H. M. Rodermond, J. Stap, J. Haveman and C. Van Bree, Clonogenic assay of cells in vitro, Nat. Protoc., 2006, 1, 2315–2319 CrossRef CAS PubMed.
- R. Preet, P. Mohapatra, S. Mohanty, S. K. Sahu, T. Choudhuri, M. D. Wyatt and C. N. Kundu, Quinacrine has anticancer activity in breast cancer cells through inhibition of topoisomerase activity, Int. J. Cancer, 2012, 130, 1660–1670 CrossRef CAS PubMed.
- K. Buch, T. Peters, T. Nawroth, M. Sänger, H. Schmidberger and P. Langguth, Determination of cell survival after irradiation via clonogenic assay versus multiple MTT Assay-A comparative study, Radiat. Oncol., 2012, 7, 1–6 CrossRef PubMed.
- F. Shamsi, P. Hasan, A. Queen, A. Hussain, P. Khan, B. Zeya, H. M. King, S. Rana, J. Garrison, M. F. Alajmi and M. M. A. Rizvi, Synthesis and SAR studies of novel 1, 2, 4-oxadiazole-sulfonamide based compounds as potential anticancer agents for colorectal cancer therapy, Bioorg. Chem., 2020, 98, 103754 CrossRef CAS PubMed.
- S. Mahira, N. Kommineni, G. M. Husain and W. Khan, Cabazitaxel and silibinin co-encapsulated cationic liposomes for CD44 targeted delivery: a new insight into nanomedicine based combinational chemotherapy for prostate cancer, Biomed. Pharmacother., 2019, 110, 803–817 CrossRef CAS PubMed.
- A. Kabała-Dzik, A. Rzepecka-Stojko, R. Kubina, Z. Jastrzębska-Stojko, R. Stojko, R. D. Wojtyczka and J. Stojko, Migration rate inhibition of breast cancer cells treated by caffeic acid and caffeic acid phenethyl ester: an in vitro comparison study, Nutrients, 2017, 9, 1144 CrossRef PubMed.
- H. Kurose, M. A. Shibata, M. Iinuma and Y. Otsuki, Alterations in cell cycle and induction of apoptotic cell death in breast cancer cells treated with α-mangostin extracted from mangosteen pericarp, J. Biomed. Biotechnol., 2012, 2012, 672428 Search PubMed.
- E. H. Dakir, A. Pickard, K. Srivastava, C. M. McCrudden, S. R. Gross, S. Lloyd, S. D. Zhang, A. Margariti, R. Morgan, P. S. Rudland and M. El-Tanani, The anti-psychotic drug pimozide is a novel chemotherapeutic for breast cancer, Oncotarget, 2018, 9, 34889 CrossRef PubMed.
- S. Şimşek, A. A. Şüküroğlu, D. Yetkin, B. Özbek, D. Battal and R. Genç, DNA-damage and cell cycle arrest initiated anti-cancer potency of super tiny carbon dots on MCF7 cell line, Sci. Rep., 2020, 10, 1–14 CrossRef PubMed.
- T. Otto and P. Sicinski, Cell cycle proteins as promising targets in cancer therapy, Nat. Rev. Cancer, 2017, 17, 93 CrossRef CAS PubMed.
- S. Khazaei, N. M. Esa, V. Ramachandran, R. A. Hamid, A. K. Pandurangan, A. Etemad and P. Ismail, In vitro antiproliferative and apoptosis inducing effect of Allium atroviolaceum bulb extract on breast, cervical, and liver cancer cells, Front. Pharmacol., 2017, 8, 5 Search PubMed.
- M. Gulfam, J. E. Kim, J. M. Lee, B. Ku, B. H. Chung and B. G. Chung, Anticancer drug-loaded gliadin nanoparticles induce apoptosis in breast cancer cells, Langmuir, 2012, 28, 8216–8223 CrossRef CAS PubMed.
- A. Arumugam, M. D. Ibrahim, S. B. Kntayya, N. Mohd Ain, R. Iori, S. Galletti, C. Ioannides and A. F. Abdull Razis, Induction of Apoptosis by Gluconasturtiin-Isothiocyanate (GNST-ITC) in Human Hepatocarcinoma HepG2 Cells and Human Breast Adenocarcinoma MCF-7 Cells, Molecules, 2020, 25, 1240 CrossRef CAS PubMed.
