DOI:
10.1039/D1RA05070B
(Paper)
RSC Adv., 2021,
11, 29934-29938
Activator free diastereoselective 1,3-dipolar cycloaddition: a quick access to coumarin based spiro multi heterocyclic adducts†
Received
30th June 2021
, Accepted 2nd September 2021
First published on 8th September 2021
Abstract
A formal diastereoselective 1,3-dipolar cycloaddition of azomethine ylide and coumarin derivatives to construct coumarin based spiro multi heterocyclics has been described. The in situ generation of azo-ylide was achieved for various heterocyclic carbonyls (indenoquinoxaline and isatin). This transformation is also suitable for maleimide dipolarophiles for the synthesis of hydro-maleimide derivatives. These decarboxylative annulations neither required any catalyst nor any activator. Further the pure products were isolated by filtration from the reaction mixture after the reaction under ambient conditions.
Introduction
Coumarin is a privileged structural motif; the core skeletons are frequently observed in many natural products and active pharmaceutical ingredients (Fig. 1).1–3 These scaffolds, possess an ample range of modulatory and cytoprotective functions, which provides therapeutic potentiality in multiple disorders such as anti-inflammatory, anti-cancer, anti-bacterial, anti-coagulant, anti-tumour, anti-HIV therapy, photo-chemotherapy etc.1,2 Therefore, the synthesis and structural modification of this motif have captured huge attention from the synthetic chemist community. Over the past few decades, several remarkable achievements were reported on the various scaffolds of coumarin and its derivatives.2,3 However, the structural decoration around these scaffolds is still in demand for the construction of multi-functionalized bioactive coumarin adducts.
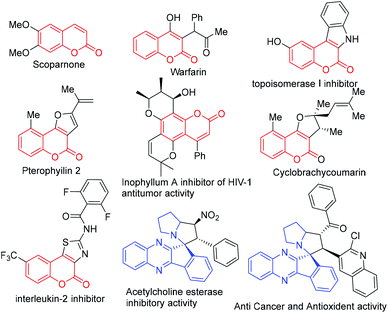 |
| Fig. 1 Some bioactive coumarin and spiro multicyclics. | |
On the other hand, spiro heterocyclics are proven to be potent pharmacologically active scaffolds (Fig. 1), which are found in a huge range of therapeutics and are also widely available in several natural products.4–6 These spiro cyclic derivatives are usually fabricated through multicomponent reactions (MCRs). Owing to their bond forming efficiency, MCRs are facile approaches to create diversified complex molecular frameworks from readily available feedstocks in a high atom economy way. In this MCR approach, the 1,3-dipolar cycloaddition path has become an essential tool to create valuable synthetic methodologies for the synthesis of various natural products and biologically active compounds.5,6 The usual nitrogen based 1,3-dipoles are azomethine ylides, which are found to be short lived or stable intermediates.5,6
Besides, a large spectrum of cycloaddition reactions is reported on these dipoles, by using an array of activated and unactivated dipolarophiles to assemble multicyclics in a stereoselective manner.5,6 Subsequently, cyclic dipolarophiles (like cyclopropene, maleimides etc.) provides these spiro cyclic heterocyclics (Scheme 1a) with an additional ring system.6 Nevertheless, huge scope in this topic is awaited for the production of new chemical entities using multiple raw materials in one operation. Further, these approaches avoid prefunctionalizations of reactive centres, which is an obvious demand in the green synthesis7 research area. In this regard, we herein report, aforementioned coumarin as dipolarophile in azo-ylide (generated from indenoquinoxalines and isatins) cycloaddition arsenal in stereo-selective way. These hybrid (coumarin based spiro multicyclics) structural motifs may also enhance the pharmacological properties of regular spiro cyclic scaffolds2,4 (Scheme 1b).