- S. S. Esnaashari, S. Muhammadnejad, S. Amanpour and A. Amani, A Combinational Approach Towards Treatment of Breast Cancer: an Analysis of Noscapine-Loaded Polymeric Nanoparticles and Doxorubicin, AAPS PharmSciTech, 2020, 21, 1–12 CrossRef PubMed.
- P. Shende, P. Kasture and R. S. Gaud, Nanoflowers: the future trend of nanotechnology for multi-applications, Artif. Cells, Nanomed., Biotechnol., 2018, 46, 413–422 CrossRef CAS PubMed.
- M. Zhang, Y. Zhang, C. Yang, C. Ma and J. Tang, Enzyme-inorganic hybrid nanoflowers: classification, synthesis, functionalization and potential applications, Chem. Eng. J., 2021, 415, 129075 CrossRef CAS.
- S. W. Lee, S. A. Cheon, M. I. Kim and T. J. Park, Organic–inorganic hybrid nanoflowers: types, characteristics, and future prospects, J. Nanobiotechnol., 2015, 13, 1–10 CrossRef PubMed.
- J. Sun, J. Ge, W. Liu, M. Lan, H. Zhang, P. Wang, Y. Wang and Z. Niu, Multi-enzyme co-embedded organic–inorganic hybrid nanoflowers: synthesis and application as a colorimetric sensor, Nanoscale, 2014, 6, 255–262 RSC.
- K. A. Al-Maqdi, M. Bilal, A. Alzamly, H. Iqbal, I. Shah and S. S. Ashraf, Enzyme-Loaded Flower-Shaped Nanomaterials: A Versatile Platform with Biosensing, Biocatalytic, and Environmental Promise, Nanomaterials, 2021, 11, 1460 CrossRef CAS PubMed.
- Y. L. Lin, N. M. Tsai, C. H. Chen, Y. K. Liu, C. J. Lee, Y. L. Chan, Y. S. Wang, Y. C. Chang, C. H. Lin, T. H. Huang and C. C. Wang, Specific drug delivery efficiently induced human breast tumor regression using a lipoplex by non-covalent association with anti-tumor antibodies, J. Nanobiotechnol., 2019, 17, 1–11 CrossRef PubMed.
- S. K. Chan and T. S. Lim, Bioengineering of microbial transglutaminase for biomedical applications, Appl. Microbiol. Biotechnol., 2019, 103, 2973–2984 CrossRef CAS PubMed.
- M. P. Savoca, E. Tonoli, A. G. Atobatele and E. A. Verderio, Biocatalysis by transglutaminases: a review of biotechnological applications, Micromachines, 2018, 9, 562 CrossRef PubMed.
- M. Akbari, S. H. Razavi and M. Kieliszek, Recent advances in microbial transglutaminase biosynthesis and its application in the food industry, Trends Food Sci. Technol., 2021, 110, 458–469 CrossRef CAS.
- M. Griffin, R. Casadio and C. M. Bergamini, Transglutaminases: nature's biological glues, Biochem. J., 2002, 368, 377–396 CrossRef CAS PubMed.
- S. Ren, C. Li, X. Jiao, S. Jia, Y. Jiang, M. Bilal and J. Cui, Recent progress in multienzymes co-immobilization and multienzyme system applications, Chem. Eng. J., 2019, 373, 1254–1278 CrossRef CAS.
- A. H. Memon, R. Ding, Q. Yuan, H. Liang and Y. Wei, Coordination of GMP ligand with Cu to enhance the multiple enzymes stability and substrate specificity by co-immobilization process, Biochem. Eng. J., 2018, 136, 102–108 CrossRef CAS.
- P. J. Birckbichler and M. K. Patterson Jr, Transglutaminase and epsilon-(gamma-glutamyl) lysine isopeptide bonds in eukaryotic cells, Prog. Clin. Biol. Res., 1980, 41, 845–855 CAS.