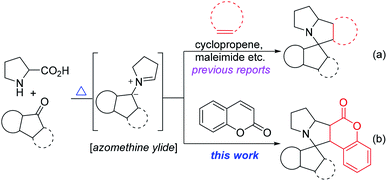 |
| Scheme 1 1,3-Dipolar cyclo-addition of azomethine ylide. | |
Results and discussion
We have optimized the reaction conditions by using indenoquinoxaline 1a, proline and coumarin 2a as reactive partners (Table 1). Initially, as a model reaction, we used catalytic amount of copper iodide (CuI) as an activator in MeCN at 60 °C and isolated the expected 3aa in 35% yield (entry 1). We further screened various solvents on this model reaction such as DMF, dioxane, toluene, methanol and the yields were observed to be improving up to 68% (entries 2–5). When we exclude the CuI in methanol, to our delight the same product was obtained in 80% yield (entry 6). Then, we have screened various solvents and temperature in absence of an activator. The best result was found in the case of the reaction in EtOH at 60 °C which provided 3aa in 89% yield (entry 7). Other solvents such as THF, IPA, iAmOH and DCE were not that effective as in the case of EtOH (entries 8–11).
Table 1 Optimized conditionsab
IPA, isopropanal, iAmOH, isoamyl alcohol. Reaction conditions: 1a (0.5 mmol), 2a (0.75 mmol) and proline (0.6 mmol) in 3 mL solvent. Isolated yields. |
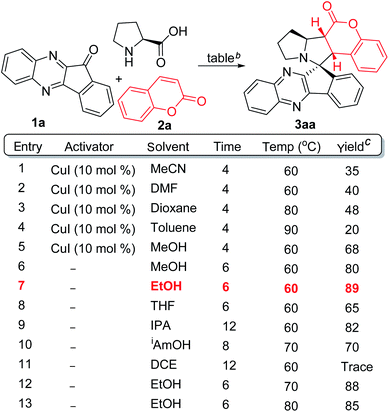 |
It is noteworthy to mention that no remarkable improvement was observed in the yield when elevated temperatures were conditioned (entries 12–13). The structure and stereochemistry of 3aa was confirmed and assigned by 1H NMR, 13C NMR and X-ray crystallography analysis (Scheme 2). Based on previous reports6 and X-ray crystal analysis, the dipole configured aza-ylide interacts with coumarin dipolarophile in endo-mode. This endo-mode approach led to a single diastereomer product in cycloaddition reaction.6b,c
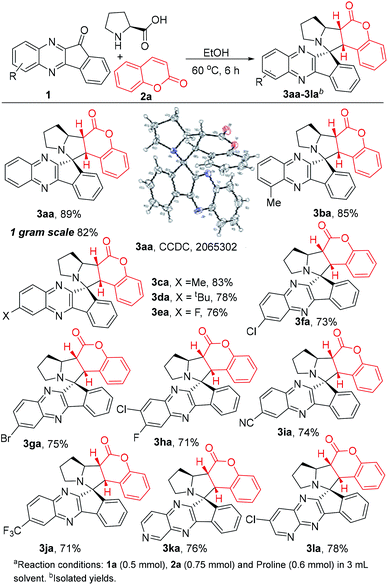 |
| Scheme 2 Scope of indenoquinoxalines. | |
With the optimized reaction conditions in hand, we have first taken up the study to generalize the substrate scope of indenoquinoxaline derivatives 1 as depicted in Scheme 2. Initially, we screened alkylated adducts 1b–d to provide the corresponding products 3ba–3da in excellent yields (78–85%). In addition, the halo substituted substrates, fluoro 1e, chloro 1f, bromo 1g and dihalo substituted substrates 1h (F and Cl) smoothly underwent the title transformation and furnished 3ea–3ha (71–76%). Also, the electron withdrawing groups, like cyano, trifluoromethyl (1i–j) substrates comfortably produced 3ia–3ja in good yields. Likewise, heterocyclic skeletons 1k and 1l showed equal ease in the reaction for the synthesis of 3ka and 3la in good yields.
Thereafter, we have investigated the substrate scope of coumarin derivatives 2 (Scheme 3). As usual, methyl 2b and chloro 2c coumarins gave corresponding adducts 3ab and 3ac in 83% and 72% yields respectively. Gratifyingly, reactive functional groups such as allyloxy- and propargyl coumarins 2d–2e were efficiently converted to expected products 3ad and 3ae in 83% and 86% yields. Worth mentioning that the other possibility of 1,3-dipolar cyclo addition (with allyloxy- and propargyl groups), indeed didn't hamper the anticipated conversion. This might be attributed to the electronic richness on these groups, which did not allow the cycloaddition with azomethine ylide. Furthermore, electron withdrawing substitutions on the 4th position of coumarin increases the reactive ability. As expected, 4-CF3 motif 2f gave final product 3af in good yield.
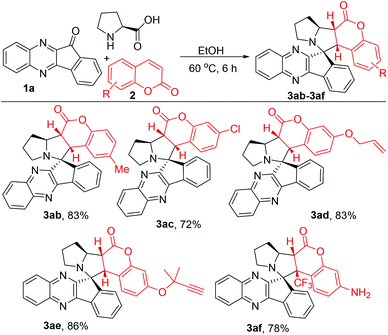 |
| Scheme 3 Scope of coumarins. | |
After establishing the applicability of coumarins as 1,3-dipolar cycloaddition partners on quinoxaline derivatives, we then quested to extend the same on the other potential heterocyclics to deliver a new class of spiro heterocyclics. At this point, we choose isatins as suitable adducts in the above discoursed cycloaddition transformation due to their wide applicability in drug discovery8 applications and comparable reactivity as indenoquinoxalines. At first, we conducted the reaction of N-methyl 4a and N-benzyl 4b isatins in optimized conditions and we have isolated 5a and 5b in 80% and 78% yield respectively (Scheme 4). The structure and stereochemistry of 5a was confirmed by X-ray analysis. Further, methyl and halo variants (F, Br and I) 4c–4f provides analogue products 5c–5f in 73–82% yields. In addition, methyl, chloro, bromo and dichloro substituted coumarins provided the corresponding products 5h–5k in good yields (72–81%).
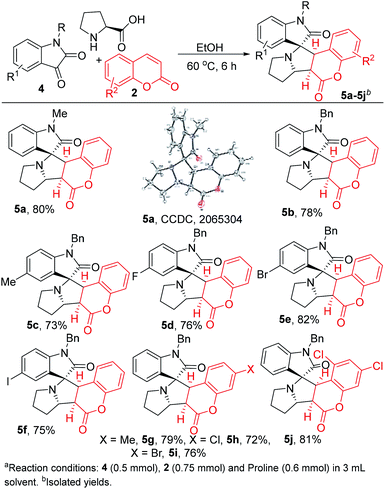 |
| Scheme 4 Scope of isatins. | |
Conversely, the synthesis of such 3D frame works was quite challenging in MCRs. The main drawback in these transformations is multiple reaction possibilities within the reactive partners. Therefore, tuning of the single reactive centered substrates is somewhat difficult.7 However, we have successfully utilized the dipolarophile behaviour of coumarins in azomethine ylide cycloaddition reactions.
Despite the dipolarophile skeleton, we further focus on the synthesis of complex spirocycles using other cyclic dipolarophiles. Therefore, we selected maleimide as cyclic dipolarophile in azomethine ylide cycloaddition. Thus, the reaction of maleimide 6a with isatin 4a offers dihydromaleimide derivative 7a in 73% (Scheme 5). We further synthesized two more derivatives 7b and 7c in good productive yields (79–82%). The structure and stereochemistry of 7b was confirmed by X-ray crystallographic analysis.
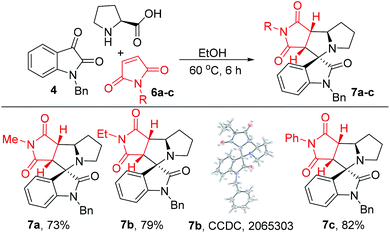 |
| Scheme 5 Scope of maleimides. | |
Conclusions
In conclusion, we have demonstrated, a formal 1,3-dipolar cycloaddition reaction of coumarins and in situ generated azomethine ylides which offers coumarin based spiro cyclic adducts in a diastereoselective manner. In situ ylides formation was achieved with indenoquinoxaline and isatin derivatives using proline. This transformation is also equally viable to maleimides for the synthesis of hydro-maleimide derivatives. This strategy delivers biologically intriguing spirocyclics in environmentally benign conditions without the addition of any activator and products were isolated by simple filtration.