- P. N. Navya, A. Kaphle, S. P. Srinivas, S. K. Bhargava, V. M. Rotello and H. K. Daima, Current trends and challenges in cancer management and therapy using designer nanomaterials, Nano Convergence, 2019, 6, 1–30 CrossRef CAS PubMed.
- T. Wu, H. Huang, Y. Sheng, H. Shi, Y. Min and Y. Liu, Transglutaminase mediated PEGylation of nanobodies for targeted nano-drug delivery, J. Mater. Chem. B, 2018, 6, 1011–1017 RSC.
- L. Deweid, O. Avrutina and H. Kolmar, Microbial transglutaminase for biotechnological and biomedical engineering, Biol. Chem., 2019, 400, 257–274 CrossRef CAS PubMed.
- L. Duarte, C. R. Matte, C. V. Bizarro and M. A. Z. Ayub, Transglutaminases: part I—origins, sources, and biotechnological characteristics, World J. Microbiol. Biotechnol., 2020, 36, 1–18 CrossRef PubMed.
- H. Schneider, L. Deweid, O. Avrutina and H. Kolmar, Recent progress in transglutaminase-mediated assembly of antibody-drug conjugates, Anal. Biochem., 2020, 595, 113615 CrossRef CAS PubMed.
- P. Strop, Versatility of microbial transglutaminase, Bioconjugate Chem., 2014, 25, 855–862 CrossRef CAS PubMed.
- R. L. Eckert, M. T. Kaartinen, M. Nurminskaya, A. M. Belkin, G. Colak, G. V. Johnson and K. Mehta, Transglutaminase regulation of cell function, Physiol. Rev., 2014, 94, 383–417 CrossRef CAS PubMed.
- S. Hauser, R. Wodtke, C. Tondera, J. Wodtke, A. T. Neffe, J. Hampe, A. Lendlein, R. Löser and J. Pietzsch, Characterization of Tissue Transglutaminase as a Potential Biomarker for Tissue Response toward Biomaterials, ACS Biomater. Sci. Eng., 2019, 5, 5979–5989 CrossRef CAS PubMed.
- L. Huang, S. Zhao, F. Fang, T. Xu, M. Lan and J. Zhang, Advances and Perspectives in Carrier-free Nanodrugs for Cancer Chemo-Monotherapy and Combination Therapy, Biomaterials, 2021, 268, 120557 CrossRef CAS PubMed.
- N. Agnihotri and K. Mehta, Transglutaminase-2: evolution from pedestrian protein to a promising therapeutic target, Amino Acids, 2017, 49, 425–439 CrossRef CAS PubMed.
- H. D. Halicka, J. Li, H. Zhao and Z. Darzynkiewicz, Concurrent detection of lysosome and tissue transglutaminase activation in relation to cell cycle position during apoptosis induced by different anticancer drugs, Cytometry, Part A, 2019, 95, 683–690 CrossRef CAS PubMed.
- S. Zafar, S. Akhter, I. Ahmad, Z. Hafeez, M. M. A. Rizvi, G. K. Jain and F. J. Ahmad, Improved chemotherapeutic efficacy against resistant human breast cancer cells with co-delivery of Docetaxel and Thymoquinone by chitosan grafted lipid nanocapsules: formulation optimization, in vitro and in vivo studies, Colloids Surf., B, 2020, 186, 110603 CrossRef CAS PubMed.
- M. A. Khan, M. D. Zafaryab, S. H. Mehdi, I. Ahmad and M. M. A. Rizvi, Characterization and anti-proliferative activity of curcumin loaded chitosan nanoparticles in cervical cancer, Int. J. Biol. Macromol., 2016, 93, 242–253 CrossRef CAS PubMed.
- S. Alshehri, S. S. Imam, M. Rizwanullah, K. U. Fakhri, M. M. A. Rizvi, W. Mahdi and M. Kazi, Effect of chitosan coating on PLGA nanoparticles for oral delivery of Thymoquinone: in vitro, ex vivo, and cancer cell line assessments, Coatings, 2021, 11, 6 CrossRef CAS.