Conflicts of interest
There are no conflicts to declare.
Acknowledgements
N. T. thanks CSIR for the fellowships MR thanks CSIR-IICT for facilities. We thank the Department of Analytical and Structural Chemistry at CSIR-IICT for all the analytical support and especially Dr Sridhar Balasubramanian for X-ray data. We gratefully acknowledge the overall support by CSIR-IICT [IICT/Pubs./2021/075].
Notes and references
-
(a) F. Rodriguez-Enriquez, D. Vina, E. Uriarte, R. Laguna and M. J. Matos, ChemMedChem, 2021, 16, 179–186 CrossRef CAS PubMed;
(b) A. Szappanos, A. Mandi, K. Gulacsi, E. Lisztes, B. I. Toth, T. Biro, A. Konya-Abraham, A. Kiss-Szikszai, A. Benyei, S. Antus and T. Kurtan, Biomolecules, 2020, 10, 1462–1504 CrossRef CAS PubMed;
(c) D. Cao, Z. liu, P. Verwilst, S. Koo, P. Jangjili, J. S. Kim and W. Lin, Chem. Rev., 2019, 119, 10403–10519 CrossRef CAS PubMed;
(d) M. B. Majnooni, S. Fakhri, A. Smeriglio, D. Trombetta, C. R. Croley, P. Bhattacharyya, E. Sobarzo-Sanchez, M. H. Farzaei and A. Bishayee, Molecules, 2019, 24, 4278–4297 CrossRef CAS PubMed;
(e) E. Jameel, T. Umar, J. Kumar and N. Hoda, Chem. Biol. Drug Des., 2016, 87, 21–38 CrossRef CAS PubMed;
(f) E. Jameel, T. Umar, J. Kumar and N. Hoda, Chem. Biol. Drug Des., 2016, 87, 21–38 CrossRef CAS PubMed;
(g) C. Kontogiorgis, A. Detsi and D. Hadjipavlou-Litina, Expert Opin. Ther. Pat., 2012, 22, 437–454 CrossRef CAS PubMed;
(h) M. A. Musa, J. S. Cooperwood, M. Omar and F. Khan, Curr. Med. Chem., 2008, 15, 2664–2679 CrossRef CAS PubMed.
-
(a) S.-M. Yang, C.-Y. Wang, C.-K. Lin, P. Karanam, G. M. Reddy, Y.-L. Tsai and W. Lin, Angew. Chem. Int. Ed., 2018, 57, 1668–1672 CrossRef CAS PubMed;
(b) J. Zhang and C. –S. Jiang, Med. Chem. Res., 2018, 27, 1717–1727 CrossRef CAS;
(c) L. Chen, L. Wu, W. Duan, T. Wang, L. Li, K. Zhang, J. Zhu, Z. Peng and F. Xiong, J. Org. Chem., 2018, 83, 8607–8614 CrossRef CAS PubMed;
(d) S. Emami and S. Dadashpour, Eur. J. Med. Chem., 2015, 102, 611–630 CrossRef CAS PubMed;
(e) S. S. Sahoo, S. Shukla, S. Nandy and H. B. Sahoo, Eur. J. Exp. Biol., 2012, 2, 899–908 CAS;
(f) F. Borges, F. Roleira, N. Milhazes, L. Santana and E. Uriarte, Curr. Med. Chem., 2005, 12, 887–916 CrossRef CAS PubMed.