- S. Nafees, S. H. Mehdi, M. Zafaryab, B. Zeya, T. Sarwar and M. M. A. Rizvi, Synergistic interaction of rutin and silibinin on human colon cancer cell line, Arch. Med. Res., 2018, 49, 226–234 CrossRef CAS PubMed.
- S. Khan, S. Setua, S. Kumari, N. Dan, A. Massey, B. B. Hafeez, M. M. Yallapu, Z. E. Stiles, A. Alabkaa, J. Yue and A. Ganju, Superparamagnetic iron oxide nanoparticles of curcumin enhance gemcitabine therapeutic response in pancreatic cancer, Biomaterials, 2019, 208, 83–97 CrossRef CAS PubMed.
- Y. Li, Y. Qi, H. Zhang, Z. Xia, T. Xie, W. Li, D. Zhong, H. Zhu and M. Zhou, Gram-scale synthesis of highly biocompatible and intravenous injectable hafnium oxide nanocrystal with enhanced radiotherapy efficacy for cancer theranostic, Biomaterials, 2020, 226, 119538 CrossRef CAS PubMed.
- A. Chhabra, A. Verma and K. Mehta, Tissue transglutaminase promotes or suppresses tumors depending on cell context, Anticancer Res., 2009, 29, 1909–1919 CAS.
- D. Ling, G. M. Marshall, P. Y. Liu, N. Xu, C. A. Nelson, S. E. Iismaa and T. Liu, Enhancing the anticancer
effect of the histone deacetylase inhibitor by activating transglutaminase, Eur. J. Cancer, 2012, 48, 3278–3287 CrossRef CAS PubMed.
- M. L. De Angelis, F. Francescangeli and A. Zeuner, Breast cancer stem cells as drivers of tumor chemoresistance, dormancy and relapse: new challenges and therapeutic opportunities, Cancers, 2019, 11, 1569 CrossRef CAS PubMed.
- E. Muntimadugu, R. Kumar, S. Saladi, T. A. Rafeeqi and W. Khan, CD44 targeted chemotherapy for co-eradication of breast cancer stem cells and cancer cells using polymeric nanoparticles of salinomycin and paclitaxel, Colloids Surf., B, 2016, 143, 532–546 CrossRef CAS PubMed.
- C. Tabolacci, A. De Martino, C. Mischiati, G. Feriotto and S. Beninati, The role of tissue transglutaminase in cancer cell initiation, survival and progression, Med. Sci., 2019, 7, 19 CAS.
- X. Tang, W. S. Loc, C. Dong, G. L. Matters, P. J. Butler, M. Kester, C. Meyers, Y. Jiang and J. H. Adair, The use of nanoparticulates to treat breast cancer, Nanomedicine, 2017, 12, 2367–2388 CrossRef CAS PubMed.
- M. S. Di Wu, H. Y. Xue and H. L. Wong, Nanomedicine applications in the treatment of breast cancer: current state of the art, Int. J. Nanomed., 2017, 12, 5879 CrossRef PubMed.
- X. Fang, J. Cao and A. Shen, Advances in anti-breast cancer drugs and the application of nano-drug delivery systems in breast cancer therapy, J. Drug Delivery Sci. Technol., 2020, 57, 101662 CrossRef CAS.
- J. Shao, Y. Fang, R. Zhao, F. Chen, M. Yang, J. Jiang, Z. Chen, X. Yuan and L. Jia, Evolution from small molecule to nano-drug delivery systems: an emerging approach for cancer therapy of ursolic acid, Asian J. Pharm. Sci., 2020, 15, 685–700 CrossRef PubMed.
- Y. Xiao, J. Liu, M. Guo, H. Zhou, J. Jin, J. Liu, Y. Liu, Z. Zhang and C. Chen, Synergistic combination chemotherapy using carrier-free celastrol and doxorubicin nanocrystals for overcoming drug resistance, Nanoscale, 2018, 10, 12639–12649 RSC.
Footnote |
† Electronic supplementary information (ESI) available. See DOI: 10.1039/d1ra04513j |
|
This journal is © The Royal Society of Chemistry 2021 |
Click here to see how this site uses Cookies. View our privacy policy here.