-
(a) B. Zhao and B. Xu, Org. Biomol. Chem., 2021, 19, 568–573 RSC;
(b) Y.-J. Wang, T.-T. Wang, L. Yao, Q.-L. Wang and L.-M. Zhao, J. Org. Chem., 2020, 85, 9514–9524 CrossRef CAS PubMed;
(c) I. Cortes, L. J. Cala, A. B. J. Bracca and T. S. Kaufman, RSC Adv., 2020, 10, 33344–33377 RSC;
(d) H. J. Yoo and S. W. Youn, Org. Lett., 2019, 21, 3422–3426 CrossRef CAS PubMed;
(e) J. Hou, A. Ee, W. Feng, J. –H. Xu, Y. Zhao and J. Wu, J. Am. Chem. Soc., 2018, 140, 5257–5263 CrossRef CAS PubMed;
(f) Y. Li, Z. Qi, H. Wang, X. Fu and C. Duan, J. Org. Chem., 2012, 77, 2053–2057 CrossRef CAS PubMed;
(g) M. S. Reddy, N. Thirupathi, M. H. Babu and S. Puri, J. Org. Chem., 2013, 78, 5878–5888 CrossRef CAS PubMed , and references therein..
-
(a) W.-F. Zuo, J. Zhou, Y.-L. Wu, H.-Y. Fang, X.-J. Lang, Y. Li, G. Zhan and B. Han, Org. Chem. Front., 2021, 8, 922–927 RSC;
(b) N. S. Zimnitskiy, A. D. Denikaev, A. Y. Barkov, I. B. Kutyashev, V. Y. Korotaev and V. Y. Sosnovskikh, J. Org. Chem., 2020, 85, 8683–8694 CrossRef CAS PubMed;
(c) Y. Ji, X. He, C. Peng and W. Huang, Org. Biomol. Chem., 2019, 17, 2850–2864 RSC;
(d) Y.-L. Ji, H.-P. Li, Y.-Y. Ai, G. Li, X.-H. He, W. Huang, R.-Z. Huang and B. Han, Org. Biomol. Chem., 2019, 17, 9217–9225 RSC;
(e) A. A. Raj, R. Raghunathan, M. R. SrideviKumari and N. Raman, Bioorg. Med. Chem., 2003, 11, 407–419 CrossRef CAS PubMed.
-
(a) Y. Ling, Y. Huang and X. Li, Chem. Heterocycl. Compd., 2021, 57, 181–186 CrossRef CAS;
(b) S. S. Shinde, S. Laha, D. T. Tiwari, B. Sridhar and P. R. Likhar, Org. Biomol. Chem., 2019, 17, 4121–4128 RSC;
(c) K. S. Mani, W. Kaminsky and S. P. Rajendran, New J. Chem., 2018, 42, 301–310 RSC;
(d) A. Yu. Barkov, N. S. Zimnitskiy, I. B. Kutyashev, V. Yu. Korotaev and V. Ya. Sosnovskikh, Chem. Heterocycl. Compd., 2018, 54, 43–50 CrossRef CAS;
(e) M. S. Reddy, N. S. Kumar and L. R. Chowhan, RSC Adv., 2018, 8, 35587–35593 RSC;
(f) A. Yu. Barkov, N. S. Zimnitskiy, I. B. Kutyashev, V. Yu. Korotaev and V. Ya. Sosnovskikh, Chem. Heterocycl. Compd., 2017, 53, 1315–1323 CrossRef CAS;
(g) A. M. Akondi, S. Mekala, M. L. Kantam, R. Trivedi, L. R. Chowhan and A. Das, New J. Chem., 2017, 41, 873–878 RSC;
(h) N. Shahrestani, F. Salahi, N. Tavakoli, K. Jadidi, M. Hamzehloueian and B. Notash, Tetrahedron: Asymmetry, 2015, 26, 1117–1129 CrossRef CAS;
(i) S. Lanka, S. Thennarasu and P. T. Perumal, RSC Adv., 2014, 4, 2263–2266 RSC;
(j) V. S. Moshkin, V. Ya. Sosnovskikh and G.-V. Roschenthaler, Tetrahedron, 2013, 69, 5884–5892 CrossRef CAS;
(k) A. A. Karsalary, M. R. Mohammadizadeh, A. R. Hasaninejad, A. A. Mohammadi and A. R. Karimi, J. Iran. Chem. Soc., 2010, 7, 45–50 CrossRef CAS , and references therein..
-
(a) A. S. Filatov, S. Wang, O. V. Khoroshilova, S. V. Lozovskiy, A. G. Larina, V. M. Boitsov and A. V. Stepakov, J. Org. Chem., 2019, 84, 7017–7036 CrossRef CAS PubMed;
(b) S. Nayak, P. Pattanaik, S. Mohapatraa, D. R. Mishra, P. Panda, B. Raiguru, N. P. Mishra, S. Jena and H. S. Biswal, Synth. Commun., 2019, 49, 1823–1835 CrossRef CAS;
(c) P. R. Mali, N. B. Khomane, B. Sridhar, H. M. Meshram and P. R. Likhar, New J. Chem., 2018, 42, 13819–13827 RSC;
(d) A. S. Filatov, N. A. Knyazev, M. N. Ryazantsev, V. V. Suslonov, A. G. Larina, A. P. Molchanov, R. R. Kostikov, V. M. Boitsov and A. V. Stepakov, Org. Chem. Front., 2018, 5, 595–605 RSC;
(e) P. Pattanaik, S. Nayak, D. R. Mishra, P. panda, B. P. Raiguru, N. P. Mishra, S. Mohapatra, N. A. Mallampudi and C. S. Purohit, Tetrahedron Lett., 2018, 59, 2688–2694 CrossRef CAS;
(f) S. Kanchithalavivan, R. V. Sumesh and R. R. Kumar, ACS Comb. Sci., 2014, 16, 566–572 CrossRef PubMed.
-
(a) Y. Wu, J.-Y. Chen, J. Ning, X. Jiang, Y. Deng, R. Xu and W.-M. He, Green Chem., 2021, 23, 3950–3954 RSC;
(b) Q.-W. Gui, B.-B. Wang, S. Zhu, F.-L. Li, M.-X. Zhu, M. Yi, J.-L. Yu, Z.-L. Wu and W.-M. He, Green Chem., 2021, 23, 4430–4434 RSC;
(c) N. Meng, Y. Lv, Q. Liu, R. Liu, X. Zhao and W. Wei, Chin. Chem. Lett., 2021, 32, 258–262 CrossRef CAS;
(d) R. O. Rocha, M. O. Rodrigues and B. A. D. Neto, ACS Omega, 2020, 5, 972–979 CrossRef CAS PubMed;
(e) X. Zhang, S. Zhi, W. Wang, S. Liu, J. P. Jasinski and W. Zhang, Green Chem., 2016, 18, 2642–2646 RSC;
(f) H. C. Malinakova, Rep. Org. Chem., 2015, 5, 75–90 CrossRef CAS;
(g) R. C. Cioc, E. Ruijter and R. V. A. Orru, Green Chem., 2014, 16, 2958–2975 RSC;
(h) P. Slobbe, E. Ruijter and R. V. A. Orru, MedChemComm, 2012, 3, 1189–1218 RSC;
(i) B. B. Toure and D. G. Hall, Chem. Rev., 2009, 109, 4439–4486 CrossRef CAS PubMed.
-
(a) A. N. Izmestev, G. A. Gazieva, V. A. Karnoukhova and A. N. Kravchenko, Org. Biomol. Chem., 2020, 18, 6905–6911 RSC;
(b) Y.-C. Wang, J.-L. Wang, K. S. Burgess, J.-W. Zhang, Q.-M. Zheng, Y. D. Pu, L.-J. Yan and X.-B. Chen, RSC Adv., 2018, 8, 5702–5713 RSC;
(c) P. Ramesh, K. S. Rao, R. Trivedi, B. S. Kumar, R. S. Prakasham and B. Sridhar, RSC Adv., 2016, 6, 26546–26552 RSC;
(d) R. Sakhuja, S. S. Panda, L. Khanna, S. Khurana and S. C. Jain, Bioorg. Med. Chem. Lett., 2011, 21, 5465–5469 CrossRef CAS PubMed;
(e) B. M. Trost and M. K. Brennan, Synthesis, 2009, 18, 3003–3025 CrossRef.
Footnote |
† Electronic supplementary information (ESI) available. CCDC 2065302–2065304. For ESI and crystallographic data in CIF or other electronic format see DOI: 10.1039/d1ra05070b |
|
This journal is © The Royal Society of Chemistry 2021 |
Click here to see how this site uses Cookies. View our privacy policy here.